ABSTRACT
Interactions between phenolic compounds in black currant leaves and foliar diseases may be important in breeding for resistant genotypes with a nutritional high profile for human applications. For increased understanding of such interactions, we evaluated the presence of major fungal diseases by visual inspection, and content of phenolic compounds by HPLC in leaves of five segregating black currant breeding populations. Eight individual flavonols (e.g. quercetin-3-O-glucoside, quercetin-3-O-rutinoside and kaempferol-malonylgucoside), three flavan-3-ols (epigallocatechin, catechin and epicatechin) and two chlorogenic acids (neochlorogenic acid and chlorogenic acid) were significantly correlated to the leaf diseases. Rib-0701 was the population possessing the highest content for several of the compounds, while genotype differences existed for content of various phenolic compounds and resistance to the diseases. The high variability of content of phenolic compounds opens up for opportunities to breed resistant genotypes with improved health properties of the leaves for functional food products.
1. Introduction
Black currant (Ribes nigrum L.) leaves are known as a rich source of phenolic compounds, normally seen as secondary metabolites. The phenolic compounds are of biological value for the plant and of nutritious value for human uses by the pharmaceutical and food industry. Phenolic compounds are known for their diverse functions in the plant, including for pigmentation, growth and reproduction, and for resistance against pathogens and fungi. Previous investigations have strongly indicated phenols to be involved in both plant–animal and plant–microorganisms relationships (Lattanzio et al. Citation2008; Witzell & Martin Citation2008). Plants are known to respond to herbivory and microbial attacks through a range of mechanisms, including those being of morphological, biochemical and molecular nature. Oxidation of phenols is known as a potential defense mechanism as is the presence of tannins (War et al. Citation2012). Phenolic compounds known to have antifungal activities are, among others, simple phenols, phenolic acids, flavonols, dihydrochalcones, flavones and flavanones (Treutter Citation2005, Citation2006; Lattanzio et al. Citation2008). The fact that the oxidation of phenols is an important defense mechanism in plants has been shown in a number of studies where the amount of polyphenol oxidase, catalyzing oxidation of phenols, has been related to diseases and to resistance to pests and pathogens (Li & Steffens Citation2002; Mohammadi & Kazemi Citation2002; Thipyapong et al. Citation2004; Pourcel et al. Citation2007; Mahanil et al. Citation2008).
If black currant leaves are to be used by the pharmaceutical or food industry, a high content and a relevant composition of polyphenols are of significant importance (Vagiri et al. Citation2015). Fungal foliar diseases may limit the production of healthy leaves for such applications, simultaneously as berry yield and quality is impacted. The main fungal diseases in black currant plantations are anthracnose caused by Drepanopeziza ribis (Kleb), septoria leaf spot caused by Mycosphaerella ribis (Fuckel) Lindau), mildew caused by Sphaerotheca mors-uvae (Schwein.) Berk & Curtis) and white pine blister rust caused by Cronatium ribicola Fisch (Brennan Citation2008; Xu et al. Citation2009; Hummer & Dale Citation2010).
Generally, the control of these diseases is dependent on the use of chemical pesticides, although concerns have been raised due to increasing costs, deteriorating soil quality and environmental risks, and also due to consumer preference of fruit free of residue (Brennan & Graham Citation2009). Another option to combat leaf fungal diseases would be to breed for genotypes with enhanced natural defense compounds, thereby lowering both production costs and pesticide use. One such type of defense compounds is the phenolics. In grape leaves, increased levels of phenolic compounds were reported in resistant varieties when compared to susceptible ones and at increasing foliar disease severity (Dai et al. Citation1994; Taware et al. Citation2010). Also, in black currants the content of certain phenolic compounds has previously been associated with resistance to powdery mildew (Trajkovski & Pääsuke Citation1976). Furthermore, high levels of hydroxycinnamic acids, epicatechin, myricetin, kaempferol and quercetin glycosides were detected in black currant plants with low symptoms of leaf spot infection (Mikulic-Petkovsek et al. Citation2013). However, there is still a lack of knowledge in the understanding of interactions between phenolic compounds in black currant leaves and resistance to fungal diseases in the plant.
Therefore, the aim of the present study was to determine the phenolic content in black currant leaves of five segregating populations and evaluate possible associations between content of major phenolic compounds and major leaf diseases in black currant.
