Abstract
Exposure to UV-B at ambient or enhanced levels is known to trigger a variety of responses in all living organisms, including higher plants. Here we show that in Cucumis sativus L. UV-B radiation affects enzyme activity of key oxydative pentose phosphate pathway (OPPP) enzymes glucose-6-phosphate dehydrogenase (G6P-DH) and 6-phosphogluconate dehydrogenase (6-PGlu-DH), of key phenolic compounds enzyme phenylalanine ammonia lyase (PAL) as well as erythrose-4-phosphate, tryptophan and tyrosine levels. Furthermore, we found an increased activity of antioxidant enzymes such as peroxidase (POX) and catalase (CAT) in treated plants, with respect to the controls. In order to confirm the biochemical results, we isolated total RNA from both controls and UV-B treated plants to be used for gene expression analysis. We demonstrated that UV-B increases the gene expression level of peroxidase (POX), catalase (CAT) and phenylalanine ammonia lyase (PAL). Finally, our results are useful for understanding protective strategies against UV-B radiation and for elucidating what components are involved in stress-induced signals within the plant.
Introduction
Ultraviolet (UV) radiation is an integral part of the sunlight that reaches Earth's surface. The UV region of the spectrum is by convention divided into three parts: UV-A (320–400 nm), UV-B (280–320 nm) and UV-C (less than 280 nm).
Decrease in the stratospheric concentration of ozone, catalyzed mainly by chlorofluorocarbons (CFCs) and other pollutants, causes increase in the amount of UV-B radiation (260–320 nm) that reaches the Earth's surface (Mackerness Citation2000). The global trend of increasing UV-B radiation has been confirmed in response to stratospheric ozone loss in mid-latitude Japan (Sasaki et al. Citation2002), in New Zealand in the 1990s (McKenzie et al. Citation1999), and measurements of global ozone levels between the 1970s and 1990s at high and mid-latitudes in both hemispheres indicated significant increases in UV-B radiation (Madronich et al. Citation1995, Munakata et al. Citation2006). Furthermore, according to the CitationUnited Nations Environmental Programme (UNEP) report (2002), several years will be needed before stratospheric ozone recovery can be confirmed unambiguously, although the concentrations of most of the anthropogenic gases causing the depletion are now decreasing (McKenzie et al. Citation2003). Even a small increase in incident UV-B radiation can have significant biological effects, since UV-B is readily absorbed by a number of important biological macromolecules, such as DNA and proteins (Frohnmeyer & Staiger Citation2003). Over the last two decades, extensive studies of the physiological, biochemical and morphological effects of UV-B in plants, as well as the mechanisms of UV-B resistance, have been carried out. Many studies have shown that UV-B affects photosynthesis by disrupting several processes including the electron transport system, photosystem II reaction centers, pigment stability and by decreasing the level of transcripts encoding products required for this process (Casati & Walbot Citation2003, Izaguirre et al. Citation2003). UV-B can also directly damage proteins and lipids (Gerhardt et al. Citation1999, Bieza & Lois Citation2001, Frohnmeyer & Staiger Citation2003).
Leaf epidermis absorbs incoming UV radiation, and flavonoid compounds and cuticular waxes are the principal agents of UV absorption. The mixture of flavonoids found in plant tissues possesses a broad absorption spectrum in the UV-B range, thus shielding plant DNA from UV-induced damage (Stapleton & Walbot Citation1994). The fact that these compounds are virtually ubiquitous in tracheophytes has led to the long-standing hypothesis that a primary adaptive advantage conferred by these compounds is that they absorb potentially harmful UV-B radiation (280–315 nm) at the leaf surface and protect underlying photosynthetic tissues. In addition, increased H2O2 levels are detected simultaneously with the inhibition of photosynthesis by UV-B irradiation (Fujibe et al. Citation2004). This observation suggests that the UV-B-induced oxidative bursts of H2O2 are associated with the damage and degradation of PSII. During normal cellular metabolism, plants are continuously producing reactive oxygen species (ROS) such as superoxide anion, H2O2, and singlet oxygen. Under normal conditions, however, plants rapidly metabolize these ROS with the help of antioxidant enzymes and/or metabolites (Asada Citation1999). Various environmental perturbations such as UV-B radiation can cause excess ROS production, overwhelming the system and necessitating additional defences. There is considerable intra- and interspecific variability in the sensitivity of crop plants to UV-B radiation, and the sensitivity of plants to UV-B radiation varies widely among species and cultivars (Teramura Citation1983, Bornman & Teramura Citation1993, Correia et al. Citation1998, Mazza et al. Citation2000). In addition, both the magnitude and extent of these UV-B responses are influenced by a number of other factors, including the physiological and developmental status of the plant, as well as the amount and spectral composition of associated background radiation, in particular ultraviolet-A (UV-A) and visible radiation (Shinkle et al. Citation2004). Furthermore, solar UV-B radiation exclusion studies have indicated that ambient levels of solar UV-B radiation reduce biomass accumulation and grain yield in cucumber (Krizet et al. Citation1997), lettuce (Krizet et al. Citation1998), barley (Mazza et al. Citation1999), soybean (Mazza et al. Citation2000), maize (Gao et al. Citation2004) and rice (Hidema & Kumagai Citation2006). Taken together, the results of these studies suggest that the enhanced solar UV-B radiation predicted by atmospheric models will result in the reduction of growth and yield of crops in the future.
The aim of this work was to assess the biochemical and molecular biological responses of cucumber (C. sativus L.), a classical test plant and an important crop, to UV-B irradiation, with regards to key enzymes of primary (OPPP pathway and scavenging enzymes) and secondary metabolism (phenolic compound pathway).
Materials and methods
Plant material and UV-B treatments
Cucumber (Cucumis sativus L. var. “Piccolo di Parigi”) seeds were purchased from Franchi Sementi, Bergamo, Italy. Seeds were washed with tap water for 4 h and then sown in quartz sand in 12 cm plastic pots in a growth chamber.
The growth conditions were as follows: a 16 h photoperiod, temperature of 26/22°C (day/night), photosynthetic photon flux rate (PPFR) of 100 µmol m−2 s−1 (Fluora Osram/Dulux Electronic Osram) and relative humidity of 65± 10%. Plants were watered every two days and fertilized weekly with standard fertilizers (N:P:K, 20:20:20). Four-week old cucumber plants were then irradiated with UV-B (310 nm, Philips TL 40W/12) for 15 min in the middle of the day. Tubes were suspended 50 cm above the plants and the distance was adjusted as plants grew. TL tubes were filtered by using 0.1 mm cellulose diacetate foil which absorbs radiation below 290 nm. UV treatment resulted in a daily UV-B radiation of 2.0 W m−2. Plants were irradiated for an average period of 3 days.
