ABSTRACT
Tomato is an important crop and has immense health benefits and medicinal value. Here, we described how salinity stress affects tomato plant growth and developmental processes and productivity. It causes ionic toxicity, oxidative damage, osmotic stress, and hormonal imbalance. In this review, we emphasized the crucial role of ethylene (ET) towards in vitro development of tomato crop by mediating stress response, particularly high salt. There are evidences that salinity stress modulates the expression of ACS and beta-CAS, which leads to ET and cyanide accumulation. We draw attention to how ET negatively or positively mediates salinity stress response by maintaining endogenous biomolecules, Na+/K+ ion balance and redox homeostasis. How ET inhibitors and polyamines as protectants reverse the negative effects of ET/salinity stress-induced cellular damage by cross talk with important physiological processes-photosynthesis and respiratory and salt overly sensitive (SOS). The literature appraised herein will contribute to a better understanding of the development of salinity stress tolerant tomato.
Introduction
The global population is expected to cross 9.6 billion by 2050, leading to exceeded demands on already extracted natural resources. Global climate change in the form of environmental stress is a major constrain to crop productivity. There is an urgent need to improve plant survival in changing environment to sustain plant productivity (Abdallah et al. Citation2014; UN-DESA Citation2017; Dahal et al. Citation2019). The diverse abiotic stresses are known to affect tomato productivity at a large scale. Salinity stress is important not because of its destructive and deleterious nature to reduce crop productivity, but also because of its mechanism of action which is not completely understood. It affects several dicotyledonous crops in which tomato is a nominated model to examine salinity stress response (Khan Citation2016; Joseph et al. Citation2018). The tomato fruit is the fruit of one of the significant horticultural crops because of antioxidative and anti-cancer properties, and have considered an excellent source of antioxidants and taurines, and dietary fiber, minerals, vitamins, and lycopene content (Grabowska et al. Citation2019). Technological management and sustainable methods are the two most important approaches to mitigate the negative effects of high soil/water salinity in agriculture. The technological approach, based on renovation, drainage, and irrigation practices with high-quality water, is costly and devoid of a complete solution. In the case of sustainable agriculture production in the presence of high salts which relies on the development of salinity stress-tolerant crops by crop substitution, adopting wild species, unmanned aerial vehicle-based phenotyping and genetically modifying crops (Epstein et al. Citation1980; Johansen et al. Citation2019). Several studies have been put forth to explain salinity tolerance in tomato crops in which plant hormones are the key players (Sahoo et al. Citation2014; Shah et al. Citation2018). In the present review, a potential role of plant hormone ethylene homeostasis and its cross talk in somaclone generated tomato plants under high salinity stress to produce salinity stress tolerant tomato will be discussed ((A)).
Figure 1. Global climate change, tomato production in response to salinity stress and management practices. Population rise causes increasing demand for tomato production which is limited due to increased salinity stress from climate change, worldwide. The management practices and ethylene mediated tomato salinity stress response to improve productivity under high salt condition (A). Tentative timeline diagram to explain salinity stress response in tomato mediated via ethylene synthesis, signaling, and cross talk (B).
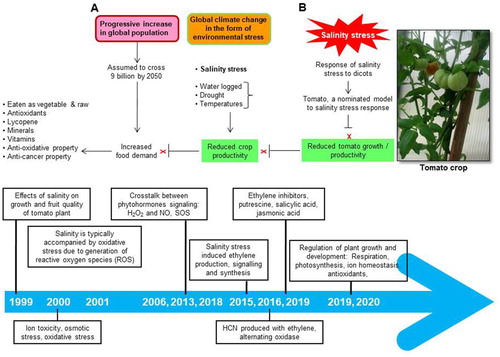
Hyper osmotic stress and hyperion toxicity are the major problems that influence salt-stressed plants (Gorham et al. Citation2010). Under high salinity stress conditions, roots are not able to uptake water directly from the soil, causing toxic concentrations of sodium and chloride, plant nutritional disorders, and oxidative damage (Rouphael et al. Citation2018). The accumulated sodium obstructs with potassium and calcium uptake and thereby negatively affecting stomatal movement and photosynthesis. Therefore, the salt stress condition of a crop relies mainly on the potassium-to-sodium ratio than on the total amount of sodium present in the cytosol (Annunziata et al. Citation2017). The increased sodium level elevated cytosolic free Ca2+ activating the salt overly sensitive (SOS) pathway signal transduction cascade (Golldack et al. Citation2014). Ca2+ is perceived by SOS3 (Salt OverlySensitive3) function as a calcium-sensor (Ji et al. Citation2013). After binding Ca2+, this SOS3 cooperates with active SOS2 and thereby the activation of the plasma membrane to SOS1, which is a Na+/H+ antiporter (Ji et al. Citation2013; Rouphael et al. Citation2018). This will leads to oxidative damage of lipid, proteins, DNA, and RNA, and thereby physiological and metabolic processes (Chrysargyris et al. Citation2018). Conversely, the functioning of SOS pathways in response to ethylene and ABA in tomato plants generated through somaclonal variation has not yet been completely understood. Somaclonal variations are modern tools of selecting, screening, and crossing plants to develop plantlets with improved traits (Verma et al. Citation2013). However, in vitro production, somaclone tomato plantlets exhibiting improved tolerance to salinity stress has not been studied so far. Several reports suggested that plant hormones play an important role in improving abiotic stress tolerance including salinity stress in plants (De-la-Torre-González et al. Citation2017). Ethylene regulates various plant growth and developmental processes and stress response. ACC (1-aminocyclo propnae 1-carbooxylic acid) synthase (ACS) is a key enzype of ethylene biosynthesis pathway as it catalyzes the rate-limiting step in the pathway, and also plays a crucial role in ethylene signaling and salinity stress response (Win et al. Citation2018). Therefore, increased endogenous ethylene is associated with enhanced ACS expression and increased cellular ACS enzyme activity (Ansari et al. Citation2019). Sato and Theologis (Citation1989) reported cloning the first ACS gene from Cucurbita pepo. ACS gene expressions have also been studied in many other plant species (Manzano et al. Citation2016). Nevertheless, ACS expression profile in somaclone tomato plantlets under salinity stress conditions has not been evaluated. The reactive oxygen species (ROS) accumulated and their detoxification in response to salinity stress represent differential tolerance of plants to abiotic stresses. Antioxidants such as ascorbate peroxidase, glutathione reductase, glutathione peroxidase, and phospholipid hydroperoxide glutathione peroxidase (APX, GR, GPX, and Ph-GPX) profiles defend salinity stress-mediated cellular damage, and many osmolytes played a crucial role to improves salinity stress tolerance. The somaclone tomato with modulated ACS resulted ethylene level cooperates with antioxidants and photosynthetic machinery for better antioxidant capacity and to a lesser ROS accumulation and improved growth and productivity under high salt (Martinez et al. Citation2018). β-cyanoalanine synthase (β-CAS) detoxifies the accumulated cyanide under abitic stress particularly salinity stress (Ansari et al. Citation2015). The considerable activity of β-CAS enzyme was reported in various plant species at immense levels (Miller and Conn Citation1980). Although β-CAS genes expression was reported in many plant species, there are no reports of β-CAS transcript accumulation and cyanide detoxification in somaclone tomato plantlets under high salt ((A)).
In this review, we will briefly appraise the current progress in our understanding of ET metabolism, and interactions with other important cellular pathways such as polyamines, antioxidants, respiration, photosynthesis, ion homeostasis to regulate salinity stress response ((B)). The present review is aimed to provide insight into the tomato salinity stress tolerance mechanism underlying ET as a key player to cross talk with cell signaling networks of salinity response in in vitro developed tomato. The information gathered herein will contribute to a better understanding of salinity stress tolerance mechanism.
Salinity stress: global perspectives
Among environmental stresses, salinity is a progressively more imperative ecological constraint to crop production globally (Ghassemi et al. Citation1995). Salinity can develop either in the soil itself or by the use of irrigation water (Acosta-Motos et al. Citation2015) in which the electrical conductivity (EC) exceeds 4 dSm−1 at 25oC (Foolad Citation2004).
Salinity is the major cause of agriculture land degradation, which makes agriculture land unsuitable for the cultivation of crops. In the current scenario of population increasing rapidly to 9.6 billion by 2050, worldwide food production needs to be increased up to 57% by 2050 (Hossain Citation2019). Salinity alone affects above 20% of irrigated land that may account for around one-third of global food production (Machado and Serralheiro Citation2017). Salts are naturally occurring in soils and water body. The ions such as Na+, K+, Ca2+, Mg2+, and Cl− contribute to salinization. The major causes of soil salinity are natural, such as weathering of parent material, deposition of sea salt carried in wind and rain, and inundation of coastal land by tidal water. Human-induced causes of soil salinity include the rise of water table due to excessive irrigation using underground water, irrigation with salt-containing water, and poor drainage (Rengasamy Citation2006; Toth et al. Citation2008; Kaushal et al. Citation2017).
