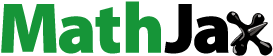
ABSTRACT
Understanding the effects of arbuscular mycorrhizal fungi (AMF) under different environmental contexts on overyielding in intercropping systems can be instructive in optimizing productivity and ecosystem services. A greenhouse study was conducted with maize and faba bean monocultures or intercropping at low phosphorus (P) and high P levels with three different water availabilities, and inoculated with or without AMF species Funneliformis mosseae. At low P level, overyielding was mainly due to the increase of maize biomass promoted by AMF relative to faba bean. Whereas in high P soils, overyielding was observed at all treatments, regardless of AMF. Inoculation of AMF significantly improved maize rather than faba bean P uptake, water use efficiency and photosynthesis rate, in particular at the low P level. This study identified the context dependence of AMF in influencing overyielding in maize/faba bean intercropping and demonstrates the importance of AMF in sustainable agricultural production.
Introduction
Intercropping has become an increasingly utilized practice designed to improve productivity and sustain agriculture development (Bedoussac et al. Citation2015). While intercropping can result in overyielding (i.e. increased yield relative to monoculture) (Li et al. Citation2014; Brooker et al. Citation2015), the underlying mechanisms of overyielding remain largely unexplored. Overyielding in intercropping is predominantly attributed to complementary resource use (Vandermeer Citation1992; Li et al. Citation2006) or interspecific facilitation (Hauggaard-Nielsen and Jensen Citation2005; Li et al. Citation2014; Muler et al. Citation2014). Furthermore, overyielding can depend on environmental contexts (Brooker et al. Citation2008). Under extreme (e.g. arctic, alpine or semi-arid) environmental conditions, net beneficial (facilitative) interactions are more common, while competition is dominant in productive (e.g. mesic) environments (He et al. Citation2013; Brooker et al. Citation2016). Hence, overyielding in intercropping is greater in low-input/resource-limited agricultural systems compared to high input systems (Vandermeer Citation1992; Li et al. Citation2007; Brooker et al. Citation2015). For example, the beneficial effects of maize/faba bean intercropping have been observed with lower phosphorus (P) fertilization but not with high P rates (Li et al. Citation2007). However, the extent to which environmental contexts influence intercropping and the underlying mechanisms of overyielding are still not well understood.
Many microorganisms are involved in soil biogeochemical processes (van der Heijden et al. Citation2008). Consequently, they also contribute to plant-plant interactions and overyielding (Duchene et al. Citation2017; Mariotte et al. Citation2018). Arbuscular mycorrhizal fungi (AMF) are ubiquitous soil symbionts that associate with approximately 80% of terrestrial plant species (Smith and Read Citation2008). AMF play a significant role in affecting interspecific interactions and plant community structure and productivity (Bever et al. Citation2010; Hodge and Fitter Citation2013; van der Heijden et al. Citation2015). For example, they have been shown to promote plant community diversity by improving competitive ability of subordinate plant species compared with dominants (van der Heijden et al. Citation1998; Vogelsang et al. Citation2006), and reduce competitive inequality between the legume Trifolium pratense and the grass Lolium multiflorum by reducing the growth suppression of the legume by the grass (Wagg et al. Citation2011). In agricultural flax and sorghum intercropping, AMF relaxed competition and generated overyielding by improving the growth of flax while sorghum was unaffected (Walder et al. Citation2012). By contrast, common mycorrhizal networks have been found to amplify interspecific plant competition. In ecosystems where the dominant plants strongly benefit form mycorrhizal fungi, such as in tall grass prairie, AMF have been shown to reduce species coexistence (Hartnett and Wilson Citation1999). Well established plants can hold competitive advantage over seedlings by common mycorrhizal networks due to increased access to nutrients (Merrild et al. Citation2013; Weremijewicz et al. Citation2016). Furthermore, resource input has been shown to affect plant response to mycorrhizal fungi (Hoeksema et al. Citation2010). For example, AMF were important for mediating plant community diversity with low but not with high P availability (Collins and Foster Citation2009). However, the influence of AMF in regulating interspecific interactions in intercropping under different environmental contexts remains poorly understood (Eisenhauer Citation2012; Ehrmann and Ritz Citation2014).
Extreme weather events are predicted to increase the frequency of drought and other abiotic stressors (Mpelasoka et al. Citation2008). The potential effects of AMF in favoring host plants resisting drought are well acknowledged, as shown to be important in affecting water relations of host plants, which is attributable to nutritional (e.g. improved P nutrition) and/or non-nutritional effects including stomatal conductance and osmotic adjustment, etc. (Augé Citation2001; Ruiz-Lozano Citation2003). Besides, AMF are also involved in the formation and maintenance of soil water-stable aggregates (Zhu and Miller Citation2003; Rillig and Mummey Citation2006), which potentially improve water retention by holding water both inside aggregate pores and between aggregate particles (Ruiz-Lozano Citation2003; Sun and Lu Citation2014). Within intercropping systems, both water use and the effect of AMF on water use have not been thoroughly studied. Increased (Yang et al. Citation2011; Chen et al. Citation2018) and decreased (Szumigalski and Van Acker Citation2008; Xu et al. Citation2008; Gao et al. Citation2009) water use may depend on plant intercropping sytems, and intercropped row management (Mao et al. Citation2012). Whether the variation of water use efficiency in intercropping highlighted in these studies is regulated by AMF remains unknown. Previously, AMF are shown to have the potential to reallocate water through shared common mycelial networks between plants to improve water use efficiency (Egerton-Warburton et al. Citation2007) and reduce interspecific competition for water. Alternatively, AMF could amplify competition and preferentially allocate more water to mycorrhizal-dependent plants.
As a scarce and nonrenewable resource, P has poor solubility and mobility in soils, and P acquisition by plants decreases when soil moisture declines (Sardans and Peñuelas Citation2004). Thus, under drought conditions, plants are subjected to both low-moisture and low-P availability. To investigate how AMF regulate both water and P use in intercropping under different environmental contexts, we investigated the effect of AMF (Funneliformis mosseae) on plant interactions and overyielding in maize/faba bean intercropping. We utilized three different water treatments (well-watered, droughted and alternative well-watered and droughted) and two P supply levels (10 and 100 mg kg−1). We selected maize/faba bean intercropping because it is a widely accepted interspecific facilitation system that faba bean benefits P uptake of maize (Li et al. Citation2007), while maize benefits nodulation formation and N2 fixation of faba bean (Li et al. Citation2016). Both faba bean and maize are responsive to mycorrhizal fungi (Qiao et al. Citation2016), and AMF may affect plant-plant interactions by mediating plant nutrient and water uptake. In the present study, we hypothesized that (1) the presence of AMF would enhance overyielding by alleviating competition between plants for limited P and/or water resources; and (2) the AMF effect under low P and water deficient conditions was greater compared to that at high P and sufficient water conditions.
Materials and methods
Soil, plant material and AMF inoculum
The soil used was collected from field plots at the Shangzhuang Experimental Station (39°59ʹN, 116°17ʹE) of China Agricultural University, Beijing, China. The soil is silt loam with a pH of 7.73 (1:5, soil to water) and field capacity of 24.7% (w/w). The soil contained 13.7 g kg−1 organic matter, 41.0 mg kg−1 mineral nitrogen (Nmin), 11.1 mg kg−1 available phosphorus (Olsen-P), and 98.4 mg kg−1 NH4OAC-potassium (K). Soil was air-dried, sieved through 2 mm and mixed with river sand (soil:sand, 4:1 w/w), and then was sterilized by γ-irradiation (25 kGray, Beijing Radiation Application Research Center). Based on previous experience, sufficient mineral (N and K) nutrients other than P were mixed thoroughly into the sterilized soil at the following concentrations (mg kg−1 soil): Ca (NO3)2-N 200 and K2SO4-K 200. Two P (Ca(H2PO4)2 · H2O) levels of 10 (low P) and 100 mg P kg−1 (high P) were set up based on previous experimental results for P requirement of maize grown in pots (Chu et al. Citation2013).