2. Materials and methods
2.1. Plant material
Five segregating populations (Rib-0701, parentage: ‘Ben Hope’ × BRi 8825-2; Rib-0702, parentage: ‘Ben Hope’ × BRi 8916-5; Rib-0704, parentage: ‘Ben Hope’ × SK 8944-13; Rib-0720, parentage: ‘Big Ben’ × BRi 8825-2 and Rib-0722, parentage: ‘Big Ben’ × BRi 8916-5) composed of 50 plants (seedlings) each were established during 2006 in an unreplicated integrated field trial at the Department of Plant Breeding, Balsgård, The Swedish University of Agricultural Sciences, in the south of Sweden (56°06′N, 14°10′E). The parental types for these populations were selected based on their diversity across a range of agronomical, developmental, pest/diseases resistance and fruits’ quality traits. The visual assessment and scoring of resistance to anthracnose, septoria, mildew and rust were carried out when these fungal diseases were fully prevalent in a natural infection background in field conditions in 2010. Disease severity was assessed using a 1–9 scale, where: 1 = no disease symptoms (0%), 2 = very small disease symptoms (< 5%), 3 = small disease symptoms (5–25%), 5 = moderate disease symptoms (26–50%), 7 = strong disease symptoms (51–75%) and 9 = heavy disease symptoms (76–100%). For phenol analyses, fully grown leaves without petioles were randomly collected, and immediately transferred to a freezer (–20°C) and freeze-dried with a tray-style freeze dryer (Christ 336, Osterode am Harz, Germany) using vacuum and cold for 1 week. Afterwards, the leaves were crushed and ground to a fine powder using an analytical mill (Yellow line, A10, IKA-Werke, Staufen, Germany) and stored at –20°C until analyzes.
2.2. Reagents and chemicals
Acetonitrile (isocratic grade, purity >99.8%), methanol (HPLC grade, purity >99.8%), Formic acid (pro analysi, purity 98−100%), 85% ortho-phosphoric acid (H3PO4) and chlorogenic acid were purchased from Sigma (St. Louis, MO). Neo-chlorogenic acid, myricetin, quercetin-3-O-rutinoside, quercetin-3-O-galactoside, quercetin-3-O-glucoside, quercetin3-6-malonylglucoside, kaempferol-3-O-glucoside, isorhamnetin-3-O-glucoside and kaempferol were purchased from Extrasynthese (Genay, France). Ultrapure water was obtained by use of Elgastat Prima UHQPS (Buckinghamshire, England). Ethanol (purity 99.98%) was purchased from VWR (Fontenay-Sous-Bois, France).
2.3. Extraction and analysis of phenolic compounds
About 50 mg of the powdered leaf samples were weighed in triplicates and extracted with 1.5 mL 50% ethanol (purity 99.98%) containing 0.05 mol/L H3PO4 in water. The extracts were vortexed and sonicated in an ultrasonic bath (Bandelin Sonorex, Berlin, Germany) at room temperature for 15 min and centrifuged at 15,300 g for 10 min. The supernatant was transferred to a vial prior to HPLC analyses.
The HPLC method used in this study is the same as previously described in Vagiri et al. (Citation2013). The samples were analyzed by a Shimadzu HPLC-DAD system (Kyoto, Japan) with a LC-20AB model dual pump, a SPD-M10A model diode array detector equipped with Waters 717 plus autosampler linked to a Shimadzu SCL-10A model system controller. The eluent consisted of formic acid/water (7:93 v/v; mobile phase A) and acetonitrile/methanol/water (90:5:5 v/v; mobile phase B) with gradient elution as follows: The linear gradient starting with 0–2 min, 8% B; 2–21.5 min, 8–16% B; 21.5–51.5 min, 16–23% B; 51.5–56.5 min, 23–40% B; and 56.5–61.5 min, back to 8% B followed by re-equilibration of the system for 2 min with initial conditions. The linear binary gradient was set to a flow rate of 1.2 mL min−1. The injection volume of samples was 10 μL and total run time was 63.5 min. The column used was a Synergie hydro RP-80A column (250 × 4.60 mm i.d., 4 μm particle size), protected with a C-18 guard cartridge (4 × 3.0 mm) both from Phenomenex (Værløse, Denmark). The column temperature was set at 24°C using a Shimadzu CTO-10AS thermostatically controlled column compartment. The chromatographic data were collected using Class VP software (Shimadzu 5.0). Acquisition wavelength was set in the range of 260–550 nm, and chromatograms were recorded at 280 nm for flavan-3-ols, 320 nm for chlorogenic acids (neo chlorogenic and chlorogenic acids) and 360 nm for flavonols. All the compounds were quantified with reference to external standards as listed in section 2.2. For compounds lacking standards, quantification was carried out using similar compounds as standards. Therefore, myricetin malonlyglucoside and kaempferol malonylglucoside were quantified as equivalents to myricetin and kaempferol, respectively. The HPLC analyses were performed using three technical replicates for each plant and thereafter means of the three replicates were calculated. Finally the population mean of all plants (n = 50) were calculated and expressed as µg/g ± S.D dry weight (DW).
