ABSTRACT
Sugarcane is a leading biofuel crop besides being a source of crystal sugar and biomaterials worldwide. This study chiefly focuses on the role of diazotrophic bacteria isolated from sugarcane rhizosphere exhibiting different plant growth-promoting traits and biocontrol activities. Several bacterial isolates were obtained from the rhizosphere soil samples of four sugarcane cultivars. Out of these, 23 strains were selected and identified by 16S rRNA gene sequencing which confirmed different PGP-traits, biocontrol, and nitrogenase activities with nifH gene amplification. Two prominent strains, Kosakonia radicincitans-BA1 and Stenotrophomonas maltophilia-COA2 were selected for further analysis. Biolog plate assay was used to establish the utilization of various carbon and nitrogen sources and pH and osmotic stress tolerance for both strains. Colonization of selected strains in the sugarcane plant was observed by scanning electron microscopy and confocal laser scanning microscopy exhibited bacterial cells were frequently located in sugarcane tissues. Inoculation of the strains in GT11 and GXB9 cultivars led to a significant increase in growth parameters, nitrogen content, expression of nifH, and stress-related genes under greenhouse conditions. This is the first study to explore K. radicincitans and S. maltophilia as nitrogen-fixing plant growth-promoting rhizobacteria in sugarcane for disease management and reduce the practice of chemical fertilizers.
Introduction
Sugarcane is an important industrial sugar crop cultivated across the world and accounts for almost 80% of total sugar production worldwide. China is the third-largest sugarcane producer after Brazil and India (FAO Citation2018). The sugar industry contributes a major role in the economic development in Guangxi making up for 65% of the total sugarcane production in China (Li et al. Citation2015). However, production has faced certain obstructions over the years in terms of over-fertilization, as well as abiotic and biotic stresses. Fungal diseases are the main biotic stress during the process of sugarcane growth, causing significant loss of sucrose content and yield (Sundar et al. Citation2012). Sugarcane being a fast-growing biomass crop necessitates a great quantity of nitrogen for growth and development (Singh et al. Citation2020). Therefore, chemical nitrogen fertilizers are used in large amounts to improve sugarcane growth. However, their application has led to significant environmental damages such as soil pollution, leaching, and denitrification (Zhang and Govindaraju Citation2018).
Biological nitrogen fixation (BNF), identified as one of the most important substitutes of the N-fertilizer practice, was confirmed to give about 30%–80% of the total nitrogen in plants (Döbereiner Citation1997; Taulé et al. Citation2012; Santi et al. Citation2013). Several diazotrophic bacteria have been identified from inside or rhizosphere of sugarcane plant can fix nitrogen in sugarcane (Sevilla et al. Citation2001; Oliveira et al. Citation2002; Baldani and Baldani Citation2005; Guo et al. Citation2020). Diazotrophs, which promote growth in sugarcane cultivars, have been isolated and studied in detail on many bacterial genera such as Azospirillum (Sahoo et al. Citation2014), Achromobacter (Silveira et al. Citation2016), Bacillus (Singh et al. Citation2020), Burkholderia (Perin et al. Citation2006), Delftia (Han et al. Citation2005), Herbaspirillum (Baldani et al. Citation1992), Enterobacter (Zhu et al. Citation2013; Guo et al. Citation2020), Gluconacetobacter (Cavalcante and Döbereiner Citation1988), Pseudomonas (Li et al. Citation2017), and Stenotrophomonas (Xing et al. Citation2016). Diazotrophs are also known to possess various plant growth-promoting (PGP) traits which consequently result in improved plant health, growth, and production (Guo et al. Citation2020). Biofertilizers have constituted a major research focus worldwide, hence isolation of diazotrophic plant growth-promoting rhizobacteria (DPGPR) with multi-stress tolerant properties can be used as biofertilizers for sugarcane growth enhancement.
