ABSTRACT
Among the heavy metals (HMs), lead (Pb) is considered as a toxic HM which adversely affects growth and development of crop plants. The present experiment was aimed to investigate the potential role of ascorbic acid (ASC) in the reversal of Pb-inhibited nitrogen and sulfur assimilation enzymes activity and activity of photosynthesis enzyme ribulose-1,5-bisphosphate carboxylase/oxygenase (Rubisco) and growth response in wheat plants. Wheat seedlings were subjected to 0 mM (control) and 0.2 mM and 0.6 mM of ASC with and without 2 mM of Pb. Plants treated with Pb exhibited the following reduced growth characteristics (root length, shoot length, root fresh weight (FW), shoot FW, root dry weight (DW) and shoot DW). A decrease was also observed in the activity of Rubisco and ATP sulfurylase (ATP-S), relative water content (RWC), accumulation of total chlorophyll (Total Chl) and content of nutrients [nitrogen (N), phosphorus (P), potassium (K), calcium (Ca) and magnesium (Mg)] in Pb-treated plants. However, an increase in Chl degradation and in the activity of O-acetylserine(thiol)lyase (OAS-TL) and accumulation of cysteine (Cys), malondialdehyde (MDA) and hydrogen peroxide (H2O2) was observed in plants under Pb stress. On the contrary, exogenous application of ASC mitigated the Pb-toxicity-induced oxidative damage by enhancing the activities of antioxidant enzymes, such as superoxide dismutase, catalase and glutathione reductase. Improved activity of antioxidant enzymes suppressed the formation of MDA and H2O2, which was reflected in the form of improved growth characteristics. Moreover, ASC induced improvement in plants defense systems by reduced Chl degradation and improved the content of essential nutrients (N, P, K, Ca and Mg) and Cys, RWC and the activity of Rubisco, ATP-S, NR and OAS-TL.
Introduction
The toxicity of heavy metals (HMs) has become a prevalent global threat to the environment. Anthropogenic activities have been considered as the worst culprit of HMs toxicity. Increasing urbanization and industrialization add HMs to the environment and cause disruption of pristine ecosystems (Ross, Citation1994; Fan et al., Citation2016). Furthermore, agriculture intensification through excessive use of fertilizers and insecticides, and wastewater discharge also add HMs to the environment (Shen et al., Citation2002; Sharma and Dubey Citation2005; Khan et al., Citation2017). Heavy metals adversely affect animals and humans. Being sessile in nature, plants are primary victims of HM-toxicity. Heavy metals-rich soil disturbs nutrients homeostasis in plants and depresses plant growth by affecting the uptake and distribution of certain nutrients in plants (Lamhamdi et al., Citation2013; Upadhyay, Citation2014; Massoud et al., Citation2018). Non-essential HMs [lead (Pb), cadmium, Mercury, and silver] enter the cell and limit cellular functions by generating reactive oxygen species (ROS). Of these, Pb is a toxic HM that adversely affects plant growth and metabolism (Sharma and Dubey Citation2005; Dogan et al., Citation2009). Lead enters the plants via their roots and alters their morphological, physio-biochemical and molecular mechanisms (Fan et al., Citation2016; Mahdavian et al., Citation2016; Malar et al., Citation2016; Simonetti et al., Citation2016). Lead limits seed germination, biomass production and also causes nutrients imbalance in plants (Sharma and Dubey Citation2005; Malar et al., Citation2016). It damages cell membranes and suppresses photosynthetic capacity and stomatal conductance of the plant by inhibiting biosynthesis of chlorophylls, activity of Rubisco and water uptake (Lunde et al., Citation2008; Rossato et al., Citation2012; Leal-Alvarado et al., Citation2016). Also, Pb induces oxidative stress by overproducing ROS, such as singlet oxygen (1O2), superoxide radical (O2 •–), hydroperoxy radical (HO2 •), hydrogen peroxide (H2O2) and hydroxyl radical (OH•). Excessive generation of ROS causes deterioration of macromolecules, resulting in decreased physiological and biochemical processes (Mahdavian et al., Citation2016; Amari et al., Citation2017), leading to reduced growth and productivity of crop plants.
To overcome the adverse effects of abiotic stresses, plants possess various systems of defense, such as enzymatic and non-enzymatic antioxidants. The enzymatic antioxidant system include superoxide dismutase (SOD), catalase (CAT), ascorbate peroxidase (APX), glutathione reductase (GR) and non-enzymatic antioxidants (glutathione, ascorbate), which continuously scavenge harmful ROS. To counter abiotic stress-induced osmotic stress, plants accumulate osmolytes, such as trehalose, polyols (glycerol, inositols, sorbitols etc.), amino acids (proline, glycine betaine and taurine), which maintain normal water status of the plant. In addition, plants counter metal stress by synthesizing metal-chelates, organic acids and polyphosphates that cause restriction and sequestration of toxic metals either in apoplasm or symplasm. Besides, one of the important detoxification mechanisms of HMs is carried out by sulfur (S) containing peptides, which cause chelation by binding with metals in cytosol, and metal-phytochelatin complex are sequestrated into the vacuole (Mendoza-Cózatl et al., Citation2005). Cysteine (Cys), the key product of S assimilation (Siddiqui et al., Citation2012a), affects various biomolecules including antioxidants and other compounds involved in detoxification of HMs. In plants, Cys is synthesized from O-acetylserine (OAS) and sulfide (Khan et al., Citation2017) by the action of ATP-sulfurylase (ATP-S; a precursor enzyme) and O-acetylserine(thiol)lyase (OAS-TL) enzymes. In addition, nitrate reductase (NR), a crucial N-assimilation enzyme, is activated by sulphate and ATP-S activity, and ATP-S is induced by nitrate (Smith Citation1980; Brunold and Suter Citation1984). Therefore, S-assimilation and nitrogen (N) assimilation are coordinated pathways that play important role in plant growth and development under abiotic stress (Kopriva and Rennenberg, Citation2004, Siddiqui et al., Citation2008, Citation2012a). However, timely and precise activation of these defense systems in response to stress stimulus prior to the onset of damage is crucial for plants growth and development. The stress stimulus and specific response to the stimulus is transmitted by a network of signaling molecules.
It is well established that ascorbic acid (ASC) is one of the important non-enzymatic antioxidants and plays vital role in the growth and normal functioning of plants. ASC regulates a number of cellular processes, such as cell division, cell differentiation and senescence (Venkatesh and Park Citation2014). Furthermore, ASC protects lipids and proteins and improves tolerance against various abiotic stresses, induces plant growth, photosynthesis, transpiration, oxidative defense potential and photosynthetic pigments (Khan et al., Citation2010; Naz et al., Citation2016, Akram et al., Citation2017). However, none or meager information is available on ASC mediated S- and N-assimilation pathway involved in detoxification of Pb in plants. Therefore, the objective of the present study was to investigate the role of ASC in S and N-assimilation and their role in the tolerance of wheat plants to Pb toxicity.