Seeds of maize (Zea mays L. cv. Zhengdan-958) and faba bean (Vicia faba L. cv. 89147) were surface-sterilized with 10% v/v hydrogen peroxide for 30 min, washed with deionized water and germinated in the dark on moist filter paper at 25°C for 60 h (faba bean) or 48 h (maize) before sowing. Seeds were selected for uniformity prior to sowing. Funneliformis mosseae (BGCXZ01), whose former nomenclature was Glomus mosseae, was provided by the Bank of Glomeromycota of China, Beijing Academy of Agriculture and Forestry Sciences. The inoculum consisted of spores, mycelium and fine root segments and was propagated in a 1:1 (v/v) mixture of zeolite and river sand with clover (T. pratense L.) grown for four months in a greenhouse. Seventy grams (approximately 2000 spores) were added to each inoculated pot, and uninoculated pots were supplied with an equal amount of sterilized inoculum (121°C for 2 h). To minimize differences in microbial communities between mycorrhizal and non-mycorrhizal soils, 5 mL of AMF-free soil filtrate was added to each non-mycorrhizal pot. The filtrate was prepared from 100 g of inoculum (non-sterile) soaked in 500 mL deionized water, filtered through filter paper (Whatman No. 4) and diluted to 800 mL.
Round plastic pots (2 L) were used and partitioned into three layers. The bottom layer was filled with 1.2 kg sterilized background soil, followed by 70 g AMF or sterilized inoculum. Afterwards, another 800 g of sterilized soil was added as the top layer. Faba bean plants were inoculated with Rhizobium leguminosarum bv. NM353 (provided by the Culture Collection of China Agricultural University). The germinated seeds of faba bean were soaked with the Rhizobium inoculation liquid for 30 min prior to sowing. In addition, 5 mL of liquid Rhizobium inoculum was also added when transferring the faba bean into the pots. Pre-germinated seeds of maize and faba bean were sowed below the soil surface at depths of 1.5 and 3 cm respectively. Seedlings were thinned to two individuals per pot for maize and faba bean, or 1:1 (maize: faba bean) in intercropping after emergence. Plant density of two individuals for maize and faba bean monoculture, and one individual for each in intercropping was based on a previous study (Li et al. Citation2007). Plants were harvested after 64 days of growth when faba bean started flowering.
Experimental design
The experiment was conducted in a greenhouse at China Agricultural University, Beijing, China. The light intensity was approximately 800–1200 μmol m−2 s−1 with a day-night temperature range of 20–30°C and a 60–80% relative humidity. There were 36 treatments in total: 3 cropping systems (maize, faba bean and their intercropping), 2 P levels (10 and 100 mg kg−1), 2 AMF treatments (inoculation and non-inoculation) and 3 water treatments (well-watered, alternative well-watered and droughted, and droughted). By using randomized-block design, each treatment had six replicates, totaling 216 pots. For three water treatments, soil water content was maintained at 70–80% of field capacity (FC) for well-watered treatments (W), and 30–40% for droughted treatments (D). Alternative well-watered and droughted treatments (W–D) were maintained by wetting and drying the soil alternatively with a week as a cycle. One W–D cycle started with a rewetting to recover soil to the well-watered situation (70–80% of FC), and after evapotranspiration, maintaining the soil in the drought condition (30–40% of FC) until the next cycle started. All plants were grown in W condition for the first 21 days after sowing (DAS), afterwards two thirds of pots of each treatment (144 pots in total) was exposed to droughted treatment, and from 42 DAS on, half of the droughted treatments (72 pots) was subjected to alternative well-watered and droughted treatment. All pots were weighted and irrigated daily with deionized water to maintain the designated soil moisture content and daily watering quantity was recorded.
Measurements, harvest and calculations
Plant growth, P uptake and water use efficiency
Net photosynthesis rate, stomatal conductance and respiration rate of plants were measured using Li-Cor 6400 (Li-Cor, Lincoln, NE, USA) portable photosynthesis measurement system. Upper surface of the top full expanded leaf of plants was chosen for measurement at 44 DAS (2 days after implementing W–D treatment) between 9 am and 12 am on a sunny day. Measurements were taken by maintaining leaf chamber at 26.5°C, a CO2 concentration of 390 ppm, 60% relative humidity and 1200 µmol m−2 s−1 of photosynthetically active radiation. Four replicates of each treatment were used considering measuring them at the same day.
When harvesting, plant shoots were cut off, and roots were firstly gently washed to rinse away soil particles using tap water and then washed again with deionized water. Approximately 0.5 g of fresh roots was sampled to assess AMF colonization. The shoots and remaining roots of plants were oven-dried (72 h, 80°C) for determination of biomass. Because shoot biomass showed similar trend with total biomass (see Results), we chose shoot part to measure the crop P uptake. Shoot samples were ground and digested in a mixture of concentrated H2SO4 and H2O2 and P concentration was determined using the molybdo-vanado method (Thomas et al. Citation1967).
Water use efficiency (WUE) is calculated according to Chen et al. (Citation2018).
(1)
(1)
where SB and ET represent shoot biomass and evapotranspiration, respectively.
(2)
(2)
where I is irrigation amount, ΔW is the difference of soil water content between start point and end point and D is the drainage water from the pot. The drainage water in the bottom of the pots was negligible, as the water supply level was carefully calculated and did not have obvious drainage from the soil. In intercropping, maize and faba bean WUE could not be calculated separately, so we measured maize monoculture, faba bean monoculture and the intercropping WUE, respectively.
Root colonization and hyphal length density (HLD)
AMF colonization was measured according to grid intersections method (McGonigle et al. Citation1990). Root segments were checked under a compound microscope at 200× magnification after clearing washed roots in 10% KOH (w/v) and staining with 0.05% (v/v) trypan blue in lactic acid. Thirty pieces of root from each sample were randomly selected and each piece of root was intersected and scored three times, resulting in 90 root scores for each sample. The number of colonized roots divided by total number of roots examined was calculated as the colonization rate.
Sub-soil samples in rhizosphere (soil tightly adhering roots, separated by shaking method) were collected with a brush for the determination of hyphal length density (HLD) of AMF. The HLD was determined according to the method of Jakobsen et al. (Citation1992). Briefly, 5 g soil was blended at high speed with 250 mL of deionized water for 30 s and then poured through a 300 µm sieve to retain the roots. The blended suspension was rapidly transferred to a flask and was agitated vigorously by hand shaking and left on the bench for 60 s, and then 5 mL aliquots were pipetted onto 25 mm Millipore filters (0.45 µm pore size). The filter was stained with lactoglycerol-Trypan blue for 5 min and transferred to microscope slides to dry. Three filters from each original sample were counted under a microscope blue and intersections between hyphae and the grid were counted in 25 fields of view at 200× magnification.