2.4. Statistical analyses
Analysis of variance was performed using 50 plants from each population. Significant differences among populations were determined using Tukey’s test (p < .05). Spearman correlation coefficients (r) were calculated to reveal relationships between phenolic compounds and leaf diseases. All the above-mentioned statistical analyses were carried out using SAS statistical package, version 9.2 (SAS Institute Inc., Cary, NC). Canonical discriminant analysis (CDA) was performed using the SPSS (version 23, SPSS Inc., Chicago, IL, USA) software.
3. Results
3.1. Variation in phenolic content and foliar diseases between populations
All populations generally showed a wide variation in content for specific phenolic compounds (; Table S1, supporting information). With regard to the flavan-3-ols, Rib-0704 had the highest amount of epigallocatechin and epicatechin, whereas Rib-0701 had the highest amount of catechin. The content of neochlorogenic acid and chlorogenic acid were highest in Rib-0701, with the lowest content reported for Rib-0702 and Rib-0722 ((A), Table S1(A)). In case of flavonols ((B), Table S1(B)), the population Rib-0701 contained abundant levels of all flavonols, whereas quercetin-3-O-galactoside was highest in Rib-0720 compared to other populations. Interestingly, for kampferol-3-O-rutinoside the difference between the populations were not so pronounced (Table S1(B)).
Figure 1. Mean (n = 50) content (µg/g DW) for the analyzed phenolic compounds (A) flavan-3-ols and chlorogenic acids, (B) flavonols in different black currant populations. Error bars indicate ± standard deviation (SD) of means. Codes: 1 = epigallocatechin; 2 = catechin; 3 = epicatechin; 4 = neochlorogenic acid; 5 = chlorogenic acid; 6 = myricetin-malonylglucoside; 7 = quercetin-3-O-galactoside; 8 = quercetin-3-O-glucoside; 9 = quercetin-3-O-rutinoside; 10 = quercetin-3-6-malonylglucoside; 11 = kampferol-3-O-rutinoside; 12 = kaempferol-3-O-glucoside; 13 = isorhamnetin-3-O-rutinoside; 14 = isorhamnetin-3-O-glucoside; 15 = kaempferol-malonylglucoside.
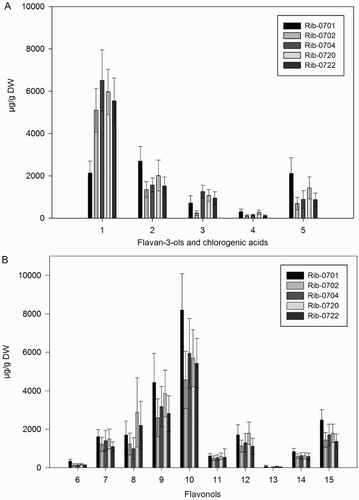
In terms of compositional differences, epigallocatechin was the most abundant compound among the flavan-3-ols ranging from 613 µg/g DW (in Rib-0701) to 9285 µg/g DW (in Rib-0704). Chlorogenic acid ranged from 167 µg/g DW (in Rib-0704) to 3954 µg/g DW (in Rib-0701), while neo chlorogenic acid ranged from 54 µg/g DW (in Rib-0722) to 783 µg/g DW (in Rib-0701) (Table S1(A)). Among the flavonols, quercetin-3-6-malonylglucoside ranging from 1157 µg/g DW (in Rib-0702) to 13693 µg/g DW (in Rib-0701) was the dominant compound, followed by quercetin-3-O-glucoside, while isorhamnetin-3-O-rutinoside was present in lowest amounts in all populations (Table S1(B)).
