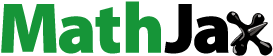
Abstract
This study aimed to study the entrapment of Enterococcus faecalis HZNU S1 using a mixture of flaxseed protein isolate (FPI) and sodium alginate (SA) as an encapsulation agent. The characteristics of free and encapsulated cells during storage and under in vitro gastrointestinal conditions were investigated. The survival rate of encapsulated cells did not decline after 2 h exposure to pH 2.5 simulated gastric juice (SGJ), while viable free probiotics treated at the same conditions, were not found. After encapsulated probiotics were exposed to SGJ pH 2.0 for 2.0 h, the viability decreased from 10.01 to 8.01 Log CFU/g. After exposure to 1.0% and 2.0% bile salt solutions for 2 h, the viable amounts of encapsulated probiotics were still higher 8.0 Log CFU/g, whereas, viable free cells were not observed after treatment at the same conditions. Encapsulated probiotics were fully released from the microbeads in simulated intestinal juice (SIJ) in around 4 h. The viable numbers of encapsulated probiotics were only reduced 0.5 Log CFU/g after two weeks of storage (4 °C), and the viable amounts of encapsulated probiotics were reduced to 8.2 Log CFU/g at 25 °C after eight days of storage. However, the viable amounts of free cells were reduced from 10.01 to 8.8 Log CFU/mL (4 °C), and 4.0 Log CFU/mL (25 °C) during the investigated storage period, respectively. Encapsulated E. faecalis HZNU S1 showed enhanced resistance to environmental conditions, allowing the delivery of probiotics that could be used to increase foods added value.
Introduction
Probiotics can be called ‘alive beneficial microorganisms that, once ingested in sufficient numbers enhance the host’s immune ability, and thus offer health benefits to the host’ [Citation1–3]. To obtain health advantages from probiotic cells, the recommended amount of probiotic bacteria in foods is at least 106 CFU/mL [Citation1, Citation4]. However, most probiotics are highly sensitive to the adverse environment in the gastrointestinal tract, for example, low pH in simulated gastric juice (SGJ) and high content of bile salt solutions, as well as during long storage periods [Citation5–7]. Therefore, the preservation of the viable numbers of probiotic cells in the gastrointestinal environment is a very crucial issue. It is necessary to develop the approaches to enhance the stability of probiotics. Among various approaches, encapsulation technology has been regarded as one of the most prospective approaches to enhance the stability of probiotics in harsh conditions [Citation8–12]. Many researches have demonstrated that encapsulation can maintain or enhance the stability of probiotics in many fermented dairy or nondairy foods [Citation13, Citation14].
It is well-known that the choice of appropriate encapsulation material is the premise of successful encapsulation [Citation5]. Sodium alginate (SA) is one of the most popular encapsulation materials due to its non-toxic nature, good gelling and bio-compatible properties [Citation12]. Although SA has been employed as a wall agent, it does not provide enough good protection to probiotics in hostile environments, for instance, low pH and high content of bile salt solutions, due to the porous structure of SA microbeads [Citation1, Citation15, Citation16]. Similarly, protein-based materials, for example, soy protein isolate, whey protein isolate, skim milk and so on, are also popular wall materials used for probiotics encapsulation due to their good gelation and film forming properties [Citation8, Citation17, Citation18]. However, they also have some limitations, such as poor gelling strength and low stability during storage. For these reasons, many studies are focused on employing the combination of SA and proteins to reduce the porosity of SA microbeads [Citation3, Citation19–21]. A study found that pea protein isolate-SA microcapsules could protect Bifidobacterium dolescentis in SGJ conditions [Citation22]. Researchers reported that the survival rate of encapsulated Lactobacillus acidophilus employing SA coated with the multilayers of whey proteins in SGJ and storage conditions, was improved [Citation23]. Flaxseed protein isolate (FPI) has various functional characteristics including emulsifying ability, water/oil adsorption ability, foaming stability. Thus, FPI shows a great potential as an encapsulation material. However, to the best of our knowledge, there are no studies using FPI, or FPI-SA for probiotics encapsulation.
The genus Enterococcus belongs to the lactic acid bacteria group, which can be found in many kinds of foods, for example sourdough, cheese, sausages, fermented vegetables [Citation24–27]. Enterococcus faecium and Enterococcus faecalis are the most investigated species from the genus Enterococcus [Citation28]. E. faecium MMRA isolated from traditional Tunisian dairy beverage ‘Rayeb’ exhibits a strong anti-Listeria activity due to the produced enterocin A [Citation29]. Mishra and Ghosh found that E. faecalis AG5 could mitigate high-fat diet-induced obesity, and produced propionic-acid-induced apoptosis in 3T3-L1 pre-adipocytes [Citation30]. In our preliminary studies, E. faecalis HZNU S1 was isolated from peacock feces, but showed poor stability after exposure to pH 2.0 and 2.5 SGJ, the bile salt solutions at 1.0 and 2.0% (Results not published). Therefore, to make better use of E. faecalis HZNU S1, in the present study, FPI-SA microbeads were prepared and were used to enhance the survival of E. faecalis HZNU S1 in harsh conditions. The viable amounts of encapsulated probiotics were determined after the incubation in pH 2.0 and 2.5 SGJ, and bile salt solutions at 1.0% and 2.0%. Additionally, the release profile of encapsulated probiotics in simulated intestinal juice (SIF) and storage stability were also studied.