In the current scenario, world's salinity affected area of land is about 1125 million hectares, of which approximately 76 million hectares are affected by human-induced salinization and sodification (Hossain Citation2019) Salinity problem in Australia is maximum due to high salt concentration found in the rhizosphere. It is followed by north and central Asia and south America that have high salt accumulation in plant root zone soil (Gurbachan Citation2018). At present, the mapping of salinity affected land represents practically all regions of world is salinity affected land. However, the degree and severity might be varied region to region ((A)). In (A), salinity degrades agriculture land area in Canada, USA, Central America, South America, North Africa, West Africa, East Africa, South Africa, West Europe, East Europe, Former USSR, Middle East, South Asia, East Asia, Southeast Asia, Oceania, and Japan (Wicke et al. Citation2011). There are world’s regions that are severely affected by salinity include the Middle East (189 Mha), Oceania-Australia (169 Mha), North Africa (144 Mha), and some part of USSR (126 Mha) ((B)) (Hossain Citation2019). In India, about 6.73 Mha land area is affected by high salinity. The major salinity affected Indian states are Andhra Pradesh, Andaman & Nicobar Island, Bihar, Gujarat, Haryana, Karnataka, Kerala, Madhya Pradesh, Maharashtra, Orissa, Punjab, Rajasthan, Tamil Nadu, and Uttar Pradesh ((C)). However, Gujarat is most affected by salinity which is followed by Uttar Pradesh state ((D)) (Mandal et al. Citation2011; Gurbachan Citation2018).
Figure 2. Distribution of salinity. Current data of mapping showing salinity affected land area (Mha) in (A) and in India (B) The largest salinity affected world’s regions: (I) middle east, (II) Australia, (III) North Africa, and (IV) USSR (C), and Indian states: (a) Gujarat and (b) Uttar Pradesh. Source: (Hossain Citation2019).
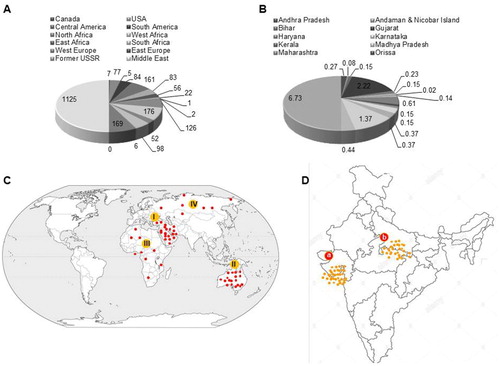
Salinity stress and response of tomato cultivars
Being tropical tomato plant is well adapted to nearly all climate regions, and it is seventh in global production i.e. 164 × 108 million tons per 4.8 million hectares area (Gerszberg and Hnatuszko-Konka Citation2017). Tomato has been extensively investigated plant due to higher content of molecules with antioxidants properties such as carotenoids, phenols, and flavonoids (Mitchell et al. Citation2007; Periago et al. Citation2009). Additionally, tomato contains an essential bioactive compound i.e. lycopene, which has antioxidant property, and it also uses to protect the human from cancer (Gerszberg and Hnatuszko-Konka Citation2017). Interestingly, lycopene has a potential role in maintaining cardiovascular function and health (Costa-Rodrigues et al. Citation2018). Despite such an important crop, quality, and productivity of tomato are badly hit by global environmental change, and thus a tomato fruit does not meet the demands of increasing population (Bai et al. Citation2018). Salinity stress, a major problem globally, affects tomato crops at different developmental stages such as plant growth and development, seed germination, vegetative growth, and reproductive development. High salinity causes uptake and distribution of Na+ and Cl− ions, its cytotoxicity due to specific sensing components, leading to nutritional deficit, water scarcity, oxidative stress, and ROS accumulation (Isayenkov and Maathuis Citation2019). However, tomato sensitivity to salinity stress varied greatly depending upon varietal type, phenotypic and genotypic attributes, and environmental conditions (Rahman et al. Citation2018). In the recent trend of global climate change, the most commercial varieties of tomato with respect to salinity stress need to be discussed.
Salinity stress significantly affects the relative plant growth, fruit weight, and size of Simge F1 variety; however, it does not significantly affect Edkawy variety of tomato (Johnson et al. Citation2003). The Rams and C10 variety of tomato shows improved tolerance to salinity stressed by improving physiological and nutritional parameters such as seed germination, seedling emergence, water imbalance plant height, fresh biomass, dry weight, and leaf number, and endogenous K, N, Na, and Ca level (Al-Daej Citation2018). Super strain B, Mahaly, and Super Marmand 1 are highly susceptible to Salinity stress (Al-Daej Citation2018). Tomato variety Shed showed affected stomatal traits such as stomatal density, stomatal width, leaf stomatal area, and leaf stomatal area index under salinity stress condition, whereas Alam variety remains unaffected (Guo et al. Citation2018). The Optima tomato variety of Southeastern Spain showed improved fruit quality by augmenting the total soluble solids and titratable acidity under salinity treatment (Rodríguez-Ortega et al. Citation2019). Tomato transgenic with overexpressed vacuolar Na+/H+ antiport showed improved vegetative and flower growth, and fruit yield in the presence of high salinity (Zhang and Blumwald Citation2001). The transgenic tomatoes with Osmotin transgene confer salinity stress tolerance by advancing photosynthetic system and antioxidant machinery, cell signaling, transcriptional regulation, proteins and membrane defense, compatible solute-betaines, sugars, polyols, and amino acids contents, and ROS scavenging (Krishna et al. Citation2019). Transgenic tomato overexpressing ethylene response factor (SlERF5) improves salinity stress tolerance by increasing relative water content (Pan et al. Citation2012)Tomato Boludo variety showed positive effect on the ameliorateion of oxidative damage by high salinity by increasing K+ and Ca2+ dose in the nutrient solution (García-Martí et al. Citation2019). Tomato Nathen and Jinpeng varieties under water and salinity treatment and its response to improved physiological parameters such as stomatal conductance, transpiration, and photosynthesis advance salinity tolerance (Yang et al. Citation2019). Proteomic analysis of Roma, Marmande, Cervil, and Levovil revealed that proteome variations are associated with salinity stress. This will lead to characterization that Cervil and Super Marmande as the most sensitive whereas Levovil and Roma as the most tolerant varieties on the basis of root growth, leaf accumulation of Na+, and leaf K/Na ratio (Manaa et al. Citation2011). The BARI T1- BARI T5 tomato variety of Bangladesh is susceptible and exhibited reduced leaf number, length and width, and flowering, under high salinity (Rahman et al. Citation2018) ().
Table 1. Response of tomato varieties/cultivars/transgenics to salinity stress.
In vitro production of salinity tolerant tomato
Somaclonal variations present an opportunity to discover the naturally occurring genetic variability in plants to develop new product development (Evans and Sharp Citation1986). These are genetic and/or epigenetic changes induced in cultured plant cell and entire tissue. It is an alternating tool to conventional breeding to instigate advantageous genetic variability in the existing gene pool (Weckx et al. Citation2019). In the global change in environment, the developments of abiotic and biotic stress-tolerant crops are of great concern. Somaclonal variation has been successful in inducing stress tolerance in many crop species (Anil et al. Citation2018). Tomato, an important crop due to its high antioxidants and lycopene content, is affected by salinity stress. Somaclonal variation might be a potential tool to screen out salt-tolerant trait in the plant to develop salt tolerance crops (Krishna at al. Citation2016; Gerszberg and Hnatuszko-Konka Citation2017). Somaclonal variations are obtained by treating plant hormone, sodium chloride (NaCl), and abscisic acid ABA to develop advantageous polygenetic traits to confer plant tolerance to abiotic stress (Halim et al. Citation2017). In tomato plant, the selection of somaclonal variants in vitro in the form of protoplast, cell colony, or callus and even complete plants becomes possible. Somaclone variant tomato produced by using fungal filtrate and fusaric acid for in vitro selection showed resistance to Fusarium oxysporum f. sp. infestan lycopersici ras 2 (Lestari Citation2006). The cellular physiological adaptation of cultured tomato plant cell to salinity stress to provide tolerance, and its potential significance in crop improvement has become paramount in the horticulture sector (Chandler et al. Citation1986; Manchanda et al. Citation2018). Somaclonal variant crop selection has been successful in improving abiotic and biotic stress tolerance in different crops (Anil et al. Citation2018).