The black currant populations demonstrated different field resistance to the fungal diseases (). All the populations showed highest resistance to mildew with mean score of 1.1–1.9, indicating disease severity of less than 5%. Rib-0701 was moderately (50%) susceptible to rust and septoria, while the lowest severity for rust was displayed by Rib-0722, and for spetoria by Rib-0722 (). The mean scores of anthracnose ranged from 1.6 to 2.9, with very small (<5%) to small (25%) disease symptoms observed in Rib-0722 and Rib-0720, respectively.
Figure 2. Mean (n = 50) scores (scale 1-9, no disease symptoms to heavy disease symptoms) of foliar diseases between black currant populations. Different letter/letters indicate significant differences (p < .05) between the populations for each foliar disease. Error bars represent ± standard error (SE) of mean.
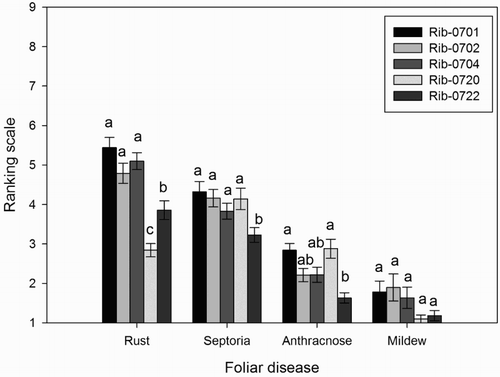
3.2. Classification of black currant populations
In order to classify black currant populations according to their content of phenolic compounds in the leaves, a canonical discriminant analysis was performed. Discriminant analysis works by creating one or more linear combinations of predictors, creating a new latent variable for each function. These functions are called discriminant functions. The first function created maximizes the differences between groups on that function. The second function maximizes differences on that function, but also must not be correlated with the previous function. This continues with subsequent functions with the requirement that the new function not be correlated with any of the previous functions. The two first discriminant functions expressed 88.2% of the total variation, with 72.5% for the first discriminant function and 15.7% for the second, and canonical correlations of 0.94 and 0.80, respectively (). The eigenvalues, which indicate the ratio of between-groups variability to within-groups variability for a function, were 8.424 and 1.818 for the first and second functions, respectively. Canonical correlation coefficients, which are measures of the degree of relatedness between the groups and the functions, are rather high, showing values between 0.945 and 0.803, respectively, for the first and the second discriminant function.
Table 1. Within-groups correlations between discriminating variables and standardized canonical discriminant functions for black currant populations according to their phenolic compounds in leaves.
The populations Rib-0701 and Rib-0702 were the most differentiated (). Epigallocatechin followed by quercetin-3-O-glucoside, as opposed to the rest, play an important role in group discrimination on Function 1, and Rib-0701 was the most unique of the five populations with respect to these discriminator variables (, ). Discriminator variables quercetin-3-O-glucoside, epicatechin and kaempferol-3-O-glucoside separated Rib-0702 from the other groups (). Epicatechin, quercetin-3-6-malonylglucoside, quercetin-3-O-galactoside in order of priority contributed to the grouping of populations Rib-0704 and Rib-0722 in function 3. Function 4 with the smallest eigenvalue contributed to the discrimination of Rib-0720, with discriminator variables being quercetin-3-6-malonylglucoside, kaempferol-malonylglucoside and quercetin-3-O-glucoside (, ).
3.3. Correlation between phenolic compounds and leaf diseases
Significant associations (p < .05) were observed between the phenolic compounds and leaf diseases for different populations studied (). A reasonable number of phenolic compounds were thus found to correlate (with R2 values above 0.2) with the leaf diseases scored and more negative correlations than positive were found.
Table 2. Significant spearman correlation coefficients between phenolic compounds and foliar diseases in black currant populations.
