ABSTRACT
Background
MiRNAs are closely related to tumor radiosensitivity. MiR-378a-5p level is down-regulated in colorectal cancer (CRC). Therefore, this study intends to explore the role of miR-378a-5p in CRC, especially radiosensitivity.
Methods
The expression of miR-378a-5p was analyzed in CRC samples. CRC cell lines were treated with different doses of X-rays. Bioinformatics analysis, dual-luciferase reporter assay and RT-qPCR were used to detect the expressions and binding relationship of miR-378a-5p and low-density lipoprotein receptor-related protein 8 (LRP8). MiR-378a-5p inhibitor or/and siLRP8 were transfected into CRC cells with or without irradiation. Subsequently, clonogenic assay, flow cytometry and in vivo experiments including tumorigenesis assay, immunohistochemistry, RT-qPCR and Western blot were performed to clarify the role of miR-378a-5p/LRP8 axis in the radiosensitivity of CRC.
Results
The down-regulated expression of miR-378a-5p in CRC is related to histological differentiation and tumor-node-metastasis (TNM) stage. After irradiation, the survival fraction of CRC cells was decreased, while the apoptotic rate and the level of miR-378a-5p were increased. Restrained miR-378a-5p repressed apoptosis and apoptosis-related protein expressions, yet promoted the proliferation and the radioresistance of cells by regulating β-catenin in CRC cells. LRP8 was highly expressed in CRC, and targeted by miR-378a-5p. SiLRP8 improved radiosensitivity and reversed the effect of miR-378a-5p down-regulation on CRC cells. Overexpressed miR-378a-5p and irradiation enhanced the level of miR-378a-5p, yet suppressed the expressions of Ki67 and LRP8 as well as tumorigenesis.
Conclusion
MiR-378a-5p may exert a radiosensitizing effect on CRC through the LRP8/β-catenin axis, which may be a new therapeutic target for CRC radioresistance.
Introduction
Colorectal cancer (CRC) is the fourth most deadly cancer in the world,Citation1 greatly threatening human health, and its early diagnosis and early treatment have attracted more and more attention.Citation2,Citation3 Radiotherapy is an important part of the comprehensive treatment of CRC, especially for patients at an advanced stage.Citation4,Citation5 However, about one-third of CRC patients show low sensitivity or complete resistance to radiotherapy.Citation6,Citation7 The occurrence of radioresistance is a complex process, including DNA damage, abnormal activation of certain signal pathways, angiogenesis and autophagy.Citation7,Citation8 Therefore, exploring the molecular mechanism of radiosensitivity regulation and identifying new early diagnostic or therapeutic targets can help improve the cure rate of CRC patients.
In the radiotherapy of CRC, radioresistance is a challenging topic. Many studies have tried to find biomarkers related to CRC radiotherapy sensitivity to better select patients, including lncRNA regulators of reprogramming (ROR), P53 and lincRNA-p21.Citation9,Citation10 Elucidating the molecular mechanism of radiosensitivity or radioresistance can help improve the effectiveness of CRC treatment. Some studies have shown that miRNAs can increase or decrease tumor radiosensitivity.Citation11,Citation12 For example, miR-145 can be used as a target to reverse snail family transcriptional repressor 1 (SNAI1)-mediated stemness and radioresistance in CRC.Citation13
MiR-378a-5p plays a tumor-suppressing role in a variety of cancers.Citation14,Citation15 Notably, miR-378a-5p is lowly expressed in renal cell carcinoma (RCC), and its overexpression can inhibit the malignant progression of RCC cells.Citation14 In choriocarcinoma cell line BeWo, miR-378a-5p is found to inhibit the differentiation of syncytiotrophoblast by reducing the expression of clinical significance of cyclin G2 (CCNG2).Citation15 Importantly, miR-378a-5p level is down-regulated in CRC and is related to the prognosis of CRC,Citation16 but there is still a lack of research on the mechanism of miR-378a-5p in CRC, and its role in the radioresistance of CRC cells is still unknown. In view of this, our study is committed to deciphering the effect of miR-378a-5p on the radioresistance of CRC cells and probing into its underpinning mechanism.
In addition, it has been found that the radioresistance of CRC cells is linked to the Wnt/β-catenin pathway.Citation17 A prior study has pointed out that low-density lipoprotein receptor-related protein 8 (LRP8) silencing can inhibit Wnt/β-catenin in breast cancer.Citation18 However, the mechanism of LRP8 and its radioresistance to tumor cells in CRC remain elusive. In light of this, our study is designed to investigate whether miR-378a-5p regulates the radioresistance of CRC cells by targeting LRP8 to regulate the Wnt/β-catenin pathway via performing cell function assays and in vivo experiments. The intention of this study is to clarify and enrich the mechanism of miR-378a-5p in modulating the radioresistance of CRC cells.
Results
The expression of miR-378a-5p in CRC was declined
Seventy pairs of CRC clinical samples were collected to detect the abnormal expression of miR-378a-5p, and it was found that the expression of miR-378a-5p in CRC tissues was remarkably lower than in normal tissues (p < .001, ). According to the median value of patients’ expression data (), the patients were divided into high expression group (n = 35) and low expression group (n = 35). The low expression of miR-378a-5p was related to histological differentiation and tumor-node-metastasis (TNM) stage (p < .05, ). Through in vitro cell experiments, it was found that the expression of miR-378a-5p was markedly higher in CCD-18Co cell lines as compared with that in HCT116, HCT-15, SW480, SW-620, and LOVO cell lines (p < .001, ). Given that HCT116 and LOVO cells presented the highest and the lowest expression of miR-378a-5p among CRC cell lines, respectively, they were selected for subsequent experiments.
Figure 1. The expression of miR-378a-5p in CRC was declined (a) the expression of miR-378a-5p in CRC and adjacent tissues (n = 70) was determined by RT-qPCR. (b) The expression of miR-378a-5p in normal colon cell line CCD-18Co and CRC cell lines (HCT116, HCT-15, SW480, SW-620, and LOVO) was detected by RT-qPCR. Results were expressed as the mean ± SD. Each experiment was independently repeated in triplicate. CRC: colorectal cancer; RT-qPCR: real-time quantitative PCR. U6 was employed as internal control. ***p < .001 vs. Normal; ^^^p < .001 vs. CCD-18Co.