Materials and methods
Preparation of probiotic bacterial culture (E. faecalis HZNU S1)
The pure culture of E. faecalis HZNU S1 was acquired from Hangzhou Normal University. It was isolated and identified from peacock faecal samples according to the study of Zheng et al. with minor modifications [Citation31]. Pure culture of E. faecalis HZNU S1 was maintained at −80 °C in MRS broth supplemented with 50% glycerol. The activation for the strain was achieved in MRS broth at 37 °C for 24 h incubation, without shaking. The activated cell culture was centrifuged at 3000×g, 4 °C for 10 min, and then washed in sterile distilled water. After that, probiotic cells were suspended in 0.85% NaCl solution. The cell suspensions were obtained and used in the subsequent studies. The final density of probiotics cells was above 1011 CFU/mL.
Encapsulation of E. faecalis HZNU S1 in FPI-SA microbeads
Flaxseed protein isolate (FPI, Hangzhou Tianlong Group Inc., Zhejiang, China; protein concentration >85%, water content <5.0%)-sodium alginate (SA, Sigma Aldrich, Shanghai) microbeads were prepared according to our previous works with slight modifications [Citation3, Citation19]. Shortly, SA and FPI solutions were prepared, and sterilized at 121 °C for 15 min and 110 °C for 10 min, respectively. SA and FPI (SA/FPI = 1:2, v/v, SA concentration 2.0%) were mixed with E. faecalis HZNU S1 culture (1011 CFU/mL). The solution of wall materials containing E. faecalis HZNU S1 was injected through a syringe (27 gauge (G) with 0.191 mm inner diameter) into a beaker containing 100 mM CaCl2. The mixture was agitated on a magnetic stirrer at 100 rpm. After being solidified for 30 min, the formed microbeads were rinsed by distilled water, and stored at 4 °C.
Determination of encapsulation efficiency
The efficiency of the microbeads for E. faecalis HZNU S1 encapsulation was studied using the methods described in our previous studies with minor modifications [Citation3, Citation20]. Briefly, 0.50 g of the microbeads was randomly selected and put into 4.5 mL sodium citrate solution (50 mmol/L) for 30 min. The amounts of released probiotics from the broken microbeads were determined by using the pour plate technique. Shortly, 100 μL of the broken microbeads suspension was added onto MRS agar plate (15% agar). The colonies were counted after the plates were cultured at 37 °C for 24 h. The encapsulation efficiency was determined by the following formula:
Measurement of microbeads size
The sizes of microbeads were determined using a digital microscope (Primotech, Carl Zeiss, Germany). Thirty microbeads were optionally selected to detect the sizes.
Determination of free probiotic numbers
Free probiotics were appropriately diluted using 0.85% NaCl solution, and 100 µL aliquots were added onto the plates with MRS agar. The numbers of free cells were determined by employing the method for the determination of encapsulation efficiency of E. faecalis HZNU S1.
Survival of free and encapsulated E. faecalis HZNU S1 in SGJ
Free and encapsulated cells in SGJ (0.20% NaCl) were evaluated through the methods reported by our group [Citation3, Citation19]. In short, 1.0 mol/L HCl was used to adjust pH. Then, 0.50 mL of the cell suspension or 0.50 g of encapsulated cells was added into 4.5 mL SGJ, and incubated at 37 °C for a specific time (10, 30, 60, 90 and 120 min). After the investigated time intervals, 100 µL of free and encapsulated cells were collected according to the above procedure for determination of free probiotic numbers and encapsulation efficiency, respectively, then diluted with 0.85% NaCl solution, and finally assayed for probiotic survival. The cell quantities were counted after MRS agar plates were cultured at 37 °C for 24 h.
Survival of free and encapsulated E. faecalis HZNU S1 in bile salt solutions
The survival of free and encapsulated cells was determined in 1.0% and 2.0% porcine bile salt solutions (Sigma-Aldrich, Shanghai, China) using the methods as described previously [Citation3, Citation20]. Briefly, both 0.50 mL of free-cell suspension and microbeads (0.50 g) were exposed to 4.5 mL bile salt solutions at 37 °C for 1 and 2 h, respectively. Free and encapsulated cells were taken out at specific time interval. Free E. faecalis HZNU S1 was determined using the above-described method for the determination of free probiotic numbers. The microbeads were disintegrated in sodium citrate solution for the viability assay of E. faecalis HZNU S1.