The rhizosphere is characterized as the soil-root surface where multifaceted influences occur among the plant and nearby soil microorganisms (Bulgarelli et al. Citation2012; Robertson-Albertyn et al. Citation2017). It has increased bacterial abundance and diversity, because root exudates provide a broad range of phenolic compounds, thereby attracting and favoring plant-specific microbiota growth (Venturi and Keel Citation2016; Hacquard et al. Citation2017). Rhizobacteria are more effective in nutrient transformation, mobilization, and solubilization, and have a great influence on the nutrient uptake ability of the plants to increasing the efficacy of the useful fertilizers (Hayat et al. Citation2010; Zewide Citation2019). Plant growth-promoting rhizobacteria (PGPR) are naturally present within the rhizosphere associated with many plants. However, plant growth-promoting endophytes (PGPE) are microorganisms that reside inside the plant throughout or part of their life cycle deprived of showing any deceptive damage to the host plant (Dobereiner Citation1992; Wilson Citation1995). Plant growth-promoting rhizobacteria are also capable to colonize inside the plants and can survive as endophytes (Compant et al. Citation2008). Both PGPR/PGPE benefitting the plant in several ways like improving the ability to fix nitrogen, producing phytohormones, 1-amino cyclopropane carboxylate (ACC) deaminase activity, solubilize phosphorus, and siderophore production and encourage antagonism (Rosenblueth and Martínez-Romero Citation2006; Gaiero et al. Citation2013; Lebeis Citation2014; Singh et al. Citation2017; Bharti and Barnawal Citation2018). Inoculation of crops with PGPR also contributes to increased activity of antioxidant enzymes that improve plant immunity (Sahran and Nehra Citation2011). Until now, a large number of rhizobacteria genera have been recognized, and some of these bacterial species have been employed as biofertilizers to improve crop production and to maintain soil fertility (De-Souza et al. Citation2015).
Stenotrophomonas, a genus of gram-negative bacteria, belongs to the family Xanthomonadaceae. In earlier studies, Stenotrophomonas genera were observed to be good phosphate solubilizers and biofertilizers (de-Freitas et al. Citation1997; Suckstorff and Berg Citation2003). S. maltophilia has been reported to be a PGPR with nitrogen-fixing ability in plants other than sugarcane, like peanut (Alexander et al. Citation2019), wheat, maize, and rice (Park et al. Citation2005). K. radicincitans is a PGP gram-negative, rod-shaped bacterium discovered within the new genus Kosakonia of the Enterobacteriaceae family (Krey et al. Citation2013). Several Kosakonia species have been isolated from different plants with the ability to improve plant growth (Berger et al. Citation2018). K. radicincitans (DSMT 16656), isolated from the wheat phyllosphere, is documented for its PGP (Berger et al. Citation2018) and nitrogen-fixing abilities (Remus et al. Citation2000).
This study aims to examine and explore the nitrogen-fixing bacteria isolated from the sugarcane rhizospheric soil in the fields of Guangxi, China, to investigate their PGP-traits and nitrogenase activity, along with biocontrol potential against the sugarcane pathogens Sporisorium scitamineum and Ceratocystis paradoxa. Selected potential diazotrophs were examined for their colonization in sugarcane plant by confocal laser scanning microscopy (CLSM) and scanning electron microscopy (SEM) techniques, and evaluated for various carbon and nitrogen substrates utilization and under stress conditions like pH and osmolytes. The expression of the nifH gene and stress-related genes was determined by qRT-PCR. Assessment of plant growth parameters and nitrogen fixation was done by using the 15N isotope dilution and total N% in two sugarcane cultivars (GT11 and GXB9) after the inoculation of K. radicincitans and S. maltophilia strains under greenhouse condition.
Materials and methods
Collection of rhizosphere soil samples from sugarcane plants
Random rhizosphere soil samples from four sugarcane varieties (GT11, GT29, GXB9, and ROC22) were obtained from the sugarcane fields of the Guangxi University, Nanning, China (latitude 22°49′1.21″ N, and longitude 108°21′59.55″ E). Five healthy sugarcane plants (six months old) were sampled with a sterile auger from different sites in a sterile sample container and instantly transferred to a laboratory. The attached roots soil particles were collected carefully after uprooting plants and assorted well and kept at 4°C for further experiments. Root debris was separated by using a 2 mm mesh. Ten grams of soil sample were mixed in 90 mL of saline water and put in an orbital shaker for 30 min at 100 rpm at 30 ± 2°C.
Media and growth conditions for the isolation of diazotrophs
A soil solution was prepared from the collected samples and spread on an Ashbey’s medium (Hi-Media, India), Yeast Mannitol Agar (Hi-Media, India), LGI, and JNFb medium (Baldani Citation1984; Baldani et al. Citation1992) for the isolation of nitrogen-fixing bacteria. These enrichment media were supplemented with various constituents that specifically promote the growth of nitrogen-fixing bacteria (Table S1). Nutrient Agar (Hi-Media, India) medium was used to study other parameters. 100 µL aliquots suspensions from serial dilutions (10−2–10−5) were spread in all mediums. After 3–5 days of incubation, morphologically different colonies were selected, purified, and stored for further studies.