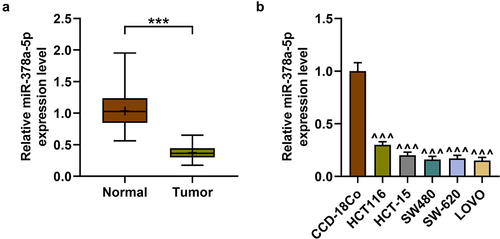
Table 1. The relationship between miR-378a-5p expression and clinical characteristics.
Effects of irradiation on the proliferation, apoptosis and miR-378a-5p level in CRC cells
Reducing the radioresistance of tumor cells is of great significance to the treatment and prognosis of patients. In the early stage, the expression of miR-378a-5p in CRC was found to be reduced, but whether it was related to radioresistance was unclear. Subsequently, irradiation was applied to construct CRC cells with radioresistance. After treatment with 2, 4, or 6 Gy of irradiation, the survival fraction of CRC cells was observed to be declined, while the apoptotic rate was clearly increased (p < .05, ). Similarly, the level of miR-378a-5p was pronouncedly up-regulated after irradiation treatment (p < .01, ), suggesting that miR-378a-5p may have a synergistic effect with irradiation. In addition, the above experiment results showed that 4 Gy of irradiation had an effect of considerably reducing cell proliferation and increasing apoptosis, but 6 Gy and 4 Gy of irradiation showed no significant difference, so 4 Gy of irradiation was selected for subsequent experiments (p < .001, ).
Figure 2. Effects of irradiation on the proliferation, apoptosis and miR-378a-5p level in CRC cells. (a-b) HCT116 and LOVO cells were treated with 2, 4, or 6 of gy irradiation, and clonogenic experiment was used to detect cell survival fraction (%). (c-d) the apoptotic rate of CRC cells treated with 2, 4, or 6 of gy irradiation was evaluated by flow cytometry. (e-f) RT-qPCR was carried out to detect the level of miR-378a-5p in irradiation-treated CRC cells. U6 was employed as the internal control. *p < .05, **p < .01, ***p < .001 vs 0 gy.
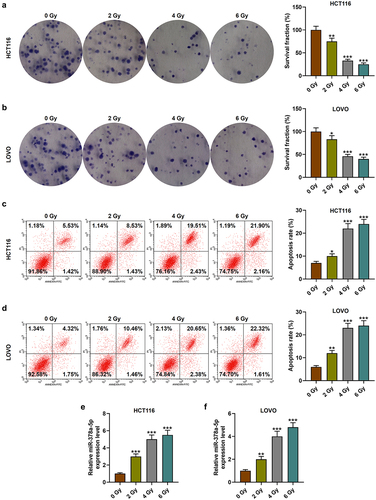
Restrained miR-378a-5p may promote the proliferation yet reduce the apoptosis of CRC cells after irradiation by regulating β-catenin
To further verify our conjecture, miR-378a-5p inhibitor was transfected into HCT116 and LOVO cells with or without irradiation (p < .001, ). MiR-378a-5p inhibitor promoted the proliferation of CRC cells and partially reversed the anti-proliferation ability of irradiation (p < .05, ). Conversely, miR-378a-5p inhibitor reduced CRC cell apoptosis and partially reversed the pro-apoptotic ability of irradiation (p < .001, ). It has been reported that the chemoradiotherapy resistance of CRC cells is related to the enhancement of Wnt/β-catenin pathway.Citation17 Therefore, we detected the level of β-catenin in CRC cells and found that irradiation reduced the level of β-catenin, while miR-378a-5p inhibitor greatly increased the expression of β-catenin and the radioresistance of CRC cells (p < .001, ).
Figure 3. The effect of restrained miR-378a-5p on the proliferation and apoptosis of CRC cells after irradiation. (a-b) CRC cells were treated with 0 or 4 gy of irradiation. RT-qPCR was performed to determine miR-378a-5p level after miR-378a-5p inhibitor transfection. (c-d) the survival fraction (%) of CRC cells after irradiation and transfection was tested by clonogenic experiment. (e-f) after treatment with 0 or 4 gy of irradiation, the effect of miR-378a-5p inhibitor on CRC cell apoptosis was evaluated by flow cytometry. IC: inhibitor control; I: inhibitor. *p < .05, ***p < .001 vs IC.
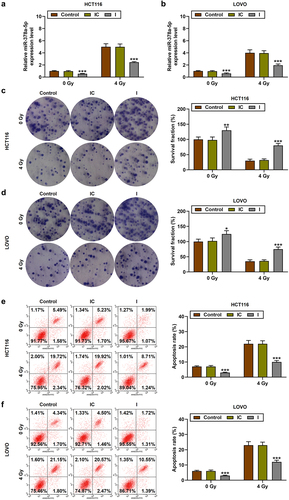
Figure 4. The effect of restrained miR-378a-5p on the β-catenin protein expression in CRC cells after irradiation. (a-b) CRC cells were treated with 0 or 4 of gy irradiation, and the β-catenin protein level in the control, IC, and I groups was evaluated by Western blot. β-actin served as the internal control. ***p < .001 vs IC.
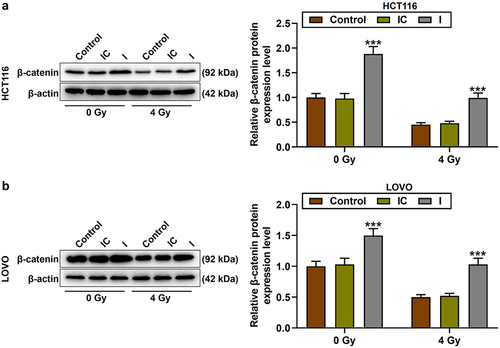
LRP8 was highly expressed in CRC and targeted by miR-378a-5p
To further study the intermediate genes of miR-378a-5p regulating β-catenin level, TargetScan v7.2 was used for bioinformatics analysis. LRP8 was found to bind to miR-378a-5p, and this result was verified by dual-luciferase reporter assay (p < .001, ). LRP8 exhibited a notably high expression in colon adenocarcinoma (COAD) and rectum adenocarcinoma (READ) (p < .05, ). Likewise, the expression of LRP8 was detected to be greatly increased in CRC cell lines (p < .001, ).