Aggregate size distribution
Soil water-stable aggregates were separated by the wet sieving method (Wilson et al. Citation2009). This was achieved by wet sieving soils through a nest of sieves of appropriate sizes. Five aggregate size classes were collected from each sample (>2000, 1000–2000, 500–1000, 250–500 and 106–250 µm diameter). The 106–250 µm size fraction was defined as microaggregates, while the size >250 µm fractions were designated as macroaggregates. Fifty gram subsamples of air-dried soil were placed on the top sieve of each set. One liter of distilled water was added to each cylinder until the soil sample and top screen were covered with water. The soils were submerged in water for 10 min before wet sieving. Oscillation time (10 min), stroke length (4 cm) and frequency (30 cycles min−1) were held constant. All aggregate fractions were oven-dried, weighted and the proportion of soil macroaggregatess (>250 µm) was calculated.
Statistical analysis
To allow comparison of biomass between monocultures and intercropping (overyielding), we used the mean of two individuals in maize or faba bean monoculture and compared this with one individual per plant species in intercropping. To meet assumptions of homogeneity of variance, biomass, P uptake and root: shoot ratio were log-transformed prior to analysis. We analyzed data with a MIXED procedure model for ANOVA using of SAS version 9.2. Planting patterns (intercropping and monoculture), plant species (maize and faba bean), P (low and high), water treatments (W, W–D and D) and AMF (non- and inoculated) and their interactions were fixed effects. To control the variation for having two plant species in the intercrop treatment, block was identified as a random effect. The significant biomass difference in planting patterns (between intercropping and monoculture) indicated overyielding effect. Effects of planting patterns, P levels, water treatments and their synergistic effects on respective maize and faba bean AMF colonization were also analyzed using MIXED model for ANOVA. In intercropping, WUE, soil macroaggregates and AMF hyphal length density of maize and faba bean could not be measured separately, therefore, cropping systems (maize, faba bean monoculture and intercropping) were used as factors for the ANOVA analysis. General linear model (GLM) was used in SAS for the analysis of WUE, soil macroaggregates and AMF hyphal length density, with the effects resulting from cropping systems, P levels, water treatments and/or AMF and their synergistic effects. Comparisons between means were conducted using Tukey’s honestly significant difference (HSD) test at the 5% (P < .05) level.
The structural equation model (SEM) was constructed to investigate the causal relationships among P uptake, WUE, photosynthesis rate, stomatal conductance and respiration rate which were suggested to affect plant growth and biomass. Because there is not AMF infection and hyphae in the non-AMF treatment, the variables of colonization rate and HLD were not included in the model, which might lose some power of predicting mycorrhizal effects. However, as AMF are important in improving P uptake and WUE (see details in results), the importance of AMF can be indirectly reflected by these two variables. In order to create the same metrics, crop biomass, P uptake and photosynthetic parameters (photosynthetic rate, stomatal conductance and respiration rate) of maize and faba bean in intercropping was averaged. The model was constructed in AMOS 21.0 (Arbuckle Citation2006). We tested the fitness of the model with the data using the maximum likelihood estimation, with Chi-square (χ2), root mean square error of approximation (RMSEA) and goodness of fit index (GFI) used to evaluate model fit based on the Bollen–Stine bootstrap test.
Results
Plant biomass and overyielding
Overall, average total plant biomass of maize and faba bean were greater in intercropping than in monoculture (P < .0001) and this overyielding effect was significantly influenced by AMF and P interactions (P = .045). At the low P level, intercropping overyielding was significantly influenced by AMF (Table S1), showing a 19.8% total biomass increase in the presence of AMF compared to the monoculture ((A)). Overyielding effect was not influenced by AMF at high P level, with a respective 18.5% higher biomass in intercropping than monoculture in both AMF and non-AMF inoculation treatments ((A)).
Figure 1. Comparison of averaged total biomass (mean + SE, n = 72) of maize and faba bean inoculated with or without AMF and grown in monoculture (Mono) and intercropping (Inter) (A); average total biomass (mean + SE, n = 6) of maize and faba bean grown in monoculture (Mono) and intercropping (Inter) under the low P (B) and high P (C) treatments. W, W–D and D represent well-watered (W), alternative well-watered and droughted (W–D) and droughted treatments (D) respectively. The same lowercase and uppercase letters indicate that faba bean or maize total biomass do not differ significantly among different planting patterns with and without AMF inoculation in each water treatment according to Tukey’s HSD test at P < 0.05.
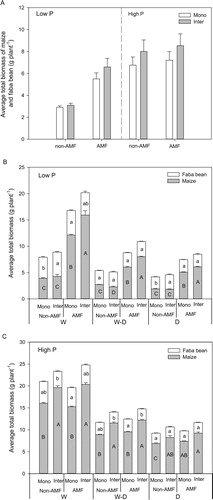
For each species, all four factors (AMF, P supply level, planting patterns and water) significantly affected maize total biomass, whereas the biomass of faba bean was mainly affected by water (Table S2). The total biomass of both maize and faba bean decreased with the decline of water supply levels regardless of P level, planting patterns, or mycorrhizal inoculation ((B, C)). At low P level, AMF significantly improved maize total biomass and this benefit was greater in intercropping than in monoculture ((B)). P addition generally increased maize total biomass, and in high P treatments, intercropping significantly increased maize biomass regardless of the AMF treatment (Table S2, (C)). In contrast to maize, the biomass of faba bean was generally not affected by AMF or P levels (Table S2).
The maize and faba bean shoot and root biomass at low P level, and shoot biomass at high P level showed the same trend that we found with total plant biomass (Figure S1(A, B, C)). At high P level, the presence of AMF generally decreased maize root biomass in all water treatments (Figure S1(D)). Irrespective of P levels, the presence of AMF tended to decrease the root: shoot ratio of maize, and the ratio was not significantly affected by planting patterns (Figure S2(A, B)). Similarly, planting patterns generally did not have significant influence on root: shoot ratio of faba bean at low P level, while AMF inoculation decreased root: shoot ratio in the W–D treatment at both P levels (Figure S2(C, D)).
P uptake, water use efficiency (WUE) and photosynthetic performances
At the low P level, compared with non-AMF treatment, inoculation of AMF significantly improved maize P uptake at all three water treatments, and when inoculating AMF, maize P uptake in intercropping was significantly higher than monoculture ((A)). At the high P level, only at W–D treatment, AMF inoculation improved maize P uptake and intercropping effect was significant ((B)). For faba bean, on average, AMF effect was only significant in low P and W treatment, showing a higher monoculture P uptake than non-AMF treatment ((C)). At low P level with AMF inoculation, monoculture P uptake was higher than intercropping at three water treatments ((C)), while at high P level, monoculture P uptake was higher than intercropping at non-AMF treatments ((D)).
Figure 2. P uptake (mean + SE, n = 6) of maize and faba bean in monoculture (Mono) and intercropping (Inter) at low P (A, C) and high P (B, D) supply levels. W, W–D and D represent well-watered (W), alternative well-watered and droughted (W–D) and droughted treatments (D) respectively. Bars topped by the same uppercase letters do not differ significantly among average photosynthesis rate of monoculture and intercropping under different AMF and water treatments according to Tukey’s HSD test at P < 0.05. Bars topped by the same lowercase letters do not differ significantly among different planting patterns with and without AMF inoculation in each water treatment at P < 0.05 according to Tukey’s HSD test.