Materials and methods
Uniform sized seeds of wheat (Triticum aestivum var. Yecora Rojo) were surface sterilized with 1% sodium hypochlorite. The sterilized seeds were sown in plastic pots (6 cm in diameter) containing sterile acid washed sand and supplied with nutrient solution (Smith et al., Citation1983). The salts used to prepare the nutrient solution were as follows: Macronutrient stock solution A (g L-1) Mg(NO3)2.6H2O, 4.94; Ca(NO3)2.4H2O, 16.78; NH4NO3, 8.48; KNO3, 2.28. Macronutrient stock solution B (g L-1) KH2PO4, 2.67; K2HPO4, 1.64; K2SO4, 6.62; Na2SO4, 0.60; NaCl, 0.33. Micronutrient supplement (mg L-1) H3BO3, 128.80; CuCl2.2H2O, 4.84; MnCl2.4H2O, 81.10; (NH4)6Mo7O24.4H2O, 0.83; ZnCl2, 23.45; ferric citrate pentahydrate, 809.84. The composition of the nutrient solution applied to the plants was prepared by mixing 200 mL of each of the macronutrient stock solution with 100 mL of the micronutrient supplement and diluting to 4.5 L with double distilled water (DDW). The pH was maintained at 6.0 by adding a solution of H2SO4 or KOH. After one week of sowing, treatments were administered to the plants. The treatments were comprised of (i) 0 mM ASC + 0 mM Pb (control), (ii) 0.2 mM ASC + 0 mM Pb, (iii) 0.6 mM ASC + 0 m Pb, (iv) 0 mM ASC + 2 mM Pb, (v) 0.2 mM ASC + 2 mM Pb and (vi) 0.6 mM ASC + 2 mM Pb. To keep the sand moist, pots were irrigated every 3 days with DDW. After 9 weeks, full-grown plants with true leaves were used to measure plant growth attributes [root length (RL) plant-1, shoot length (SL) plant-1, root fresh weight (RFW) plant-1, shoot fresh weight (SFW) plant-1, root dry weight (RDW) plant-1, and shoot dry weight (SDW) plant-1] and physiological and biochemical characteristics including total chlorophyll (Total Chl), Chl degradation, leaf relative water content (RWC), concentration of malondialdehyde (MDA), hydrogen peroxide (H2O2), and Cys content, and enzymes activities (NR, OAS-TL, Rubisco, ATP-S, SOD, CAT and GR).
Measurement of growth characteristics
Plant height was recorded through a meter scale. After measuring the FW, the experimental plants were then kept in an oven run at 60°C. After 48 h, the DW of the plants was weighed.
Determination of physiological and biochemical parameters
Chlorophylls content were recorded by adopting the dimethyl sulfoxide method of Barnes et al. (Citation1992).
Chl degradation was expressed as the ratio between its absorbance at 435 and that at 415 nm (A435/A415), as suggested by Ronen and Galun (Citation1984).
Leaf relative water content (LRWC) was estimated according to the method of Yamasaki and Dillenburg (Citation1999) and calculated by adopting the following formula: RWC (%) = [(FW-DW)/(TW-DW0] x 100, where FW- leaf fresh weight, DW- leaf dry weight was taken after drying the leaves at 80 °C for 24 h; and turgid weight (TW) was measured after keeping the leaves in deionized water for 4 h.
Electrolyte leakage (EL) was measured according to the method of Lutts et al. (Citation1995).
Malondialdehyde (MDA) content was quantified according to the method of Heath and Packer (Citation1968). Leaves were homogenized in a solution containing 10% trichloroacetic acid and 0.65% 2-thiobarbituric acid. The extract was heated at 95°C for 60 min. after centrifuging at 10,000×g for 10 min. The absorbance of the supernatant was read at A532 and A600 against a reagent blank.
Endogenous hydrogen peroxide (H2O2) content in the leaves of treated and non-treated plants was measured as described by Velikova et al. (Citation2000).
Estimation of nutrients and Pb content in plants
For leaf N and P content, dried-leaf material of wheat plants was powdered and digested by a Kjeldahl method. Digested material was stored for the analysis of N and P in leaf. Leaf N and P contents were measured by the method of Lindner (Citation1944) and Fiske and Subba Row (Citation1925), respectively. Some mineral elements such as potassium (K), calcium (Ca) and magnesium (Mg), and heavy metal Pb were determined according to the Association of Official Analytical Chemistry methods (AOAC, Citation1984) using Atomic Absorption Spectrophotometer AA-675 Series.
Determination of nitrogen-assimilation enzyme activity
The activity of NR in fresh leaf was assayed using a method of Jaworski (Citation1971). Fresh leaves were sampled and frozen in liquid nitrogen, and then stored at – 80 °C. Samples were weighed and transferred to plastic vials containing 2.5 mL phosphate buffer (pH 7.5), 0.2 M potassium nitrate and 5% isopropanol solutions. After incubation of samples for 2 h in the dark at 30 °C, a mixture of 1% sulphanilamide and 0.2% NED-HCl (N-1-naphthylethylene-diamine dihydrochloride) was added to each vial. The absorbance was read at 540 nm after 20 min and then compared with that of the calibration curve. The activity of NR was expressed as nM NO2 h-1g-1 leaf FW.
Determination of activity of OAS-TL enzyme and Cys content
The activity of O-acetylserine (thiol) lyase (OAS-TL) and Cys content in leaves were determined spectrophotometrically according to the method of Riemenschneider et al. (Citation2005) and Gaitonde (Citation1967), respectively with slight modification. Soluble protein extract was prepared by homogenizing 100 mg leaf tissues with 1 mL of 20 mM Tris-HCl (pH 8.0). The homogenates were centrifuged at 13,000 ×g for 10 min. Enzyme extract (50 µL) was added to the reaction mixture (1 mL) containing OAS-TL activity (5 mM O-acetyl serine, 5 mM Na2S, 33.4 mM dithiotreitol, 100 mM TRIS/HCl (pH 7.5) Schmidt (Citation1990). The Cys concentration was estimated by adding Na2S to the reaction mixture and incubated for 30 min at 37 °C. After addition of acidic ninhydrin reagent, the samples were incubated at 100 °C for 10 min to allow color development and then cooled on ice. The absorbance was read at 560 nm after the stabilization of color complex by adding 900 mL 70% ethanol to the samples. The content of Cys in leaves was calculated using calibration curve obtained for pure Cys and the result was given as nmol g-1 FW.