Figure 5. LRP8 was targeted by miR-378a-5p. (a) TargetScan v7.2 website (http://www.Targetscan.org/vert_72/) was applied to predict the binding sites between LRP8 and miR-378a-5p. (b-c) after the wild-type and mutant luciferase reporter plasmids of LRP8 3’UTR were constructed, the dual-luciferase reporter assay was used to verify the targeting relationship between LRP8 and miR-378a-5p. (d) The expression of LRP8 in READ and COAD was analyzed by GEPIA website (http://gepia2.Cancer-pku.Cn/#index), p < .05. (e) RT-qPCR was employed to determine the expression of LRP8 in normal colon cell line CCD-18Co and CRC cell lines (HCT116, HCT-15, SW480, SW-620, and LOVO). β-actin was employed as the internal control. LRP8: low-density lipoprotein receptor-related protein 8; rectum adenocarcinoma (READ); colorectal adenocarcinoma(COAD); MC: mimic control. +++p < .001 vs MC; ^^^p < .001 vs CCD-18Co.
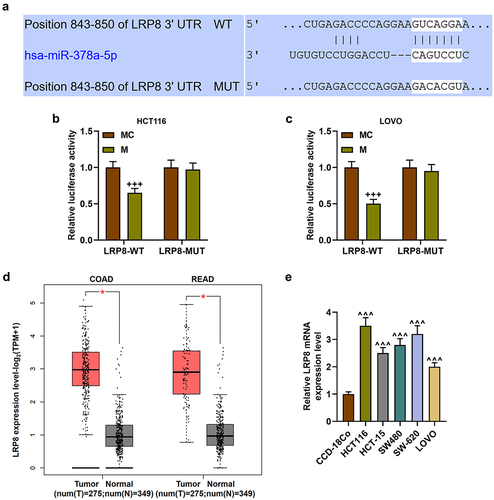
MiR-378a-5p regulated the radiosensitivity of CRC cells by targeting LRP8 to regulate the β-catenin pathway
Small interfering RNA (siRNA) targeting LRP8 (siLRP8) was successfully transfected into HCT116 and LOVO cells, as evidenced by the markedly reduced mRNA and protein levels of LRP8 (p < .001, ). Then, CRC cell lines were subjected to co-transfection and irradiation treatment. The results showed that compared with 0 Gy of irradiation, 4 Gy of irradiation significantly inhibited LRP8 level; siLRP8 dramatically diminished LRP8 level and enhanced the sensitivity of CRC cells to irradiation; and miR-378a-5p inhibitor, on the contrary, partially reversed the effect of siLRP8 on LRP8 level (p < .001, ). SiLRP8 was found to inhibit cell proliferation, promote cell apoptosis, partially offset the effects of miR-378a-5p inhibitor on the above functions in CRC cells, and enhance the sensitivity of CRC cells to irradiation (p < .001, ).
Figure 6. SiLRP8 reduced the level of LRP8 that had been raised by miR-378a-5p inhibitor. (a-d) after the transfection of siLRP8, the mRNA and protein levels of LRP8 in CRC cells were assessed by RT-qPCR and Western blot. (e-h) after treatment of CRC cells with 0 or 4 gy of irradiation, RT-qPCR and Western blot were performed to detect the effects of siLRP8 and miR-378a-5p inhibitor on LRP8 levels. β-actin was employed as the control. SiLRP8: small interfering RNA targeting LRP8; NC: negative control. +++p < .001 vs siNC; ***p < .001 vs IC + siNC; ^^^p < .001 vs IC + siLRP8; ###p < .001 vs I + siLRP8.
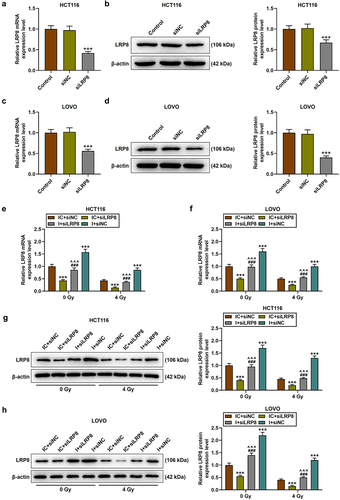
Figure 7. SiLRP8 partially offset the effects of miR-378a-5p inhibitor on the proliferation and apoptosis of CRC cells after irradiation. (a-b) after treatment with 0 or 4 gy of irradiation, the survival fractions (%) in the IC + siNC, IC + siLRP8, I + siLRP8, and I + siNC groups were analyzed by clone formation assay. (c-d) the apoptotic rate in each group was evaluated by flow cytometry. ***p < .001 vs IC + siNC; ^^^p < .001 vs IC + siLRP8; ###p < .001 vs I + siLRP8.
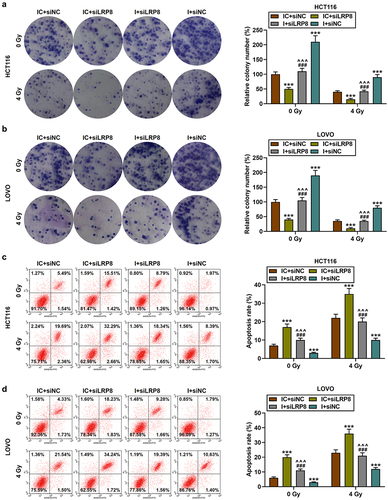
Mechanically, siLRP8 down-regulated the expression of β-catenin and partially suppressed the up-regulation of β-catenin caused by miR-378a-5p inhibitor (p < .01, ). 4 Gy of irradiation promoted Bax level yet inhibited Bcl-2 level relative to 0 Gy of irradiation; miR-378a-5p knockdown reduced the protein level of Bax, but increased the level of Bcl-2; siLRP8 had the opposite effect, partially reversing the impact of miR-378a-5p knockdown upon apoptosis-related proteins and enhancing the role of irradiation in CRC cells (p < .05, ).