Soluble protein, total phenol and photosynthetic pigment determination
After the last UV-B treatment, soluble proteins, total phenols and photosynthetic pigments were extracted and determined. Soluble protein concentration was evaluated by the method of Bradford (Citation1976) using BSA as standard. Total phenols were determined according to Bray and Thorpe (Citation1954) with the Folin–Ciocalteu reagent using rutin as standard. Photosynthetic pigments were measured according to Lichtenthaler (Citation1987) using aqueous acetone (80%).
OPPP pathway enzyme extraction and assay
After the last UV-B treatment, both control and treated leaves were ground in liquid N2 in cold 50 mM Tris-HCl pH 7.50, 1 mM EDTA, 2 mM MgCl2, 24 mM NADP+ and 2.5% w/V Polyclar AT in a 1:5 proportion (w/v). The homogenate was then filtered through eight layers of cheesecloth and centrifuged at 20 000 g for 30 min at 4°C. The supernatant was used directly for enzyme assays according to Simcox et al. (Citation1977).
Glucose-6-phosphate dehydrogenase (G6P-DH; E.C.1.1.1.49)
G6P-DH activities were measured as reduction of NADP+ (ε = 6.22 mM−1 cm−1) in the presence of glucose-6-phosphate. The reaction mixture contained 50 mM Tris-HCl (pH 7.50), 2 mM MgCl2, 0.17 mM NADP+, 6.6 mM glucose-6-phosphate and enzyme extract in 1.0 ml final volume. The reaction was started by the addition of glucose-6-phosphate and the NADP+ reduction was followed spectrophotometrically at 340 nm.
6-Phosphogluconate dehydrogenase (6-PGlu-DH; E.C.1.1.1.43)
6-Phosphogluconate dehydrogenase activities were measured as reduction of NADP+ (ε = 6.22 mM−1 cm−1) in the presence of 6-phosphogluconate. The reaction mixture contained 50 mM Tris-HCl (pH 7.50), 2 mM MgCl2, 0.17 mM NADP+, 0.2 mM 6-phosphogluconate and enzyme extract in 1.0 ml final volume. The reaction was started by the addition of glucose-6-phosphate and NADP+ reduction was followed spectrophotometrically at 340 nm.
Phenylalanine ammonia lyase extraction and assay
Phenylalanine ammonia lyase (PAL, E.C. 4.3.1.5) was extracted and assayed according to Hadwinger and Schwonchau (Citation1971). After the last UV-B treatment, both control and treated leaves were ground in liquid N2 in cold 100 mM Tris-HCl pH 7.50, 15 mM EDTA, 20 mM β-mercaptoethanol, 1% (w/V) PVPP (polyvynilpolypirrolidone) in a 1:5 proportion (w/V). The homogenate was then filtered through eight layers of cheesecloth and centrifuged at 20 000 g for 10 min at 4°C. The resulting supernatant was brought to 40% saturation with the addition of solid NH4(SO4)2 and was stirred gently for several hours at 4°C. After centrifugation at 15 000 g for 10 min the supernatant was brought to 60% saturation with solid NH4(SO4)2 stirred for several hours at 4°C and then centrifuged at 15 000 g for 10 min. Pellets containing most of the enzyme activity were resuspended in a small volume of extraction buffer.
Erythrose-4-phosphate, tyrosine and tryptophan determination
Erythrose-4-phosphate content was determined by using the method of Ashwell (Citation1966). Tyrosine and tryptophan content were determined according to Undenfriend (Citation1957) and Undenfriend and Peterson (Citation1957), respectively. All the measurements were done after the last UV-B treatment.
Scavenging enzyme extraction and assay
Leaves were collected 24, 48 and 72 hours after the first UV-B treatment. ROS scavenger enzymes were extracted following the method of Zhang and Kirkham (Citation1996) with some modifications (Maffei et al. Citation2006). All operations were carried out at 4°C. One gram of plant material was ground with a mortar and pestle with liquid nitrogen in cold 50 mM sodium phosphate (pH 7.5) 0.2 mM EDTA and 1% w/V PVPP (polyvinylpolypirrolidone) in a 1:25 proportion (w/V). The homogenate was then filtered through eight layers of cheesecloth and centrifuged at 25 000 g for 20 min at 4°C. The supernatant was used directly for enzyme assays.
Peroxidase (POX; EC 1.11.1.7)
Peroxidase activities were measured as oxidation of guaiacol (ε = 26.6 mM−1 cm−1) in the presence of H2O2 (Zhang & Kirkham Citation1996). The reaction mixture contained 50 mM Na-phosphate (pH 7.0), 0.33 mM guaiacol, 0.27 mM H2O2 and enzyme extract in 1.0 ml final volume. The reaction was started by addition of guaiacol and followed spectrophotometrically at 470 nm.
Catalase (CAT; EC 1.11.1.6)
Catalase activities were assayed spectrophotometrically by monitoring the change in absorbance at 240 nm due to the decreased absorption of H2O2 (ε H2O2=39.4 mM−1 cm−1) (Zhang & Kirkham Citation1996). The reaction mixture in 1 ml final volume contained 50 mM Na-phosphate (pH 7.0), 15 mM H2O2 and enzyme extract. The reaction was initiated by the addition of H2O2.
Total RNA isolation
Total RNA was isolated from leaf tissues of control and UV-B treated plants, after 7, 24, 31, 48, 56 and 72 h following the first UV-B treatment using SV Total RNA Isolation System (Promega) according the manufacturer's instructions. The quality and quantity of the isolated RNA was assessed by using the Agilent 2100 Bioanalyzer (Agilent Technologies) and the RNA 6000 Nano LabChip® Kit (Agilent Technologies) following manufacturer's protocols.
cDNA synthesis, PCR amplifications and semi-quantitative analysis
For first strand cDNA synthesis, 2 µg of total RNA, 0.5 µg of oligo(dT)21 primer and sterile H2O up to 20 µl were incubated at 70°C for 5 min. After cooling the mixture for 10 min at room temperature, the following reagents were added: 20 mM DTT, 10 mM dNTP, 5X First Strand Buffer and 200 units of RevertAid™ H Minus M-MuLV Reverse Transcriptase (Fermentas) to a total volume of 40 µl. Samples were then incubated at 42°C for 1 hour and then the reverse transcriptase was inactivated by heating the mixture at 70°C for 10 min.
Primers for standard and real-time polymerase chain reaction (PCR) were designed using the Primer 3 Software (http://frodo.wi.mit.edu/cgi-bin/primer3/primer3_www.cgi) for a length of the resulting PCR product ranging from 100 to 150 bp.
RT-PCR analysis
The amplification reaction was carried out in a 25 µl reaction mixture containing 2.5 µl 10X PCR reaction buffer (Fermentas), 1.25 mM MgCl2, 0.2 mM dNTPs, 25 pmol forward and reverse primers, 1.0 units of Taq DNA polymerase (Fermentas), 2.0 µl of first strand cDNA as template and sterile H2O to final volume. The PCR reactions were carried out in a Whatman Biometra® T-Gradient Thermocycler. Cycling conditions consisted of an initial 2 min at 94°C followed by 40 sec denaturing at 94°C, 1.30 min annealing at 55.2°C and 1 min elongation at 72°C repeated for 25 cycles and with 7 min extension at 72°C.