Determination of sulfur-assimilation enzyme activity
The activity of ATP-S was determined according to the method of Lappartient and Touraine (Citation1996). Fresh leaves were homogenized in a reaction buffer containing 10 mM Na2EDTA, 20 mM Tris-HC1 (pH 8.0), 2 mM DTT, and approximately 0.01 g/mL insoluble PVP. After the centrifugation of homogenates at 20,000 ×g for 10 min at 4 °C, the supernatant was used for in vitro ATP-S assays by adding 0.1 mL of the enzyme extract to 0.5 ml of the reaction mixture, which comprised of 7 mM MgCl2, 5 mM Na2MoO4, 2 mM Na2ATP, and 0.032 units/mL of sulfate – free inorganic pyrophosphatase (Sigma) in an 80 mM Tris-HC1 buffer (pH 8.0). In parallel, the same enzymes extract was added to the same reaction solution except that Na2MoO4 was excluded, after which side-by-side incubations were carried at 37 °C for 15 min and then phosphate was determined calorimetrically.
Determination of enzyme activity involved in photosynthesis
Rubisco activity was determined by measuring NADH oxidation at 340 nm using a UV-vis spectrophotometer (SPEKOL 1500; Analytik Jena AG, Jena, Germany) (Usuda Citation1985). Ice-cold extraction buffer solution (100 mM Hepes – KOH, pH 7.5, 0.5 mM EDTA, pH 8.0, 10 mM potassium acetate, 5 mM DTT, 20 mM 0-mercaptoethanol, 5% [v/v] glycerol, 1% [w/v) PVP, 0.05% [w/v] Triton X-100, and 0.5 mM PMSF) and a mortar pestle were used for enzyme extraction by homogenizing of fresh leaf samples. To remove debris, centrifugation of the extract was done at 10,000 ×g for 10 min at 4 °C. Supernatant was added to the reaction mixture, which included 100 mM Tris-HCl (pH 8.0), 10 mM NaHCO3, 10 mM MgCl2, 0.2 mM NADH, 1 mM ATP, 1 mM EDTA, 5 mM DTT, 4 units of glyceraldehyde-3-phosphate dehydrogenase, and 4 units of 3-phosphoglycerate phosphokinase. NADH oxidation was initiated by adding the enzyme extract to 1 mM ribulose-1,5-bisphosphate (RuBP). The absorbance was read for 1 min after the reaction was stopped. Enzyme activity was presented in μmol CO2 fixed min-1 mg-1 protein. The quantification of protein was done using the method of Bradford (Citation1976).
Determination of antioxidant enzymes
To determine the activity of the antioxidant enzymes, using a pre-chilled mortar and pestle, fresh leaf samples were homogenized in a pre-cooled buffer (0.5% Triton X-100 and 1% polyvinylpyrrolidone in 100 mM potassium phosphate buffer, pH 7.0). The supernatant was stored at – 20 °C for the enzymatic assays after centrifugation of the homogenates at 15,000 × g for 20 min at 4°C. The following activities were determined using the methods of the listed authors: SOD by Giannopolitis and Ries (Citation1977); CAT by Aebi (Citation1984) and GR by Foyer and Halliwell (Citation1976). Enzyme activities were expressed as units of enzyme activity mg-1 protein.
Statistical analysis
Statistical analysis of the data was analyzed using SPSS, statistical software version 22.0 for Mac. A one-way analysis of variances (ANOVA) was used to compare the means, followed by Duncan’s multiple-range test (DMRT) to find significance (P < 0.05) differences between the individual means of the treatments.
Results
Under non-stress conditions, the growth performance of wheat plants in terms of RL, SL, RFW, SFW, RDW and SDW was gauged and recorded maximum with the application of both doses of ASC (0.2 and 0.6 mM) as compared to the plants treated with Pb and control plants (). However, the application of 0.6 mM ASC proved better by yielding higher values for these attributes than did 0.2 mM ASC. Under the Pb toxicity condition, the growth characteristics of the wheat plant were significantly decreased (). However, both levels of ASC substantially improved growth attributes but 0.6 mM ASC was more effective in alleviating Pb toxicity.
Table 1. Effect of ASC on root length (RL) plant-1, shoot length (SL) plant-1, root fresh weight (RFW) plant-1, shoot fresh weight (SFW) plant-1, root dry weight (RDW) plant-1 and shoot dry weight (SDW) plant-1 of wheat plants under Pb-toxicity condition.
The data in reveal that application of ASC increased the content of essential nutrients (N, P, K, Ca and Mg) and Cys in plants under non-stress conditions. The maximum content of these nutrients was recorded in plants treated with 0.6 mM of ASC. However, the concertation of these essential nutrients decreased and Cys and heavy metal Pb concentration increased under Pb exposure. On the contrary, application of ASC significantly improved the content of these nutrients and Cys and decreased Pb in leaf of Pb-treated wheat plants. However, application of 0.6 mM ASC was found more effective than 0.2 mM ASC in increasing the contents of nutrients and Cys and also in suppressing the concertation Pb in leaf of wheat plants ().
Table 2. Effect of ASC on the content of nitrogen (N), phosphorus (P), potassium (K), calcium (Ca), magnesium (Mg), cysteine (Cys) and lead (Pb) in wheat plants under Pb-toxicity condition.
Total Chl content was markedly enhanced in the leaves of wheat plants subjected to ASC (0.2 and 0.6 mM), while Chl degradation was decreased for both levels of ASC under non-stress condition ( A&B). The application of 0.6 mM of ASC yielded a higher total Chl value than did 0.2 mM of ASC. Wheat plants subjected to Pb had the lowest value for total Chl and highest value for Chl degradation; both differences were significant. However, total Chl content and Chl degradation were substantially affected at both the levels of ASC. A distinct enhancement in total Chl content and a decrease in Chl degradation were seen in response to 0.6 mM of ASC under Pb toxicity.
Figure 1. Effect of ASC on (A) Total Chl content, (B) Chl degradation, (C) Rubisco activity and (D) ATP-S activity in wheat plants under Pb-toxicity condition. Mean (±SE) was calculated from four replicates for each treatment. Bars with different letters are significantly different at P < 0.05, applying a Duncan Multiple Range Test.
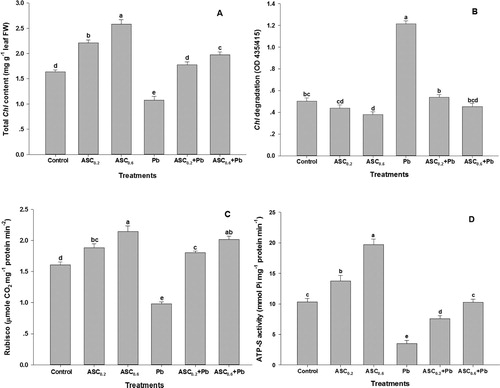
An increase in the activity of Rubisco and ATP-S was observed with the applications of 0.2 mM and 0.6 mM of ASC under non-stress condition ( C&D). However, a higher increase in the activity of both enzymes was observed with the application of 0.6 mM of ASC than with 0.2 mM of ASC. A considerable decrease in the activity Rubisco and ATP-S was noted in the plants subjected to Pb ( C&D). On the other hand, a substantial improvement in the activity of these enzymes was recorded in the plants that received any of the two doses of ASC under Pb toxicity. The maximum improvement in the activity of enzymes was observed in response to 0.6 mM of ASC rather than to 0.2 mM of ASC.