Figure 8. SiLRP8 partially offset the effects of miR-378a-5p inhibitor on the protein expressions of β-catenin, Bax and bcl-2 in CRC cells after irradiation. (a-b) after treatment with 0 or 4 of gy irradiation, the protein level of β-catenin in the IC + siNC, IC + siLRP8, I + siLRP8, and I + siNC groups was evaluated by Western blot. (c-d) Western blot was performed to determine the protein expressions of Bax and bcl-2 in CRC cells. β-actin was employed as the internal control. **p < .01, ***p < .001 vs IC + siNC; ^^p < .01, ^^^p < .001 vs IC + siLRP8; #p < .05, ###p < .001 vs I + siLRP8.
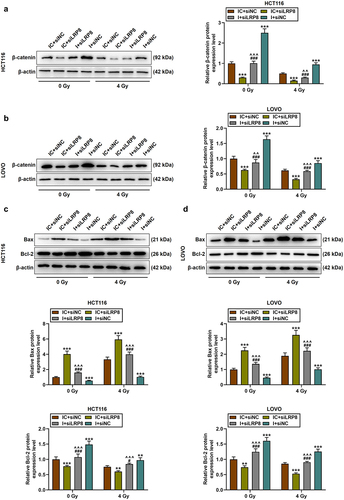
MiR-378a-5p enhanced the radiosensitivity of CRC yet reduced tumorigenesis through LRP8
Both the high expression of miR-378a-5p and irradiation reduced tumor size and tumor weight, and the high expression of miR-378a-5p further enhanced the radiosensitivity of tumor cells (p < .01, ). Then, overexpression of miR-378a-5p and irradiation were found to inhibit the level of representative proliferation marker Ki67, and high expression of miR-378a-5p enhanced the effect of irradiation on the level of ki67 (). In addition, RT-qPCR and Western blot results showed that agomir-miR-378a-5p or irradiation alone increased the level of miR-378a-5p, yet decreased the level of LRP8, while more stronger regulatory effects were induced by the combined use of the two (p < .01, ).
Figure 9. MiR-378a-5p enhanced the radiosensitivity of CRC cells and reduced tumorigenesis through LRP8. (a-c) the effects of irradiation treatment and overexpressed miR-378a-5p on tumor formation were tested by nude mouse tumorigenesis experiment. (d) Immunohistochemistry was used to detect the level of Ki67 in the control, agomir-miR-378a-5p, IR (irradiation), and IR + agomir-miR-378a-5p groups (magnification: ×200, scale bar = 50 μm). (e) The level of miR-378a-5p in each group was analyzed by RT-qPCR. U6 was employed as the internal control. (f) Western blot was exploited to evaluate the protein level of LRP8 in each group. β-actin was employed as the internal control. **p < .01, ***p < .001 vs Control; ^^p < .01, ^^^p < .001 vs agomir-miR-378a-5p; ##p < .01, ###p < .001 vs IR.
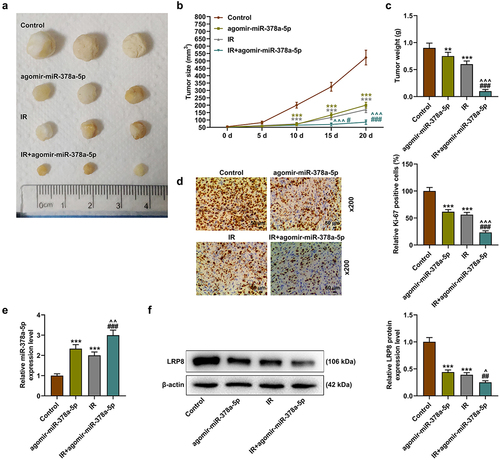
Discussion
CRC is a relatively common malignant tumor of the digestive tract, and many miRNAs are related to the malignant biological behavior and drug resistance of CRC tumors.Citation1,Citation11,Citation12 Our study found that miR-378a-5p is under-expressed in CRC and is related to the clinicopathological characteristics. Both in vivo and in vitro experiments have proved that miR-378a-5p attenuates tumor formation and inhibits the malignant progression of cancer cells, suggesting that miR-378a-5p may be a potential therapeutic target in CRC. At present, radiotherapy is an important part of comprehensive treatment of CRC.Citation4,Citation5 In this study, we aimed to uncover the effect of miR-378a-5p on the radioresistance of CRC cells and its mechanism. Thus, CRC cell lines were subsequently treated with irradiation. It was found that irradiation treatment could significantly reduce the proliferation of CRC cells and induce apoptosis. Interestingly, after irradiation, the level of miR-378a-5p in CRC cells showed a very significant upward trend, suggesting that miR-378a-5p may play a role in the radiosensitivity of CRC cells.
The role of miRNAs in affecting the radiosensitivity of tumors is currently a new research focus.Citation19 It has been confirmed that miRNA-214, miR-20a, miR-185 and other miRNAs can regulate the proliferation and apoptosis of various tumor cells, and participate in the regulation of tumor radiotherapy sensitivity.Citation20–24 In addition, downregulated miR-1 in CRC is able to promote radioresistance and aggressive phenotypes,Citation25 while miR-124 up-regulation enhances the radiosensitivity of CRC cells by targeting paired-related homeobox 1 (PRRX1).Citation26 In our study, CRC cells with or without irradiation treatment were compared. It turned out that miR-378a-5p inhibitor significantly enhanced the radioresistance of CRC cells. Contrarily, overexpression of miR-378a-5p further strengthened the effect of irradiation on inhibiting tumors during in vivo experiments, suggesting that up-regulation of miR-378a-5p can be used as a therapeutic target to enhance radiosensitivity, providing new ideas for personalized treatment.