In vitro screening of plant-growth-promoting attributes
All selected rhizobacteria were screened for their PGP attributes in terms of phosphate (P) solubilization, siderophore, ammonia, hydrogen cyanide (HCN), and indole-3-acetic acid (IAA) production. All tests were performed two times with three replicates per isolate along with control.
Qualitative P-solubilization activity by diazotrophic strains was observed by freshly grown colonies of all the selected strains in an aliquot of 10 μL were dotted on Pikovskaya's agar media (Hi-Media) plate. All plates were kept in an incubator at 30 ± 2°C for 3–5 days and observed for the formation of a clear zone near the bacterial colony (Pikovskaya Citation1948). The test for siderophore production was performed in Chrome Azurol S (CAS) agar medium (Schwyn and Neilands Citation1987). All isolates were spot-inoculated onto CAS agar, kept at 30 ± 2°C for 3 days, positive siderophore-producing rhizobacteria were identified by the presence of an orange halo zone around bacterial colonies on the plates. The ammonia production by diazotrophic strains was carried out by adding 0.5 ml of Nessler reagent. Freshly grown strains were transferred in peptone water tubes and kept at 30 ± 2°C for 2–3 days. After an incubation period that yielded a yellow coloration upon the addition of Nessler's reagent indicated the accumulation of ammonia (Dey et al. Citation2004). Hydrogen cyanide (HCN) production by nitrogen-fixing strains was assessed according to the Lorck method (Citation1948). All selected strains were grown in a nutrient broth medium amended with glycine (4.4 g L−1) in the test tube. A sterile filter paper strip (Whatman No.1) soaked in a picric acid solution (1%) was placed in each test tube (by hanging from the cotton plug), containing bacterial cultures sealed with a parafilm and incubated at 30 ± 2°C for 3–5 days. A change of color from yellow to orange, red, brown, or reddish-brown indicated the HCN producing potential of the bacteria at weak, moderate, or high levels, respectively. The production of Indole-3-acetic acid (IAA) was measured by the colorimetric method described by Glickmann and Dessaux (Citation1995). The pure colony of the selected DPGPR strain was grown for 3 days at 30 ± 2°C in LB broth in the presence and absence of L-tryptophan. After incubation, bacterial cells were removed by centrifugation at 3824 g for 15 min. It was mixed with 2 mL of the supernatant, 2 drops of orthophosphoric acid, and 4 mL Salkowski's reagent, and measured at 530 nm by spectrophotometry (UV-160 A, Shimadzu, Japan)
Test for biocontrol activity against S. scitamineum and C. paradoxa
The isolates selected using the above-mentioned tests were then screened for their antagonistic properties to suppress the growth of fungal pathogens (S. scitamineum and C. paradoxa), commonly affecting sugarcane production. Dual culture assays, using nutrient agar and potato dextrose agar at a 1:1 ratio, were used for screening the bacteria demonstrating antifungal activity. Isolates that suppressed mycelial growth by more than 50% were selected (Singh et al. Citation2013).
Nitrogen fixation assay by acetylene reduction assay (ARA)
The ability of bacteria to fix nitrogen can be determined in vitro by the reduction of acetylene as proposed by Hardy et al. (Citation1968). A freshly grown pure bacterial colony was inoculated on 10 mL semi-solid NFb medium in a tube sealed with serum caps and incubated at 32 ± 2°C for 36–48 h. Then, under the antiseptic conditions, approximately 5% of the air from the incubated tubes was substituted with acetylene gas was injected through the serum caps, and the tube was further re-incubated for 12–16 h. Briefly, 0.5 mL of gas was sucked through a syringe from each tube and infused in a gas chromatograph (GC-17A, Shimadzu, Kyoto, Japan) to measure the amount of ethylene produced in the tube with a flame ionization detector and a column filled with DB-1701. Bradford (Citation1976) method was used to estimate the protein concentration, and the amount of ethylene (C2 H4) produced by each strain was presented as nmol C2H4 produced mg protein−1 h−1.