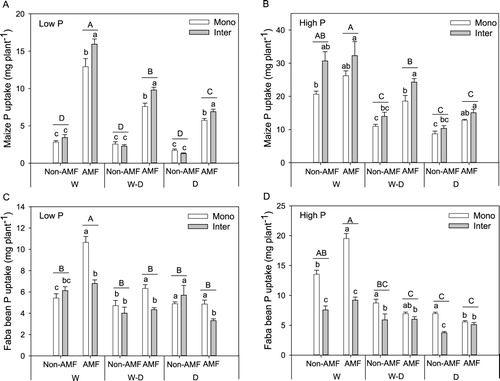
On average, P supply levels, inoculation with AMF and water treatments significantly affected the water use efficiency (WUE) of maize plants, whereas faba bean WUE was only affected by AMF (Table S3). At low P level, AMF significantly increased WUE in both maize monoculture and intercropping, and WUE had the highest value under well-watered (W) conditions and decreased with the severity of drought stress ((A)). At high P level, WUE of maize monoculture and maize/faba bean intercropping were slightly improved by AMF inoculation ((B)). Faba bean monoculture WUE was not significantly affected by P levels or water treatments (Table S3).
Figure 3. Water use efficiency (WUE) (mean + SE, n = 6) in maize (M) and faba bean (F) grown in monoculture or intercropping (M/F) at low P (A) and high P (B) supply levels. W, W–D and D represent well-watered (W), alternative well-watered and droughted (W–D) and droughted treatments (D) respectively. Bars topped by the same uppercase letters do not differ significantly among average WUE of the three water treatments in the two AMF and three cropping system treatments according to Tukey’s HSD test at P < 0.05. Bars topped by the same lowercase letters do not significantly differ among water treatments with and without AMF inoculation in each cropping system at P < 0.05 according to Tukey’s HSD test.
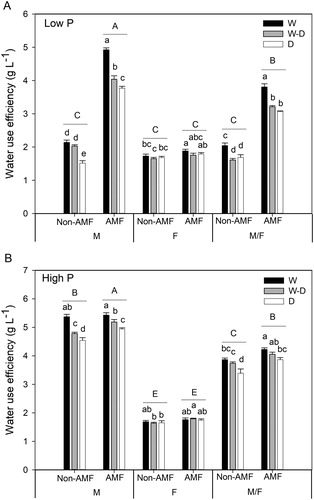
Drought treatment (D) significant decreased photosynthesis rate, stomatal conductance and transpiration rate of both crops, and well-watered (W) and rewetting (W–D) tended to improve the photosynthetic performances, in particular for maize at both P levels and faba bean at low P level (, 5, S3). AMF inoculation generally improved photosynthesis rate ((A, B)), stomatal conductance ((A, B)) and transpiration rate (Figure S3(A, B)) of maize at both P levels, and these effects were greater at lower P than at high P level. However, AMF only showed significant effect on faba bean photosynthetic performances at low P level rather than at high P level (panel C and D in , 5, S3). Intercropping tended to decrease photosynthetic performances of maize in W–D and D treatments without AMF inoculation at low P level (panel A in , 5, S3). In contrast, intercropping improved photosynthetic performances of maize in W treatment at high P level when inoculating AMF (panel B in , 5, S3). As for faba bean, intercropping did not have a significant effect except tended to improve photosynthesis rate in D treatments at both P levels ((C, D)).
Figure 4. Photosynthesis rate (mean + SE, n = 4) of maize and faba bean in monoculture (Mono) and intercropping (Inter) at low P (A, C) and high P (B, D) supply levels. W, W–D and D represent well-watered (W), alternative well-watered and droughted (W–D) and droughted treatments (D) respectively. Bars topped by the same uppercase letters do not differ significantly among average photosynthesis rate of monoculture and intercropping under different AMF and water treatments according to Tukey’s HSD test at P < 0.05. Bars topped by the same lowercase letters do not differ significantly among different planting patterns with and without AMF inoculation in each water treatment at P < 0.05 according to Tukey’s HSD test.
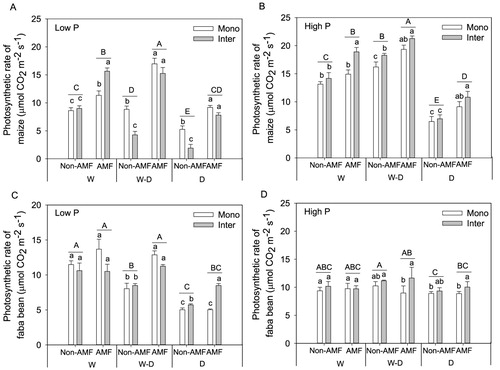
Figure 5. Stomatal conductance (mean + SE, n = 4) of maize and faba bean in monoculture (Mono) and intercropping (Inter) at low P (A, C) and high P (B, D) supply levels. W, W–D and D represent well-watered (W), alternative well-watered and droughted (W–D) and droughted treatments (D) respectively. Bars topped by the same uppercase letters do not differ significantly among average stomatal conductance of monoculture and intercropping under different AMF and water treatments according to Tukey’s HSD test at P < 0.05. Bars topped by the same lowercase letters do not differ significantly among different planting patterns with and without AMF inoculation in each water treatment at P < 0.05 according to Tukey’s HSD test.
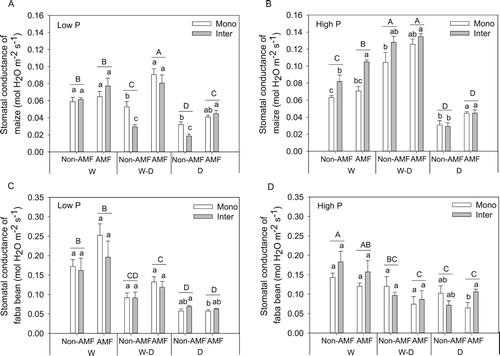
Relationships between observable variables and plant biomass
Our hypothesized SEM fit well with the data at both low P ((A)) and high P levels ((B)). The models explained 88% and 87% of the variance for plant biomass at low P and high P respectively. Regarding the total effects, P uptake was the strongest predictor for crop biomass, followed by WUE, and the WUE effect was larger at low P than at high P level (Table S4). Besides, P uptake affected biomass indirectly by mediating WUE ((A, B)). The photosynthetic rate did not exert significant effect on plant biomass. The direct effects of P uptake on the photosynthesis rate and stomatal conductance were weak, but the indirect effects of P uptake on photosynthesis rate were stronger by affecting the stomatal conductance and respiration rate ((A, B)).
Figure 6. Effects of five variables on crop biomass at low P (A) and high P level (B). The width of arrows indicates the strength of the causal effect. Bold and dashed lines indicate significant (P ≤ 0.05) and non-significant (P > 0.05) pathway respectively. The numbers above the arrows indicate path coefficients. R2 values represent the proportion of the variance explained for each variable. ‘χ2’, ‘df’, ‘GFI’ and ‘RMSEA’ represent Chi-square, degree of freedom, goodness of fit index and root mean square error of approximation respectively.
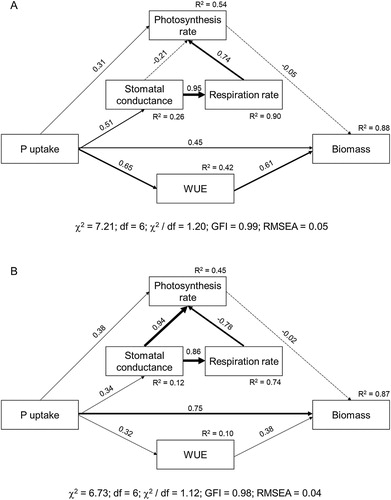
AMF colonization of roots, hyphal length density and soil macroaggregates
Non-inoculated plants did not show any AMF colonization in plant roots. High P inhibited root colonization of both maize (P < .0001, Figure S4(A, B)) and faba bean (P < .0001, Figure S4(C, D)). Compared with W treatment, drought stress (W–D and D) increased mycorrhizal colonization of maize in both monoculture and intercropping and faba bean in intercropping when P was low (Figure S4(A, C)). At low P level, compared to monoculture, intercropping did not have significant influence on root colonization in maize plants (Figure S4(A)), however, root colonization in faba bean intercropping decreased in the W treatment and increased in the D treatment (Figure S4(C)). At high P supply level, neither water treatments nor planting patterns had significant influence on maize root colonization (Figure S4(B)). However, root colonization of faba bean in the droughted treatment was significantly decreased compared to other water treatments (Figure S4(D)).