Delving into the molecular mechanism of miR-378a-5p in CRC can better explain its radiosensitizing effect. A number of studies have shown that miR-378a-5p specifically binds to the downstream target mRNA at 3’ untranslated regions (3’UTRs) to inhibit the process of translation, thereby regulating its downstream pathways and ultimately participating in cell biological behaviors.Citation27,Citation28 LRP8 is one of the target genes of miR-378a-5p, and it is highly expressed in CRC, signifying that miR-378a-5p may mediate the malignant phenotype and radioresistance of CRC through LRP8. LRP8 has been found to be a target for cancers including breast cancer and colon cancer.Citation29,Citation30 Also, LRP8 acts as a positive regulator of Wnt/β-catenin signaling, participating in osteogenic differentiation.Citation31 Moreover, LRP8 reduction partially regulates the degradation of β-catenin in colon cancer cells.Citation30 Interestingly, in oral squamous cell carcinoma, miR-378a-5p activates Wnt/β-catenin signaling pathway through KLK4.Citation32 In this study, we found that miR-378a-5p inhibitor boosted β-catenin level, while diminishing the expression of LRP8, suggesting that miR-378a-5p may regulate β-catenin pathway through LRP8 to play an anti-tumor role in CRC.
The Wnt/β-catenin signaling pathway is one of the classic signaling pathways that regulate the occurrence and development of various tumors including CRC.Citation33 In addition, many studies have shown that the Wnt/β-catenin signaling pathway can be targeted by multiple genes, such as LIG4, miR-532-3p, miR-148a and WNT10b, to mediate the radiosensitivity of CRC.Citation24,Citation34,Citation35 Besides, we also discovered that LRP8 silencing enhanced the radiosensitivity of CRC cells and suppressed β-catenin level. Combined with previous studies, we have reason to speculate that miR-378a-5p may play a role in radiosensitivity in CRC cells through LRP8/β-catenin.
In summary, up-regulation of miR-378a-5p in CRC is related to the prognosis of patients and inhibits the malignant progression of cancer cells. In addition, up-regulated miR-378a-5p can increase the radiosensitivity of CRC cells by down-regulating the expression of LRP8 to decline β-catenin level. The radioresistance of miR-378a-5p inhibitor to CRC cells can be partially but not completely eliminated by LRP8 silencing. Namely, the radiosensitizing effect of miR-378a-5p is only partially mediated by LRP8/β-catenin. There are other pathways mediating this effect, which needs to be probed in the near future. All in all, miR-378a-5p may become a promising target to treat CRC by enhancing the radiosensitivity.
Materials and methods
Ethics statement and tissues
70 tumor tissues and adjacent normal tissues were collected from individuals who had signed the written informed consent. The present study was approved by the Ethics Committee of Qingyuan People’s Hospital (EAR201906002). The relationship between miR-378a-5p expression and clinicopathological data of CRC patients was analyzed, as shown in .
Cell lines and culture conditions
Normal colon cell line CCD-18Co (CRL-1459) and CRC cell lines HCT116 (CCL-247), HCT-15 (CCL-225), SW480 (CCL-228), SW-620 (CCL-227) and LOVO (CCL-229) were purchased from American Type Culture Collection (ATCC) (USA). Cells were maintained in high-glucose Dulbecco’s Modified Eagle Medium (DMEM) (11965092, Gibco, USA) supplemented with 10% fetal bovine serum (FBS) (16140063, Gibco, USA) and were placed in a 5% CO2 incubator (Steri-Cycle i250, Thermo Scientific, USA) at 37°C.
Transfection and irradiation treatment
MiR-378a-5p inhibitor (I) (miR20000731-1-5) and inhibitor control (IC) (miR2N0000002-1-5) were bought from RIBOBIO (China). The negative control (NC) of siRNA (siNC) (A06001) and siLRP8 (A09003) (TTGCGAAAAGGACCAATTCCAGT) were synthesized from GenePharma Company (China). MiR-378a-5p inhibitor or inhibitor control was transfected into HCT116 and LOVO cells using Lipofectamine 3000 reagent (L3000015, Invitrogen, USA), whilst siLRP8 or siNC was transfected into HCT116 and LOVO cells using Lipofectamine RNAiMAX transfection reagent (13778500, Invitrogen, USA). 24 h after transfection, CRC cells were irradiated once with 0, 2, 4, or 6 Gy of X-rays (200 kV, 10 mA, 1 Gy per 66 s, Model X-RAD 320, Precision X-ray, USA),Citation36 and the cell culture continued for 48 h following irradiation.
Real-time quantitative PCR (RT-qPCR)
The total RNAs from cultured cells or tumor tissues were extracted using the Triquick Reagent (R1100, Solarbio, China). Reverse transcription was performed according to the supplied protocol of cDNA Synthesis Kit (D7170, Beyotime, China) or miRNA first-strand cDNA synthesis kit (KR211, TIANGEN, China). Thereafter, real-time PCR was performed in RT-PCR system (4485695, Applied Biosystems, USA) using SYBR Green kit (FP313, TIANGEN, China). The relative expression levels were determined using 2−ΔΔCt method,Citation10 with β-actin or U6 serving as housekeeping genes. Primers were as follows. MiR-378a-5p: CTGACTCCAGGTCCTGTGTA, TATCCAGTGCGTGTCGTGGA; U6: CTCGCTTCGGCAGCACATATACT, ACGCTTCACGAATTTGCGTGTC; LRP8: CTGTGGCGACATTGATGAGTG, TCTTGGTCAGTAGGTCCATCTC; β-actin: CATGTACGTTGCTATCCAGGC, CTCCTTAATGTCACGCACGAT.
Clonogenic assay
Clonogenic assay was conducted in HCT116 and LOVO cells (approximately 1 × 10Citation5/well) with transfection or/and irradiation treatment to detect survival fraction (%). CRC cells maintained in the complete medium were placed in 6-well culture plates and incubated for about 10 days. After that, the colonies were fixed by methanol (322415, Sigma-Aldrich, USA), reacted with 0.5% crystal violet (C8470, Solarbio, China) for 20 minutes (min), and eventually recorded using a light microscope (Nikon, Japan).