Primers used for standard RT-PCR analysis were the following: catalase (GenBank accession number AAP57673) CAT F 5′-CTCCCAAATGTCCTCACCAT-3′ (forward primer), CAT R 5′-CATCGGGAACTTCTCAGCAT -3′ (reverse primer); peroxidase (GenBank accession number AAA33127) POX F 5′-CCACACGTTTGGAAAATCAA-3′ (forward primer), POX R 5′-TTCGAAGCTGCTCCCTGTAT-3′ (reverse primer); β-actin (GenBank accession number AB010922), ACT F 5′-GGAAATTGTCCGTGACATGA-3′ (forward primer), ACT R 5′-GAATCTCTCAGCTCCGATGG-3′ (reverse primer). One µl of the amplification reaction was analyzed by using the Agilent 2100 Bioanalyzer (Agilent Technologies) and the DNA 1000 LabChip® Kit (Agilent Technologies) following manufacturer's instructions.
Primer dropping RT-PCR analysis of PAL
Specific primers for cucumber PAL were used. The primer pairs yield PCR product of 138 bp. As internal standard we chose NS (White et al. Citation1990) which was amplified with a pair of primers which yield 520 bp PCR product.
The amplification reactions were carried out in a 25 µl reaction mixture containing 2.5 µl 10X PCR reaction buffer (Fermentas), 1.25 mM MgCl2, 0.2 mM dNTPs, 1.0 units of Taq DNA polymerase (Fermentas), 1.0 µl of first strand cDNA as template and 0.5 µM specific primer pairs. Equal aliquot of secondary primer sets (NS) were added at the appropriate cycle number by the primer dropping method (Wong et al. Citation1994, Gottwald et al. Citation2001). This technique uses multiple sets of primers in a co-amplification reaction that amplifies the target of interest within a predetermined range specific for each target. PCR was performed on a Whatman Biometra® T-Gradient Thermocycler. Cycling conditions for PAL consisted of an initial 2 min at 94°C followed by 30 sec denaturing at 94°C, 45 sec annealing at 55°C and 30 sec elongation at 72°C repeated for 13 cycles. After the last extension step NS forward and reverse primers at 0.5 µM concentration were added and the PCR reaction left to proceed for other 18 cycles with final 7 min extension at 72°C.
Primers used for the RT-PCR using the primer dropping method were the following: phenylalanine ammonia lyase (Genbank accession number AF529240) PAL F 5′- ACTCGTCTTGCCATTGCTTC-3′ (forward primer) and PAL R 5′- GCTCGGGTTTCTACTTGCAG-3′ (reverse primer); 18S ribosomal RNA sequence NS1 5′-GTAGTCATATGCTTGTCTC-3′ (forward primer) and NS2 5′- GGCTGCTGGCACCAGACTTGC-3′ (reverse primer). The reaction products were separated, detected and quantified with the Agilent 2100 Bioanalyzer (Agilent Technologies) and the DNA 1000 LabChip® Kit (Agilent Technologies) following manufacturer's instructions.
Quantitative Real-time PCR on PAL
The real-time PCR was done on an Mx3000P Real-Time PCR System (Stratagene, La Jolla, CA). The process was performed with 25 µl of reaction mixture consisting of 12.5 µl of 2X Brilliant SYBR Green QPCR Master Mix (Stratagene), 1 µl cDNA, 100 nM primers, and 30 nM ROX as a reference dye. The following protocol was applied: initial polymerase activation: 10 min at 95°C; 40 cycles of 30 sec at 95°C, 1 min at 55°C and 30 sec at 72°C. Relative expression levels of PAL have been calculated by using the method of Pfaffl (Citation2001). For qRT-PCR we employed the same set of primers for PAL and β-actin previously reported.
Statistical analysis
At least ten repetitions were used for the statistical treatment of the data which are expressed as mean values. Metric bars indicate the SD. Significance of differences observed in data sets was tested by Student's t-test at p<0.05 using SYSTAT 10 software.
Results
In irradiated plants a general decrease of leaf chlorophyll content was observed (). Chlorophyll b with a 40% average reduction was the chlorophyll most affected by UV-B-induced thylakoid membrane disruption, whereas less difference was found in the carotenoid content. In cucumber, the exposure to UV-B prompted a significant increase in total phenol content (+34%) (). Because UV-B treatments stimulated the production of phenolic compounds, we decided to monitor the amount of possible precursors in the pathway. Tyrosine (TYR) and tryptophan (TRP) levels were altered by UV-B radiation, with the former increasing up to 49% with respect to controls and the latter, decreasing by almost 100% (). The cell level of erythrose-4-phosphate, one of the end products of OPPP pathway, was also determined in treated and control plants. This compound showed an average 10% increase in cucumber irradiated leaves (). The high total and specific activity of phenylalanine ammonia lyase (PAL) (), the key enzyme of the phenol metabolic pathway, corresponded well to the above reported increase in total leaf phenol content ().
Figure 1. Total photosynthetic pigment content in leaf extracts from C. sativus control plants and irradiated with UV-B light. Values are expressed in mg g−1 fresh weight±SD. Different letters indicate highly significant differences (p<0.05).
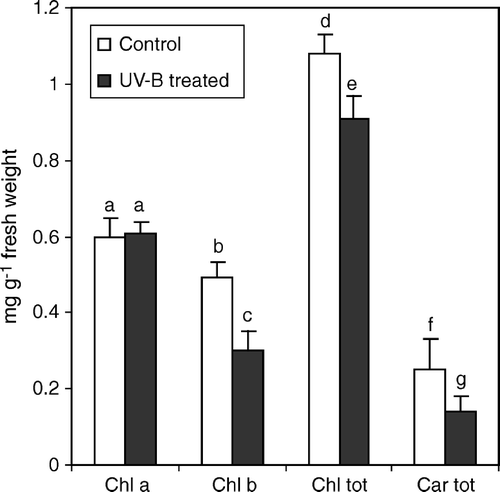
Figure 2. Total phenolic compound, erythrose 4 phosphate (E-4-P), tyrosine (TYR) and tryptophan (TRP) contents, in leaf extracts from C. sativus control plants and irradiated with UV-B light. Values are expressed in mg g−1 fresh weight±SD. Different letters indicate highly significant differences (p<0.05).
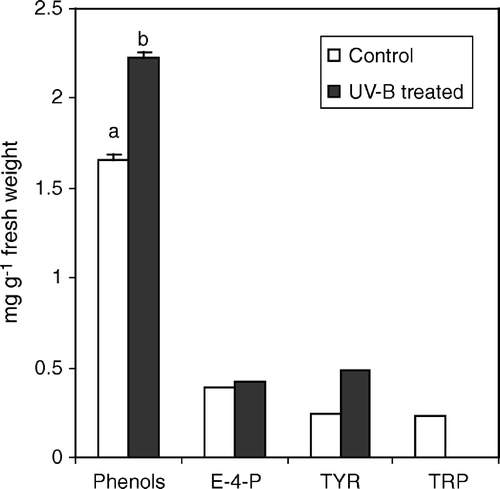
Figure 3. PAL specific activity in leaf extracts from C. sativus control plants and irradiated with UV-B light. Values are expressed in nKat mg−1 prot±SD. Different letters indicate highly significant differences (p<0.05).