1-Aminocyclopropane-1-carboxylate (ACC) deaminase activity
Bacterial strains were examined for ACC deaminase activity, based on their ability to utilize ACC as the sole nitrogen source. All isolates were initially grown in 10 mL of Luria–Bertani (LB) broth for 24–36 h and harvested through centrifugation at 10621 g for 5 min. Harvested bacteria were washed with 0.1 M tris-HCL and spotted on modified Dworkin and Foster (DF) medium (Jacobson et al. Citation1994) supplemented with ACC (3 mM) as the sole nitrogen source. Dworkin and Foster agar plates without ACC were maintained as negative controls. ACC deaminase activity was determined by the presence of colonies on the DF plates supplemented with ACC, while quantitative determination was done using the Honma and Shimomura’s (Citation1978) protocol.
DNA extraction, 16S rRNA gene sequencing, and phylogenetic analysis
Identification of the bacterial isolates and subsequent phylogenetic analyses were based on partial 16S rRNA gene sequencing (). By comparing the 16S rRNA sequences with the reference sequences from the National Center for Biotechnology Information (NCBI) GenBank public depository, the phylogenetic and evolutionary relationships were analyzed. While sequence alignment was achieved with ClustalW (Saitou and Nei Citation1987), and Neighbor-Joining method was used for the creation of a phylogenetic tree with MEGA software (version 7.0) (Sneath and Sokal Citation1973; Tamura et al. Citation2004; Kumar et al. Citation2016). Gaps were treated with pairwise deletions, to achieve the confidence values. A pseudoreplication of 1000 was used for bootstrap analysis by the Felsenstein method (Citation1985).
Table 1. List of primers used in this study for 16S rRNA and nifH gene amplification, and expression of stress-related genes.
nifH gene amplification, and sequencing
All selected isolates were also screened for the existence of the nifH gene using the PolF and PolR primers based on the procedure described by Poly et al. (Citation2001) (). nifH gene fragments from DNA templates isolated from selected bacterial strains by PCR were amplified, purified, and cloned (pMD® 18-TVector) consistent with the manufacturer’s (TaKaRa, Japan) directions. The recombinant colonies grown on Luria–Bertani agar medium plates comprising 50 μgmL− 1 ampicillin were recognized through colony PCR and cloned PCR products were sequenced (Sangon Biotech, Shanghai, China).
BIOLOG(R) phenotypic microarray assays
Phenotypic assays of carbon and, nitrogen utilization, osmotic and pH tolerance were conducted using the BIOLOG Phenotype Micro-Array™ GENIII, PM3B, PM9, and PM10 plates. For this study, only the two most potent strains (BA1 and COA2) were selected based on different PGP traits, biocontrol, and nitrogenase activity. GENIII and PM3B plates were employed for assessing carbon and nitrogen use, and the tolerance to osmotic and pH stress was evaluated on the PM9 and PM10 plates. Various compounds were supplemented as the sole sources of carbon and nitrogen while different osmolytes were used to evaluate osmotic stress tolerance. pH tolerance was assessed for pH values between 3.5 and 10. The pure culture of the isolates was grown at 32 ± 2°C on LB broth medium and mixed with an inoculation fluid after washing at the transmittance of 90%–98%. All plates contained 96 wells added 100 mL of above cell suspension and were transferred and incubated at 32 ± 2°C for 48 h to allow the purple color development. After incubation, the readings were obtained using BIOLOG(R) Micro-Station reader according to the directions of the manufacturer. Each analysis was performed in three replicates.
Genetic transformation of BA1 and COA2 strains with green fluorescent protein (GFP)
Green fluorescent protein (GFP) tagging
The selected strains, K. radicincitans (BA1) and S. maltophilia (COA2) were selected for gene tagging with GFP-pPROBE-pTetr-OT plasmid (obtained from College of Agriculture, Guangxi University, Nanning), to study the colonization pattern in sugarcane plants. Using E. coli TG1 as the donor strain, plasmid pPROBE-pTetr-OT was introduced by biparental mating. Briefly, pure culture of selected strains and freshly grown plasmid was transferred in LB broth separately and incubated for 3 days at 30 ± 2°C. After incubation, both are mixed with 1:3 ration and re-incubated again for the same conditions. To check the purity, tagged strains were streaked on LB agar plate. Ex-conjugants that yielded green fluorescence were observed under UV light was confirmed and selected for further study.