At low P supply level, hyphal length density (HLD) was greatest in maize monoculture, followed by intercropping and faba bean monoculture (Figure S5(A)). Compared with low P, high P decreased HLD of maize (P < .0001) and faba bean (P = .01) monoculture, but not intercropping (P = .8). At high P supply level, HLD of intercropping was comparable to maize monoculture, and both were significantly higher than faba bean monoculture (Figure S5(B)). Water treatments generally did not have significant effect on HLD (except decreased trend in the droughted treatment for maize at low P and for faba bean at high P level).
Both cropping systems (P = .5) and water treatments (P = .8) showed no significant influence on the proportion of soil macroaggregates (Figure S6). AMF inoculation significantly increased soil macroaggregates by 32.1% at low P level (P < .0001, Figure S6(A)), by contrast, at high P supply level, AMF did not show significant effect (P = .8, Figure S6B).
Discussion
Effects of AMF, P and water on interspecific interactions and overyielding
We found strong evidence for asymmetric facilitation of maize favored by AMF that contributed to overyielding at low P levels ((B)), which verified our hypothesis. However, at high P level, overyielding was not dependent on AMF inoculation ((C)). Maize P uptake was highly improved by AMF inoculation at low P level ((A)), and these results are consistent with AMF mediating overyielding by affecting P uptake of maize (Qiao et al. Citation2016). Resource partitioning is a commonly expected mechanism for overyielding (Tilman Citation1999; Loreau and Hector Citation2001) that can be mediated by interactions with soil symbionts like AMF (van der Heijden et al. Citation2003; Bever et al. Citation2010). Maize growth was positively influenced by AMF (when P is limited) and P addition (high P), while faba bean did not respond to both AMF and P, these results imply that maize and faba bean might have different P acquisition strategies. Faba bean have strong rhizosphere acidification ability (proton release and organic acids exudation) in the mobilization of insoluble inorganic and organic P mobilizing (Li et al. Citation2007), and/or a large P reserve in the seeds that may provide sufficient P for faba bean seedling growth. While maize is a mycorrhizal-dependent plant species, which relies on AMF to acquire soil available P. These results suggest that association with mycorrhiza might relax crop competition and prime the resource partitioning between maize and faba bean at low P soil, allowing for greater biomass production and overyielding in the presence of AMF (Table S1, (A)). These results are consistent with previous reports that AMF facilitate interspecific interactions and promote overyielding in plant diversity ecosystems, including agriculture (Walder et al. Citation2012; Meng et al. Citation2015), grassland (Nyfeler et al. Citation2009; Wagg et al. Citation2011) and forest (de Araujo Pereira et al. Citation2018). The increased facilitation or reduced competition might be attributed to niche partitioning mediated by common mycorrhizal networks of mycorrhizal fungi when associating with different plant species. For example, forest tree species that associate with AMF are expected to have increased access to inorganic P, whereas species associating with ectomycorrhizal fungi are expected to have increased access to N (Aerts Citation2002) or organic P (Liu et al. Citation2018). Besides, AMF could benefit non-legumes by assisting the transfer of nitrogen from legumes to non-legumes through hyphae, as showed in maize/soybean intercropping (Meng et al. Citation2015) and in Eucalyptus grandis and Acacia mangium mixture (de Araujo Pereira et al. Citation2018). In addition, asymmetric benefit delivery between plants and AM fungal species may favor those less competitive plants and contribute to the plants coexistence and overyielding (Bever Citation2002; Walder et al. Citation2012). For instance, in sorghum and flax intercropping, sorghum invested more carbon to AMF while flax gained up more nutrients provided by AMF (Walder et al. Citation2012). These results imply the importance of mycorrhizal fungi in mediating plant interactions and productivity in different terrestrial ecosystems, and relevant measures to maintain mycorrhzial fungi diversity are necessary to exploit their potential benefits.
According to ‘stress-gradient hypothesis’, in relatively harsh and high-stress abiotic environments, facilitative effects between plants are enhanced (Bertness and Callaway Citation1994). Contrary to this hypothesis, we did not find that water treatments strongly affected planting patterns (Table S1), indicating the variation between intercropping and monoculture (overyielding effect) was not caused by water treatments. Facilitation and overyielding are less likely to occur when there is overlap in resource use (Maestre et al. Citation2009). In the present study, both maize and faba bean biomass decreased simultaneously in droughted conditions (Table S2, (B, C)), hence the overlapping utilization for water between the two crops might explain why water treatment did not have significant effects on overyielding. Similar competition for limited water resources has been reported in maize/cowpea (Adiku et al. Citation2001) and pearl millet/cowpea intercropping (Zegada-Lizarazu et al. Citation2006). However, water supply was important for plant growth, and the effect was larger at low P than high P level, as shown by the higher path coefficient of WUE on crop biomass (). As our experiment was conducted in pots, both spatial and temporal niche differences in water usage were largely overlooked. More field work is needed on niche complementarity between different horizontal and vertical rooting species.
Effects of AMF and P on water utilization
Others have shown a positive regulation effect of AMF on plant–water relationships (Augé Citation2001; Ruiz-Lozano Citation2003; Augé et al. Citation2015). We found that WUE in intercropping was significantly promoted by AMF, in particular at low P level, and this was attributed to the AMF effect on improving maize WUE but not faba bean WUE ((A)). The indirect effects of AMF on the improvement of N and P nutrition should be considered (Waraich et al. Citation2011). The strong P dependence of maize on AMF is consistent with improved P nutrition driving higher WUE, as also shown by the higher path coefficient of P uptake on WUE at low P than high P level (). Another evidence in the present study is that even without AMF inoculation, P addition (high P) improved maize WUE relative to faba bean (Table S3, ). The positive effects of P on WUE can be attributed to improving root growth, maintaining high cell-membrane stability and leaf water potential, and increasing stomatal conductance, etc. (Brück et al. Citation2000; Sawwan et al. Citation2000; Waraich et al. Citation2011). In the present study, we observed more root biomass of maize associated with AMF at low P (Figure S1C), thus plants could extend out more roots to absorb water. Also, AMF improved plant stomatal conductance, especially for maize under water-limited conditions ((A)). This is consistent with a meta-analysis study showing that AMF contributed more to stomatal conductance of host plants under drought than under amply watered conditions (Augé et al. Citation2015). The significantly increased photosynthesis rate (A) of maize in the presence of AMF indicated that AMF inoculation lead to more CO2 assimilation and photosynthates, which would result in higher WUE. Other studies also reported increases of plant WUE induced by AMF inoculation (Kaya et al. Citation2003; Ruiz-Lozano and Aroca Citation2010).