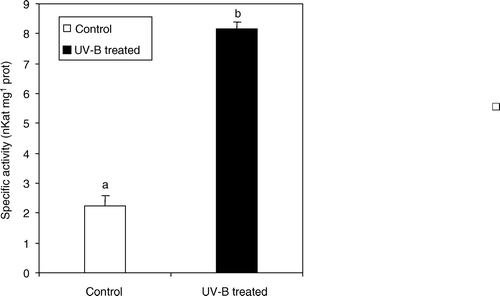
In order to assess whether UV-B light could lead to significant changes in primary plant metabolism, activities of two key enzymes of the OPPP pathway, namely β-d-glucose-6-phosphate dehydrogenase (G6P-DH) and 6-phosphogluconate dehydrogenase (6P-GluDH) were monitored. UV-B radiation induced significant higher specific activity of 6P-GluDH (0.77 mKat±0.2 in treated plants against 0.28 mKat±0.06 in control plants) whereas no significant differences were detected for G6P-DH specific activity ().
Figure 4. Glucose-6-dehydrogenase (G6P-DH) and 6-phosphogluconate dehydrogenase (6-PGlu-DH) specific activity in leaf extracts from C. sativus control plants and irradiated with UV-B light. Values are expressed in mKat mg−1 prot±SD. Different letters indicate highly significant differences (p<0.05).
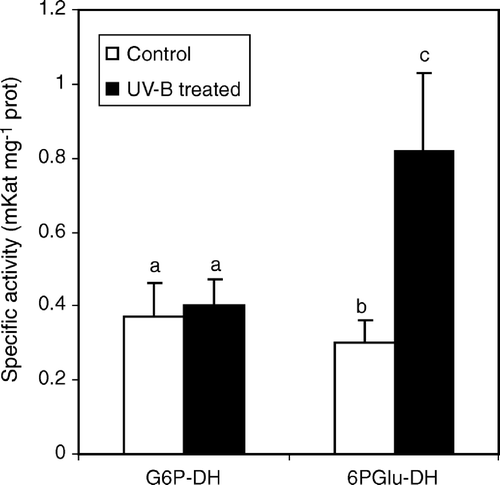
CAT and POX specific activities were stimulated by UV-B treatments. In particular, POX activity increased more than 24 times following the first treatment (24 h) with respect to the control. The increase was even higher, almost 140 times more, following the second (48 h) and third (72 h) UV-B treatments ().
Figure 5. Enzyme activity and gene expression of ROS-scavenging enzymes CAT and POX. Enzyme activities were assayed before and after each UV-B treatment at different sampling times. Values are expressed in µ Kat mg−1 prot±SD. CAT and POX expression levels were analyzed by semi-quantitative RT-PCR starting from total RNA. The ratio between each sample and the corresponding reference gene (β-actin) value has been calculated and results normalized to the control. Different letters above the metric bars indicate highly significant differences (p<0.05). Hours reported on the graphs are counted starting from the first UV-B treatment.
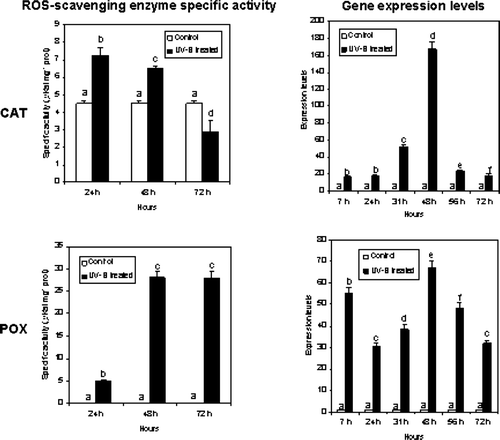
CAT specific activity was also induced by UV-B light. In particular, CAT activity almost doubled following the first (24 h) and the second treatments (48 h), whereas a decrease of the activity was recorded after the third UV-B treatment (72 h) ().
These preliminary biochemical results were confirmed by semi-quantitative RT-PCR analysis done on total RNA isolated from UV-B treated and control plants. In this case samples were taken after 7, 24, 31, 48, 56 and 72 h following the first UV-B treatment, in order to monitor mRNA expression on shorter time and the effect of the increased number of treatments. Both CAT and POX gene expression were stimulated by UV-B treatments (). PCR products obtained by using specific primers were analyzed using the Agilent 2100 Bioanalyzer (Agilent Technologies) (). POX gene expression levels increased following the three UV-B treatments, with a maximum expression at 48 h. These molecular biological data are in accordance with our preliminary biochemical results obtained by measuring POX specific activity (). However, a different expression profile was found for CAT, where a peak in the expression level was recorded at 48 h when the leaves were collected after a second UV-B treatment, whereas the values of transcripts detected at all the other time points were comparable ( and ).
Figure 6. Expression of CAT and POX in cucumber plants treated with UV-B radiation. Leaves were collected before and after each UV-B treatment and used for RT-PCR semi-quantitative analysis. PCR products were separated and quantified by using the Agilent 2100 Bioanalyzer (Agilent Technologies) and the DNA 1000 LabChip® Kit (Agilent Technologies). L = ladder, 1, control; 2, 7 h; 3, 24 h; 4, 31 h; 5, 48 h; 6, 56 h; 7, 72 h. Hours reported are counted starting from the first UV-B treatment.
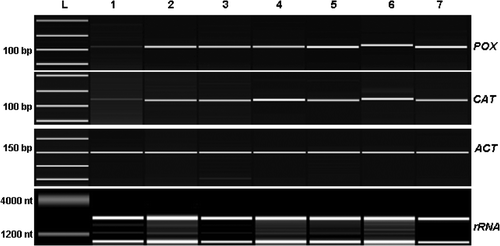
Furthermore, we have applied a method to monitor PAL mRNA expression in a semi-quantitative fashion on the Agilent 2100 Bioanalyzer by using the “primer-dropping” method (). Using specific primers designed on the sequences of C. sativus PAL (Genbank accession number AF475285) gene and primers for 18 S ribosomal RNA (NS) as an internal standard we were able to monitor and quantify the change in expression of one of the key genes involved in phenolic compound biosynthesis following UV-B radiation ().
Figure 7. Expression of PAL in cucumber plants treated with UV-B radiation. Leaves were collected before and after each UV-B treatment and used for RT-PCR semi-quantitative analysis. PCR products were separated and quantified by using the Agilent 2100 Bioanalyzer (Agilent Technologies) and the DNA 1000 LabChip® Kit (Agilent Technologies). L = ladder, 1, control; 2, 7 h; 3, 24 h; 4, 31 h; 5, 48 h; 6, 56 h; 7, 72 h.