Mitigation strategies for salinity stress in tomato
Salinity is a serious abiotic stress affecting 20% of irrigated soil, stimulates physiological changes that alter metabolism, and results in a decreased crop yield including tomato (Kalaji et al. Citation2016; Albaladejo et al. Citation2017; Machado and Serralheiro Citation2017). The major problems arises in plants due to salinity are osmotic stress and ion toxicity (Haq et al. Citation2010) as salinity significantly inhibits the water uptake from the soil that ultimately disturbs the osmotic balance in the affected plants (Munns and Tester Citation2008). In salt-affected plants, the water deficiency results in nutritional disorders and oxidative stress that leads to higher accumulation of NaCl in the cytosol and cell organelles that disturbs the ionic imbalance and water uptake (Munns and Tester Citation2008; Tavakkoli et al. Citation2010). However, tomato is a moderately salt-tolerant crop by regulating water and ionic homeostasis (Martinez-Rodriguez et al. Citation2008) and reports suggest that salinity can positively moderate tomato fruit metabolism and increases the sensorial/nutritional value of the crop (D’Amico et al. Citation2003). It also increases the total soluble content (°Brix) and the titratable acidity which directly corresponds to the tomato fruit quality. Besides that, a high concentration of salt in irrigation water stimulates the defense system of the plant, which tends to increase the secondary metabolites (carotenoids, polyphenols, and terpenoids) in different tissues (Ndhlala et al. Citation2010). In hydroponically grown tomato plants, high salinity can increase lycopene biosynthesis (Wu and Kubota Citation2008). Despite the above-mentioned positive reports Cuartero et al. (Citation2006) suggested that high salinity adversely affects seed germination and inhibits growth and fruit development in tomato plants. Different plants evolve various methods to overcome the high concentrations of salts from their surrounding environment (Chen and Murata Citation2002; Munns and Gilliham Citation2015). In case of tomato following mitigation strategies to overcome salinity stress has been used effectively.
The ameliorative effects of Se applications in tomato plants significantly improve root and shoot fresh weight and shoot dry weight and stem growth (diameter). It also improves relative water contents (Diao et al. Citation2014; Mozafariyan et al. Citation2016) as well as photosynthetic activities such as the net photosynthetic rate, the actual photochemical efficiency of photosystem II (PSII), maximum quantum yield of PSII (Fv/Fm), photochemical quenching coefficient (qP), and non-photochemical quenching coefficient (qN) of tomato cultivars (Diao et al. Citation2014). Another level of Se protection comes at the cell membrane levels against lipid peroxidation thereby reducing oxidative stress by chloroplast regulation. This is strongly linked with increasing the photosynthetic rates by improving the PSII, and thereby, enhanced plant strength (Diao et al. Citation2014). Another element used for amelioration of salinity stress is Ca2+ (Arshi et al. Citation2010; Khan et al. Citation2010). There are reports that suggest calcium prevent sodium chloride toxicity (Abdel Latef Citation2011; Tanveer et al. Citation2020). The combined application of NaCl and CaCl2 improves the growth parameters increased antioxidant activity (Jaleel et al. Citation2008) In case of tomato plants the ameliorative effects of calcium were seen positive on germination and growth indices with increased concentration of calcium. The best mitigation was recorded at 10 mM calcium, an increase in the promptness index by 20.7%, seedling length and vigor by 15.1% and germination stress tolerance index by 20.7%. It also improved root fresh and dry weight, shoot fresh and dry weight, relative water content, and leaf area (Tanveer et al. Citation2020).
The endophytic plant-fungal (EP) association can alleviate salinity stress in plants (Gupta et al. Citation2020). In salinity stress, an increase ROS accumulation occurs in tomato, rice, and dunegrass without endophytic associations, whereas the EP had low values of ROS accumulation by mitigating several different pathways (Singh et al. Citation2011). The measurement of total biomass is employed to calculate plant’s potential to endure salinity. The role of endophytic fungi in salinity tolerance in host plants by increasing biomass as compared to non-endophytic plants (NEP) has been emphasized by (Rho et al. Citation2018; Gupta et al. Citation2020). In another report after the colonization of an endophytic fungus, Piriformospora indica in tomato plants the proline accumulation was reduced as the toxic effects of salinity were reduced (Abdelaziz et al. Citation2019). EP maintains the integrity of photosystem II in tomato plants by repairing salt-induced degradation of D1/D2/Cytb 559 complex by the accumulation of glycine betaine (Rivero et al. Citation2014). Apart from EP There are several reports on tomato that suggests Arbuscular mycorrhizal fungi (AMF) is an effective method for bio-amelioration of salinity stress. AMF installs a range of biochemical and physiological programs that act in a combined way to give enhanced salinity tolerance to the host plant. Some of the well-known mechanisms include preservation of cell ultrastructure, superior water use efficiency and osmoprotection, improved nutrient uptake and maintenance of ionic homeostasis, enhanced photosynthetic efficiency, and reinforced antioxidant metabolism (Ouziad et al. Citation2006; Olías et al. Citation2009; Hajiboland et al. Citation2010; Latef and Chaoxing Citation2011; Khalloufi et al. Citation2017). The effects of commercially available AMF inoculant (Glomus sp. mixture) on growth and nutrient acquisition in tomato under salinity stress was reported by Balliu et al. (Citation2015). The salinity stress resulted in reduce leaf area ratio, net assimilation rate and root relative growth rate in NEP as compared to EP. Further, relative uptake rate of nutrient elements values of EP tomato plants in non-saline and moderate saline conditions were higher than in NEP.
Plant hormones ethylene mediates salinity stress response
Salinity symptoms first appear such as reduced surface expansion, yellowing, browning, epinasty, reduced growth, senescence, and necrosis first appears in older leaf which then spread to younger leaf (Acosta-Motos et al. Citation2017). Plant hormones play an important role in mediating various plant responses between cell and environment, including plant response to salinity (NaCl) stress. Under high NaCl stress condition, the chloride accumulating in the calyx stimulates ethylene production. This will negatively affect persimmon fruit qualitatively and quantitatively (Besada et al. Citation2016). The leaf epinasty by either salinity stress alone (200 mM NaCl) or salt induces ethylene overproduction is a sensitive marker of salt-sensitivity in tomato cutivars. This provides a genetic basis to discover and select salinity susceptible and tolerant cultivar (Jones and El-Beltagy Citation1989). In vitro induced salinity physiological and biochemical responses of ‘Roma’ and dwarf F1 hybrid ‘Patio’ tomato revealed possible implication of ethylene in causing symptoms associated with high salt. Salinity stress (50–200 mM) significantly augments ethylene production in the headspace and shows increased leaf epinasty in each cultivar (Shibli et al. Citation2007). In salinized (100 mM NaCl) Moneymaker cultivar of tomato, the endogenous ethylene precursor ACC content increases up to 12-fold higher than in the control plant, which leads to leaf senescence (Ghanem et al. Citation2008). In tomato cvs. 100 RILs and Boludo F1, the endogenous ethylene precursor ACC in the xylem sap was 40% higher in control than high vigor and low vigor combinations regulate leaf size and senescence (Albacete et al. Citation2009). Thus, various negative effects of ethylene such as epinasty, reduced plant growth, deformed leaf morphology, senescence, necrosis, and cell death are similar to those which are actually caused by high sanity (Ansari et al. Citation2015). This will suggest that salinity symptoms appear in plant is mediated via ethylene ((A)). In vitro application of ethylene inhibitors significantly reduces various negative effects of ethylene overproduction due to high salinity stress in cultivars ‘Roma’ and dwarf F1 hybrid ‘Patio’. This will include reduced plant growth epinasty, deformed morphology of leaf, senescence, necrosis, cell death, and leaf and flower abscission (Shibli et al. Citation2007).
Figure 3. Ethylene metabolism in response to salinity stress in tomato plant. Salinity stress symptoms appear in tomato young and old leaves, and modulated expression of ACS and ACO (1-aminocyclopropane-1-carboxylic acid oxidase) by salinity stress induce sanity stress response in tomato (A). Inhibition of ethylene synthesis and ethylene action by ethylene inhibitors mitigates various adverse effects of sanity stress in tomato plant, and thereby improved productivity (B).
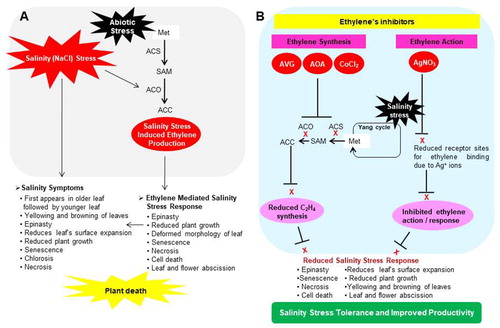
Calcium-dependent SOS signaling: ethylene and salinity stress
Soil salinity is of greater concern across the world with special relevance to crop plants which impair crop productivity and growth. In order to cope with prevailing stress condition, plants have developed a variety of defense mechanisms which involves morphological and physiological changes, in which many genes and pathways are involved (Julkowska and Testerink Citation2015). Several studies on plant salt stress in Arabidopsis have led to the identification of some important pathways which are involved in salt stress signal transduction (Xiong et al. Citation2002). These include mainly SOS pathway (SOS3–SOS2–SOS1) which regulates ion homeostasis under salt stress and results in Na+ efflux and vacuolar compartmentation (Sanchez-Barrena et al. Citation2005); calcium-dependent protein kinase pathway (CDPK) pathway, which plays an important role in osmotic stress (Sanchez-Barrena et al. Citation2005); and the mitogen-activated protein kinase (MAPK) pathway, which is important for counteracting both abiotic and biotic stresses (Nakagami et al. Citation2005).