Anthracnose correlated positively with chlorogenic acid and total chlorogenic acids in Rib-0704 and negatively with catechin in Rib-0722. Septoria correlated negatively with epigallocatechin, neochlorogenic acid, kaempferol-3-O-glucoside, isorhamnetin-3-O-rutinoside, isorhamnetin-3-O-glucoside and total flavan-3-ols (r = –.45) in Rib-0701, with quercetin-3-O-rutinoside and epicatechin in Rib-0720 and Rib-0722, respectively. In case of rust, negative correlations were observed with epigallocatechin and catechin in Rib-0701, and with quercetin-3-O-galactoside (r = –.43), quercetin-3-O-glucoside (r = –.53), quercetin-3-6-malonylglucoside and with total flavonols in Rib-0702. A positive correlation between rust and epigallocatechin was noticed in Rib-0720. Mildew correlated negatively with isorhamnetin-3-O-rutinoside in Rib-0701 and positively with kaempferol-malonylglucoside in Rib-0704.
4. Discussion
Our study clearly revealed interactions between phenolic compounds in black currant leaves and leaf diseases, although, the found interactions varied among the investigated populations. Therefore, not only the abundance of individual phenolic compounds in black currant genotypes, but also their variations are important for an induced resistance against the different fungal diseases.
Eight individual flavonols (e.g. quercetin-3-O-glucoside, quercetin-3-O-rutinoside and kaempferol-malonylgucoside) were significantly correlated to the leaf diseases in the present study. In addition we also found that three flavan-3-ols (epigallocatechin, catechin and epicatechin) and two chlorogenic acids (neochlorogenic acid and chlorogenic acid) were related to resistance to leaf diseases. The associations revealed could probably be seen as expected as several of these compounds have previously been reported to be pre-formed in plant cells or produced after stimulation by different stresses and plant defense reactions against pathogens (VanEtten et al. Citation1994; Wink Citation2003). A number of phenolic compounds are also regarded as pre-infection inhibitors, providing plants with a certain degree of basic resistance against pathogenic microorganisms (Wink Citation2003; Schijlen et al. Citation2004; Treutter Citation2006).
Importance of phenolic compounds in resistance reactions have been reported for a range of crops and also in trees. A high content of flavonol glycosides have been reported in grapevines leaves with low susceptibility to powdery mildew (Erysiphe necator; Keller et al. Citation2003). In groundnut, quercetin and its glycoside rutin were related to larval mortality of the tobacco armyworm (syn. oriental leaf worm) Spodoptera litura (Mallikarjuna et al. Citation2004). Similarly, it has been reported that the presence of some preformed antifungal compounds, such as catechin and epicatechin, proved to be responsible for resistance against gray mold (Botrytis cinerea) in strawberries (Terry et al. Citation2004). Phenolic metabolites have also been reported as responsible for resistance in Northern forest trees (Witzell & Martin Citation2008).
Cytotoxic and antimicrobial activities of some flavones (e.g. quercetin-3-O-glucoside) have been reported by Razavi et al. (Citation2009), whereas other authors showed a synergistic efficacy of the antibacterial activity of quercetin-3-O-glucoside and kaempferol-3-O-glucoside (Akroum et al. Citation2009).
The content of phenolic compounds is known to be more or less affected by genotype (Brennan & Graham Citation2009). In our study, canonical discriminant analysis showed an obvious variability among the five breeding populations, especially between Rib-0701 and the other four populations. The population Rib-0701 displayed the highest content for several of the phenolic compounds among the populations, providing an opportunity of enhancing the levels by the use of this population in breeding. The genetic differences in terms of parentage among the populations could further explain why the corresponding leaf samples had significant differences in their phenolic content. The lowest and highest values of each phenolic compound together with coefficient of variation show a representation of how diverse the individuals within a population are. The large variation within populations indicate large opportunities to improve the concentrations of specific compounds through active breeding work. Genetic variation in terms of flavonol glycosided and other phenolic compounds in the leaves of black currant has also been reported recently (Liu et al. Citation2014).
The results of the present study are sometimes contradictory, for example, epigallocatechin correlates positively with rusts in population Rib-0701, while negatively in population Rib-0720. This can be speculated as an environmental variation (e.g. soil fertility, moisture, temperature, radiation) that could profoundly modify the chemical basis and outcome of interactions between plants and their pathogens. The present study should be seen as an introgression toward a more in-depth metabolomics study, where leaves are inoculated with different fungi and thereafter analyzed for composition of various secondary metabolites that would provide insights into the resistance mechanism.