Release behavior of encapsulated E. faecalis HZNU S1 in SIJ
The release profile of encapsulated cells in SIJ (pH 6.8, 50 mmol/L KH2PO4) was studied by employing the reported methods with little modification [Citation3, Citation19]. Briefly, 0.50 g of microbeads was placed in 4.5 mL SIJ at 37 °C with agitating at 100 rpm. At regular time intervals (0, 10, 30, 60, 90, 120, 180, 210 and 240 min), 100 μL of probiotic suspension was removed for cell counting at an appropriate dilution with 0.85% NaCl solution. The survival of the probiotics on MRS agar plate was measured. One hundred microliters of the fresh NaCl solution were added as a replacement for the same volume of samples withdrawn.
Storage stability of free and encapsulated E. faecalis HZNU S1
To investigate the effect of storage on the viability of free and encapsulated probiotics, free and encapsulated probiotics were stored at 4 °C for 0, 2, 4, 6, 8, 10, 12, and 14 d, and 25 °C for 0, 1, 2, 4, 6, and 8 d. After pre-determined time, the survival of free and encapsulated cells was measured according to the methods for determination of free probiotic numbers and encapsulation efficiency.
Statistics analysis
Analysis of variance (ANOVA) was carried out, followed by Student’s t test (95% confidence interval), using Origin 8.0 for Windows. All the studies were carried out as independent triplicates, and the results were expressed as mean values with standard deviation (±SD).
Results
Size of microbeads
The size of the obtained microbeads varied from around 1.75–1.95 mm in diameter (mean 1.85 mm) ().
Survival of free and encapsulated E. faecalis HZNU S1 in SGJ
The results of the exposure of encapsulated probiotics to SGJ are shown in . The decrease in viable counts was faster for free cells compared to encapsulated probiotics. No viable cells were found in 30 min after the exposure of free E. faecalis HZNU S1 to pH 2.5 and 2.0 SGJ (results not shown). However, there was no reduction in the viable encapsulated cells at pH 2.5 SGJ for 2.0 h exposure. A gradual reduction in the number of encapsulated cells was observed at pH 2.0 SGJ. The probiotics encapsulated with FPI-SA were reduced from 10.01 to 8.01 Log CFU/g at pH 2.0 SGJ for an exposure time of 2.0 h.
Figure 2. Viability of encapsulated E. faecalis HZNU S1 in simulated gastric juice (SGJ) pH 2.0 and 2.5 for 0, 10, 30, 60, 90, and 120 min. Values presented are means ± standard deviations (n = 3). free (non-encapsulated) E. faecalis HZNU S1 showed no viable cells after 30 min at pH 2.5 and 2.0 SGJ (results not shown).
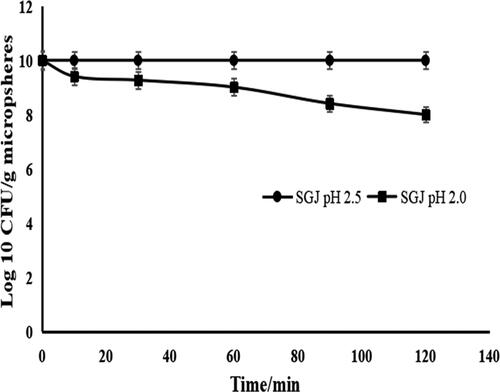
Survival of free and encapsulated E. faecalis HZNU S1 in bile salt solutions
The survival of free and encapsulated cells was investigated in 1.0 and 2.0% bile salt solutions (). After exposure to 1.0% and 2.0% bile salt solutions for 2 h (), no viable free cells could be found. However, the viable cell counts of the encapsulated formulations exceeded 8 Log CFU/g at the same conditions. These results indicated that encapsulation could protect probiotics from destruction by bile salt solutions.
Table 1. Stability of free and encapsulated E. faecalis HZNU S1 after exposure to 1.0 and 2.0% bile salt solution for 1 and 2 h (Log CFU/mL or g microbeads).
Release profile of encapsulated E. faecalis HZNU S1 in SIJ
shows the release profile of encapsulated cells after exposure to SIF. The probiotics were completely released from the microbeads at approximately 4 h, as the microbeads had completely disintegrated.
Storage stability of free and encapsulated E. faecalis HZNU S1
Aiming to evaluate the storage stability of encapsulated probiotics, the amounts of free and encapsulated cells stored at 4 or 25 °C, were determined (). The viable numbers of encapsulated probiotics at the investigated temperatures were higher than those of free probiotics. The viable numbers of free probiotics stored at refrigeration temperature (4 °C) declined from 10.01 to 9.21 Log CFU/mL after storage for eight days, and decreased to around 8.8 Log CFU/mL after storage of two weeks, whereas, the amounts of encapsulated cells decreased to 9.1 Log CFU/mL after two weeks of storage (). Likewise, the viable numbers of free cells preserved at room temperature (25 °C) declined from 10.01 to 8.20 Log CFU/mL after the storage of 4 days, and decreased to around 4.0 Log CFU/mL after the storage of eight days (). Nevertheless, the amount of encapsulated cells were decreased to 8.2 Log CFU/mL () after eight days of storage.