Under non-stress conditions, the activity of N and S –assimilation enzymes, NR and OAS-TL, respectively increased with the increasing dose of ASC as compared to the control ( A&B). Whereas, under Pb-stressed conditions, the activity of NR decreased, while OAS-TL increased as compared to the control. However, application of ASC improved the activity of NR and further enhanced OAS-TL activity in the plants exposed to Pb toxicity. Under Pb stress, the highest activity of both the enzymes was recorded in the plants treated with 0.6 mM of ASC as compared to 0.2 mM under Pb stress.
Figure 2. Effect of ASC on the activity of (A) nitrate reductase (NR) and (B) O-acetylserine (thiol) lyase (OAS-TL) in wheat plants under Pb-toxicity condition. Mean (±SE) was calculated from four replicates for each treatment. Bars with different letters are significantly different at P < .05, applying a Duncan Multiple Range Test.
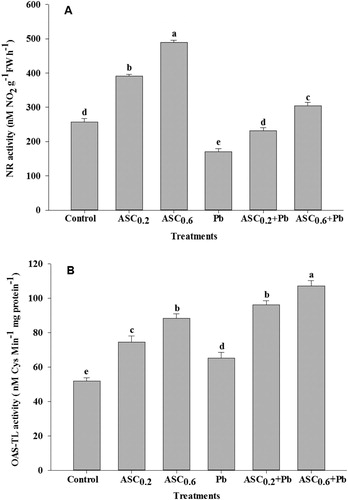
A significant increase in LRWC was observed in the plants treated with 0.2 and 0.6 mM of ASC under non-stress conditions ( A), but the effect was most notable with the application of 0.6 mM of ASC. Plants subjected to Pb had the lowest LRWC. However, plants that received both levels of ASC exhibited improved LRWC under Pb stress. The maximum improvement was recorded in Pb-stressed plants treated with 0.6 mM of ASC under Pb toxicity ( A).
Figure 3. Effect of ASC on (A) relative water content (RWC) and electrolyte leakage (EL), (B) Malondialdehyde (MDA) and (C) H2O2 content in wheat plants under Pb-toxicity condition. Mean (±SE) was calculated from four replicates for each treatment. Bars with different letters are significantly different at P < .05, applying a Duncan Multiple Range Test.
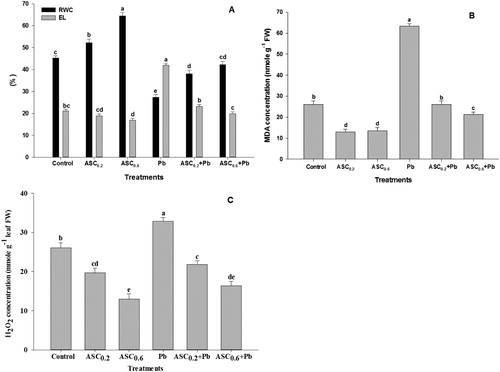
Under non-stress conditions, lower levels of EL and the accumulation of MDA and H2O2 were observed in the leaves of plants ( A-C), while, plants grown in growth medium containing Pb had the highest levels of EL, MDA, and H2O2. On the contrary, plants exhibited decreased EL and the content of MDA and H2O2 at both the levels of ASC under Pb-toxicity. Lower levels of EL, MDA, and H2O2 were observed with the application of 0.6 mM of ASC than with the application of 0.2 mM of ASC ( A-C).
A slight increase in the activity of SOD and CAT was observed in response to 0.2 mM ASC applications except for GR activity under non-stress condition, which showed a decrease compared to respective controls ( A-C). However, the activity of antioxidant enzymes was enhanced when plants were grown in a growth medium containing Pb. The activity of SOD, CAT, and GR was further enhanced in the plants treated with ASC under Pb toxicity. However, the highest enhancement in the activity of these enzymes was observed at 0.6 mM of ASC ( A-C).
Discussion
The growth parameters are important factors used to determine growth performance and crop productivity. In the present experiment, wheat plants exhibited enhanced growth attributes, such as RL, SL, RFW, SFW, RDW, and SDW with applied ASC doses (). These results are consistent with the findings of Sofy et al. (Citation2016). However, plants subjected to Pb showed reduced growth parameters compared with the control. This may be due to the toxic effect of Pb, which disturbs several physiological and biochemical processes such as ion toxicity, enzymes activity, respiration, and photosynthesis (Stevens et al., Citation1997; Hadi, Citation2015). Interestingly, under Pb toxicity, both levels of ASC improved growth characteristics (). Under Pb stress, the restoration of growth parameters by the application of ASC may be because ASC acts as an antioxidant and stimulates cell division and cell expansion by regulating the physiological and biochemical processes of plants (Ivanov, Citation2014; Kaviani, Citation2014;).
A balanced supply of nutrients is vital for normal plant growth and development under both stress and non-stress conditions. In the present experiment, content of Pb in leaf of wheat plants was higher under Pb stress (). Under Pb stress, plants had minimum content of essential nutrients. It may be due to the physical damage of absorption sites of roots which are unable to uptake many ions (Lamhamdi et al., Citation2013). However, application of ASC increased nutrients (N, P, K, Ca and Mg) content in both stressed and non-stressed plants (). These essential nutrients are the key growth limiting essential nutrients, which alleviate the adverse effect of different environmental stresses by improving physiological and molecular mechanisms of plants (Marschner Citation2002, Siddiqui et al., Citation2012a; Arshad et al., Citation2016). The increased concentrations of N, P, K, Ca and Mg in Pb-stressed plants treated with ASC might be one of the reasons for improved growth by increasing tolerance of wheat plants to Pb toxicity (). As we know that N, P, K, Ca and Mg play important roles directly or indirectly in cell division, cell enlargement and differentiation because all nutrients are the key constituents of many metabolically active compounds that regulate various physiological functions (Marschner Citation2002). Alone, K plays an important role in the activation of more than 50 enzymes ((Bhandal and Malik Citation1988). In this study, increased uptake of nutrients may be one of the reasons for decreased uptake of Pb by supporting plants to develop different types of physical barrier against uptake of Pb, resulting in better plants growth and development (). Siddiqui et al. (Citation2012b) reported that increased content of Ca and K in faba bean inhibited the uptake and deleterious effects of heavy metal cadmium. An increase in the content of nutrients may be responsible for the improvement of tolerance of plants to Pb toxicity by inducing mechanisms for detoxification and excretion of Pb to extra-cellular space (Siddiqui et al., Citation2012b; Ashraf et al., Citation2017).