A total of 15 phenolic compounds (which included three flavan-3-ols, two chlorogenic acids and 10 flavonols) were identified and quantified in the black currant leaves in this study. All these compounds have been previously reported in the leaves of black currant (Oszmiański et al. Citation2011; Vagiri et al. Citation2012). The results here agree with previous findings of black currant leaves being a rich source of bioactive phenolic compounds, particularly hydroxycinnamic acids, and flavonols as well as procyanidins (Tabart et al. Citation2006; Ehrhardt et al. Citation2013; Vagiri et al. Citation2015; Yang et al. Citation2015). As to specific phenolic compounds, particularly epigallocatechin, chlorogenic acid, quercetin-3-6-malonylglucoside, quercetin-3-O-glucoside, quercetin-3-O-rutinoside and kaempferol-malonylglucoside were among the most abundant in this study. This is in agreement with Liu et al. (Citation2014), who have also reported these compounds to be most abundant among the flavonols.
2011 For human applications (food or medical), phenolic compounds are associated with decreased risk of cardiovascular diseases, anti-diabetic and/or anti-inflammatory activities (Del Rio et al. Citation2013; Nile & Park Citation2014). For instance, chlorogenic acid is known to be a strong antioxidant and it is also known to reduce plasma glucose in humans (Iwai et al. Citation2012). Similarly, quercetin-3-6-malonylglucoside has been reported to be highly bioactive and known to be effective against oxidation of low-density lipoprotein and oxidative stress in the liver (Katsube et al. Citation2010). Extracts of black currant leaves have been demonstrated to possess anti-inflammatory and anti-virus activities (Tabart et al. Citation2011; Ehrhardt et al. Citation2013). The leaves of black currant might therefore be of interest as a potential source of extracts for the pharmaceutical and food industry, thereby increasing the utilization and value of the plant material. The results of this study also points on the direction that breeding-resistant genotypes toward leaf diseases, that is, with high levels of certain phenolic compounds, might lead to the development of genotypes with high levels of health beneficial phenolic compounds.
5. Conclusion
Our results clearly show presence of interactions between content of phenolic compounds in leaves of black currants and foliar diseases. Eight individual flavonols (e.g. quercetin-3-O-glucoside, quercetin-3-O-rutinoside and kaempferol-malonylgucoside), three flavan-3-ols (epigallocatechin, catechin and epicatechin) and two chlorogenic acids (neochlorogenic acid and chlorogenic acid) played important role in plant resistance to foliar diseases. This study thereby supports the hypothesis that phenolic compounds play an important role in plant resistance to fungal diseases, although we cannot conclusively identify the main mechanism involved. The high variability in content of phenolic compounds noted within the black currant populations may be the result of phenolic compounds being used as protection against fungal diseases. This study also depicted the importance of the genetic background as a crucial factor for content and composition of phenolic compounds in black currant leaves, and thereby for resistance toward foliar diseases. Thus, metabolomics to profile black currant populations should be a useful tool in breeding programs to develop cultivars with resistance to various fungal diseases and with high contents of nutritional compound for human applications.
Supplemental_Material.docx
Download MS Word (82 KB)Disclosure statement
No potential conflict of interest was reported by the authors.
Additional information
Funding
References
- Akroum S, Bendjeddou D, Satta D, Lalaoui K. 2009. Antibacterial activity and acute toxicity effect of flavonoids extracted from mentha longifolia. Am Eurasian J Sci Res. 4:93–96.
- Brennan R. 2008. Currants and gooseberries, temperate fruit crop breeding. Dordrecht: Springer; p. 177–196.
- Brennan R, Graham J. 2009. Improving fruit quality in Rubus and Ribes through breeding. Func Plant Sci Biotec. 3:22–29.