Discussion
Encapsulation efficiency influences the final content of probiotics in food matrices. Therefore, it is crucial to guarantee the recommended probiotic content in foods [Citation19, Citation32]. The amount of free E. faecalis HZNU S1 used for encapsulation was approximately 10 Log CFU/mL. The viable counts of probiotics in the microbeads were 9.97 Log CFU/g. In the present work, high encapsulation efficiency (≈ 100%) was obtained. Numerous parameters, for example, CaCl2 content, the nature of wall materials, the species of encapsulated probiotics, the microbeads size and the employed method, and so on, can affect the encapsulation efficiency [Citation15, Citation18]. Previous results suggested that soy protein concentrate not only provided high encapsulation efficiency to two probiotic strains of Lactobacillus, but also improved probiotic survival in harsh environment [Citation33]. High efficiency (94%) was obtained for encapsulation of Lactobacillus casei by employing SA and whey protein concentrate [Citation15]. In the present study, the high encapsulation efficiency in FPI-SA microbeads was observed due to good emulsifying and gelling properties of the mixture of FPI and SA [Citation3].
The microbeads properties, such as shape and size, are influenced by the content of the total solids, and the viscosity of the encapsulation materials [Citation2, Citation4]. The size of FPI-SA microbeads could be affected by the nature and viscosity of the encapsulating material. The combination of SA and FPI could enhance the total solids content, and thus affect the final size of the microbeads. A similar observation was demonstrated by Praepanitchai et al. who reported that the types of encapsulation materials affected the microbeads size [Citation34]. The size of the microbeads is a significant factor related to its applications in foods [Citation3, Citation19]. In this study, the size of FPI-SA microbeads was higher than 1.0 mm. Thus, the size may produce some unfavorable effects on foods texture. Smaller size of the microbeads should be obtained by using a smaller needle size.
The viability of probiotics in SGJ are of vital importance. High viability of probiotics in SGJ is one of pre-requisites for achieving the expected benefits of probiotics [Citation19, Citation20, Citation35, Citation36]. The results confirmed that encapsulation could enhance the probiotics stability in SGJ, and show protection towards probiotics exposed to SGJ. The adverse effect of SGJ to probiotics was reduced due to the buffering capacity of FPI [Citation37, Citation38]. The obtained results in the present work are consistent with several reports that demonstrated that encapsulation could preserve the expected survival of probiotic cells in SGJ [Citation3, Citation19, Citation20, Citation35, Citation36, Citation39, Citation40]. Hitherto, many studies have been reported on the use of plant protein isolate as an encapsulating material for encapsulating probiotics. For example, the viable amounts of B. adolescentis in pea protein isolate-SA microcapsules decreased 1.0 Log CFU/g in pH 2.0 SGJ at 37 °C for 2 h treatment [Citation22]. Azizi et al. reported that Lactobacillus rhamnosus encapsulated in sesame protein isolate microbeads only exhibited a decline of 1.4 Log CFU/mL after being exposed to pH 1.5 SGJ for 1 h. The reason was probably attributed to the formation of protective layer through sesame protein isolate on the microbeads [Citation39]. A study demonstrated that whey proteins as encapsulating materials could provide protection for probiotics in various harsh conditions [Citation41]. The enhanced survival of encapsulated probiotics was attributed to the buffering ability of proteins and low porous structure of the microbeads [Citation41].
The susceptibility of probiotics to bile salt solutions has been manifested in various studies [Citation19, Citation20, Citation35, Citation36, Citation42]. In our present study, free E. faecalis HZNU S1 showed poor bile salt tolerance characteristics (). Many investigations have shown that the viable amounts of probiotics in bile salt solutions can be enhanced by encapsulation technology [Citation19, Citation20, Citation35, Citation36]. E. fecium exhibited a viability of 93.3%, 91.1% and 88.8% in SA-sugar beet microbeads and 88.7%, 85% and 85% in SA-chicory microbeads in 0.3, 0.6 and 0.8 g/100 mL [Citation42] bile salt solutions, respectively. However, a significant loss of free cells was observed in their survival rate of 82%, 76%, and 70.2% in 0.3, 0.6 and 0.8 g/100 mL bile salt solutions, respectively [Citation42]. A study employed SA-soy protein isolate microbeads to encapsulate E. faecalis HZNU P2, and found that a minor viable amounts of encapsulated probiotics decreased after being treated in bile salt solutions (1.0 and 2.0%) for 1 and 2 h, whereas, the viable free probiotics were not observed at the same conditions [Citation3]. The viability of encapsulated Lactobacillus plantarum 15HN under high bile salt condition was higher than 80% [Citation43]. The reduction in the viability of encapsulated probiotics in SA microbeads was 0.97 Log CFU/g in 0.50% bile salt solution, and 1.35 Log CFU/g in 1.0% bile salt solution, respectively [Citation44]. The protective effect induced by encapsulation was more obvious after the samples were treated in 1.0% bile salt solution for 6 h. However, compared to our results, Trindade and Grosso [Citation45] did not observe a significant enhancement of viable rate when encapsulated L. acidophilus and Bifidobacterium spp. in SA–starch microcapsules were exposed to high bile salt solutions (2%–4%). In the present investigation, highly viable amounts of encapsulated cells treated with bile salt solution, were obtained, which is a pre-requisite for applications in the food industry.