We found that AMF also slightly improved maize WUE under W–D and D treatments in high P soil ((B)), indicating that AMF may improve maize WUE in semi-arid or arid conditions regardless of soil P. In addition to improved photosynthesis rate ((B)), other underlying mechanisms may be related to hormonal (abscisic acid) effects, improved soil-hyphae contact (Augé Citation2001; Smith et al. Citation2010), and regulations of aquaporin gene expression (Li et al. Citation2013; Bárzana et al. Citation2014; Recchia et al. Citation2018). AMF showed no significant effect on average faba bean WUE at both P levels (). Under water-limited conditions, plants may preferentially allocate more photosynthates for root growth to acquire water (Smucker and Aiken Citation1992; Fort et al. Citation2015). Although faba bean was not responsive to AMF, it may rely more on root to acquire water from soil, as we found that faba bean had greater root: shoot ratio than maize (Figure S2).
In the present study, we observed an increase in the proportion of soil macroaggregates as a result of AMF inoculation at low P levels, but not at high P levels (Figure S6). AMF have been found to improve soil aggregation through external hyphae networking, and the exudation of a sticky protein called glomalin which can enmesh soil particles to form macroaggregate structures (Zhu and Miller Citation2003; Wilson et al. Citation2009; Rillig et al. Citation2010). The aggregation of soil contributed by AMF may be especially important under drought conditions, as highly aggregated soils can retain more water than those that are not well aggregated (Connolly Citation1998; Sun and Lu Citation2014). Furthermore, improved soil aggregation might exert a positive feedback effect on subsequent water usage due to the development of mycorrhizal fungi. For example, previous work found that more aggregated soil resulted in more fungal growth (Rillig and Steinberg Citation2002), which could further improve plant water and nutrient uptake.
Conclusion
This study showed that resource partitioning driven by AMF was a crucial mechanism that results in overyielding of maize/faba bean intercropping when P is limited, with maize is highly mycorrhizal-dependent while faba bean is more of self-dependent. The impact of AMF on overyielding was diminished under high P condition because overyielding was observed in all the treatments, which was attributed to competitive advantage of maize against faba bean. Though overyielding of maize/faba bean intercropping was not affected by water regimes, AMF inoculation showed a critical role in facilitating P uptake, drought resistence with enhanced WUE, photosynthesis rate and stomatal conductance, in particular for maize at low P level. Beside, soil macroaggregate formation was also promoted by AMF at low P level, suggesting a potential positive feedback effect on subsequent water retention capacity. Overall, our study highlights the importance of AMF in facilitating interspecific interactions that can improve the productivity and sustainability of agriculture in intercropping systems.
Supplemental Material
Download MS Word (117.4 KB)Acknowledgements
We thank Professor Youshan Wang (Institute of Plant Nutrition and Resources, Beijing Academy of Agriculture and Forestry Research, Beijing, China) for providing us with the AMF inoculum.
Disclosure statement
No potential conflict of interest was reported by the authors.
Additional information
Funding
References
- Adiku SG, Ozier-Lafontaine H, Bajazet T. 2001. Patterns of root growth and water uptake of a maize-cowpea mixture grown under greenhouse conditions. Plant Soil. 235:85–94. doi: 10.1023/A:1011847214706
- Aerts R. 2002. The role of various types of mycorrhizal fungi in nutrient cycling and plant competition. In: van der Heijden MGA, Sanders IR, editors. Mycorrhizal Ecology. Berlin: Springer-Verlag; p. 117–133.
- Arbuckle JL. 2006. Amos (version 7.0) [computer program]. Chicago: SPSS.
- Augé RM. 2001. Water relations, drought and vesicular-arbuscular mycorrhizal symbiosis. Mycorrhiza. 11:3–42. doi: 10.1007/s005720100097
- Augé RM, Toler HD, Saxton AM. 2015. Arbuscular mycorrhizal symbiosis alters stomatal conductance of host plants more under drought than under amply watered conditions: a meta-analysis. Mycorrhiza. 25:13–24. doi: 10.1007/s00572-014-0585-4
- Bárzana G, Aroca R, Bienert GP, Chaumont F, Ruiz-Lozano JM. 2014. New insights into the regulation of aquaporins by the arbuscular mycorrhizal symbiosis in maize plants under drought stress and possible implications for plant performance. Mol Plant Microbe In. 27:349–363. doi: 10.1094/MPMI-09-13-0268-R
- Bedoussac L, Journet EP, Hauggaard-Nielsen H, Naudin C, Corre-Hellou G, Jensen E, Prieur L, Justes E. 2015. Ecological principles underlying the increase of productivity achieved by cereal-grain legume intercrops in organic farming. A review. Agron Sustain Dev. 35:911–935. doi: 10.1007/s13593-014-0277-7
- Bertness MD, Callaway R. 1994. Positive interactions in communities. Trends Ecol Evol. 9:191–193. doi: 10.1016/0169-5347(94)90088-4
- Bever JD. 2002. Negative feedback within a mutualism: host-specific growth of mycorrhizal fungi reduces plant benefit. Proc Roy Soc B Biol Sci. 269:2595–2601. doi: 10.1098/rspb.2002.2162
- Bever JD, Dickie IA, Facelli E, Facelli JM, Klironomos J, Moora M, Rillig MC, Stock WD, Tibbett M, Zobel M. 2010. Rooting theories of plant community ecology in microbial interactions. Trends Ecol Evol. 25:468–478. doi: 10.1016/j.tree.2010.05.004
- Brooker RW, Bennett AE, Cong WF, Daniell TJ, George TS, Hallett PD, Hawes C, Iannetta PP, Jones HG, Karley AJ. 2015. Improving intercropping: a synthesis of research in agronomy, plant physiology and ecology. New Phytol. 206:107–117. doi: 10.1111/nph.13132
- Brooker RW, Karley AJ, Newton AC, Pakeman RJ, Schöb C. 2016. Facilitation and sustainable agriculture: a mechanistic approach to reconciling crop production and conservation. Funct Ecol. 30:98–107. doi: 10.1111/1365-2435.12496
- Brooker RW, Maestre FT, Callaway RM, Lortie CL, Cavieres LA, Kunstler G, Liancourt P, Tielbörger K, Travis JM, Anthelme F. 2008. Importance: an overlooked concept in plant interaction research. J Ecol. 96:703–708. doi: 10.1111/j.1365-2745.2008.01373.x
- Brück H, Payne W, Sattelmacher B. 2000. Effects of phosphorus and water supply on yield, transpirational water-use efficiency, and carbon isotope discrimination of pearl millet. Crop Sci. 40:120–125. doi: 10.2135/cropsci2000.401120x