In plant cells, calcium has been found to be a major point of signaling cross talk between abiotic, developmental, hormonal, and biotic stresses (Sanders et al. Citation1999; Kiegle et al. Citation2000). Salt stress-induced Ca2+ signal activates different components of SOS (SOS1, SOS2, and SOS3) pathway to achieve cellular ion homeostasis (Zhu Citation2002). Genetic analysis has confirmed that SOS3, SOS2, and SOS1 function in a common pathway of salt tolerance (Zhu et al. Citation1998). SOS3, a calcineurin B-like calcium-binding protein, has been found to be direct calcium ion sensor in response to salt stress, and subsequently transmits it to SOS2, a serine/threonine protein kinase as evident from study in Arabidopsis. Na+−induced activity of SOS1, a putative plasma membrane Na+/H+ antiporter depends on SOS3–SOS2 complex through phosphorylation (Shabala et al. Citation2005, Zhu Citation2002). Study in tomato and Arabidopsis has revealed the role of SOS1 in the partitioning of toxic ion between plant organs (Olías et al. Citation2009; Ji et al. Citation2013). This suggests that SOS pathway is branched and is a point of cross talk with other signaling pathways (Katiyar-Agarwal et al. Citation2006; Jaspers et al. Citation2010). SOS1, SOS2, and SOS3 pathways have been found to be spatially separated in plants. SOS1 and SOS2 are expressed in both roots and shoots, while SOS3 is mainly expressed in root tissues to overcome salt stress (Liu et al. Citation2000; Shi et al. Citation2002; Quan et al. Citation2007).
Characterization of new tomato mutant has led to the identification of SlCBL10, calcineurin B-like protein, which ensures plant growth under salt stress by regulating Na+ and Ca2+ homeostasis (Egea et al. Citation2018). Recent studies using genetic and biochemical approaches have revealed a novel component of SOS signaling pathway i.e. SOS3-like calcium-binding proteins (SCaBP8) which acts as Ca2+ sensor involved in leaf Na+ exclusion (Lin et al. Citation2009, Xie et al. Citation2009). SCaBP8 like SOS3 interacts with and recruits SOS2 to the plasma membrane, thus, activating SOS1 Na+/H+ antiporter activity (Guo et al. Citation2001). Through microarray analysis and suppression subtractive hybridization, 24 early salt response genes were identified in tomato root (Ouyang et al. Citation2007). A large number of these genes regulated by salt stress was found to encode unknown proteins, indicating that a lot of investigation is still required for understanding the mechanism of salt tolerance in tomato. Two calcium-binding proteins and several protein kinases have also been identified in tomato which indicates that a conserved SOS signaling pathway exists to regulate plant responses to salt stress (Ouyang et al. Citation2007). Transcriptomic analysis and genome sequencing in tomato has further added stress tolerant genes to the list (Bolger et al. Citation2014). The tomato whole genome sequencing and functional genomics projects taken up in recent years will further strengthen the understanding of novel salt tolerance-related genes (Mueller et al. Citation2005; Fei et al. Citation2006).
In addition to calcium-induced SOS signaling, plant hormones, mainly ethylene, and abscisic acid (ABA), also play an important role in overcoming salt stress (Tanaka et al. Citation2005). Ethylene plays an important role in combating salt stress through up-regulation of rate-limiting enzyme of ethylene biosynthesis. However, diverse roles of ethylene have been reported in response to salinity stress (Tao et al. Citation2015). Huang et al. (Citation2004) identified a novel ethylene-responsive factor called TERF1 from tomato, which might function as a link between the ethylene and osmotic stress pathways. Expression of several genes implicated in defense, auxin, and ethylene signaling was affected by the SOS2 mutation in contrast to SOS3 mutation, suggesting multiple novel roles of SOS2 in plant growth, development, and protection from stress injury as evident through microarray analyses (Kamei et al. Citation2005). Still, there are many lacunas in an understanding of the complexity of signaling pathways which needs to be further elucidated in order to achieve combined stress resilience by balancing hormonal signaling. Several studies have revealed that SOS pathway is branched, incorporates additional functions, and is a point of cross talk with other signaling pathways but this area needs to be further explored (Katiyar-Agarwal et al. Citation2006; Jaspers et al. Citation2010).
Conclusion
However, the mechanisms of tomato plant which help to induce salinity tolerance in cells, tissues, and at entire plant level, need to be explored. Here, we discuss the potential application of the nature of the genetic variation obtained in vitro response to salinity stress mediated through plant hormone ethylene, and its future relevance for crop improvement. The global climate change is an important constraint affecting crop productivity. Several environmental stresses including salinity stress showed unkind deduction in tomato crop productivity. The role of biotechnology and plant tissue culture in crop improvement is immense. In light of these facts, in the present study, an effort has been made to improve tomato crop for better yield and salinity stress tolerance. Several recent studies would be a step to contribute to scientific challenges to secure food for increasing population which is envisaged to cross 9 billion by 2050.
Realizing the potential significance of plant tissue culture technology to produce elite, high yielding, disease free, and stress-tolerant plants, the present investigation was made to produce salinity stress tolerant somaclone plantlets. Our study will provide the first evidence of the involvement of ACS, beta-CAS expression mediated production of endogenous ethylene and cyanide and thereby antioxidants and photosynthetic machinery in tomato somaclone plantlets to improve salinity stress tolerance. This will help to comprehend the salinity stress mechanism in tomato plants involving ethylene, salicylic acid, cyanide detoxification, antioxidants, and photosynthetic pathways at transcript and protein levels. The present study will offer ideas to a number of molecular and breeding programs with aim of manipulating tomato and other related crops genetically for better yield and improved resistance to salinity stress, and to exploit the technology in an integrated manner.
Acknowledgements
MWA is thankful to the Department of Science and Technology (DST) for funding under DST fast track scheme of young scientist. Work on signal transduction and plant stress tolerance in NT’s laboratory is partially supported by the Department of Science and Technology (DST) and Department of Biotechnology (DBT), Government of India.
Disclosure statement
No potential conflict of interest was reported by the author(s).
Additional information
Funding
Notes on contributors
Yalaga Rama Rao
Yalaga Rama Rao is currently a PhD student of Vignan University, India. He has been Assistant Professor in Madwalabu University, Ethiopia.
Mohammad Wahid Ansari
Mohammad Wahid Ansari is currently an Assistant Professor, Department of Botany (ZHDC), University of Delhi. His special interest lies in plant hormone homeostasis and cross talk to improve abiotic stress tolerance in plants. He is currently in Editorial Board of Plant Signaling and Behaviors and Plos One Journal.
Anil Kumar Singh
Anil Kumar Singh is presently working as Senior Scientist at ICAR-Indian Institute of Agricultural Biotechnology, Ranchi. He has made outstanding contribution in the area of plant functional genomics. He is serving as editor of journal Frontiers in Plant Science.
Niharika Bharti
Niharika Bharti is presentlyDepartment of Botany (ZHDC), University of Delhi, and has completed PhD from Department of Botany, University of Delhi. Her research interest lies on plant physiology.
Varsha Rani
Varsha Rani is an Assistant Professor in Jharkhand Rai University, and has completed MSc and PhD degree from G.B. Pant University of Agriculture & Technology, Pantnagar, Uttarakhand.
Amit Verma
Amit Verma is Assistant Professor, Department of Biochemistry, S D Agricultural University. He has completed his PhD from GBPUAT, Pantnagar, India. His areas of interest include enzymology, nutraceuticals and plant metabolism under climate change.
Ramwant Gupta
Ramwant Gupta is currently an assistant professor in Biology at Fiji National University, Fiji Islands. His current research project focus on plant reproductive biology, pollen-pistil dynamics in particular, to understand sexual reproduction in plants under varied climatic condition.
Ranjan Kumar Sahoo
Ranjan Kumar Sahoo is currently an Assistant Professor in Centurion University of Technology and Management, Bhubaneswar, India, and has completed PhD in Biotechnology from Utkal University. He research interest focuses on abiotic stress tolerance in plants.
Zahid Hameed Siddiqui
Zahid Hameed Siddiqui is presently an Assistant Professor in the Department of Biology at University of Tabuk, Kingdom of Saudi Arabia. His research interests include conservation biology, secondary metabolite production and environmental pollution.