Encapsulated probiotics showed a fast release profile in SIJ within 60 min. It was in favor of E. faecalis HZNU S1 colonizing the intestine, and thus may provide benefits to the host. The data was consistent with Shi et al. [Citation46]. Some other similar release profiles have been reported. Nami et al. [Citation47] reported that all encapsulated E. durans IW3 was released from the prepared beads of SA after 90 min, whereas both probiotics encapsulated in SA-gum arabic and SA-psyllium were released after 60 min. Wang et al. [Citation48] observed that about 7 Log CFU/mL and 4 Log CFU/mL of Lactobacillus plantarum from inulin-SA and SA microbeads, respectively, were liberated in SIJ after 20 min exposure. The faster release rate of encapsulated cells from inulin-SA microbeads in the first 20 min could be induced by the addition of inulin in SA to affect the binding of calcium ions [Citation48]. Klemmer et al. [Citation22] used pea protein-SA microbeads to encapsulate B. adolescentis, and showed similar probiotics release behavior in SIJ with our present study. The authors indicated that the obtained microbeads showed a great potential to deliver probiotics into SIJ [Citation22]. Shi et al. [Citation46] reported that E. faecalis HZNU P2 encapsulated within soy milk-SA microbeads was completely released in SIJ in 60 min. Similarly, E. faecalis HZNU P2 entrapped in milk-SA microbeads was also fully released in SIJ within 1 h [Citation35].
Many studies demonstrate that poor stability of free probiotics prevents their applications in the food industry. The greatest challenge of incorporating probiotics into various food matrices is to preserve their viable stability during processing, storage and product consumption [Citation49, Citation50]. The obtained results demonstrated that storage conditions exhibited an unfavorable effect on probiotics viability. FPI-SA microbeads could enhance the viable amounts of probiotics during the storage period. Many authors state that encapsulation can enhance the viable stability of probiotics, and the low storage temperature is more favorable to maintain probiotics viability due to the reduction in the metabolic activities of probiotics [Citation3, Citation49, Citation51]. Moumita et al. [Citation52] encapsulated E. faecium by alginate microcapsules with Pleurotus florida extract through lyophilization and spray-drying methods. The authors found that encapsulation could enhance probiotic survival in dry food matrix during storage; 4.0 Log CFU/g reduction in lyophilized formulation was observed, as compared to 3.0 Log CFU/g reduction in spray-dried form, after 15 weeks of storage [Citation52]. Azizi et al. [Citation39] used sesame protein isolate microcapsules treated by MTGase to entrap L. rhamnosus, and the results showed that the viable numbers in the microcapsules were not reduced significantly at 4 and 25 °C during the period of 30 days, with only around 0.40 Log CFU/g reduction. Etchepare et al. [Citation23] found that 4.0% of whey protein coated SA microbeads could enhance the viable stability of L. acidophilus at 4 °C for storage of 120 days. Likewise, Adilah et al. [Citation12] reported that the encapsulation of B. subtilis E20 by SA could significantly maintain higher viability, from 50.6% to 79.3%, compared to free probiotics during storage at 4, 25, and 37 °C, respectively [Citation12]. It is the first time that FPI-SA has been used as an encapsulation agent to protect probiotics. The present study may pave the way for the use of FPI-based encapsulating agents to produce probiotic delivery systems, and provide an opportunity to open up the probiotic market to applications beyond the dairy industry.
Conclusions
E. faecalis HZNU S1 was successfully encapsulated using a mixture of sodium alginate and flaxseed protein isolate. We observed high encapsulation efficiency, high survival rate after exposure to pH 2.0 and 2.5 SGJ, 1.0% and 2.0% bile salt solutions, and during the storage periods. A fast-release profile from the microbeads in SIJ (pH 6.8) was observed. The findings from this investigation indicate that flaxseed-based systems show great promise for the encapsulation of functional ingredients.
Author’s contributions
Z-X T, Z X, Y-J C, T-Y Z, Z-B Y and L-E S: Participated in the whole experiment process and also contributed to the interpretation of the results and manuscript preparation. Q-N M, X-Y C and H-Q C: Participated in data analysis and literature search. Z-X T and L-E S: Conceptualized this study, ameliorated the manuscript and contributed to the funding support. All authors read and approved the final manuscript.
Disclosure statement
No potential conflict of interest was reported by the authors.
Data availability statement
The experimental data supporting this study are freely available from the corresponding authors upon reasonable request.