Confocal laser scanning microscopy
Sugarcane rooted plantlets were moved to a glass bottle containing 50 ml of the MS liquid medium (saccharose and basal salt mixture) (Reis et al. Citation1998). After, 2–3 days plants were transferred in other bottles containing GFP-tagged bacterial suspension. Plantlets without inoculation of strains have been prepared for control. Plantlets were placed in a growth chamber, raised at 30°C with a 14 h photoperiod at a 60 μmoL m−2 s−1 photon flux density (Lin et al. Citation2012). Sugarcane plantlets were moved three days after inoculation and roots were cleaned with sterile distilled water. Different tissues of sugarcane plantlets were cut into small pieces after the root and stem tissues were mounted discretely on a bridge slide with 10% (v/v) glycerol. The small pieces of root and stem were observed with a Leica DMI 6000 microscope attached to a Leica TCS SP5 laser scanning confocal microscope (Mannheim, Germany). Green fluorescent protein fluorescence was observed using CLSM at an emission length of 500–530 nm, while root autofluorescence was observed based on the intensity of autofluorescence by changing the bandwidth from 600 nm to 800 nm.
Scanning electron microscopy (SEM)
Scanning electron microscopy was used to study the morphology and colonization of both selected strains in sugarcane plants with a scanning electron microscope (Hitachi SU8100). And, the inoculated samples were fixed with a glutaraldehyde solution (Smyth et al. Citation1990).
Evaluation of BA1 and COA2 strains in greenhouse conditions
Plant materials and experimental design
This study was performed on two sugarcane cultivars, GT11 and GXB9, in greenhouse conditions at the Guangxi University, Nanning, China. These cultivars were provided by the Sugarcane Research Institute, Guangxi Academy of Agricultural Sciences, Nanning, China. Sugarcane cultivars, GT11 requires high nitrogen concentration, and GXB9 requires low nitrogen concentration for their growth and development. Before sowing, the sugarcane cane buds were dipped in 45°C hot water for 60–90 min. After 45 days, all plantlets were collected and washed with slow running water to eliminate all soil particles attached to the plant surface and the root system. This study comprised of three groups, (1) control: no bacterial inoculation (inoculation with sterile water), (2) inoculation with K. radicincitans (BA1), and (3) inoculation with S. maltophilia (COA2), and the experiment was designed to be completely randomized with five replicates per treatment for GT11 and GXB9 cultivars. A bacterial suspension was prepared in autoclaved distilled water containing diazotrophic bacterial cells at 107 CFU mL−1 with 1.0% carboxymethyl cellulose solution, and all sugarcane plantlets were placed in the above solution for one hour. After treated plantlets were moved to plastic pots (30 × 40 cm) containing soil and sand mixed at a 1:3 ratio. Each pot held three plantlets. Plantlets were then grown under controlled conditions (30 ± 2°C, >80% ratio, and 16/8 h light/dark cycle) in the greenhouse.
For sampling, leaf and root tissues of both sugarcane varieties were collected at 30, 60, and 90 days after treatments for the analysis of plant growth parameters (plant height, plant weight, root weight, leaf area, and chlorophyll content) and activities of stress-related enzymes i.e. superoxide dismutase (SOD), catalase (CAT), phenylalanine ammonia-lyase (PAL), chitinase (CHI), and β-1, 3-glucanase (GLU). Whereas all gene expression analysis (nifH, SuSOD, SuCAT, SuPAL, SuCHI, and SuGLU) was completed at 30 and 60 days after the inoculation with K. radicincitans (BA1) and S. maltophilia (COA2) strains. Five plants were harvested at 30, 60, and 90 days for all analyses.
Measurement of stress-related enzymes activity
The changes in the activity of five essential enzymes, CHI, GLU, CAT, SOD, and PAL, were measured at 30, 60, and 90 days after K. radicincitans (BA1) and S. maltophilia (COA2) strains inoculation. Leaf and root samples of both varieties were collected as described above. For SOD, CAT, and PAL enzyme analysis, extraction methods were used as earlier described by Singh et al. (Citation2019). The CHI and GLU enzymes were extracted according to Su et al. (Citation2013, Citation2014). As a crude enzyme extract, the extracted supernatants were used to measure enzyme activities, such as SOD (product no. DRE97029), CAT (product no. DRE97028), PAL (product no. DRE97102), CHI (product no. DRE97033), and GLU (product no. DRE97287), by enzyme-linked immune sorbent assays (ELISA) kit (Wuhan Colorful Gene Biological Technology Co. Ltd, China) as per manufacture’s procedure.