Cys is one of the important metabolic precursors of essential biomolecules, such as vitamins, co-factors, antioxidants and defense compounds (Álvarez et al., Citation2012). Under Pb stress and non-stress conditions, ASC supplied to wheat plants increased Cys content (). Increased Cys might be due to the increased activity of OAS-TL in plants supplemented with ASC (B). The enzyme OAS-TL, compartmentalized in mitochondria, chloroplast and cytosol, plays an important role in the first step of Cys biosynthesis (Noda et al., Citation2016). Cys is formed in plants by the sequential reactions catalyzed by enzymes serine acetyl transferase and OAS-TL through incorporating a sulfide molecule into OAS molecule (Álvarez et al., Citation2012). An increase in the concentration of Cys due to the increased activity of OAS-TL might be responsible for tolerance of plant to Pb stress by serving as a donor of sulfur for sulfur-containing compounds that act as various antioxidants and defense compounds (Álvarez et al., Citation2012; Khan et al., Citation2017).
Chloroplast pigments are the key components for photosynthesis, and are responsible for biomass production in plants. We observed that supplied doses of ASC enhanced total Chl in plants under non-toxic conditions. However, plants subjected to Pb exhibited reduced biosynthesis of total Chl and increased Chl degradation ( A&B). A decrease in total Chl in plants subjected to Pb might be due to Chl degradation ( A) by enhanced activity of chlorophyllase, the disruption of chloroplast stroma volume induced by ROS (Stefanov et al., Citation1995; Hadi, Citation2015), or due to an imbalance in the supply of nutrients, such as Mg and iron (Burzynski, Citation1987). When ASC was added to the growth medium, the biosynthesis of total Chl was improved and Chl degradation decreased ( A&B). An increase in Chl in the leaves of plants may be due to the increased content of Mg induced by the application of ASC (). Mg is an essential element that attached as a central atom of the porphyrin ring of the photosynthetic pigments. Under Pb toxicity, increase in total Chl and decrease in Chl degradation by the application of ASC might be due to ASC’s effective role in the suppression of MDA and membrane permeability (EL) ( A&B). Additionally, ASC protects chloroplasts from oxidative damage by stimulating IAA and GA3 synthesis and by inhibiting ABA formation (Saeidi-Sar et al., Citation2013; Gul et al., Citation2015).
Rubisco is the first enzyme that participates in carbon fixation (the process by which plants convert atmospheric carbon dioxide to energy-rich macromolecules). Plants subjected to Pb had a significant reduction in the activity of Rubisco (C). However, the addition of ASC in the growth medium significantly improved the activity of Rubisco under Pb stress. Also, a substantial increase in Rubisco activity in plants was found with the application of ASC under non-stress conditions. This increase in the activity of Rubisco could be responsible for improved growth attributes () because Rubisco is the most abundant enzyme that constitutes half of the soluble proteins in the leaves of almost all plants and also regulates photosynthesis (Parry et al., Citation2007). Therefore, we postulated that exogenous application of ASC restored plant growth by improving the activity of Rubisco.
It is well established that ATP-S is the first enzyme involved in sulfate assimilation pathway (the process through which Cys is formed), resulting in the formation of S-containing compounds, such as glutathione, amino acids, and methionine (Giordano and Raven, Citation2014; Prioretti et al., Citation2014). In this experiment, plants treated with Pb showed reduced activity of ATP-S ( D). However, the application of ASC yielded significantly better results for the enzyme ATP-S over the control under non-stress and Pb stress conditions. Under Pb toxicity, the application of ASC mitigated the adverse effect of Pb by improving ATP-S activity, which could be responsible for the enhancement of S-containing compounds. These compounds might be the reason for the improved tolerance of plants to Pb toxicity. According to Couturier et al. (Citation2013), Cys and glutathione improve plant’s resistance to abiotic stress by regulating metabolic redox.
NR, a primary precursor for N-assimilation pathway, is induced by sulfate and the activity of ATP-S, an S-assimilation enzyme. Similarly, OAS-TL is a key enzyme for S-assimilation to produce Cys. In the present experiment, application of ASC increased both enzymes (NR and OAS-TL) under Pb stress and non-stress conditions ( A & B). Enhanced activity of NR and OAS-TL with the application of ASC ( A&B) might have served in boosting N and S –assimilation and antioxidant system (). Both enzymes activity could be helpful in improving the tolerance of wheat plants to Pb-toxicity. It is reported that NR induced nitric oxide (NO) synthesis that protects plants against abiotic stress (Sun et al., Citation2014; Khan et al., Citation2017).
The growth and physiological mechanisms of plants are directly or indirectly regulated by the supply of water. The exogenous application of Pb decreased LRWC (A), which may be due to inhibition of root growth or the alteration of cell wall extensibility and cell wall elasticity (Poschenrieder and Barceló, Citation1999). However, the application of ASC enhanced LRWC in both the groups of plants, the plants exposed to Pb and plants under non-toxic conditions. Enhanced LRWC provided sufficient amount of water required for various cellular activities leading to enhanced levels of total Chl and nutrients content, and activity of several enzymes. All these improved physiological processes together contributed to enhanced resistance of wheat plants to Pb toxicity. Our results are further supported by the finding that exogenous application of ASC enhanced the utilization and uptake of water in cucumber plants under drought stress (Naz et al., Citation2016).
Excessive generation of ROS is one of the fatal consequences of abiotic stress that causes denaturation of DNA, proteins, and carbohydrates, resulting in disordered physiological and biochemical processes. The accumulation of MDA and H2O2 were significantly greater in plants subjected to Pb ( B&C). These parameters indicate the level of oxidative damage induced by Pb toxicity. The formation of H2O2 causes membrane damage and induces a Haber–Weiss reaction, resulting in lipid peroxidation by forming hydroxyl radical in plants (Mittler, Citation2002). In order to perform normally under toxic conditions, plants must minimize the levels of ROS in cells by activating enzymatic and non-enzymatic antioxidant defense systems. In this investigation, the application of ASC was found to be effective in lowering the levels of MDA and H2O2 by improving plant immune systems associated with enhanced activity of SOD, CAT, and GR ( (A-C). These results validate the findings of Saeidi-Sar et al. (Citation2013) and Younis et al. (Citation2010). On the other hand, the exogenous application of ASC might be responsible for the inhibition of ROS formation due to its antioxidant properties. Increased activity of antioxidant enzymes (SOD, CAT, GR) in plants treated with ASC might be due to increased activity of N and S –assimilation enzymes (NR and OAS-TL) ( A&B). Increased activity of antioxidant enzymes could be responsible for improved tolerance to Pb-toxicity by protecting membrane functions (Abd_Allah et al., Citation2017). According to Lu et al. (Citation2014), NR induces production of NO that is responsible for the activity of antioxidant enzyme. Similarly, ASC-induced OAS-TL activity might be involved in maintaining S-assimilation and thiols levels, resulting in improved tolerance of plants to Pb stress. Therefore, it is clear that ASC is involved in the quenching of excess of ROS induced by Pb toxicity by enhancing the activity of antioxidant enzymes.