Additional information
Funding
References
- Dong QY, Chen MY, Xin Y, et al. Alginate-based and protein-based materials for probiotics encapsulation: a review. Int J Food Sci Technol. 2013;48(7):1–10. doi: 10.1111/ijfs.12078.
- FAO. /WHO. Probiotics in food health and nutritional properties and guidelines for evaluation: report of a joint FAO/WHO expert consultation on evaluation of health and nutritional properties of probiotics in food including powder milk with live lactic acid bateria. 2006.
- Zhang Y, Zheng W, Gu JF, et al. Soy protein isolate-alginate microspheres for encapsulation of Enterococcus faecalis HZNU P2. Braz. arch. biol. technol. 2015;58(5):805–811. doi: 10.1590/S1516-89132015050260.
- Afzaal M, Khan AU, Saeed F, et al. Functional exploration of free and encapsulated probiotic bacteria in yogurt and simulated gastrointestinal conditions. Food Sci Nutr. 2019;7(12):3931–3940. doi: 10.1002/fsn3.1254.
- Frakolaki G, Giannou V, Kekos D, et al. A review of the microencapsulation techniques for the incorporation of probiotic bacteria in functional foods. Crit Rev Food Sci Nutr. 2021;61(9):1515–1536. doi: 10.1080/10408398.2020.1761773.
- Ta LP, Bujna E, Antal O, et al. Effects of various polysaccharides (alginate, carrageenan, gums, chitosan) and their combination with prebiotic saccharides (resistant starch, lactosucrose, lactulose) on the encapsulation of probiotic bacteria Lactobacillus casei 01 strain. Int J Biol Macromol. 2021;183:1136–1144. doi: 10.1016/j.ijbiomac.2021.04.170.
- Vaziri AS, Alemzadeh I, Vossoughi M, et al. Co-microencapsulation of Lactobacillus plantarum and DHA fatty acid in alginate-pectin-gelatin biocomposites. Carbohydr Polym. 2018;199:266–275. doi: 10.1016/j.carbpol.2018.07.002.
- Braber NV, Vergara LD, Rossi Y, et al. Effect of microencapsulation in whey protein and water-soluble chitosan derivative on the viability of the probiotic Kluyveromyces marxianus VM004 during storage and in simulated gastrointestinal conditions. LWT Food Sci Technol. 2020;118:108844. doi: 10.1016/j.lwt.2019.108844.
- Nualkaekul S, Lenton D, Cook MT, et al. Chitosan coated alginate beads for the survival of microencapsulated Lactobacillus plantarum in pomegranate juice. Carbohydr Polym. 2012;90(3):1281–1287. doi: 10.1016/j.carbpol.2012.06.073.
- Reque PM, Brandelli A. Encapsulation of probiotics and nutraceuticals: applications in functional food industry. Trends Food Sci Tech. 2021;114:1–10. doi: 10.1016/j.tifs.2021.05.022.
- Tolve R, Cela N, Condelli N, et al. Microencapsulation as a tool for the formulation of functional foods: the phytosterols’ case study. Foods. 2020;9(4):470. doi: 10.3390/foods9040470.
- Adilah RN, Chiu ST, Hu SY, et al. Improvement in the probiotic efficacy of Bacillus subtilis E20-stimulates growth and health status of white shrimp, Litopenaeus vannamei via encapsulation in alginate and coated with chitosan. Fish Shellfish Immunol. 2022;125:74–83. doi: 10.1016/j.fsi.2022.05.002.
- Peng M, Tabashsum Z, Anderson M, et al. Effectiveness of probiotics, prebiotics, and prebiotic-like components in common functional foods. Compr Rev Food Sci Food Saf. 2020;19(4):1908–1933. doi: 10.1111/1541-4337.12565.
- Pourjafar H, Noori N, Gandomi H, et al. Viability of microencapsulated and nonmicroencapsulated lactobacilli in a commercial beverage. Biotechnol Rep (Amst). 2020;25:e00432. doi: 10.1016/j.btre.2020.e00432.
- Afzaal M, Khan AU, Saeed F, et al. Survival and stability of free and encapsulated probiotic bacteria under simulated gastrointestinal conditions and in ice cream. Food Sci Nutr. 2020;8(3):1649–1656. doi: 10.1002/fsn3.1451.
- Martin MJ, Lara-Villoslada F, Ruiz MA, et al. Microencapsulation of bacteria: a review of different technologies and their impact on the probiotic effects. Innov Food Sci Emerg. 2015;27:15–25. doi: 10.1016/j.ifset.2014.09.010.
- Nesterenko A, Alric I, Silvestre F, et al. Vegetable proteins in microencapsulation: a review of recent interventions and their effectiveness. Ind Crop Prod. 2013;42:469–479. doi: 10.1016/j.indcrop.2012.06.035.
- Saeed F, Afzaal M, Ahmad A, et al. Enhanced viability of microencapsulated lyophilized probiotics under in vitro simulated gastrointestinal conditions. J Food Process Pres. 2022;46:e16543.