Zahid Khorshid Abbas
Zahid Khorshid Abbas is Associate Professor in the Department of Biology at University of Tabuk, Kingdom of Saudi Arabia. His research interests include conservation biology and plant adaptation to environmental change.
Gurdeep Bains
Gurdeep Bains is Associate professor in Department of Plant Physiology, GB Pant University of Agriculture and Technology, Pantnagar, India. Her research interest lies on mango malformation with particular emphasis to ethylene.
Brajendra
Brajendra is currently Senior Scientist in ICAR-Indian Institute of Rice Research, Rajendranagar, Hyderabad, Telangana. His research interest focuses in the production of rice under environmental stresses.
S.K. Guru
S.K. Guru is Associate professor in Department of Plant Physiology, GB Pant University of Agriculture and Technology, Pantnagar, India. His research interest lies on Weed Biology and Plant Molecular Biology.
Randeep Rakwal
Randeep Rakwal is a Professor of TIAS and Faculty of Health and Sport Sciences, University of Tsukuba (Tsukuba, Japan). He has completed PhD in Biochemistry and Biotechnology, Tokyo University of Agriculture and Technology, Japan, with Monbusho Scholarship. He has researched on environmental stress biology. He is also one of the initiators of INPPO, and the founding member.
Narendra Tuteja
Narendra Tuteja has been Senior Scientist at ICGEB, New Delhi, and Professor and Director at Amity University, Noida. He also worked as postdoc in NIH, Bethesda, USA and UCLA, Los Angeles. He has been Associate Editor and member of Editorial Board of international journals.
Vellanki R. Kumar
Vellanki R. Kumar is Professor and Dean Admission in Vignan University, India. His research interest focuses on effect of heterosis in hexaploid tritcale.
References
- Abdallah NA, Moses V, Prakash CS. 2014. The impact of possible climate changes on developing countries: the needs for plants tolerant to abiotic stresses. GM Crops Food. 5:77–80. doi: 10.4161/gmcr.32208
- Abdelaziz ME, Abdelsattar M, Abdeldaym EA, Atia MA, Mahmoud AWM, Saad MM, Hirt H. 2019. Piriformospora indica alters Na+/K+ homeostasis, antioxidant enzymes and LeNHX1 expression of greenhouse tomato grown under salt stress. Sci Hort. 256:108532. doi: 10.1016/j.scienta.2019.05.059
- Abdel Latef AAH. 2011. Ameliorative effect of calcium chloride on growth, antioxidant enzymes, protein patterns and some metabolic activities of canola (Brassica napus L.) under seawater stress. J Plant Nutr. 34(9):1303–1320. doi: 10.1080/01904167.2011.580817
- Acosta-Motos JR, Dias-Vivancos P, Álvarez S, Fernández-García N, Sánchez-Blanco MJ, Hernández JA. 2015. NaCl-induced physiological and biochemical adaptative mechanismsin the ornamental Myrtus communis L. plants. J Plant Physiol. 183:41–51. doi: 10.1016/j.jplph.2015.05.005
- Acosta-Motos J, Ortuño MF, Bernal-Vicente A, Diaz-Vivancos P, Sanchez-Blanco MJ, Hernandez JA. 2017. Plant responses to salt stress: adaptive mechanisms. Agronomy. 7:1–38. doi: 10.3390/agronomy7010018
- Al-Daej MI. 2018. Salt tolerance of some tomato (Solanum lycoversicum L.) cultivars for salinity under controlled conditions. Am J Plant Physiol. 13:58–64. doi: 10.3923/ajpp.2018.58.64
- Albacete A, Martínez-Andújar C, Ghanem ME, Acosta M, Sánchez-Bravo J, Asins MJ, Cuartero J, Lutts S, Dodd IC, Pérez-Alfocea F. 2009. Rootstock-mediated changes in xylem ionic and hormonal status are correlated with delayed leaf senescence, and increased leaf area and crop productivity in salinized tomato. Plant Cell Environ. 32:928–938. doi: 10.1111/j.1365-3040.2009.01973.x
- Albaladejo I, Meco V, Plasencia F, Flores FB, Bolarin MC, Egea I. 2017. Unravelling the strategies used by the wild tomato species Solanum pennellii to confront salt stress: from leaf anatomical adaptations to molecular responses. Environ Exp Bot. 135:1–12. doi: 10.1016/j.envexpbot.2016.12.003
- Anil VS, Bennur S, Lobo S. 2018. Somaclonal variations for crop improvement: selection for disease resistant variants in vitro. Plant Sci. 5:44–54.
- Annunziata MG, Ciarmiello LF, Woodrow P, Maximova E, Fuggi A, Carillo P. 2017. Durum wheat roots adapt to salinity remodeling the cellular content of nitrogen metabolites and sucrose. Front Plant Sci. 7:2035. doi: 10.3389/fpls.2016.02035
- Ansari MW, Kaushik S, Bains G, Tula S, Joshi B, Rani V, Wattal RK, Rakwal R, Shukla A, Pant RC, et al. 2019. Cyanide produced with ethylene by ACS and its incomplete detoxification by β-CAS in mango inflorescence leads to malformation. Sci Rep. 9:18361. doi: 10.1038/s41598-019-54787-7
- Ansari MW, Rani V, Shukla A, Bains G, Pant RC, Tuteja N. 2015. Mango (Mangifera indica L.) malformation: a malady of stress ethylene origin. Physiol Mol Biol Plants. 21:1–8. doi: 10.1007/s12298-014-0258-y
- Arshi A, Ahmad A, Aref IM, Iqbal M. 2010. Calcium interaction with salinity-induced effects on growth and metabolism of soybean (Glycine max L.) cultivars. J Environ Biol. 31(5):795.
- Bai Y, Kissoudis C, Yan Z, Visser RGF, van der Linden G. 2018. Plant behaviour under combined stress: tomato responses to combined salinity and pathogen stress. Plant J. 93:781–793. doi: 10.1111/tpj.13800
- Balliu A, Sallaku G, Rewald B. 2015. AMF inoculation enhances growth and improves the nutrient uptake rates of transplanted, salt-stressed tomato seedlings. Sustainability. 7:15967–15981. doi: 10.3390/su71215799
- Besada C1, Gil R2, Bonet L2, Quiñones A2, Intrigliolo D2, Salvador A2. 2016 Mar. Chloride stress triggers maturation and negatively affects the postharvest quality of persimmon fruit. Involvement of calyx ethylene production. Plant Physiol Biochem. 100:105–112. Epub 2016 Jan 14. doi: 10.1016/j.plaphy.2016.01.006
- Bolger A, Scossa F, Bolger ME, Lanz C, Maumus F, Tohge T. 2014. The genome of the stress tolerant wild tomato species Solanum pennellii. Nat Genet. 46:1034–1038. doi: 10.1038/ng.3046
- Chandler SF, Thorpe TA. 1986. Variation from plant tissue cultures: biotechnological application to improving salinity tolerance. Biotechnol Adv. 4:117–135. doi: 10.1016/0734-9750(86)90007-8
- Chen THH, Murata N. 2002. Enhancement of tolerance of abiotic stress by metabolic engineering of betaines and other compatible solutes. Curr Opin Plant Biol. 5:250–257. doi: 10.1016/S1369-5266(02)00255-8
- Chrysargyris A, Michailidi E, Tzortzakis N. 2018. Physiological and biochemical responses of Lavandula angustifolia to salinity under mineral foliar application. Front Plant Sci. 9:489. doi: 10.3389/fpls.2018.00489
- Costa-Rodrigues J, Pinho O, Monteiro PRR. 2018. Can lycopene be considered an effective protection against cardiovascular disease? Food Chem. 245:1148–1153. doi: 10.1016/j.foodchem.2017.11.055
- Cuartero J, Bolarin MC, Asins MJ, Moreno V. 2006. Increasing salt tolerance in the tomato. J Exp Bot. 57:1045–1058. doi: 10.1093/jxb/erj102
- Dahal K, Li XQ, Tai H, Creelman A, Bizimungu B. 2019. Improving potato stress tolerance and tuber yield under a climate change scenario – a current overview. Front Plant Sci. 10:563. doi: 10.3389/fpls.2019.00563
- D’Amico ML, Izzo R, Tognoni F, Pardossi A, Navari-Izzo F. 2003. Application of diluted sea water to soilless culture of tomato (Lycopersicon esculentum Mill.): effects on plant growth, yield, fruit quality and antioxidant capacity. J Food Agr Environ. 1:112–116.