- Chen G, Kong X, Gan Y, Zhang R, Feng F, Yu A, Zhao C, Wan S, Chai Q. 2018. Enhancing the systems productivity and water use efficiency through coordinated soil water sharing and compensation in strip-intercropping. Sci Rep. 8:10494. doi: 10.1038/s41598-018-28612-6
- Chu Q, Wang X, Yang Y, Chen F, Zhang F, Feng G. 2013. Mycorrhizal responsiveness of maize (Zea mays L.) genotypes as related to releasing date and available P content in soil. Mycorrhiza. 23:497–505. doi: 10.1007/s00572-013-0492-0
- Collins CD, Foster BL. 2009. Community-level consequences of mycorrhizae depend on phosphorus availability. Ecology. 90:2567–2576. doi: 10.1890/08-1560.1
- Connolly R. 1998. Modelling effects of soil structure on the water balance of soil-crop systems: a review. Soil Tillage Res. 48:1–19. doi: 10.1016/S0167-1987(98)00128-7
- de Araujo Pereira AP, Santana MC, Bonfim JA, de Lourdes Mescolotti D, Cardoso ELBN. 2018. Digging deeper to study the distribution of mycorrhizal arbuscular fungi along the soil profile in pure and mixed Eucalyptus grandis and Acacia mangium plantations. Appl Soil Ecol. 128:1–11. doi: 10.1016/j.apsoil.2018.03.015
- Duchene O, Vian JF, Celette F. 2017. Intercropping with legume for agroecological cropping systems: complementarity and facilitation processes and the importance of soil microorganisms. A review. Agr Ecosyst Environ. 240:148–161. doi: 10.1016/j.agee.2017.02.019
- Egerton-Warburton LM, Querejeta JI, Allen MF. 2007. Common mycorrhizal networks provide a potential pathway for the transfer of hydraulically lifted water between plants. J Exp Bot. 58:1473–1483. doi: 10.1093/jxb/erm009
- Ehrmann J, Ritz K. 2014. Plant: soil interactions in temperate multi-cropping production systems. Plant Soil. 376:1–29. doi: 10.1007/s11104-013-1921-8
- Eisenhauer N. 2012. Aboveground-belowground interactions as a source of complementarity effects in biodiversity experiments. Plant Soil. 351:1–22. doi: 10.1007/s11104-011-1027-0
- Fort F, Cruz P, Catrice O, Delbrut A, Luzarreta M, Stroia C, Jouany C. 2015. Root functional trait syndromes and plasticity drive the ability of grassland Fabaceae to tolerate water and phosphorus shortage. Environ Exp Bot. 110:62–72. doi: 10.1016/j.envexpbot.2014.09.007
- Gao Y, Duan A, Sun J, Li F, Liu Z, Liu H, Liu Z. 2009. Crop coefficient and water-use efficiency of winter wheat/spring maize strip intercropping. Field Crop Res. 111:65–73. doi: 10.1016/j.fcr.2008.10.007
- Hartnett DC, Wilson GW. 1999. Mycorrhizae influence plant community structure and diversity in tallgrass prairie. Ecology. 80:1187–1195. doi: 10.1890/0012-9658(1999)080[1187:MIPCSA]2.0.CO;2
- Hauggaard-Nielsen H, Jensen ES. 2005. Facilitative root interactions in intercrops. Plant Soil. 274:237–250. doi: 10.1007/s11104-004-1305-1
- He Q, Bertness MD, Altieri AH. 2013. Global shifts towards positive species interactions with increasing environmental stress. Ecol Lett. 16:695–706. doi: 10.1111/ele.12080
- Hodge A, Fitter AH. 2013. Microbial mediation of plant competition and community structure. Funct Ecol. 27:865–875. doi: 10.1111/1365-2435.12002
- Hoeksema JD, Chaudhary VB, Gehring CA, Johnson NC, Karst J, Koide RT, Pringle A, Zabinski C, Bever JD, Moore JC. 2010. A meta-analysis of context-dependency in plant response to inoculation with mycorrhizal fungi. Ecol Lett. 13:394–407. doi: 10.1111/j.1461-0248.2009.01430.x
- Jakobsen I, Abbott L, Robson A. 1992. External hyphae of vesicular-arbuscular mycorrhizal fungi associated with Trifolium subterraneum L. 1. Spread of hyphae and phosphorus inflow into roots. New Phytol. 120:371–380. doi: 10.1111/j.1469-8137.1992.tb01077.x
- Kaya C, Higgs D, Kirnak H, Tas I. 2003. Mycorrhizal colonisation improves fruit yield and water use efficiency in watermelon (Citrullus lanatus Thunb.) grown under well-watered and water-stressed conditions. Plant Soil. 253:287–292. doi: 10.1023/A:1024843419670
- Li T, Hu YJ, Hao ZP, Li H, Wang YS, Chen BD. 2013. First cloning and characterization of two functional aquaporin genes from an arbuscular mycorrhizal fungus Glomus intraradices. New Phytol. 197:617–630. doi: 10.1111/nph.12011
- Li L, Li SM, Sun JH, Zhou LL, Bao XG, Zhang HG, Zhang FS. 2007. Diversity enhances agricultural productivity via rhizosphere phosphorus facilitation on phosphorus-deficient soils. Proc Natl Acad Sci USA. 104:11192–11196. doi: 10.1073/pnas.0704591104
- Li B, Li YY, Wu HM, Zhang FF, Li CJ, Li XX, Lambers H, Li L. 2016. Root exudates drive interspecific facilitation by enhancing nodulation and N2 fixation. Proc Natl Acad Sci USA. 113:6496–6501. doi: 10.1073/pnas.1523580113
- Li L, Sun JH, Zhang FS, Guo TW, Bao XG, Smith FA, Smith SE. 2006. Root distribution and interactions between intercropped species. Oecologia. 147:280–290. doi: 10.1007/s00442-005-0256-4
- Li L, Tilman D, Lambers H, Zhang FS. 2014. Plant diversity and overyielding: insights from belowground facilitation of intercropping in agriculture. New Phytol. 203:63–69. doi: 10.1111/nph.12778
- Liu X, Burslem DF, Taylor JD, Taylor AF, Khoo E, Majalap-Lee N, Helgason T, Johnson D. 2018. Partitioning of soil phosphorus among arbuscular and ectomycorrhizal trees in tropical and subtropical forests. Ecol Lett. 21:713–723. doi: 10.1111/ele.12939
- Loreau M, Hector A. 2001. Partitioning selection and complementarity in biodiversity experiments. Nature. 412:72–76. doi: 10.1038/35083573
- Maestre FT, Callaway RM, Valladares F, Lortie CJ. 2009. Refining the stress-gradient hypothesis for competition and facilitation in plant communities. J Ecol. 97:199–205. doi: 10.1111/j.1365-2745.2008.01476.x
- Mao L, Zhang L, Li W, van der Werf W, Sun J, Spiertz H, Li L. 2012. Yield advantage and water saving in maize/pea intercrop. Field Crop Res. 138:11–20. doi: 10.1016/j.fcr.2012.09.019
- Mariotte P, Mehrabi Z, Bezemer TM, De Deyn GB, Kulmatiski A, Drigo B, Veen GF, van der Heijden MGA, Kardol P. 2018. Plant-soil feedback: bridging natural and agricultural sciences. Trends Ecol Evol. 33:129–142. doi: 10.1016/j.tree.2017.11.005
- McGonigle T, Miller M, Evans D, Fairchild G, Swan J. 1990. A new method which gives an objective measure of colonization of roots by vesicular-arbuscular mycorrhizal fungi. New Phytol. 115:495–501. doi: 10.1111/j.1469-8137.1990.tb00476.x
- Meng LB, Zhang AY, Wang F, Han XG, Wang DJ, Li SM. 2015. Arbuscular mycorrhizal fungi and rhizobium facilitate nitrogen uptake and transfer in soybean/maize intercropping system. Front Plant Sci. 6:339.