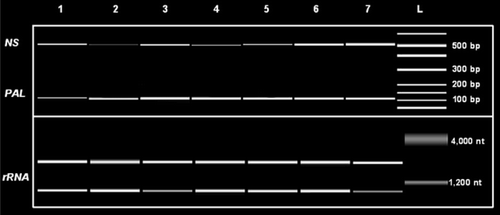
Figure 8. A) Expression levels of PAL obtained with a semi-quantitative method (primer dropping). The ratio between each sample and the corresponding reference gene (NS) value has been calculated and results normalized to the control. B) Relative expression of PAL by quantitative Real-Time PCR. Values were calculated according to Pfaffl (Citation2001). C. sativus β-actin was used as internal standard. Hours reported on the graph are counted starting from the first UV-B treatment.
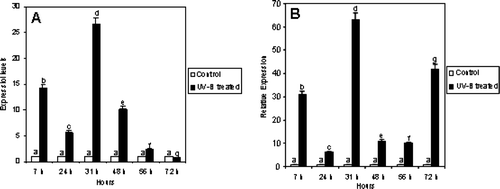
The results gave a first indication of the effect of UV-B radiation on the expression of genes involved in phenolic compound biosynthesis in cucumber. In particular, UV-B treatments seem to increase transcription of PAL. As shown in , PAL transcripts markedly increased after the second UV-B treatment (31 h), and the high transcription level remained until it began to decline, reaching a value comparable to the control at 72 h after the three UV-B treatments.
Similar results were obtained by employing a quantitative method (). In general, the expression profile was quite similar in semi-quantitative and quantitative experiments, with the exception of PAL at 72 h, in which qRT-PCR revealed a higher expression value with respect to the results obtained by using the semi-quantitative approach.
Discussion
Considering the depletion of stratospheric ozone and the resulting increased UV-B irradiation reaching the surface of Earth, many studies have recently focused on the physiological and biochemical effects of UV radiation on living organisms. In mammalian cells, UV-B radiation has been shown to interfere with processes such as transcription and replication, resulting in the reduction of RNA synthesis, arrest of cell cycle progression, and apoptosis (Sancar et al. Citation2004). Because plants must be exposed to sunlight to power photosynthesis, they are inevitably exposed to the damaging UV-B (Boldt & Scandalios Citation1997).
When a plant absorbs UV-B radiation, leaf photosynthetic apparatus is heavily damaged. Pigment degradation (chlorophylls and carotenoids) and thylakoid disruption occurs and this explains why chlorophyll and carotenoid decrease in cucumber leaves. As reported for other plant species (Strid & Porra Citation1992), this process mainly affects phothosystem II, thus reducing chlorophyll b content. Carotenoids were less affected than chlorophylls by UV-B treatment. The higher stability of carotenoid composition under UV-B has also been observed in other studies (Sharma et al. Citation1998, Barsig & Malz Citation2000). Under UV-B stress, energetic and metabolic resources are diverted towards the synthesis of scavenger compounds, among which are phenols and, especially, flavonoids. The latter are localized in the uppermost parts of the leaf mesophyll as protective compounds, which reduce disruption of the photosynthetic apparatus. Direct evidence of the role of phenolic accumulation in conferring UV tolerance has been obtained in Arabidopsis thaliana (L.) Heynh. mutants deficient in specific phenolic compound biosynthetic enzyme activities. This allowed determination of the relative importance of phenylpropanoids and flavonoids in conferring UV tolerance in A. thaliana (Sheahan Citation1996).
Accumulation of phenols in UV-B treated leaves is probably induced by the activation of PAL, whose activity can be regulated both by a direct effect of UV-B light and through phytocrome activation (Reddy et al. Citation1994). The observed changes in cucumber tryptophan and tyrosine levels could be ascribed to a diversion occurring within the biosynthetic pathway of aromatic compounds, with a repression of indol derivatives synthesis (Deckmyn et al. Citation1994), in favor of tyrosine production and its subsequent conversion into flavonoids. The increased activity registered in C. sativus for G6P-DH and 6P-GluDH of the OPPP pathway, can be reasonably interpreted with the activation of phenol biosynthesis. These two synthetic processes are linked by the production of erythrose-4-phosphate that through phosphoenolpyruvic acid can start phenolic compound synthesis. OPPP cycle activation could depend on the major cell demand for both erythrose-4-phosphate leading to aromatic compounds and for nucleotides precursors as well as d-ribose-5-phosphate, which is implicated in DNA repair cell processes.
Generally, when plants are allowed to develop under enhanced UV-B regimes or allowed to acclimate to doses of supplemental UV-B radiation, soluble phenolics and photosynthetic tolerance to UV increase simultaneously (Gitz et al. Citation2004).
Aside from increasing phenolic compound content, other adaptive responses have been observed, such as an increase of free radical scavenging capacity due to the fact that UV-B can generate reactive oxygen species at various sites of respiratory and photosynthetic electron transport (Arora et al. Citation2002, Matysik et al. Citation2002, Stratmann Citation2003) as well as during various biochemical reactions in cellular systems. These reactive oxygen species (ROS) are highly deleterious for cell structures and functions (Halliwell & Gutteridge Citation1984, Hideg & Vass Citation1996, Foyer et al. Citation1997). In order to prevent the harmful effects caused by these stresses, organisms develop radical quenchers and antioxidants that provide protection by scavenging harmful radical or oxygen species (Mittler & Tel-Or Citation1991, Middleton & Teramura Citation1993, Prasad & Zeeshan, Citation2005).
To minimize oxidative damage, plants have evolved various non-enzymatic and enzymatic defense mechanisms to detoxify ROS and reduce oxidative stress. Non-enzymatic antioxidants include ascorbate, α-tocopherol and β-carotene. The antioxidant defense system includes enzymes such as superoxide dismutase (SOD), glutathione reductase (GR) catalase (CAT) and peroxidases (POX). Among these defenses, catalase and peroxidases are known to play a key role in protecting cells against oxidative stress.
The production of ROS species following UV-B radiation has already been demonstrated in cucumber cotyledons (Takeuchi et al. Citation1995).
Exposure of cucumber plants to UV-B enhanced the activity of catalase and peroxidase of leaf crude extracts with respect to the controls. The enhancement was particularly evident for POX, in which the activity recorded following the second UV-B treatment was 140 fold higher than in controls. Our results are in agreement with previous studies conducted by Rao et al. (Citation1996), in which it has been demonstrated that UV-B preferentially enhances peroxidase activity over other antioxidant enzymes such as superoxide dismutase, glutathione reductase and catalase. An increase in ascorbate peroxidase activity was also observed in response to supplemental UV-B radiation in cucumber cotyledons (Takeuchi et al. Citation1996), rice and cucumber mature leaves (Kim et al. Citation1996). Peroxidase activity measured with guaiacol as a substrate was reported to be increased by UV-B radiation in Hibiscus rosa-sinensis (Panagopoulos et al. Citation1989) and Beta vulgaris (Panagopoulos et al. Citation1990) and in cucumber cotyledons (Tekchandani & Guruprasad Citation1998).