Gene expression of nifH and defense-related enzymes
The expression of nifH and stress-related (SuSOD, SuCAT, SuPAL, and SuChi, SuGlu) genes was studied at 30 and 60 days after the treatment of K. radicincitans (BA1) and S. maltophilia (COA2) strains. Foliate tissue samples of control and treated sugarcane varieties (GT11 and GXB9) was used for qRT-PCR analysis. Total RNA was isolated from 100 mg of leaf tissue samples in triplicates with trizol reagent (Tiangen, Beijing), following the manufacturer’s guidelines. To eliminate the DNA impurity of RNA, DNase I (Promega, USA) was used. A Nano photometer (Pearl, Implen-3780, USA) was used to verify the purity and quantity of extracted RNA. One µg of total RNA was used by the Prime-ScriptTM RT Reagent kit (TaKaRa, China) for single-stranded cDNA synthesis. The study was done as per the guidelines described by Singh et al. (Citation2018, Citation2019). , represents the primers used in this analysis, and glyceraldehyde-3-phosphate dehydrogenase (GAPDH) was used as the reference gene. The overall volume of reactions mixture was 20 μL, comprising 0.8 μL of each primer (10 μM), 2 μL of RNA template (10 x diluted cDNA), 10 μL of SYBR Premix Ex TapTM II, and 6.4 μL of ddH2O. Water was used as a template for blank control. The qRT-PCR analysis was carried out with five replicates in a Real-Time PCR detection machine (Bio-Rad, Hercules, California, USA) as followed by the program of Niu et al. (Citation2015) and the specificity of the reaction was determined by the melting curve analysis. Quantification of the relative gene expression was carried out using the 2−ΔΔCt method (Livak and Schmittgen Citation2001).
15N and %N by isotope dilution assay
Biological nitrogen fixation in sugarcane cultivars (GT11 and GXB9) inoculated with K. radicincitans (BA1) and S. maltophilia (COA2) strains were determined by using 15N isotope dilution and natural 15N abundance techniques, where the biologically fixed nitrogen was estimated in correlation with the total nitrogen uptake by Asis et al. (Citation2002). The determination was achieved following the process described by Li et al. (Citation2017). The nitrogen content (root, leaf, and stem) of the dried fine powder of plant tissues was determined by the Institute of Genetics and Physiology, Agriculture, and Forestry Sciences Academy of Hebei, China. Percent 15N was determined by K05 automatic Kjeldahl nitrogen determination equipment (Shanghai Sonnen automated research instrument co. Ltd.), and elementary analysis isotope ratio mass spectrometers (Thermo Fisher DeltaV Advantage IRMS).
Briefly, Soil mixed with sand at a ratio of 1:3 (w/w) was sterilized for 60 min in plastic bags at 121°C, and it allows to cool, and again re-autoclaved. 10 mg ammonium sulfate-15N (10.12 percent atom 15N excess; Shanghai Research Institute of Chemical Laboratory, China) per kg of soil was applied to the soil-sand mixture and homogeneous for two weeks for proper distribution of 15N. 15 kg of 15N soil-sand mixture was transferred in the pots with completely randomized designs. This experiment included three treatments: (1) control: no bacterial inoculation (inoculation with sterile water), (2) inoculation with K. radicincitans (BA1), and (3) inoculation with S. maltophilia (COA2). In each pot, three plants of comparable sizes were transplanted with five replicates per treatment. After six months, the sugarcane plants were picked and cleaned to eliminate the attached soil, and separated into leaf, stem, and roots, and dried at 70°C for 48 h. To make a fine powder of the root, leaf, and stem separately then sieved using a 0.5 mm mesh. The percentage of N in the plant was calculated by the following equation (Urquiaga et al. Citation1992).
Statistical analysis
Biochemical experiments were completed in triplicate with an average of the three readings taken as the final value. Statistical analyses were carried out using ANOVA followed by Duncan's Multiple Range Test (DMRT) for all data generated in this study. Mean values were evaluated for standard errors, and differences at p ≤ 0.05 were regarded as significant. The software Heml 1.0 was used for the construction of the Heatmap illustrator (Deng et al. Citation2014).