- Merrild MP, Ambus P, Rosendahl S, Jakobsen I. 2013. Common arbuscular mycorrhizal networks amplify competition for phosphorus between seedlings and established plants. New Phytol. 200:229–240. doi: 10.1111/nph.12351
- Mpelasoka F, Hennessy K, Jones R, Bates B. 2008. Comparison of suitable drought indices for climate change impacts assessment over Australia towards resource management. Int J Climatology. 28:1283–1292. doi: 10.1002/joc.1649
- Muler AL, Oliveira RS, Lambers H, Veneklaas EJ. 2014. Does cluster-root activity benefit nutrient uptake and growth of co-existing species? Oecologia. 174:23–31. doi: 10.1007/s00442-013-2747-z
- Nyfeler D, Huguenin-Elie O, Suter M, Frossard E, Connolly J, Lüscher A. 2009. Strong mixture effects among four species in fertilized agricultural grassland led to persistent and consistent transgressive overyielding. J Appl Ecol. 46:683–691. doi: 10.1111/j.1365-2664.2009.01653.x
- Qiao X, Bei S, Li H, Christie P, Zhang F, Zhang J. 2016. Arbuscular mycorrhizal fungi contribute to overyielding by enhancing crop biomass while suppressing weed biomass in intercropping systems. Plant Soil. 406:173–185. doi: 10.1007/s11104-016-2863-8
- Recchia GH, Konzen ER, Cassieri F, Caldas DGG, Tsai SM. 2018. Arbuscular mycorrhizal symbiosis leads to differential regulation of drought-responsive genes in tissue-specific root cells of common bean. Front Microbiol. 9:1339. doi: 10.3389/fmicb.2018.01339
- Rillig MC, Mardatin NF, Leifheit EF, Antunes PM. 2010. Mycelium of arbuscular mycorrhizal fungi increases soil water repellency and is sufficient to maintain water-stable soil aggregates. Soil Biol Biochem. 42:1189–1191. doi: 10.1016/j.soilbio.2010.03.027
- Rillig MC, Mummey DL. 2006. Mycorrhizas and soil structure. New Phytol. 171:41–53. doi: 10.1111/j.1469-8137.2006.01750.x
- Rillig MC, Steinberg PD. 2002. Glomalin production by an arbuscular mycorrhizal fungus: a mechanism of habitat modification? Soil Biol Biochem. 34:1371–1374. doi: 10.1016/S0038-0717(02)00060-3
- Ruiz-Lozano JM. 2003. Arbuscular mycorrhizal symbiosis and alleviation of osmotic stress. New perspectives for molecular studies. Mycorrhiza. 13:309–317. doi: 10.1007/s00572-003-0237-6
- Ruiz-Lozano JM, Aroca R. 2010. Host response to osmotic stresses: stomatal behaviour and water use efficiency of arbuscular mycorrhizal plants. In: Koltai H, Kapulnik Y, editor. Arbuscular mycorrhizas: physiology and function. Berlin: Springer; p. 239–256.
- Sardans J, Peñuelas J. 2004. Increasing drought decreases phosphorus availability in an evergreen Mediterranean forest. Plant Soil. 267:367–377. doi: 10.1007/s11104-005-0172-8
- Sawwan J, Shibli RA, Swaidat I, Tahat M. 2000. Phosphorus regulates osmotic potential and growth of African violet under in vitro-induced water deficit. J Plant Nutr. 23:759–771. doi: 10.1080/01904160009382057
- Smith SE, Facelli E, Pope S, Smith FA. 2010. Plant performance in stressful environments: interpreting new and established knowledge of the roles of arbuscular mycorrhizas. Plant Soil. 326:3–20. doi: 10.1007/s11104-009-9981-5
- Smith SE, Read DJ. 2008. Mycorrhizal symbiosis. London: Academic Press.
- Smucker A, Aiken R. 1992. Dynamic root responses to water deficits. Soil Sci. 154:281–289. doi: 10.1097/00010694-199210000-00004
- Sun F, Lu S. 2014. Biochars improve aggregate stability, water retention, and pore-space properties of clayey soil. J Plant Nutr Soil Sci. 177:26–33. doi: 10.1002/jpln.201200639
- Szumigalski AR, Van Acker RC. 2008. Land equivalent ratios, light interception, and water use in annual intercrops in the presence or absence of in-crop herbicides. Agron J. 100:1145–1154. doi: 10.2134/agronj2006.0343
- Thomas RL, Sheard RW, Moyer JR. 1967. Comparison of conventional and automated procedures for nitrogen, phosphorus, and potassium analysis of plant material using a single digestion. Agron J. 59:240–243. doi: 10.2134/agronj1967.00021962005900030010x
- Tilman D. 1999. The ecological consequences of changes in biodiversity: a search for general principles. Ecology. 80:1455–1474.
- van der Heijden MG, Bardgett RD, Van Straalen NM. 2008. The unseen majority: soil microbes as drivers of plant diversity and productivity in terrestrial ecosystems. Ecol Lett. 11:296–310. doi: 10.1111/j.1461-0248.2007.01139.x
- van der Heijden MG, Klironomos JN, Ursic M, Moutoglis P, Streitwolf-Engel R, Boller T, Wiemken A, Sanders IR. 1998. Mycorrhizal fungal diversity determines plant biodiversity, ecosystem variability and productivity. Nature. 396:69–72. doi: 10.1038/23932
- van der Heijden MG, Martin FM, Selosse MA, Sanders IR. 2015. Mycorrhizal ecology and evolution: the past, the present, and the future. New Phytol. 205:1406–1423. doi: 10.1111/nph.13288
- van der Heijden MG, Wiemken A, Sanders IR. 2003. Different arbuscular mycorrhizal fungi alter coexistence and resource distribution between co-occurring plant. New Phytol. 157:569–578. doi: 10.1046/j.1469-8137.2003.00688.x
- Vandermeer JH. 1992. The ecology of intercropping. Cambridge: Cambridge University Press.
- Vogelsang KM, Reynolds HL, Bever JD. 2006. Mycorrhizal fungal identity and richness determine the diversity and productivity of a tallgrass prairie system. New Phytol. 172:554–562. doi: 10.1111/j.1469-8137.2006.01854.x
- Wagg C, Jansa J, Stadler M, Schmid B, van der Heijden MG. 2011. Mycorrhizal fungal identity and diversity relaxes plant-plant competition. Ecology. 92:1303–1313. doi: 10.1890/10-1915.1
- Walder F, Niemann H, Natarajan M, Lehmann MF, Boller T, Wiemken A. 2012. Mycorrhizal networks: common goods of plants shared under unequal terms of trade. Plant Physiol. 159:789–797. doi: 10.1104/pp.112.195727
- Waraich EA, Ahmad R, Ashraf MY, Saifullah AM. 2011. Improving agricultural water use efficiency by nutrient management in crop plants. Acta Agr Scand B-S. 61:291–304.
- Weremijewicz J, LdSLO S, Janos DP. 2016. Common mycorrhizal networks amplify competition by preferential mineral nutrient allocation to large host plants. New Phytol. 212:461–471. doi: 10.1111/nph.14041
- Wilson GW, Rice CW, Rillig MC, Springer A, Hartnett DC. 2009. Soil aggregation and carbon sequestration are tightly correlated with the abundance of arbuscular mycorrhizal fungi: results from long-term field experiments. Ecol Lett. 12:452–461. doi: 10.1111/j.1461-0248.2009.01303.x
- Xu B, Li F, Shan L. 2008. Switchgrass and milkvetch intercropping under 2: 1 row-replacement in semiarid region, northwest China: aboveground biomass and water use efficiency. Eur J Agron. 28:485–492. doi: 10.1016/j.eja.2007.11.011
- Yang C, Huang G, Chai Q, Luo Z. 2011. Water use and yield of wheat/maize intercropping under alternate irrigation in the oasis field of northwest China. Field Crop Res. 124:426–432. doi: 10.1016/j.fcr.2011.07.013
- Zegada-Lizarazu W, Izumi Y, Iijima M. 2006. Water competition of intercropped pearl millet with cowpea under drought and soil compaction stresses. Plant Prod Sci. 9:123–132. doi: 10.1626/pps.9.123
- Zhu YG, Miller RM. 2003. Carbon cycling by arbuscular mycorrhizal fungi in soil-plant systems. Trends Plant Sci. 8:407–409. doi: 10.1016/S1360-1385(03)00184-5