In cucumber, the expression levels of genes coding for CAT and POX were enhanced by UV-B treatments. With regard to CAT, the maximum peak in the expression was recorded after the second UV-B treatment. Also the CAT-specific activity was still very high at the same time point. Following the third treatment both enzyme activity and expression level dropped. A different response of catalase to UV-B has been reported in Nicotiana plumbaginifolia (Willekens et al. Citation1994) and Zea mays (Boldt & Scandalios Citation1997) due to the presence of several isoforms and to the different metabolic functions. It has already been demonstrated that UV-B treatment can trigger up-regulation of antioxidant enzymes (Chen et al. Citation2003). Studies conducted by Yannarelli et al. (Citation2006) in soybean showed an increase of CAT activity under the lower UV-B doses demonstrating that this enzyme is up-regulated to safeguard normal cellular functions and survival. However, when the plants were exposed to high UV-B doses, a significant decrease in this antioxidant activity was observed. Taking into account the fact that endogenous production of ROS induces modifications of proteins, such as fragmentation, increased susceptibility to proteolysis and cross-linking reactions (Stadtman Citation1992) it is possible to conclude that at the highest UV-B dose, CAT activity was diminished as a result of ROS overproduction. Our experiments showed a decrease of CAT activity after the third UV-B treatment. This decrease was not paralleled by gene expression level suggesting a regulation mechanism at the protein level.
With regard to POX, the expression profile was quite comparable to the enzyme specific activity profile. UV-B radiation dramatically stimulates gene expression and enzyme activity of POX. However, following the third UV-B treatment the enzyme activity was still very high in comparison to the level of expression. Enhancement in the activity of POX may either be due to de novo synthesis of the enzyme, related to the rather high amount of POX transcripts accumulated after the second treatment, or suppression of the activity of a natural enzyme inhibitor caused by UV-B radiation. A regulatory role for a peroxidase inhibitor in the UV-B induced enhancement of peroxidase activity has been demonstrated by Tekchandani & Guruprasad (Citation1998) in cucumber cotyledons.
Aside from its destructive effects, UV-B radiation can also elicit gene activation. The induction of key enzyme synthesis in the phenylpropanoid pathway is known to be an early, rapid event in response to UV-B radiation (Jordan et al. Citation1994), followed by the synthesis and accumulation of effective, wavelength-selective filtering phenolics (mainly flavonoids) in the leaf epidermal cells. This biosynthetic process is slower than the expression of genes related to phenylpropanoid metabolism, and changes in the amount of gene transcripts are in fact reportedly more rapid than the biochemical inhibitory effects of UV-B on the photosynthetic components (Strid et al. Citation1994). In our experiments we monitored the changes in the expression levels of the gene coding for PAL in which we observed an increase in the level of transcripts following the first and the second UV-B treatment, whereas the expression levels reached almost the control values after the third treatment. These results are in accordance with the increased level of PAL activity and with the accumulation of phenolic compounds measured in our experiments. Other studies showed that UV-absorbing compounds, mainly flavonoid and sinapate esters, other metabolites of the phenylpropanoid pathway, markedly accumulated upon UV-B irradiation, which is a consequence of an increase in transcription of PAL and other genes of phenylpropanoid enzymes (Shirley Citation1996, Liu et al. Citation2006).
In conclusion, increased fluence rates of UV-B on cucumber cause significant biochemical changes paralleled by strong modulation of gene expression. These findings have been achieved through studies in controlled environmental conditions and exposure to artificial sources of ultraviolet radiation, thus extrapolation to changes on crop yield as a result of increases in terrestrial solar UV-B radiation remains difficult to evaluate.
References
- Arora , A , Sairam , RK and Srivastava , GC . 2002 . Oxidative stress and antioxidant system in plants . Curr Sci , 82 : 1227 – 38 .
- Asada , K . 1999 . The water-water cycle in chloroplasts: scavenging of active oxygens and dissipation of excess photons . Annu Rev Plant Physiol Plant Mol Biol , 50 : 601 – 39 .
- Ashwell G. 1966 . New colorimetric methods of sugars analysis. Methods Enzymol . London : Academic Press . vol 3 . p 85 – 6 .
- Barsig , M and Malz , R . 2000 . Fine structure, carbohydrates and photosynthetic pigments of sugar maize leaves under UV-B radiation . Environ Exp Bot , 43 : 121 – 30 .
- Bieza , K and Lois , R . 2001 . An Arabidopsis mutant tolerant to lethal ultraviolet-B levels shows constitutively elevated accumulation of flavanoids and other phenolics . Plant Physiol , 126 : 1105 – 15 .
- Boldt , R and Scandalios , JG . 1997 . Influence of UV-light on the expression of the Cat2 and Cat3 catalase genes in maize . Free Radical Biol Med , 43 : 505 – 14 .
- Bornman , JF and Teramura , AH . 1993 . “ Effects of ultraviolet-B radiation on terrestrial plants ” . In Environmental UV photobiology , Edited by: Young , AR , Björn , LO , Moan , J and Nultsch , W . 427 – 71 . New York : Plenum Press .
- Bradford , MM . 1976 . A rapid and sensitive method for the quantitation of microgram quantities of protein utilizing the principle of protein-dye binding . Anal Biochem , 72 : 248 – 54 .
- Bray , HG and Thorpe , WV . 1954 . “ Estimation of phenols ” . In Methods of biochemical analysis , Edited by: Glick , G . 27 – 55 . New York : Interscience Publ. Inc .
- Casati , P and Walbot , V . 2003 . Rapid transcriptome responses of maize (Zea mays) to UV-B in irradiated and shielded tissues . Genome Biology , 5 : R16
- Chen , K , Feng , H , Zhang , M and Wang , X . 2003 . Nitric oxide alleviates oxidative damage in the green alga Chlorella pyrenoidosa caused by UV-B radiation . Folia Microbiol , 48 : 389 – 93 .
- Correia , CM , Areal , ELV , Torres-Pereira , MS and Torres-Pereira , JMG . 1998 . Intraspecific variation in sensitivity to ultraviolet-B radiation in maize grown under field conditions. I. Growth and morphological aspects . Field Crop Res , 59 : 81 – 9 .
- Deckmyn , G , Martens , C and Impens , I . 1994 . The importance of the ratio UV-B/photosynthetic active radiation (PAR) during leaf development as a determining factor of plant sensitivity UV-B irradiance: effects on growth, gas exchange and pigmentation of bean plants (Phaseolus vulgaris cv. Label) . Plant Cell Environ , 17 : 295 – 301 .
- Foyer , CH , Delgado , HL , Dat , JF and Scott , IM . 1997 . Hydrogen peroxide and glutathione associated mechanisms of acclamatory stress tolerance and signalling . Physiol Plant , 100 : 241 – 54 .