- Chen MY, Zheng W, Dong QY, et al. Activity of encapsulated Lactobacillus bulgaricus in alginate-whey protein microspheres. Braz. arch. biol. technol. 2014;57(5):736–741. doi: 10.1590/S1516-8913201402377.
- Pan LX, Fang XJ, Yu Z, et al. Encapsulation in alginate-skim milk microspheres improves viability of Lactobacillus bulgaricus in gastrointestinal conditions. Int J Food Sci Nutr. 2013;64(3):380–384. doi: 10.3109/09637486.2012.749841.
- Silva KS, Mauro MA, Goncalves MP, et al. Synergistic interactions of locust bean gum with whey proteins: effect on physicochemical and microstructural properties of whey protein-based films. Food Hydrocolloid. 2016;54:179–188. doi: 10.1016/j.foodhyd.2015.09.028.
- Klemmer KJ, Korber DR, Low NH, et al. Pea protein-based capsules for probiotic and prebiotic delivery. Int J Food Sci Tech. 2011;46(11):2248–2256. doi: 10.1111/j.1365-2621.2011.02743.x.
- Etchepare MA, Nunes GL, Nicoloso BR, et al. Improvement of the viability of encapsulated probiotics using whey proteins. LWT Food Sci Technol. 2020;117:108601. doi: 10.1016/j.lwt.2019.108601.
- Ahmadova A, Todorov SD, Choiset Y, et al. Evaluation of antimicrobial activity, probiotic properties and safety of wild strain Enterococcus faecium AQ71 isolated from azerbaijani motal cheese. Food Control. 2013;30(2):631–641. doi: 10.1016/j.foodcont.2012.08.009.
- Daba GM, El-Dien AN, Saleh SAA, et al. Evaluation of enterococcus strains newly isolated from egyptian sources for bacteriocin production and probiotic potential. Biocatal Agr Biotech. 2021;35:102058. doi: 10.1016/j.bcab.2021.102058.
- El-Ghaish S, Khalifa M, Elmahdy A. Antimicrobial impact for lactococcus lactis subsp. Lactis A15 and Enterococcus faecium A15 isolated from some traditional egyptian dairy products on some pathogenic bacteria. J Food Biochem. 2017;41(1):e12279. doi: 10.1111/jfbc.12279.
- Roselino MN, Sakamoto IK, Adorno MAT, et al. Effect of fermented sausages with probiotic Enterococcus faecium CRL 183 on gut microbiota using dynamic colonic model. LWT Food Sci Technol. 2020;132:109876. doi: 10.1016/j.lwt.2020.109876.
- Gaglio R, Couto N, Marques C, et al. Evaluation of antimicrobial resistance and virulence of enterococci from equipment surfaces, raw materials, and traditional cheeses. Int J Food Microbiol. 2016;236:107–114. doi: 10.1016/j.ijfoodmicro.2016.07.020.
- Rehaiem A, Martinez B, Manai M, et al. Production of enterocin a by Enterococcus faecium MMRA isolated from Rayeb, a traditional Tunisian dairy beverage. J Appl Microbiol. 2010;108(5):1685–1693. doi: 10.1111/j.1365-2672.2009.04565.x.
- Mishra AK, Ghosh AR. Probiotic Enterococcus faecalis AG5 mitigated high fat diet induced obesity and produced propionic acid stimulated apoptosis in 3T3-L1 pre-adipocyte. Life Sci. 2020;261:118292. doi: 10.1016/j.lfs.2020.118292.
- Zheng W, Zhang Y, Lu H, et al. Antimicrobial activity and safety evaluation of Enterococcus faecium KQ 2.6 isolated from peacock feces. BMC Biotechnol. 2015;15(1):30. doi: 10.1186/s12896-015-0151-y.
- Iqbal R, Liaqat A, Yasmin I, et al. Double layered encapsulation to immobilize Bifidobacterium bifidum ATCC 35914 in polysaccharide-protein matrices and their viability in set type yoghurt. J Food Process Pres. 2022;46:e16748.
- González-Ferrero C, Irache JM, González-Navarro CJ. Soybean protein-based microparticles for oral delivery of probiotics with improved stability during storage and gut resistance. Food Chem. 2018;239:879–888. doi: 10.1016/j.foodchem.2017.07.022.
- Praepanitchai O-A, Noomhorm A, Anal AK. Survival and behavior of encapsulated probiotics (Lactobacillus plantarum) in calcium-alginate-soy protein isolate-based hydrogel beads in different processing conditions (pH and temperature) and in pasteurized mango juice. Biomed Res Int. 2019;2019:9768152–9768158. doi: 10.1155/2019/9768152.
- Shi L-E, Li Z-H, Li D-T, et al. Encapsulation of probiotics Lactobacillus bulgaricus in alginate-milk microspheres and evaluation of survival in simulated gastrointestinal conditions. J Food Eng. 2013;117(1):99–104. doi: 10.1016/j.jfoodeng.2013.02.012.