- De-la-Torre-González A, Navarro-León E, Albacete A, Blasco B, Ruiz JM. 2017. Study of phytohormone profile and oxidative metabolism as key process to identification of salinity response in tomato commercial genotypes. J Plant Physiol. 216:164–173. doi: 10.1016/j.jplph.2017.05.016
- Diao M, Ma L, Wang J, Cui J, Fu A, Liu HY. 2014. Selenium promotes the growth and photosynthesis of tomato seedlings under salt stress by enhancing chloroplast antioxidant defense system. J Plant Grow Regul. 33:671–682. doi: 10.1007/s00344-014-9416-2
- Egea I, Pineda B, Ortíz-Atienza A, Plasencia FA, Drevensek S, García-Sogo B, Yuste-Lisbona FJ, Barrero-Gil J, Atarés A, Flores FB, et al. 2018. The SlCBL10 calcineurin B-like protein ensures plant growth under salt stress by regulating Na+ and Ca2+ homeostasis. Plant Physiol. 176:1676–1693. doi: 10.1104/pp.17.01605
- Epstein E, Norlyn JD, Rush DW, Kingsbury RW, Kelly DB, Gunningham GA, Wrona AF. 1980. Saline culture of crops: a genetic approach. Science. 210:399–404. doi: 10.1126/science.210.4468.399
- Evans DA, Sharp WR. 1986. Applications of somaclonal variation. Nature Biotechnol. 4:528–532. doi: 10.1038/nbt0686-528
- Fei Z, Tang X, Alba R, Giovannoni J. 2006. Tomato expression database (TED): a suite of data presentation and analysis tools. Nucleic Acids Res. 34:766–770. doi: 10.1093/nar/gkj110
- Foolad MR. 2004. Recent advances in genetics of salt tolerance in tomato. Plant Cell Tiss Org. 76:101–119. doi: 10.1023/B:TICU.0000007308.47608.88
- García-Martí M, Piñero MC, García-Sanchez F, Mestre TC, López-Delacalle M, Martínez V, Rivero RM. 2019. Amelioration of the oxidative stress generated by simple or combined abiotic stress through the K+ and Ca2+ supplementation in tomato plants. Antioxidants. 8(4). doi:10.3390/antiox8040081.
- Gerszberg A, Hnatuszko-Konka K. 2017. Tomato tolerance to abiotic stress: a review of most often engineered target sequence. Plant Growth Regul. 83:175–198. doi: 10.1007/s10725-017-0251-x
- Ghanem ME, Albacete A, Martínez-Andújar C, Acosta M, Romero-Aranda R, Dodd IC, Lutts S, Pérez-Alfocea F. 2008. Hormonal changes during salinity-induced leaf senescence in tomato (Solanum lycopersicum L.). J Exp Bot. 59:3039–3050. doi: 10.1093/jxb/ern153
- Ghassemi F, Jakeman AJ, Nix HA. 1995. Salinisation of land and water resources: human causes, extent, management and case studies. Sydney: UNSW Press.
- Golldack D, Li C, Mohan H, Probst N. 2014. Tolerance to drought and salt stress in plants: unraveling the signaling networks. Front Plant Sci. 5:151. doi: 10.3389/fpls.2014.00151
- Gorham J, Läuchli A, Leidi EO. 2010. Plant responses to salinity in physiology of cotton. eds JM Stewart, DM Oosterhuis, JJ Heitholt, JR Mauney. Dordrecht: Springer, 129–141.
- Grabowska M, Wawrzyniak D, Rolle K, Chomczyński P, Oziewicz S, Jurga S, Barciszewski J. 2019. Let food be your medicine: nutraceutical properties of lycopene. Food Funct. 10:3090–3102. doi: 10.1039/C9FO00580C
- Guo Y, Halfter U, Ishitani M, Zhu JK. 2001. Molecular characterization of functional domains in the protein kinase SOS2 that is required for plant salt tolerance. Plant Cell. 13:1383–1400. doi: 10.1105/TPC.010021
- Guo LL, Hao LH, Jia HH, Li F, Zhang XX, Cao X, Xu M, Zheng YP. 2018. Effects of NaCl stress on stomatal traits, leaf gas exchange parameters, and biomass of two tomato cultivars. Ying Yong Sheng Tai Xue Bao. 29:3949–3958.
- Gupta S, Schillaci M, Walker R, Smith PMC, Watt M, Roessner U. 2020. Alleviation of salinity stress in plants by endophytic plant-fungal symbiosis: current knowledge, perspectives and future directions. Plant Soil. doi:10.1007/s11104-020-04618-w.
- Gurbachan S. 2018. Climate change and sustainable management of salinity in agriculture. Res Med Eng Sci. 6(2):1–7.
- Hajiboland R, Aliasgharzadeh N, Laiegh SF, Poschenrieder C. 2010. Colonization with arbuscular mycorrhizal fungi improves salinity tolerance of tomato (Solanum lycopersicum L.) plants. Plant Soil. 331:313–327. doi: 10.1007/s11104-009-0255-z
- Halim NSA, Tan BC, Midin MR, Madon M, Khalid N, Yaacob JS. 2017. Abscisic acid and salinity stress induced somaclonal variation and increased histone deacetylase (HDAC) activity in Ananas comosus var. MD2. Plant Cell Tissue Organ Cult. 133(6). doi: 10.1007/s11240-017-1367-4
- Haq TU, Gorham J, Akhtar J, Akhtar N, Steele KA. 2010. Dynamic quantitative trait loci for salt stress components on chromosome 1 of rice. Functional Plant Biol. 37(7):634–645. doi: 10.1071/FP09247
- Hossain MS. 2019. Present scenario of global salt affected soils, its management and importance of salinity research. Int J Biol Sci. 1:1–3.
- Huang Z, Zhang Z, Zhang X, Zhang H, Huang D, Huang R. 2004. Tomato TERF1 modulates ethylene response and enhances osmotic stress tolerance by activating expression of downstream genes. FEBS Lett. 573:110–116. doi: 10.1016/j.febslet.2004.07.064
- Isayenkov SV, Maathuis FJM. 2019. Plant salinity stress: many unanswered questions remain. Front Plant Sci. 10:80. doi: 10.3389/fpls.2019.00080
- Jaleel CA, Kishorekumar A, Manivannan P, Saankar B, Gomathinayagam M, Panneerselvam R. 2008. Salt stress mitigation by calcium chloride in Phyllanthus amarus. Acta Bot Croat. 67(1):53–62.
- Jaspers P, Brosché M, Overmyer K, Kangasjär J. 2010. The transcription factor interacting protein RCD1 contains a novel conserved domain. Plant Signal Behav. 5:78–80. doi: 10.4161/psb.5.1.10293
- Ji HT, Pardo JM, Batelli G, Van Oosten MJ, Bressan RA, Li X. 2013. The salt overly sensitive (SOS) pathway: established and emerging roles. Mol Plant. 6:275–286. doi: 10.1093/mp/sst017
- Johansen K, Morton MJL, Malbeteau YM, Aragon B, Al-Mashharawi SK, Ziliani MG, Angel Y, Fiene GM, Negrão SSC, Mousa MAA, et al. 2019 Mar 29. Unmanned aerial vehicle-based phenotyping using morphometric and spectral analysis can quantify responses of wild tomato plants to salinity stress. Front Plant Sci. 10:370. doi:10.3389/fpls.2019.00370.eCollection 2019. doi: 10.3389/fpls.2019.00370
- Johnson HE, Broadhurst D, Goodacre R, Smith AR. 2003. Metabolic fingerprinting of salt-stressed tomatoes. Phytochemistry. 62:919–928. doi: 10.1016/S0031-9422(02)00722-7
- Jones RA, El-Beltagy AS. 1989. Epinasty promoted by salinity or ethylene is an indicator of salt-sensitivity in tomatoes. Plant Cell Environ. 12:813–817. doi: 10.1111/j.1365-3040.1989.tb01643.x
- Joseph JT, Poolakkalody NJ, Shah JM. 2018. Plant reference genes for development and stress response studies. J Biosci. 43:173–187. doi: 10.1007/s12038-017-9728-z
- Julkowska MM, Testerink C. 2015. Tuning plant signaling and growth to survive salt. Trends Plant Sci. 20:586–594. doi: 10.1016/j.tplants.2015.06.008
- Kalaji HM, Jajoo A, Oukarroum A, Brestic M, Zivcak M, Samborska IA, Cetner MD, Łukasik I, Goltsev V, Ladle RJ. 2016. Chlorophyll a fluorescence as a tool to monitor physiological status of plants under abiotic stress conditions. Acta Physiol Planta. 38(4). doi:10.1007/s11738-016-2113-y.