Conclusion
The present study describes that the application of ASC significantly countered Pb-induced ROS generation through enhancing the activities of antioxidant enzymes, N and S -assimilation by increasing the activity of NR and OAS-TL, content of essential nutrients (N, P, K, Ca and Mg) and Cys. Also, the activity of Rubisco and ATP-S and total Chl were increased in plants treated with ASC under Pb stress. Application of ASC mitigated the toxic effects of Pb-induced generation of ROS and causes reduction in H2O2 content and electrolyte leakage that caused reduction in lipid peroxidation and improvement in LRWC. Improved water status assisted stressed plants in enhancing Chl content and Rubisco activity that caused higher accumulation of organic matter, resulting in better growth of ASC treated plants even under Pb stress ().
Figure 5. Diagrammatic presentation of ASC (ascorbic acid) role in tolerance of plants to lead Pb (lead) toxicity. RWC – relative water content, NR – nitrate reductase, Rubisco – ribulose-1,5-bisphosphate carboxylase/oxygenase, ATP-S – ATP sulfurylase, OAS-TL – O-acetylserine(thiol)lyase, Chl – chlorophyll, Cys – Cysteine, ROS – reactive oxygen species.
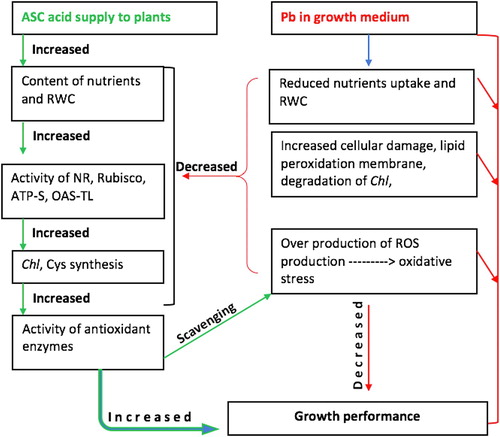
Conflict of interest
The authors declare that they have no conflicts of interest.
Acknowledgments
Financial support was provided by the Deanship of Scientific Research of King Saud University, Riyadh, Saudi Arabia.
Disclosure Statement
No potential conflict of interest was reported by the author(s).
ORCID
Manzer H Siddiqui http://orcid.org/0000-0003-2840-4608
Additional information
Funding
References
- Abd_Allah EF, Hashem A, Alqarawia AA, Wirth S, Egamberdieva D. 2017. Calcium application enhances growth and alleviates the damaging effects induced by Cd stress in sesame (sesamum indicum L.). J Plant Interact. 12(1):237–243. doi: 10.1080/17429145.2017.1319500
- Aebi H. 1984. Catalase in vitro. Methods Enzymol. 105:121–126. doi: 10.1016/S0076-6879(84)05016-3
- Akram NA, Shafiq F, Ashraf M. 2017. Ascorbic acid-a potential oxidant scavenger and its role in plant development and abiotic stress tolerance. Front Plant Sci. doi10.3389/fpls.2017.00613
- Álvarez C, Bermúdez MÁ, Romero LC, Gotor C, García I. 2012. Cysteine homeostasis plays an essential role in plant immunity. New Phytol. 193:165–177. doi: 10.1111/j.1469-8137.2011.03889.x
- Amari T, Ghnaya T, Abdelly C. 2017. Nickel, cadmium and lead phytotoxicity and potential of halophytic plants in heavy metal extraction. S Afr J Bot. 111:99–110. doi: 10.1016/j.sajb.2017.03.011
- Arshad M, Ali S, Noman A, Ali Q, Rizwan M, Farid M, Irshad MK. 2016. Phosphorus amendment decreased cadmium (Cd) uptake and ameliorates chlorophyll contents, gas exchange attributes, antioxidants, and mineral nutrients in wheat (triticum aestivum L.) under Cd stress. Arc Agron Soil Sci. 62:533–546. doi: 10.1080/03650340.2015.1064903
- Ashraf U, Kanu1 AS, Deng1 Q, Mo Z, Pan1 S, Tian H, Tang X. 2017. Lead (Pb) toxicity:physio-biochemical mechanisms, grain yield, quality, and Pb distribution proportions in scented rice. Front Plant Sci. 8:259. doi: 10.3389/fpls.2017.00259
- Association of Official Analytical Chemistry methods (AOAC). 1984. Association of official analytical chemistry. 14th ed. William Horwitz, editor. P.O. BOX 540. Franklin Station, Washington.
- Barnes JD, Balaguer L, Manrique E, Elvira S, Davison AW. 1992. A reappraisal of the use of DMSO for the extraction and determination of chlorophylls a and b in lichens and higher plants. Environ Exp Bot. 32:85–100. doi: 10.1016/0098-8472(92)90034-Y
- Bhandal IS, Malik CP (1988) Potassium estimation, uptake and its role in the physiology and metabolism of flowering plants. Int Rev Cytol. 110:205–254. doi: 10.1016/S0074-7696(08)61851-3
- Bradford MM. 1976. A rapid and sensitive method for the quantitation of microgram quantities of protein utilizing the principle of protein-dye binding. Anal Biochem. 72:248–254. doi: 10.1016/0003-2697(76)90527-3
- Brunold C, Suter M. 1984. Regulation of sulfate assimilation by nitrogen nutrition in the duckweed lemma minor L. Plant Physiol. 76:579–583. doi: 10.1104/pp.76.3.579
- Burzynski M. 1987. The influence of lead and cadmium on the absorption and distribution of potassium, calcium, magnesium and iron in cucumber seedlings. Acta Physiol Plant. 9:229–238.
- Couturier J, Chibani K, Jacquot JP, Rouhier N. 2013. Cysteine-based redox regulation and signaling in plants. Front. Plant Sci. 4:105.
- Dogan, M., Saygideger, S.D., Colak, U. 2009. Effect of lead toxicity on aquatic macrophyte elodea canadensis michx. Bull. Environ. Cont Toxicol. 83:249–254. doi: 10.1007/s00128-009-9733-5
- Fan T, Yang L, Wu X, Ni J, Jiang H, Zhang Q, Fang L, Sheng Y, Ren Y, Cao S. 2016. The PSE1 gene modulates lead tolerance in arabidopsis. J. Exp. Bot. 67:4685–4695. doi: 10.1093/jxb/erw251
- Fiske CH, Subba Row Y. 1925. The colorimetric determination of phos-phorus. J Biol Chem. 66: 375–400.