- Shi L-E, Li Z-H, Zhang Z-L, et al. Encapsulation of Lactobacillus bulgaricus in carragenan-locust bean gum coated milk microspheres with double layer structure. LWT Food Sci Technol. 2013;54(1):147–151. doi: 10.1016/j.lwt.2013.05.027.
- Ying D, Stephanie S, Rangika W, et al. Microencapsulated lactobaciilus rhamnosus GG in whey protein and resistant starch matrices: probiotic survival in fruit juice. J Funct Foods. 2013;5(1):98–105. doi: 10.1016/j.jff.2012.08.009.
- Ye X, Xu M, Tang Z, et al. Flaxseed protein: extraction, functionalities and applications. Food Sci Tech. 2022;42:e22021.
- Azizi S, Rezazadeh-Bari M, Almasi H, et al. Microencapsulation of Lactobacillus rhamnosus using sesame protein isolate: effect of encapsulation method and transglutaminase. Food Biosci. 2021;41:101012. doi: 10.1016/j.fbio.2021.101012.
- Moayyedi M, Eskandari MH, Rad AHE, et al. Effect of drying methods (electrospraying, freeze drying and spray drying) on survival and viability of microencapsulated Lactobacillus rhamnosus ATCC 7469. J Funct Foods. 2018;40:391–399. doi: 10.1016/j.jff.2017.11.016.
- Yasmin I, Saeed M, Pasha I, et al. Development of whey protein concentrate-pectin-alginate based delivery system to improve survival of B. longum BL-05 in simulated gastrointestinal conditions. Probiotics Antimicrob Proteins. 2019;11(2):413–426. doi: 10.1007/s12602-018-9407-x.
- Sathyabama S, Ranjith Kumar M, Bruntha Devi P, et al. Co-encapsulation of probiotics with prebiotics on alginate matrix and its effect on viability in simulated gastric environment. LWT Food Sci Technol. 2014;57(1):419–425. doi: 10.1016/j.lwt.2013.12.024.
- Haghshenas B, Abdullah N, Nami Y, et al. Microencapsulation of probiotic bacteria Lactobacillus plantarum 15HN using alginate-psyllium-fenugreek polymeric blends. J Appl Microbiol. 2015;118(4):1048–1057. doi: 10.1111/jam.12762.
- Dimitrellou D, Kandylis P, Levic S, et al. Encapsulation of Lactobacillus casei ATCC 393 in alginate capsules for probiotic fermented milk production. LWT Food Sci Technol. 2019;116:108501. doi: 10.1016/j.lwt.2019.108501.
- Trindade CSF, Grosso CRF. The effect of the immobilization of Lactobacillus acidophilus and Bifidobacterium lactis in alginate on their tolerance to gastrointestinal secretions. Milchwissenschaft Milk Sci Int. 2000;55:496–499.
- Shi LE, Zheng W, Zhang Y, et al. Soy milk-based microspheres as potential carriers for the protection of Enterococcus faecalis HZNU P2. J Funct Foods. 2015;18:487–491. doi: 10.1016/j.jff.2015.08.007.
- Nami Y, Haghshenas B, Khosroushahi AY. Effect of psyllium and gum arabic biopolymers on the survival rate and storage stability in yogurt of Enterococcus durans IW3 encapsulated in alginate. Food Sci Nutr. 2017;5(3):554–563. doi: 10.1002/fsn3.430.
- Wang L, Yu X, Xu H, et al. Effect of skim milk coated inulin-alginate encapsulation beads on viability and gene expression of Lactobacillus plantarum during freeze-drying. LWT Food Sci Technol. 2016;68:8–13. doi: 10.1016/j.lwt.2015.12.001.
- Apiwattanasiri P, Charoen R, Rittisak S, et al. Co-encapsulation efficiency of silk sericin-alginate-prebiotics and the effectiveness of silk sericin coating layer on the survival of probiotic Lactobacillus casei. Food Biosci. 2022;46:101576. doi: 10.1016/j.fbio.2022.101576.
- Dimitrellou D, Kandylis P, Petrović T, et al. Survival of spray dried microencapsulated Lactobacillus casei ATCC 393 in simulated gastrointestinal conditions and fermented milk. LWT Food Sci Technol. 2016;71:169–174. doi: 10.1016/j.lwt.2016.03.007.
- Silva HL, Balthazar CF, Silva R, et al. Sodium reduction and flavor enhancer addition in probiotic prato cheese: contributions of quantitative descriptive analysis and temporal dominance of sensations for sensory profiling. J Dairy Sci. 2018;101(10):8837–8846. doi: 10.3168/jds.2018-14819.
- Moumita S, Das B, Hasan U, et al. Effect of long-term storage on viability and acceptability of lyophilized and spray-dried synbiotic microcapsules in dry functional food fomulations. LWT Food Sci Technol. 2018;96:127–132. doi: 10.1016/j.lwt.2018.05.030.