Cell apoptosis assay
The apoptosis of HCT116 and LOVO cells was determined using Cell Apoptosis Analysis Kit (C1062, Beyotime, China). Transfected or/and irradiated cells (1 × 10Citation5/well) were stained with 5 μL of Annexin V-FITC and 10 μL of propidium iodide solution at room temperature for 10 min in the dark. Lastly, cell apoptotic rate was quantified using a CytoFLEX flow cytometer (Beckman Coulter, USA).
Bioinformatics assay and dual-luciferase reporter assay
The expressions of LRP8 in COAD and READ were predicted using GEPIA 2 (Gene Expression Profiling Interactive Analysis) (http://gepia2.cancer-pku.cn/#index). TargetScan v7.2 (http://www.targetscan.org/vert_72/) was used to analyze the target relationship between LRP8 and miR-378a-5p.
HCT116 and LOVO cells (3 × 10Citation4/well) were incubated for 24 h and then transfected with the pmirGLO recombinant vector (5001, Tiandz, China) containing wild-type (WT) or mutated (MUT) LRP8 sequences. Thereafter, miR-378a-5p mimic (M) (miR10000731-1-5, RIBOBIO, China) or mimic control (MC) (miR1N0000002-1-5, RIBOBIO, China) was transfected into the HCT116 or LOVO cells using Lipofectamine 3000 reagent. After 48 h of transfection, relative luciferase activities of CRC cells were assessed using dual-luciferase detection kits (30005–1, Biotium, USA).
Western blot
HCT116 and LOVO cells were suspended in RIPA lysis buffer (PC901, Biomiga, USA) to extract total proteins. Protein concentrations were quantified by BCA assay kit (AO45-3-2, Jiancheng, China). After that, proteins were resolved with 10% sodium dodecyl sulfate-polyacrylamide gel and electrophoretically transferred onto polyvinylidene fluoride membranes (2215, Millipore, USA). The membranes were subsequently blocked in 5% nonfat milk and then hybridized with specific antibodies (Abcam, UK). Primary antibodies were detailed as follows: Bax (ab32503, 21 kDa, 1 μg/ml); Bcl-2 (ab59348, 26 kDa, 2 μg/ml); β-Catenin (ab32572, 92 kDa, 0.2 μg/ml); LRP8 (ab204112, 106 kDa, 2 μg/ml). The information of secondary antibodies (Abcam, UK) was listed below: goat anti-rabbit IgG (ab205718, 0.2 μg/ml) and goat anti-mouse IgG (ab205719, 0.2 μg/ml). Ultimately, protein bands were visualized with BeyoECL Moon ECL reagent (P0018FS, Beyotime, China) and gel imaging system (A44114, Invitrogen, USA). β-actin (ab8226, 42 kDa, 1 µg/mL, Abcam, UK) was used as the internal control.
Tumorigenesis assay
All animal experiments were approved by the Animal Care and Use Committee of Qingyuan People’s Hospital (ODR202005021), and conducted in accordance with the guidelines of the China Council on Animal Care and Use. The pain and discomfort to the animals were minimized. BABL/c nude mice (female, 4–6 weeks old) (401) purchased from Charles River (China) were maintained in a specific pathogen-free animal room with controlled temperature and humidity. Mice were divided into the following groups with random number table method: Control group (n = 8), irradiation group (IR) (n = 8), agomir-miR-378a-5p group (n = 8) and IR + agomir-miR-378a-5p group (n = 8). Determination of the sample size in each group referred to the previous study.Citation37 A total of 5 × 10Citation6 HCT116 cells in 200 μL phosphate-buffered saline (PBS) (P1032, Solarbio, China) were subcutaneously injected into the mice from Control group. HCT116 cells pre-transfected with agomir-miR-378a-5p (miR40000731-4-5, RIBOBIO, China) in 200 μL PBS were subcutaneously injected into the mice from agomir-miR-378a-5p group or IR + agomir-miR-378a-5p group. When the tumor volume reached about 50–100 mm3 (the smallest tumor grew to 50 mm3), the mice in IR group and IR + agomir-miR-378a-5p group were exposed to 2 Gy of X-rays (1.53 Gy/min, 300 kV, filter: 0.5 mm Cu, 10 mA, 5-mm tissue depth, twice a day) per day until 20 days.Citation38 The tumor volumes were measured with calipers every 5 days. After that, the mice were sacrificed by excessive anesthesia using pentobarbital sodium (150 mg/kg, P0500000, Merck, Germany). Then, the tumor was exposed, weighed and stored for further analysis.
Immunohistochemistry (IHC)
CRC tumor tissues were embedded in paraffin (1496904, Merck, Germany). Sections (4 μm thick) were routinely deparaffinized, rehydrated, and heated in sodium citrate buffer (C9999, Sigma-Aldrich, USA). Next, sections were sealed with goat serum (G9023, Sigma-Aldrich, USA) and then incubated with anti-Ki67 antibody (Abcam, UK) in the dilution of 1 : 150 at 4°C for 12 h, followed by incubation with goat anti-rabbit IgG (1/2000). Furthermore, sections were stained with DAB substrate kit (ab64238, Abcam, UK) and analyzed under a microscope (magnifications 200×).
Statistical analysis
All results from at least triplicate experiments were expressed as mean ± standard deviation. The data analysis was carried out using SPSS 20.0 (IBM, USA). Comparisons between two groups were evaluated using independent-samples t test. Paired-samples t-test was used to compare data between CRC tissues and normal tissues, and χ2 test was employed for comparing miR-378a-5p expression and clinicopathological parameters of CRC patients. One-way analysis of variance was used to evaluate the significance among multiple groups, followed by the Bonferroni post hoc test. p < .05 was identified to be statistically significant.
Author contributions
Substantial contributions to conception and design: Guolin Hu; Data acquisition, data analysis and interpretation: Pengbiao Che, Ling Deng, Lei Liu, Jia Liao, Qi Liu; Drafting the article or critically revising it for important intellectual content: All authors; Final approval of the version to be published: All authors; Agreement to be accountable for all aspects of the work in ensuring that questions related to the accuracy or integrity of the work are appropriately investigated and resolved: All authors.
Availability of data and materials
The analyzed data sets generated during the study are available from the corresponding author on reasonable request.
Disclosure statement
No potential conflict of interest was reported by the author(s).