- Kamei A, Seki M, Umezawa T, Ishida J, Satou M, Akiyama K, Zhu JK, Shinozaki K. 2005. Analysis of gene expression profiles in Arabidopsis salt overly sensitive mutants sos2-1 and sos3-1. Plant Cell Environ. 28:1267–1275. doi: 10.1111/j.1365-3040.2005.01363.x
- Katiyar-Agarwal S, Zhu J, Kim K, Agarwal M, Fu X, Huang A, Zhu JK. 2006. The plasmamembrane Na+/H+ antiporter SOS1 interacts with RCD1 and functions in oxidative stress tolerance in Arabidopsis. Proc Natl Acad Sci USA. 103:18816–18821. doi: 10.1073/pnas.0604711103
- Kaushal SS, Duan S, Doody TR, Haq S, Smith RM, Newcomer Johnson TA, Newcomb KD, Gorman J, Bowman N, Mayer PM, et al. 2017. Human-accelerated weathering increases salinization, major ions, and alkalinization in fresh water across land use. Appl Geochem. 83:121–135. doi: 10.1016/j.apgeochem.2017.02.006
- Khalloufi M, Martínez-Andújar C, Lachaâl M, Karray-Bouraoui N, Pérez- Alfocea F, Albacete A. 2017. The interaction between foliar GA3 application and arbuscular mycorrhizal fungi inoculation improves growth in salinized tomato (Solanum lycopersicum L.) plants by modifying the hormonal balance. J Plant Physiol. 214:134–144. doi:10.1016/j.jplph.2017.04.012.
- Khan MN. 2016. Growth and physiological attributes of tomato (Lycopersicon esculentum Mill.) genotypes as affected by NaCl stress. American J Plant Sci. 7:453–460. doi: 10.4236/ajps.2016.73039
- Khan MN, Siddiqui MH, Mohammad F, Naeem M, Khan MMA. 2010. Calcium chloride and gibberellic acid protect linseed (Linum usitatissimum L.) from NaCl stress by inducing antioxidative defence system and osmoprotectant accumulation. Acta Physiol Plant. 32(1):121. doi:10.1007/s11738-009-0387-z.
- Kiegle E, Moore CA, Haseloff J, Tester MA, Knight MR. 2000. Cell-type-specific calcium responses to drought, salt and cold in the Arabidopsis root. Plant J. 23:267–278. doi: 10.1046/j.1365-313x.2000.00786.x
- Krishna H, Alizadeh M, Singh D, Singh U, Chauhan N, Eftekhari M, Sadh RK. 2016. Somaclonal variations and their applications in horticultural crops improvement. 3 Biotech. 6:54. doi: 10.1007/s13205-016-0389-7
- Krishna R, Karkute SG, Ansari WA, Jaiswal DK, Verma JP, Singh M. 2019. Transgenic tomatoes for abiotic stress tolerance: status and way ahead. 3 Biotech. 9:143. doi: 10.1007/s13205-019-1665-0
- Latef AAHA, Chaoxing H. 2011. Effect of arbuscular mycorrhizal fungi on growth, mineral nutrition, antioxidant enzymes activity and fruit yield of tomato grown under salinity stress. Sci Hortic. 127:228–233. doi:10.1016/j.scienta.2010.09.020.
- Lestari EG. 2006. In vitro selection and somaclonal variation for biotic and abiotic stress tolerance. Biodiversita. 7:297–301. doi: 10.13057/biodiv/d070320
- Lin Z, Zhong S, Grierson D. 2009. Recent advances in ethylene research. J Exp Bot. 60:3311–3336. doi: 10.1093/jxb/erp204
- Liu J, Ishitani M, Halfter U, Kim CS, Zhu JK. 2000. The Arabidopsis thaliana SOS2 gene encodes a protein kinase that is required for salt tolerance. Proc Nat Acad Sci USA. 97:3730–3734. doi: 10.1073/pnas.97.7.3730
- Machado RMA, Serralheiro RP. 2017. Soil salinity: effect on vegetable crop growth. management practices to prevent and mitigate soil salinization. Horticulturae. 3:30. doi: 10.3390/horticulturae3020030
- Manaa A, Ben Ahmed H, Valot B, Bouchet JP, Aschi-Smiti S, Causse M, Faurobert M. 2011. Salt and genotype impact on plant physiology and root proteome variations in tomato. J Exp Bot. 62:2797–2813. doi: 10.1093/jxb/erq460
- Manchanda P, Kaur A, Gosal SS. 2018. Somaclonal variation for sugarcane improvement. In: Gosal S, Wani S, editors. Biotechnologies of crop improvement, Volume. Cham: Springer; p. 1.
- Mandal AK, Obi-Reddy GP, Ravisankar T. 2011. Digital database of salt affected soils in India using geographic information system. JSSWQ. 3:16–29.
- Manzano S, Aguado E, Martínez C, Megías Z, García A, Jamilena M. 2016. The ethylene biosynthesis gene CitACS4 regulates monoecy/andromonoecy in watermelon (Citrullus lanatus). PLoS One. 11:e0154362. doi: 10.1371/journal.pone.0154362
- Martinez-Rodriguez MM, Estan MT, Moyano E, Garcia-Abellan JO, Flores FB. 2008. The effectiveness of grafting to improve salt tolerance in tomato when an excluder genotype is used as scion. Environ Exp Bot. 63:392–401. doi: 10.1016/j.envexpbot.2007.12.007
- Martinez V, Nieves-Cordones M, Lopez-Delacalle M, Rodenas R, Mestre TC, Garcia-Sanchez F, Rubio F, Nortes PA, Mittler R, Rivero RM. 2018. Tolerance to stress combination in tomato plants: new insights in the protective role of melatonin. Molecules. 23(3). doi: 10.3390/molecules23030535
- Miller JM, Conn JE. 1980. Metabolism of hydrogen cyanide in higher plants. Plant Physiol. 65:1199–1202. doi: 10.1104/pp.65.6.1199
- Mitchell AE, Hong YJ, Koh E. 2007. Ten-year comparison of the influence of organic and conventional crop management practices on the content of flavonoids in tomatoes. J Agric Food Chem. 55:6154–6159. doi: 10.1021/jf070344+
- Mozafariyan M, Kamelmanesh MM, Hawrylak-Nowak B. 2016. Ameliorative effect of selenium on tomato plants grown under salinity stress. Arch Agron Soil Sci. 62:1368–1380. doi: 10.1080/03650340.2016.1149816
- Mueller LA, Solow TH, Taylor N, Skwarecki B, Buels R, Binns J, Lin C, Wright MH, Ahrens R, Wang Y. 2005. The SOL genomics network. A comparative resource for solanaceae biology and beyond. Plant Physiol. 138:1310–1317. doi: 10.1104/pp.105.060707
- Munns R, Gilliham M. 2015. Salinity tolerance of crops-what is the cost? New Phytol. 208:668–673. doi: 10.1111/nph.13519
- Munns R, Tester M. 2008. Mechanisms of salinity tolerance. Annu Rev Plant Biol. 59:651–681. doi: 10.1146/annurev.arplant.59.032607.092911
- Nakagami H, Pitzschke A, Hirt H. 2005. Emerging MAP kinase pathways in plant stress signalling. Trends Plant Sci. 10:339–346. doi: 10.1016/j.tplants.2005.05.009
- Ndhlala AR, Moyo M, Van Staden J. 2010. Natural antioxidants: fascinating or mythical biomolecules? Molecules. 15:6905–6930. doi: 10.3390/molecules15106905
- Olías R, Eljakaoui ZLJUN, De Morales PA, Marín-Manzano MC, Pardo JM, Belver A. 2009. The plasma membrane Na+/H+ antiporter SOS1 is essential for salt tolerance in tomato and affects the partitioning of Na+ between plant organs. Plant Cell Environ. 32:904–916. doi: 10.1111/j.1365-3040.2009.01971.x
- Ouyang B, Yang T, Hanxia L, Zhang L, Zhang Y, Zhang J, Fei Z, Ye Z. 2007. Identification of early salt stress response genes in tomato root by suppression subtractive hybridization and microarray analysis. J Exp Bot. 58:507–520. doi: 10.1093/jxb/erl258
- Ouziad F, Wilde P, Schmelzer E, Hildebrandt U, Bothe H. 2006. Analysis of expression of aquaporins and NaC/HC transporters in tomato colonized by arbuscular mycorrhizal fungi and affected by salt stress. Environ Exp Bot. 57:177–186. doi: 10.1016/j.envexpbot.2005.05.011
- Pan Y, Seymour GB, Lu C, Hu Z, Chen X, Chen G. 2012. An ethylene response factor (ERF5) promoting adaptation to drought and salt tolerance in tomato. Plant Cell Rep. 31:349–360. doi: 10.1007/s00299-011-1170-3
- Periago MJ, García-Alonso J, Jacob K. 2009. Bioactive compounds, folates and antioxidant properties of tomatoes (Lycopersicum esculentum) during vine ripening. Intr J Food Sci Nutr. 60:694–708. doi: 10.3109/09637480701833457