- Foyer CH, Halliwell B. 1976. The presence of glutathione and glutathione reductase in chloroplasts: a proposed role in ascorbic acid metabolism. Planta 133:21–25. doi: 10.1007/BF00386001
- Gaitonde MK. 1967. A spectrophotometric method for the direct determination of cysteine in the presence of other naturally occurring amino acids. Biochem J. 104:627–633. doi: 10.1042/bj1040627
- Giannopolitis CN, Ries SK. 1977. Superoxide dismutases. I. occurrence in higher plants. Plant Physiol. 59:309–314. doi: 10.1104/pp.59.2.309
- Giordano M, Raven JA. 2014. Nitrogen and sulfur assimilation in plants and algae. Aquat Bot. 118:45–61. doi: 10.1016/j.aquabot.2014.06.012
- Gul H, Ahmad R, Hamayun M. 2015. Impact of exogenously applied ascorbic acid on growth, some biochemical constituents and ionic composition of guar (cymopsis tetragonoloba) subjected to salinity stress. Pakhtunkhwa J Life Sci. 3:22–40.
- Hadi F. 2015. A mini review on lead (Pb) toxicity in plants. J Biol Life Sci. 6:91–101. doi: 10.5296/jbls.v6i2.7152
- Heath RL, Packer L. 1968. Photoperoxidation in isolated chloroplasts. I. kinetics and stoichiometry of fatty acid peroxidation. Arch Biochem Biophys. 125:189–198. doi: 10.1016/0003-9861(68)90654-1
- Ivanov BN. 2014. Role of ascorbic acid in photosynthesis. Biochem (Moscow). 79:282–289. doi: 10.1134/S0006297914030146
- Jaworski EG. 1971. Nitrate reductase assay in intact plant tissues. Biochem Biophy Res Commun. 43:1274–1279. doi: 10.1016/S0006-291X(71)80010-4
- Kaviani B. 2014. Effect of ascorbic acid concentration on structural characteristics of appical meristems on in vitro aloe barbadensis mill. Acta Sci Pol Hortorum Cultus. 13:49–56.
- Khan A, Iqbal I, Shah A, Ahmad A, Ibrahim M. 2010. Alleviation of adverse effects of salt stress in brassica (brassica campestris) by pre-sowing seed treatment with ascorbic acid. J Agric Environ Sci. 7:557–560.
- Khan MN, Mobin M, Abbas ZK, Siddiqui MH. 2017. Nitric oxide-induced synthesis of hydrogen sulfide alleviates osmotic stress in wheat seedlings through sustaining antioxidant enzymes, osmolyte accumulation and cysteine homeostasis. Nitric Oxide 68:91–102. doi: 10.1016/j.niox.2017.01.001
- Kopriva S, Rennenberg H. 2004. Control of sulphate assimilation and glutathione synthesis: interaction with N and C metabolism. J Exp Bot. 55:1831–1842. doi: 10.1093/jxb/erh203
- Lamhamdi M., El Galiou O., Bakrim A., Nóvoa-Muñoz J.C., Arias-Estévez M., Aarab A., Lafont R. 2013. Effect of lead stress on mineral content and growth of wheat (triticum aestivum) and spinach (spinacia oleracea) seedlings. Saudi J Biol Sci. 20:29–36. doi: 10.1016/j.sjbs.2012.09.001
- Lappartient AG, Touraine B. 1996. Demand-driven control of root ATP sulfurylase activity and S02-uptake in intact canola: The role of phloem-translocated glutathione. Plant Physiol. 111:147–157. doi: 10.1104/pp.111.1.147
- Leal-Alvarado DA, Espadas-Gil F, Sáenz-Carbonell L, Talavera-May C, Santamaría J.M. 2016. Lead accumulation reduces photosynthesis in the lead hyper-accumulator salvinia minima baker by affecting the cell membrane and inducing stomatal closure. Aquat Toxicol. 171:37–47. doi: 10.1016/j.aquatox.2015.12.008
- Lindner RC. 1944. Rapid analytical methods for some of the more common inorganic constituents of plant tissue. Plant Physiol. 19:76–89. doi: 10.1104/pp.19.1.76
- Lu S, Zhuo C, Wang X, Guo Z. 2014. Nitrate reductase (NR)-dependent NO production mediates ABA- and H2O2-induced antioxidant enzymes. Plant Physiol Biochem. 74:9–15. doi: 10.1016/j.plaphy.2013.10.030
- Lunde C, Zygadlo A, Simonsen HT, Nielsen PL, Blennow A, Haldrup A. 2008. Sulfur starvation in rice: the effect on photosynthesis, carbohydrate metabolism, and oxidative stress protective pathways. Physiol Plant. 134:508–521. doi: 10.1111/j.1399-3054.2008.01159.x
- Lutts S, Kinet JM, Bouharmont J. 1995. Changes in plant response to NaCl during development of rice (oryza sativa L) varieties differing in salinity resistance. J Exp Bot. 46:1843–1852. doi: 10.1093/jxb/46.12.1843
- Mahdavian K, Ghaderian SM, Schat H. 2016. Pb accumulation, Pb tolerance, antioxidants, thiols, and organic acids in metallicolous and non-metallicolous peganum harmala L. under Pb exposure. Environ Exp Bot. 126:21–31. doi: 10.1016/j.envexpbot.2016.01.010
- Malar S, Vikram SS, Favas PJC, Perumal V. 2016. Lead heavy metal toxicity induced changes ongrowth and antioxidative enzymes level in water hyacinths [eichhornia crassipes (mart.)]. Bot Stud. 55:213. doi: 10.1186/s40529-014-0054-6
- Marschner H. 2002. Mineral nutrition of higher plants, 2nd ed. London: Academic.
- Massoud M.B., Karmous I., El Ferjani E., Chaoui A. 2018. Alleviation of copper toxicity in germinating pea seeds by IAA, GA3, Ca and citric acid. J Plant Interact. 13:21–29. doi: 10.1080/17429145.2017.1410733
- Mendoza-Cózatl D, Loza-Tavera H, Hernández-Navarro A, Moreno-Sánchez R. 2005. Sulfur assimilation and glutathione metabolism under cadmium stress in yeast, protists and plants. FEMS Microbiol Rev. 29:653–671. doi: 10.1016/j.femsre.2004.09.004
- Mittler R. 2002. Oxidative stress, antioxidants and stress tolerance. Trends Plant Sci. 7:405–410. doi: 10.1016/S1360-1385(02)02312-9
- Naz H, Akram NA, Ashraf M. 2016. Impact of ascorbic acid on growth and some physiological attributes of cucumber (cucumis sativus) plants under water-deficit conditions. Pak J Bot. 48:877–883.