Additional information
Funding
References
- Brody H. Colorectal cancer. Nature. 2015;521(7551):S1. doi:10.1038/521S1a.
- Lech G, Slotwinski R, Slodkowski M, Krasnodebski IW. Colorectal cancer tumour markers and biomarkers: recent therapeutic advances. World J Gastroenterol. 2016;22(5):1745–14. doi:10.3748/wjg.v22.i5.1745.
- Balchen V, Simon K. Colorectal cancer development and advances in screening. Clin Interv Aging. 2016;11:967–76. doi:10.2147/CIA.S109285.
- Kobiela J, Spychalski P, Marvaso G, Ciardo D, Dell’acqua V, Kraja F, Błażyńska-Spychalska A, Łachiński AJ, Surgo A, Glynne-Jones R, et al. Ablative stereotactic radiotherapy for oligometastatic colorectal cancer: systematic review. Crit Rev Oncol Hematol. 2018;129:91–101. doi:10.1016/j.critrevonc.2018.06.005.
- Chavali LB, Llanos AAM, Yun JP, Hill SM, Tan XL, Zhang L. Radiotherapy for patients with resected tumor deposit–positive colorectal cancer: a surveillance, epidemiology, and end results–based population study. Arch Pathol Lab Med. 2018;142(6):721–729. doi:10.5858/arpa.2017-0099-OA.
- Liu N, Cui W, Jiang X, Zhang Z, Gnosa S, Ali Z, Jensen L, Jönsson J-I, Blockhuys S, Lam EWF, et al. The critical role of dysregulated RhoB signaling pathway in radioresistance of colorectal cancer. Int J Radiat Oncol Biol Phys. 2019;104(5):1153–64. doi:10.1016/j.ijrobp.2019.04.021.
- Na YJ, Kim BR, Kim JL, Kang S, Jeong YA, Park SH, Jo MJ, Kim J-Y, Kim HJ, Oh SC, et al. Deficiency of 15-LOX-1 induces radioresistance through downregulation of MacroH2A2 in colorectal cancer. Cancers Basel. 2019;11(11):11. doi:10.3390/cancers11111776.
- Park SY, Lee CJ, Choi JH, Kim JH, Kim JW, Kim JY, Nam JS. The JAK2/STAT3/CCND2 Axis promotes colorectal cancer stem cell persistence and radioresistance. J Exp Clin Cancer Res. 2019;38(1):399. doi:10.1186/s13046-019-1405-7.
- Yang P, Yang Y, An W, Xu J, Zhang G, Jie J, Zhang Q. The long noncoding RNA-ROR promotes the resistance of radiotherapy for human colorectal cancer cells by targeting the p53/miR-145 pathway. J of Gastro and Hepatol. 2017;32(4):837–845. doi:10.1111/jgh.13606.
- Wang G, Li Z, Zhao Q, Zhu Y, Zhao C, Li X, MA Z, LI X, ZHANG Y. LincRNA-p21 enhances the sensitivity of radiotherapy for human colorectal cancer by targeting the Wnt/β-catenin signaling pathway. Oncol Rep. 2014;31(4):1839–1845. doi:10.3892/or.2014.3047.
- Yang XD, Xu XH, Zhang SY, Wu Y, Xing CG, Ru G, Xu HT, Cao JP. Role of miR-100 in the radioresistance of colorectal cancer cells. Am J Cancer Res. 2015;5(2):545–559.
- Zheng L, Zhang Y, Liu Y, Zhou M, Lu Y, Yuan L, Zhang C, Hong M, Wang S, Li X, et al. MiR-106b induces cell radioresistance via the PTEN/PI3K/AKT pathways and p21 in colorectal cancer. J Transl Med. 2015;13(1):252. doi:10.1186/s12967-015-0592-z.
- Zhu Y, Wang C, Becker SA, Hurst K, Nogueira LM, Findlay VJ, Camp ER. miR-145 antagonizes SNAI1-mediated stemness and radiation resistance in colorectal cancer. Mol Ther. 2018;26(3):744–754. doi:10.1016/j.ymthe.2017.12.023.
- Pan X, Zhao L, Quan J, Liu K, Lai Y, Li Z, Zhang Z, Xu J, Xu W, Guan X, et al. MiR-378a-5p acts as a tumor suppressor in renal cell carcinoma and is associated with the good prognosis of patients. Am J Transl Res. 2019;11(4):2207–2218.
- Nadeem U, Ye G, Salem M, Peng C. MicroRNA-378a-5p targets cyclin G2 to inhibit fusion and differentiation in BeWo cells. Biol Reprod. 2014;91(3):76. doi:10.1095/biolreprod.114.119065.
- Li H, Dai S, Zhen T, Shi H, Zhang F, Yang Y, Kang L, Liang Y, Han A. Clinical and biological significance of miR-378a-3p and miR-378a-5p in colorectal cancer. Eur J Cancer. 2014;50(6):1207–1221. doi:10.1016/j.ejca.2013.12.010.
- Emons G, Spitzner M, Reineke S, Moller J, Auslander N, Kramer F, Hu Y, Beissbarth T, Wolff HA, Rave-Fränk M, et al. Chemoradiotherapy resistance in colorectal cancer cells is mediated by Wnt/β-catenin signaling. Mol Cancer Res. 2017;15(11):1481–1490. doi:10.1158/1541-7786.MCR-17-0205.
- Lin CC, Lo MC, Moody R, Jiang H, Harouaka R, Stevers N, Tinsley S, Gasparyan M, Wicha M, Sun D, et al. Targeting LRP8 inhibits breast cancer stem cells in triple-negative breast cancer. Cancer Lett. 2018;438:165–73. doi:10.1016/j.canlet.2018.09.022.
- Zhao L, Bode AM, Cao Y, Dong Z. Regulatory mechanisms and clinical perspectives of miRNA in tumor radiosensitivity. Carcinogen. 2012;33(11):2220–7. doi:10.1093/carcin/bgs235.
- Wang XC, Wang W, Zhang ZB, Zhao J, Tan XG, Luo JC. Overexpression of miRNA-21 promotes radiation-resistance of non-small cell lung cancer. Radiat Oncol. 2013;8(1):146. doi:10.1186/1748-717X-8-146.