- Frohnmeyer , H and Staiger , D . 2003 . Ultraviolet-B radiation-mediated responses in plants. Balancing damage and protection . Plant Physiol , 133 : 1420 – 8 .
- Fujibe , T , Saji , H , Arakawa , K , Yabe , N , Takeuchi , Y and Yamamoto , KT . 2004 . A methyl viologen-resistant mutant of Arabidopsis, which is allelic to ozone-sensitive rcd1, is tolerant to supplemental Ultraviolet-B Irradiation1 . Plant Physiol , 134 : 275 – 85 .
- Gao , W , Zheng , Y , Slusser , JR , Heisler , GM , Grant , RH , Xu , J and He , D . 2004 . Effects of supplementary ultraviolet-B irradiation on maize yield and qualities: a field experiment . Photochem Photobiol , 80 : 127 – 31 .
- Gerhardt , KE , Wilson , MI and Greenberg , BM . 1999 . Tryptophan photolysis leads to a UVB-induced 66 kDa photoproduct of ribulose-1,5-bisphosphate carboxylase/oxygenase (Rubisco) in vitro and in vivo . Photochem Photobiol , 70 : 49 – 56 .
- Gitz , DCIII , Liu-Gitz , L , McClure , JW and Huerta , AJ . 2004 . Effect of PAL inhibitor on phenolic accumulation and UV-B tolerance in Sirodela intermedia (Koch) . J Exp Bot. , 55 : 919 – 27 .
- Gottwald , E , Müller , O and Polten , A . 2001 . Semiquantitative reverse transcription-polymerase chain reaction with the Agilent 2100 Bioanalyzer . Electrophoresis , 22 : 4016 – 22 .
- Hadwinger , LA and Schwonchau , ME. 1971 . Ultraviolet light induced formation of pisatin and phenylalanine ammonia lyase . Plant Physiol , 47 : 588 – 590 .
- Halliwell , B and Gutteridge , MC . 1984 . Oxygen toxicity, oxygen radicals, transition metals and disease . Biochem J , 219 : 1 – 14 .
- Hideg , E and Vass , I . 1996 . UV-B induced free radical production in plant leaves and isolated thylakoid membranes . Plant Sci , 115 : 251 – 60 .
- Hidema , J and Kumagai , T. 2006 . Sensitivity of rice to ultraviolet-B radiation . Ann Bot , 97 : 933 – 42 .
- Izaguirre , MM , Scopel , AL , Baldwin , IT and Ballaré , CL . 2003 . Convergent responses to stress. Solar ultraviolet-B radiation and Manduca sexta herbivory elicit overlapping transcriptional responses in field-grown plants of Nicotiana longiflora . Plant Physiol , 132 : 1755 – 67 .
- Jordan , BR , James , PE , Strid , A and Anthony , RG . 1994 . The effect of ultraviolet-B radiation on gene expression and pigment composition in etiolated and green pea leaf tissue: UV-B-induced changes are gene-specific and dependent upon the developmental stage . Plant Cell Environ , 17 : 45 – 54 .
- Kim , HY , Kobayashi , K , Nouchi , I and Yoneyama , T . 1996 . Differential influences of UV-B radiation on antioxidants and related enzymes between rice (Oryza sativa L.) and cucumber (Cucumis sativus L.) leaves . Environ Sci , 9 : 55 – 63 .
- Krizet , DT , Britz , S and Mirecki , RM . 1998 . Inhibitory effects of ambient levels of solar UV-A and UV-B radiation on growth of cv. New Red Fire lettuce . Physiol Plant , 103 : 1 – 7 .
- Krizet , DT , Mirecki , RM and Britz , S . 1997 . Inhibitory effects of ambient levels of solar UV-A and UV-B radiation on growth of cucumber . Physiol Plant , 100 : 886 – 93 .
- Lichtenthaler , HK . 1987 . Chlorophylls and carotenoids: pigments of photosynthetic biomembranes . Methods Enzymol , 148 : 350 – 82 .
- Mackerness , A-H . 2000 . Plant responses to ultraviolet-B (UV-B: 280–320 nm) stress: what are the key regulators? . Plant Growth Reg , 32 : 27 – 39 .
- Madronich , S , McKenzie , RL , Caldwell , MM and Björn , LO . 1995 . Changes in ultraviolet radiation reaching the earth's surface . Ambio , 24 : 143 – 52 .
- Liu , R , Xu , S , Li , J , Hu , Y and Lin , Z. 2006 . Expression profile of a PAL gene from Astragalus membranaceus var. Mongholicus and its crucial role in flux into flavonoid biosynthesis . Plant Cell Rep , 25 : 705 – 10 .
- Maffei , ME , Mithöfer , A , Arimura , G-I , Uchtenhagen , H , Bossi , S , Bertea , CM , Starvaggi Cucuzza , L , Novero , M , Volpe , V Quadro , S . 2006 . Effects of feeding Spodoptera littoralis on lima bean leaves. III. Membrane depolarization and involvement of hydrogen peroxide . Plant Phys , 140 : 1022 – 35 .
- Matysik , J , Bhalu , AB and Mohanty , P . 2002 . Molecular mechanisms of quenching of reactive oxygen species by praline under stress in plants . Curr Sci , 82 : 525 – 32 .
- Mazza , CA , Battista , D , Zima , AM , Szwarcberg-Bracchitta , M , Giordano , CV , Acevedo , A , Scopel , AL and Ballare , CL . 1999 . The effects of solar ultraviolet-B radiation on the growth and yield of barley are accompanied by increased DNA damage and antioxidant responses . Plant Cell Environ , 22 : 61 – 70 .
- Mazza , CA , Boccalandro , HE , Giordano , CV , Battista , D , Zima , AM , Scopel , AL and Ballare , CL . 2000 . Functional significance and induction by solar radiation of ultraviolet-absorbing sunscreens in field-grown soybean crops . Plant Physiol , 122 : 117 – 25 .
- McKenzie , R , Connor , B and Bodeker , G . 1999 . Increased summertime UV radiation in New Zealand in response to ozone loss . Science , 285 : 1709 – 11 .
- McKenzie , RL , Björn , LO , Bais , A and Ilyasd , M . 2003 . Changes in biologically active ultraviolet radiation reaching the Earth's surface . Photochem Photobiol Sci , 2 : 5 – 15 .
- Middleton , EM and Teramura , AH . 1993 . The role of flavonol glycosides and carotenoids in protecting soybean from ultraviolet-B damage . Plant Physiol , 103 : 741 – 52 .
- Mittler , R and Tel-Or , E . 1991 . Oxidative stress responses in the unicellular cyanobacterium Synechococcus PCC 7942 . Free Radical Res Commun , 12 : 845 – 50 .
- Munkata N , Schuch N , Casiccia C , Kaneko M , Liu C-M , Jimbow K , Saida T , Nishigori C , Ogata K , Inafuku K , Cornain S , Ichihashi M Kanoko M , Mulyadi K , Lestari S , Wirohadidjojo W , Bolseé D , Kazadzis S , Meyer-Rochow V . Biological Monitoring of Solar UV radiation at 17 sites in Asia, Europe and South America from 1999 to 2004 . Photochem Photobiol , DOI: 10.1562/2005-07-07-RA-602 .