- Noda M, Nakamura M, Takamiya R, Tamura T, Ito T, Kodama H. 2016. A spinach O-acetylserine(thiol)lyase homologue, SoCSaseLP, suppresses cysteine biosynthesis catalysed by other enzyme isoforms. Biochim Open. 2:24–32. doi: 10.1016/j.biopen.2016.01.002
- Parry MAJ, Keys AJ, Madgwick PJ, Carmo-Silva AE, Andralojc PJ. 2007. RubisCO regulation: a role for inhibitors. J Exp Bot. 59:1569–1580. doi: 10.1093/jxb/ern084
- Poschenrieder C, Barcelo J. 1999. Water relations in heavy metal stressed plants. In: Prasad MNV, Hagemeyer J, editors. Heavy metal stress in plants from molecules to ecosystems. Berlin: Springer-Verlag; p. 207–229.
- Prioretti L, Gontero B, Hell R, Giordano M. 2014. Diversity and regulation of ATP sulfurylase in photosynthetic organisms. Front Plant Sci. 5:207–212. doi: 10.3389/fpls.2014.00597
- Riemenschneider A, Riedel K, Hoefgen R, Papenbrock J, Hesse H. 2005. Impact of reduced O-acetylserine(thiol)lyase isoform contents on potato plant meta- bolism, Plant Physiol. 137:892–900. doi: 10.1104/pp.104.057125
- Ronen R, Galun M. 1984. Pigment extraction from lichens with dimethyl sulfoxide (DMSO) and estimation of chlorophyll degradation. Environ Exp Bot. 24:239–245. doi: 10.1016/0098-8472(84)90004-2
- Ross SM. 1994. Sources and forms of potentially toxic metal in soil–plant systems. In: Ross SM, editor. Toxic metals in soil–plant systems. New York: John Wiley & Sons; p. 3–25.
- Rossato LV, Nicoloso FT, Farias JG, Cargnelluti D, Tabaldi LA, Antes FG, Dressler VL, Morsch VM, Schetinger MRC. 2012. Effects of lead on the growth, lead accumulation and physiological responses of pluchea sagittalis. Ecotoxicol. 21:111–123. doi: 10.1007/s10646-011-0771-5
- Saeidi-Sar S, Abbaspour H, Afshari H, Yaghoobi SR. 2013. Effects of ascorbic acid and gibberellin A3 on alleviation of salt stress in common bean (phaseolus vulgaris L.) seedlings. Acta Physiol Plant. 35:667–677. doi: 10.1007/s11738-012-1107-7
- Schmidt A. 1990. Sulphur metabolism. D. cysteine synthase. Methods Plant Bio-Chem. 3: 349–354. doi: 10.1016/B978-0-12-461013-2.50031-9
- Sharma P, Dubey RS. 2005. Lead toxicity in plants. Braz J Plant Physiol. 17:35–52. doi: 10.1590/S1677-04202005000100004
- Shen ZG, Li XD, Wang CC, Chen HM, Chu H. 2002. Lead phytoextraction from contaminated soil with high-biomass plant species. J Environ Qual. 31:1893–1900. doi: 10.2134/jeq2002.1893
- Siddiqui MH, Al-Whaibi MH, Sakran AM, Basalah MO, Ali HM. 2012b. Effect of calcium and potassium on antioxidant system of vicia faba L. under cadmium stress. Int J Mol Sci. 13:6604–6619. doi: 10.3390/ijms13066604
- Siddiqui MH, Mohammad F, Khan MMA, Al-Whaibi MH. 2012a. Cumulative effect of nitrogen and sulphur on brassica juncea L. genotypes under NaCl stress. Protoplasma 249:139–153. doi: 10.1007/s00709-011-0273-6
- Siddiqui MH, Mohammad F, Khan MN, Khan MMA. 2008. Cumulative effect of soil and foliar application of nitrogen, phosphorus, and sulfur on growth, physico-biochemical parameters yield attributes, and fatty acid composition in oil of erucic acid-free rapeseed-mustard genotypes. J Plant Nutr. 31:1284–1298. doi: 10.1080/01904160802135068
- Simonetti B, Schmidt ÉC, Pereira DT, Bouzon ZL, Ouriques LC. 2016. Effects of cadmium on the morphology, pigments, and ultrastructure of palisada flagellifera (ceramiales, rhodophyta) cultivated in vitro. Braz J Bot. 39:465–473. doi: 10.1007/s40415-016-0254-2
- Smith IK. 1980. Regulation of sulfate assimilation in tobacco cells: effect of nitrogen and sulfur nutrition on sulfate permease and oacetylserine sulfhydryllase. Plant Physiol. 66:877–883. doi: 10.1104/pp.66.5.877
- Smith GS, Johnston CM, Cornforth IS. 1983. Comparison of nutrient solutions for growth of plants in sand culture. New Phytol. 94:537–548. doi: 10.1111/j.1469-8137.1983.tb04863.x
- Sofy MR, Sharaf AEM, Fouda HM. 2016. Stimulatory effect of hormones, vitamin C on growth, yield and some metabolic activities of chenopodium quinoa plants in Egypt. J Plant Biochem Physiol. 4:161.
- Stefanov K, Seizova K, Popova I, Petkov VL, Kimenov G, Popov S. 1995. Effects of lead ions on the phospholipids composition in leaves of Zea mays and phaseolus vulgaris. J Plant Physiol. 147:243–246. doi: 10.1016/S0176-1617(11)81511-7
- Stevens RG, Creissen GP, Mullineaux PM. 1997. Clonning and characterization of a cytosolic glutathione reductase cDNA from pea (pisum sativum L.) and its expression in response to stress. Plant Mol Biol. 35:641–654. doi: 10.1023/A:1005858120232
- Sun C, Lu L, Liu L, Liu W, Yu Y, Liu X, Hu Y, Jin C, Lin X. 2014. Nitrate reductase-mediated early nitric oxide burst alleviates oxidative damage induced by aluminum through enhancement of antioxidant defenses in roots of wheat (triticum aestivum). New Phytol. 201:1240–1250. doi: 10.1111/nph.12597
- Upadhyay RK. 2014. Metal stress in plants: its detoxification in natural environment. Braz J Bot. 37:377–382. doi: 10.1007/s40415-014-0087-9
- Usuda, H. 1985. The activation state of ribulose 1,5-bisphosphate carboxylase in maize leaves in dark and light. Plant Cell Physiol. 91:1455–1463.
- Velikova V, Yordanov I, Edreva A. 2000. Oxidative stress and some antioxidant systems in acid rain-treated bean plants. Plant Sci. 151:59–66. doi: 10.1016/S0168-9452(99)00197-1
- Venkatesh J, Park SW. 2014. Role of L-ascorbate in alleviating abiotic stresses in crop plants. Bot Stud. 55:38. doi: 10.1186/1999-3110-55-38
- Yamasaki S, Dillenburg LC. 1999. Measurements of leaf relative water content in araucaria angustifolia. R Bras Fisiol Veg. 11:69–75.
- Younis ME, Hasaneen MN, Kazamel AM. 2010. Exogenously applied ascorbic acid ameliorates detrimental effects of NaCl and mannitol stress in vicia faba seedlings. Protoplasma. 239:39–48. doi: 10.1007/s00709-009-0080-5