- Zhang Y, Zheng L, Ding Y, Li Q, Wang R, Liu T, Sun Q, Yang H, Peng S, Wang W, et al. MiR-20a induces cell radioresistance by activating the PTEN/PI3K/Akt signaling pathway in hepatocellular carcinoma. Int J Radiat Oncol Biol Phys. 2015;92(5):1132–40. doi:10.1016/j.ijrobp.2015.04.007.
- Tian Y, Tian Y, Tu Y, Zhang G, Zeng X, Lin J, Ai M, Mao Z, Zheng R, Yuan Y, et al. microRNA-124 inhibits stem-like properties and enhances radiosensitivity in nasopharyngeal carcinoma cells via direct repression of expression of JAMA. J Cell Mol Med. 2020;24(17):9533–44. doi:10.1111/jcmm.15177.
- Afshar S, Najafi R, Sedighi Pashaki A, Sharifi M, Nikzad S, Gholami MH, Khoshghadam A, Amini R, Karimi J, Saidijam M, et al. MiR-185 enhances radiosensitivity of colorectal cancer cells by targeting IGF1R and IGF2. Biomed Pharmacother. 2018;106:763–9. doi:10.1016/j.biopha.2018.07.002.
- Gu C, Cai J, Xu Z, Zhou S, Ye L, Yan Q, Zhang Y, Fang Y, Liu Y, Tu C, et al. MiR-532-3p suppresses colorectal cancer progression by disrupting the ETS1/TGM2 axis-mediated Wnt/β-catenin signaling. Cell Death Dis. 2019;10(10):739. doi:10.1038/s41419-019-1962-x.
- Wu Y, Pu N, Su W, Yang X, Xing C. Downregulation of miR-1 in colorectal cancer promotes radioresistance and aggressive phenotypes. J Cancer. 2020;11(16):4832–40. doi:10.7150/jca.44753.
- Zhang Y, Zheng L, Huang J, Gao F, Lin X, He L, Li D, Li Z, Ding Y, Chen L, et al. MiR-124 radiosensitizes human colorectal cancer cells by targeting PRRX1. PloS ONE. 2014;9(4):e93917. doi:10.1371/journal.pone.0093917.
- Machado IF, Teodoro JS, Palmeira CM, Rolo AP. miR-378a: a new emerging microRNA in metabolism. Cell Mol Life Sci. 2020;77(10):1947–58. doi:10.1007/s00018-019-03375-z.
- Yang R, Wei M, Yang F, Sheng Y, Ji L. Diosbulbin B induced G2/M cell cycle arrest in hepatocytes by miRNA-186-3p and miRNA-378a-5p-mediated the decreased expression of CDK1. Toxicol Appl Pharmacol. 2018;357:1–9. doi:10.1016/j.taap.2018.08.016.
- Maire V, Mahmood F, Rigaill G, Ye M, Brisson A, Nemati F, Gentien D, Tucker GC, Roman‐Roman S, Dubois T, et al. LRP8 is overexpressed in estrogen-negative breast cancers and a potential target for these tumors. Cancer Med. 2019;8(1):325–36. doi:10.1002/cam4.1923.
- Liu S, Fan W, Gao X, Huang K, Ding C, Ma G, Yan L, Song S. Estrogen receptor alpha regulates the Wnt/β-catenin signaling pathway in colon cancer by targeting the NOD-like receptors. Cell Signal. 2019;61:86–92. doi:10.1016/j.cellsig.2019.05.009.
- Zhang J, Zhang X, Zhang L, Zhou F, van Dinther M, Ten Dijke P. LRP8 mediates Wnt/β-catenin signaling and controls osteoblast differentiation. J Bone Miner Res. 2012;27(10):2065–2074. doi:10.1002/jbmr.1661.
- Cui Z, Liu QL, Sun SQ, Jiao K, Liu DR, Zhou XC, Huang L. MiR-378a-5p inhibits angiogenesis of oral squamous cell carcinoma by targeting KLK4. Neoplasma. 2020;67(1):85–92. doi:10.4149/neo_2019_190306N191.
- Cheng X, Xu X, Chen D, Zhao F, Wang W. Therapeutic potential of targeting the Wnt/beta-catenin signaling pathway in colorectal cancer. Biomed Pharmacother. 2019;110:473–481. doi:10.1016/j.biopha.2018.11.082.
- Jun S, Jung YS, Suh HN, Wang W, Kim MJ, Oh YS, Lien EM, Shen X, Matsumoto Y, McCrea PD, et al. LIG4 mediates wnt signalling-induced radioresistance. Nat Commun. 2016;7(1):10994. doi:10.1038/ncomms10994.
- Shi L, Xi J, Xu X, Peng B, Zhang B. MiR-148a suppressed cell invasion and migration via targeting WNT10b and modulating beta-catenin signaling in cisplatin-resistant colorectal cancer cells. Biomed Pharmacother. 2019;109:902–909. doi:10.1016/j.biopha.2018.10.080.
- Feng H, Zhao JK, Schiergens TS, Wang PX, Ou BC, Al-Sayegh R, Li M-L, Lu A-G, Yin S, Thasler WE, et al. Bone marrow-derived mesenchymal stromal cells promote colorectal cancer cell death under low-dose irradiation. Br J Cancer. 2018;118(3):353–65. doi:10.1038/bjc.2017.415.
- Ochiai S, Nishida Y, Higuchi Y, Morita D, Makida K, Seki T, Ikuta K, Imagama S. Short-range UV-LED irradiation in postmenopausal osteoporosis using ovariectomized mice. Sci Rep. 2021;11(1):7875. doi:10.1038/s41598-021-86730-0.
- vMeyer AV, Klein D, de Leve S, Szymonowicz K, Stuschke M, Robson SC, Jendrossek V, Wirsdörfer F. Host CD39 deficiency affects radiation-induced tumor growth delay and aggravates radiation-induced normal tissue toxicity. Front Oncol. 2020;10:554883. doi:10.3389/fonc.2020.554883.