- Panagopoulos , I , Bornman , JF and Björn , LO . 1989 . The effect of UV-B and UV-C radiation on Hibiscus leaves determined by ultraweak luminescence and fluorescecnce induction . Physiol Plant , 76 : 461 – 5 .
- Panagopoulos , I , Bornman , JF and Björn , LO . 1990 . Effects of ultraviolet radiation and visible light on growth, fluorescence induction, ultraweak luminescence and peroxidase activity in sugar beet plants . J Photochem Photobiol , 8 : 73 – 87 .
- Pfaffl , MW . 2001 . A new mathematical model for relative quantification in real-time RT-PCR . Nucl Acid Res :e , 29 : 45
- Prasad , SM and Zeeshan , M . 2005 . UV-B radiation and cadmium induced changes in growth, photosynthesis, and antioxidant enzymes of cyanobacterium Plectonema boryanum . Biol Plantarum , 49 : 229 – 36 .
- Rao , MV , Paliyath , G and Ormrod , DP . 1996 . Ultraviolet-B and ozone-induced biochemical changes in antioxidant enzymes of Arabidopsis thaliana . Plant Physiol , 110 : 125 – 36 .
- Reddy , VS , Goud , KV , Sharma , R and Reddy , AR . 1994 . Ultraviolet-B-responsive anthocyanin production in a rice cultivar is associated with a specific phase of phenylalanine ammoniaca lyase biosynthesis . Plant Physiol , 105 : 1059 – 66 .
- Sancar , A , Lindsey-Boltz , LA , Unsal-Kacmaz , K and Linn , S . 2004 . Molecular mechanisms of mammalian DNA repair and the DNA damage checkpoints . Annu Rev Biochem , 73 : 39 – 85 .
- Sasaki , M , Takeshita , S , Oyanagi , T , Miyake , Y and Sakata , T . 2002 . Increasing trend of biologically active solar ultraviolet-B irradiation in mid-latitude Japan in the 1990s . Ortical Engineering , 41 : 3062 – 9 .
- Sharma , PK , Anand , P , Sankhalkar , S and Shetye , R . 1998 . Photochemical and biochemical changes in wheat seedlings exposed to supplementary ultraviolet-B radiation . Plant Sci , 132 : 210 – 30 .
- Sheahan , JJ . 1996 . Sinapate esters provide greater UV-B attenuation than flavonoids in Arabidopsis thaliana (Brassicaceae) . Am J Bot , 83 : 679 – 86 .
- Shinkle , JR , Atkins , AK , Humphrey , EE , Rodgers , CW , Wheeler , SL and Barnes , PW . 2004 . Growth and morphological responses to different UV wavebands in cucumber (Cucumis sativum) and other dicotyledonous seedlings . Physiol Plant , 120 : 240 – 8 .
- Shirley , BW . 1996 . Flavonoid biosynthesis: “new” functions for an “old” pathway . Trends Plant Sci , 1 : 377 – 82 .
- Simcox , DP , Reid , EE , Canvin , DT and Dennis , DT . 1977 . Enzymes of the glycolytic and penthose phosphate pathways in proplastids from the developing endosperm of Ricinus communis L . Plant Phys , 59 : 1128 – 32 .
- Stadtman , ER . 1992 . Protein oxidation and aging . Science , 257 : 1220 – 4 .
- Stapleton , AE and Walbot , V . 1994 . Flavonoids can protect maize DNA from the induction of ultraviolet-radiation damage . Plant Physiol , 105 : 881 – 9 .
- Stratmann , J . 2003 . Ultraviolet-B radiation co-opts defense signaling pathways . Trends Plant Sci , 8 : 526 – 33 .
- Strid , Å and Porra , RJ . 1992 . Alterations in pigment content in leaves of Pisum sativum after exposure to supplementary UV-B . Plant Cell Physiol , 33 : 1015 – 23 .
- Strid , Å , Chow , WS and Anderson , JM . 1994 . UV-B damage and protection at the molecular level in plants . Photosynth Res , 39 : 475 – 89 .
- Takeuchi , Y , Fukumoto , R , Kasahara , H , Sakaki , T and Kitao , M . 1995 . Peroxidation of lipids and growth inhibition induced by UV-B irradiation . Plant Cell Rep , 14 : 566 – 70 .
- Takeuchi , Y , Kubo , H , Kasahara , H and Sakaki , T . 1996 . Adaptive alterations in the activities of scavengers of active oxygen in cucumber cotyledons irradiated with UV-B . J Plant Physiol , 147 : 589 – 92 .
- Tekchandani , S and Guruprasad , KN . 1998 . Modulation of a guaiacol peroxidase inhibitor by UV-B in cucumber cotyledons . Plant Sci , 136 : 131 – 7 .
- Teramura , A . 1983 . Effects of ultraviolet-B radiation on the growth and yield of crop plants . Physiol Plant , 58 : 415 – 27 .
- Undenfriend S . 1957 . Assay of aromatic aminoacid: tyrosine . Methods Enzymol . London : Academic Press . vol 3 . p 610 – 3 .
- Undenfriend S , Peterson RE . 1957 . Assay of aromatic aminoacid: tryptophan. Methods Enzymol London : Academic Press . vol. 3 . p 613 – 4 .
- United Nations Environmental Programme . 2002 . Environmental effects of ozone depletion and its interactions with climate change
- White , TJ , Bruns , TD , Lee , S and Taylor , JW . 1990 . “ Amplification and direct sequencing of fungal ribosomal RNA genes for phylogenetics ” . In PCR protocols: a guide to methods and applications , Edited by: Innis , MA , Gelfand , DH , Sninsky , JJ and White , TJ . 315 – 22 . New York : Academic Press .
- Willekens , H , Van Camp , W , Van Montagu , M , Sandermann , HJr and Langebartels , C . 1994 . Ozone, sulfur dioxide, and ultraviolet B have similar effects on mRNA accumulation of antioxidant genes in Nicotiana plumbagifolia . Plant Physiol , 106 : 1007 – 14 .
- Wong , H , Anderson , WD , Cheng , T and Riabowol , KT . 1994 . Monitoring mRNA expression by polymerase chain reaction: the “Primer-Dropping” method . Anal Bioch , 223 : 251 – 8 .
- Yannarelli GG , Noriega GO , Batlle A , Tomaro ML . 2006 . Heme oxygenase up-regulation in ultraviolet, Tomaro ML. 2006 . Heme oxygenase up-regulation in ultraviolet-B irradiated soybean plants involves reactive oxygen species . Planta , DOI: 10.1007/s00425-006-297-x .
- Zhang , J and Kirkham , MB . 1996 . Antioxidant responses to drought in sun-flower and sorghum seedlings . New Phytol , 132 : 361 – 73 .