- Quan R, Lin H, Mendoza I, Zhang Y, Cao W, Yang Y, Shang M, Chen S, Pardo JM, Guo Y. 2007. SCABP8/CBL10, a putative calcium sensor, interacts with the protein kinase SOS2 to protect Arabidopsis shoots from salt stress. Plant Cell. 19:1415–1431. doi: 10.1105/tpc.106.042291
- Rahman MM, Hossain M, Hossain KFB, Sikder MT, Shammi M, Rasheduzzaman M, Hossain MA, Mahbubul-Alam AKM, Uddin MK. 2018. Effects of NaCl salinity on tomato (Lycopersicon esculentum Mill.) plants in a pot Experiment. Open Agric. 3:578–585. doi: 10.1515/opag-2018-0061
- Rengasamy P. 2006. World salinization with emphasis on Australia. J Exp Bot. 57:1017–1023. doi: 10.1093/jxb/erj108
- Rho H, Hsieh M, Kandel SL, Cantillo J, Doty SL, Kim S-H. 2018. Do endophytes promote growth of host plants under stress? A meta-analysis on plant stress mitigation by endophytes. Microb Ecol. 75:407–418. doi: 10.1007/s00248-017-1054-3
- Rivero RM, Mestre TC, Mittler R, Rubio F, Garcia-Sanchez F, Martinez V. 2014. The combined effect of salinity and heat reveals a specific physiological, biochemical and molecular response in tomato plants. Plant Cell Environ. 37:1059–1073. doi: 10.1111/pce.12199
- Rodríguez-Ortega WM, Martínez V, Nieves M, Simón I, Lidón V, Fernandez-Zapata JC, Martinez-Nicolas JJ, Cámara-Zapata JM, García-Sánchez F. 2019. Agricultural and physiological responses of tomato plants grown in different soilless culture systems with saline water under greenhouse conditions. Sci Rep. 9:6733. doi: 10.1038/s41598-019-42805-7
- Rouphael Y, Raimondi C, Lucini L, Carillo P, Kyriacou MC, Colla G, Cirillo V, Pannico A, El-Nakhel C, De-Pascale S. 2018. Physiological and metabolic responses triggered by omeprazole improve tomato plant tolerance to NaCl stress. Front Plant Sci. doi: 10.3389/fpls.2018.00249
- Sahoo RK, Ansari MW, Tuteja N. 2014. OsSUV3 transgenic rice maintained higher endogenous level of plant hormones to eradicate the adverse effects of salinity on crop productivity. Rice. 7:1–3. doi: 10.1186/s12284-014-0017-2
- Sanchez-Barrena MJ, Martinez-Ripoll M, Zhu JK, Albert A. 2005. The structure of the Arabidopsis thaliana SOS3: molecular mechanism of sensing calcium for salt stress response. J Mol Biol. 345:1253–1264. doi: 10.1016/j.jmb.2004.11.025
- Sanders D, Brownlee C, Harper JF. 1999. Communicating with calcium. Plant Cell. 11:691–706. doi: 10.1105/tpc.11.4.691
- Sato T, Theologis A. 1989. Cloning the mRNA encoding 1aminocyclopropane-1-carboxylate synthase, the key enzyme for ethylene biosynthesis in plants. Proc Natl Acad Sci USA. 86:6621–6625. doi: 10.1073/pnas.86.17.6621
- Shabala L, Cuin TA, Newman IA, Shabala S. 2005. Salinity-induced ion flux patterns from the excised roots of Arabidopsis sos mutants. Planta. 222:1041–1050. doi: 10.1007/s00425-005-0074-2
- Shah N, Anwar S, Xu J, Hou Z, Salah A, Khan S, Gong J, Shang Z, Qian L, Zhang C. 2018. The response of transgenic Brassica species to salt stress: a review. Biotechnol Lett. 40:1159–1165. doi: 10.1007/s10529-018-2570-z
- Shi H, Quintero FJ, Pardo JM, Zhu JK. 2002. The putative plasma membrane Na+/H+ antiporter SOS1 controls long distance Na+ transport in plants. Plant Cell. 14:465–477. doi: 10.1105/tpc.010371
- Shibli RA, Kushad M, Yousef GG, Lila MA. 2007. Physiological and biochemical responses of tomato microshoots to induced salinity stress with associated ethylene accumulation. Plant Growth Regul. 51:159–169. doi: 10.1007/s10725-006-9158-7
- Singh LP, Gill SS, Tuteja N. 2011. Unraveling the role of fungal symbionts in plant abiotic stress tolerance. Plant Signal Behav. 6:175–191. doi: 10.4161/psb.6.2.14146
- Tanaka Y, Sano T, Tamaoki M, Nakajima N, Kondo N, Hasezawa S. 2005. Ethylene inhibits abscisic acid-induced stomatal closure in Arabidopsis. Plant Physiol. 138:2337–2343. doi: 10.1104/pp.105.063503
- Tanveer K, Gilani S, Hussain Z, Ishaq R, Adeel M, Ilyas N. 2020. Effect of salt stress on tomato plant and the role of calcium. J Plant Nutri. 43(1):28–35. doi: 10.1080/01904167.2019.1659324
- Tao J-J, Chen H-W, Ma B, Zhang W-K, Chen S-Y, Zhang J-S. 2015. The role of ethylene in plants under salinity stress. Front Plant Sci. 6:1059. doi: 10.3389/fpls.2015.01059
- Tavakkoli E, Rengasamy P, McDonald GK. 2010. High concentrations of Naþand Cl–ions in soil solution have simultaneous detrimental effects on growth of Faba bean under salinity stress. J Exp Bot. 61(15):4449–4459. doi: 10.1093/jxb/erq251
- Toth G, Adhikari K, Varallyay G, Toth T, Bodis K, Stolbovoy V. 2008. Updated map of salt affected soils in the European Union. In: Teth G, Montanarella L, Rusco E, editors. Threats to soil quality in Europe. EUR 23438 EN - 2008. Luxembourg: European Commission. Joint Research Centre. Institute for Environment and Sustainability; p. 65–77.
- UN-DESA. 2017. World population projected to reach 9.8 billion in 2050, and 11.2 billion in 2100. United Nations department of economic and social affairs. https://www.un.org/development/desa/en/news/population/world-population-prospects-2017.html.
- Verma D, Ansari MW, Agrawal GK, Rakwal R, Shukla A, Tuteja N. 2013. In vitro selection and field responses of somaclonal variant plants of rice cv. PR113 for drought tolerance. Plant Signal Behav. 8:e23519. doi: 10.4161/psb.23519
- Weckx S, Inzé D, Maene L. 2019. Tissue culture of oil palm: finding the balance between mass propagation and somaclonal variation. Front Plant Sci. 10:722. doi: 10.3389/fpls.2019.00722
- Wicke B, Smeets E, Dornburg V, Vashev B, Gaiser T, Turkenburga W, Faaija A. 2011. The global technical and economic potential of bioenergy from salt-affected soils. Energy Environ Sci. 4:2669–2681. doi: 10.1039/C1EE01029H
- Win KT, Fukuyo T, Keiki O, Ohwaki Y. 2018. The ACC deaminase expressing endophyte Pseudomonas spp. Enhances NaCl stress tolerance by reducing stress-related ethylene production, resulting in improved growth, photosynthetic performance, and ionic balance in tomato plants. Plant Physiol Biochem. 127:599–607. doi: 10.1016/j.plaphy.2018.04.038
- Wu M, Kubota C. 2008. Effects of high electrical conductivity of nutrient solution and its application timing on lycopene, chlorophyll and sugar concentrations of hydroponic tomatoes during ripening. Sci Hortic. 116:122–129. doi: 10.1016/j.scienta.2007.11.014
- Xie CG, Lin H, deng XW, Guo Y. 2009. Roles of SCaBP8 in salt stress response. Plant Signal Behav. 4:956–958. doi: 10.4161/psb.4.10.9641
- Xiong L, Lee H, Ishitani M, Zhu JK. 2002. Regulation of osmotic stress-responsive gene expression by the LOS6/ABA1 locus in Arabidopsis. J Biol Chem. 277:8588–8569. doi: 10.1074/jbc.M109275200
- Yang H, Shukla MK, Mao X, Kang S, Du T. 2019. Interactive regimes of reduced irrigation and salt stress depressed tomato water use efficiency at leaf and plant scales by affecting leaf physiology and stem sap flow. Front Plant Sci. 10:160. doi:10.3389/fpls.2019.00160.
- Zhang HX, Blumwald E. 2001. Transgenic salt tolerant tomato plants accumulate salt in the foliage but not in the fruits. Nat Biotechnol. 19:765–768. doi: 10.1038/90824
- Zhu JK. 2002. Salt and drought stress signal transduction in plants. Annu Rev Plant Biol. 53:247–273. doi: 10.1146/annurev.arplant.53.091401.143329
- Zhu JK, Liu J, Xion L. 1998. Genetic analysis of salt tolerance in Arabidopsis: evidence for a critical role of potassium nutrition. Plant Cell. 10:1181–1191. doi: 10.1105/tpc.10.7.1181