- Dai GH, Andary C, Cosson-Mondolot L, Boubals D. 1994. Polyphenols and resistance of grapevines to downy mildew. Acta Hort. 381:763–766. doi: 10.17660/ActaHortic.1994.381.110
- Del Rio D, Rodriguez-Mateos A, Spencer JP, Tognolini M, Borges G, Crozier A. 2013. Dietary (poly) phenolics in human health: structures, bioavailability, and evidence of protective effects against chronic diseases. Antiox Redox Sig. 18:1818–1892. doi: 10.1089/ars.2012.4581
- Ehrhardt C, Dudek SE, Holzberg M, Urban S, Hrincius ER, Haasbach E, Seyer R, Lapuse J, Planz O, Ludwig S. 2013. A plant extract of Ribes nigrum folium possesses anti-influenza virus activity in vitro and in vivo by preventing virus entry to host cells. PloS One. 8:e63657. doi: 10.1371/journal.pone.0063657
- Hummer K, Dale A. 2010. Horticulture of Ribes. For Pathol. 40:251–263. doi: 10.1111/j.1439-0329.2010.00657.x
- Iwai K, Narita Y, Fukunaga T, Nakagiri O, Kamiya T, Ikeguchi M, Kikuchi Y. 2012. Study on the postprandial glucose responses to a chlorogenic acid-rich extract of decaffeinated green coffee beans in rats and healthy human subjects. Food Sci Technol Res. 18:849–860. doi: 10.3136/fstr.18.849
- Katsube T, Yamasaki M, Shiwaku K, Ishijima T, Matsumoto I, Abe K, Yamasaki Y. 2010. Effect of flavonol glycoside in mulberry (Morus alba L.) leaf on glucose metabolism and oxidative stress in liver in diet-induced obese mice. J Sci Food Agric. 90:2386–2392. doi: 10.1002/jsfa.4096
- Keller M, Viret O, Cole FM. 2003. Botrytis cinerea infection in grape flowers: defense reaction, latency, and disease expression. Biochem Cell Biol. 93:316–322.
- Lattanzio V, Kroon PA, Quideau S, Treutter D. 2008. Plant phenolics – secondary metabolites with diverse functions. In: Daayf F, Lattanzio V, editors. Recent advances in polyphenol research. Oxford: Wiley; p. 1–35.
- Li L, Steffens JC. 2002. Overexpression of polyphenol oxidase in transgenic tomato plants results in enhanced bacterial disease resistance. Planta. 215:239–247. doi: 10.1007/s00425-002-0750-4
- Liu P, Kallio H, Yang B. 2014. Flavonol glycosides and other phenolic compounds in buds and leaves of different varieties of black currant (Ribes nigrum L.) and changes during growing season. Food Chem. 160:180–189. doi: 10.1016/j.foodchem.2014.03.056
- Mahanil S, Attajarusit J, Stout MJ, Thipyapong P. 2008. Overexpression of tomato polyphenol oxidase increases resistance to common cutworm. Plant Sci. 174:456–466. doi: 10.1016/j.plantsci.2008.01.006
- Mallikarjuna N, Kranthi KR, Jadhav DR, Kranthi S, Chandra S. 2004. Influence of foliar chemical compounds on the development of Spodoptera litura (Fab.) in interspecific derivatives of groundnut. J Appl Entomol. 128:321–328. doi: 10.1111/j.1439-0418.2004.00834.x
- Mikulic-Petkovsek M, Slatnar A, Schmitzer V, Stampar F, Veberic R, Koron D. 2013. Chemical profile of black currant fruit modified by different degree of infection with black currant leaf spot. Sci Hort. 150:399–409. doi: 10.1016/j.scienta.2012.11.038
- Mohammadi M, Kazemi H. 2002. Changes in peroxidase and polyphenol oxidase activities in susceptible and resistant wheat heads inoculated with Fusarium graminearum and induced resistance. Plant Sci. 162:491–498. doi: 10.1016/S0168-9452(01)00538-6
- Nile SH, Park SW. 2014. Edible berries: bioactive components and their effect on human health. Nutri. 30:134–144. doi: 10.1016/j.nut.2013.04.007
- Oszmiański J, Wojdyl̷o A, Gorzelany J, Kapusta I. 2011. Identification and characterization of low-molecular-weight polyphenols in berry leaf extracts by HPLC-DAD and LC-ESI/MS. J Agric Food Chem. 59:12830–12835. doi: 10.1021/jf203052j
- Pourcel L, Routaboul J-M, Cheynier V, Lepiniec L, Debeaujon I. 2007. Flavonoid oxidation in plants: from biochemical properties to physiological functions. Trends Plant Sci. 12:29–36. doi: 10.1016/j.tplants.2006.11.006
- Razavi SM, Zahri S, Zarrini G, Nazemiyeh H, Mohammadi S. 2009. Biological activity of quercetin-3-O-glucoside, a known plant flavonoid. Russ J Bioorg Chem. 35:376–378. doi: 10.1134/S1068162009030133
- Schijlen EG, Ric De Vos CH, van Tunen AJ, Bovy AG. 2004. Modification of flavonoid biosynthesis in crop plants. Phytochem. 65:2631–2648. doi: 10.1016/j.phytochem.2004.07.028
- Tabart J, Kevers C, Evers D, Dommes J. 2011. Ascorbic acid, phenolic acid, flavonoid, and carotenoid profiles of selected extracts from Ribes nigrum.. J Agric Food Chem.. 59:4763–4770. doi: 10.1021/jf104445c
- Tabart J, Kevers C, Pincemail J, Defraigne JO, Dommes J. 2006. Antioxidant capacity of black currant varies with organ, season, and cultivar J Agric Food Chem 54:6271–6276. doi: 10.1021/jf061112y
- Taware PB, Dhumal KN, Oulkar DP, Patil SH, Banerjee K. 2010. Phenolic alterations in grape leaves, berries and wines due to foliar and cluster podery mildew infections. Int J Pharma Bio Sci. 1:1–14.
- Terry LA, Joyce DC, Adikaram NK, Khambay BP. 2004. Preformed antifungal compounds in strawberry fruit and flower tissues. Postharvest Biol Technol. 31:201–212. doi: 10.1016/j.postharvbio.2003.08.003
- Thipyapong P, Hunt MD, Steffens JC. 2004. Antisense downregulation of polyphenol oxidase results in enhanced disease susceptibility. Planta. 220:105–117.
- Trajkovski V, Pääsuke R. 1976. Resistance to Sphaerotheca mors-uvae (Schw.) Berk. In Ribes nigrum L. 5. Studies on breeding black currants for resistance to Sphaerotheca mors-uvae (Schw.) Berk. Swed J Agric Res. 6:201–214.
- Treutter D. 2005. Significance of flavonoids in plant resistance and enhancement of their biosynthesis. Plant Biol. 7:581–591. doi: 10.1055/s-2005-873009
- Treutter D. 2006. Significance of flavonoids in plant resistance: a review. Environ Chem Letters 4:147–157. doi: 10.1007/s10311-006-0068-8
- Vagiri M, Conner S, Stewart D, Andersson SC, Verrall S, Johansson E, Rumpunen K. 2015. Phenolic compounds in blackcurrant (Ribes nigrum L.) leaves relative to leaf position and harvest date. Food Chem. 172:135–142. doi: 10.1016/j.foodchem.2014.09.041
- Vagiri M, Ekholm A, Öberg E, Johansson E, Andersson SC, Rumpunen K. 2013. Phenols and ascorbic acid in black currants (Ribes nigrum L.): variation due to genotype, location, and year. J Agric Food Chem. 61:9298–9306. doi: 10.1021/jf402891s
- Vagiri MR, Ekholm A, Andersson SC, Johansson E, Rumpunen K. 2012. An optimized method for analysis of phenolic compounds in buds, leaves and fruits of black currant (Ribes nigrum L.). J Agric Food Chem. 60:10501–10510. doi: 10.1021/jf303398z
- Van Etten HD, Mansfield JW, Bailey JA, Farmer EE. 1994. Two classes of plant antibiotics: phytoalexins versus ‘phytoanticipins.’ Plant Cell. 6:1191–1192. doi: 10.1105/tpc.6.9.1191
- War AR, Paulraj MG, Ahmad T, Buhroo AA, Hussain B, Ignacimuthu S, Sharma HC. 2012. Mechanisms of plant defense against insect herbivores. Plant Sign Behav. 7:1306–1320. doi: 10.4161/psb.21663
- Wink M. 2003. Evolution of secondary metabolites from an ecological and molecular phylogenetic perspective. Phytochem. 64:3–19. doi: 10.1016/S0031-9422(03)00300-5
- Witzell J, Martin JA. 2008. Phenolic metabolites in the resistance of the northern forest trees to pathogens – past experiences and future prospects. Can J Forest Res. 38:2711–2727. doi: 10.1139/X08-112
- Xu XM, Robinson JD, Berrie AM. 2009. Infection of blackcurrant leaves by Drepanopeziza ribis in relation to weather conditions and leaf position. J Phytopathol. 157:280–286. doi: 10.1111/j.1439-0434.2008.01488.x
- Yang W, Alanne AL, Liu P, Kallio H, Yang B. 2015. Flavonol glycosides in currant leaves and variation with growth season, growth location, and leaf position. J Agric Food Chem. 63:9269–9276. doi: 10.1021/acs.jafc.5b04171