Results
Isolation of bacteria from sugarcane rhizosphere
A total of 350 bacterial strains were isolated from the rhizospheric soil samples of four sugarcane cultivars (GT11, GXB9, GT29, and ROC22) using various enrichment media. Out of these, 102 strains were selected based on different morphology and investigated for their nitrogen fixation, PGP traits, and biocontrol activity.
In vitro nitrogen fixation, PGP traits, and biocontrol activity
Among 102 selected bacterial strains, 23 strains demonstrated an ideal PGP potential i.e. IAA production, phosphate solubilization, siderophore production, ACC deaminase activity, nitrogen fixation, HCN, and ammonia production, along with biocontrol activity against sugarcane pathogens ( & ).
Figure 1. Details of various media used for the isolation of selected twenty-three diazotrophic rhizobacteria from sugarcane.
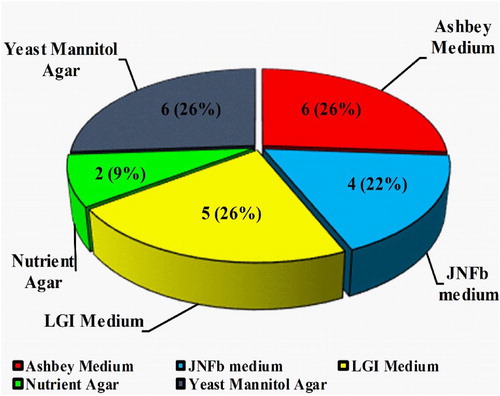
Table 2. Identification of selected 23 diazotrophic strains based on 16S rRNA gene sequencing and their in vitro PGP traits and biocontrol activities against sugarcane pathogens.
Results of all PGP and biocontrol activities are presented in . Out of 23 strains, 19 strains (83%) showed halo zones on the Pikovskaya's agar medium, indicating their ability to solubilize tricalcium phosphate and 5 strains (CON3, AN1, AN2, BA1, and BN9) showed strong activity, whereas siderophore production was confirmed by 18 (78%) strains. In an in vitro test for ammonia production, 16 (70%) strains showed a positive result, while 7 (30%) strains changed the color of the picric acid-saturated Whatman filter paper from yellow to reddish-brown, exhibiting strong HCN production. For ACC deaminase activity, only 16 (70%) strains were able to grow on DF medium enriched with 3 m moL L−1 of ACC ().
When checking for biocontrol ability of the 23 strains against the two sugarcane pathogens, S. scitamineum, and C. paradoxa. Out of the 23 identified strains, 21 demonstrated antagonism, 14 of which were antagonistic against C. paradoxa, and 15 against S. scitamineum; while eight strains showed low to strong antagonistic activity against both pathogens ().
On quantitative estimation, IAA production ranged between 29.20 and 577.17 μg mL−1 in tryptophan added medium and 11.94–39.78 μg mL−1 in the tryptophan-lacking medium. The strain BA1, followed by COA2, showed maximum IAA production in both the absence and presence of tryptophan. Whereas, minimum IAA production was observed by the strain N2 in the presence and by AY4 in the absence of tryptophan in medium (). The quantitative ACC deaminase activity results of all positive strains ranged from 20.58 to 115.26 nmol α-ketobutyrate mg−1 h−1, with COA4 and BA1 showing the maximum and minimum activities, respectively (). However, maximum nitrogenase activity was demonstrated by the strain AN1 (26.86 nmol C2H4 mg protein h-1) followed by BA3, BA1, AN5, and COA2 (26.34, 24.64, 24.56, and 24.44 nmol C2H4 mg protein h−1 respectively) with minimum activity by BN9 and CY8 strains through the ARA method ().
Table 3. In vitro screening of selected bacterial strains for indole-3-acetic acid production, acetylene reduction assay, and 1-aminocyclopropane-1-carboxylic deaminase activity.
Identification and phylogenetic analysis
The selected 23 strains were identified based on partial sequencing for the 16S rRNA gene (). Using the BlastN tool, the sequences were compared with nucleotide sequences in the NCBI GenBank database, and homology of ≥97% was obtained. Species belonging to the genus Kosakonia, Stenotrophomonas, Achromobacter, Azospirillum, Burkholderia, Klebsiella, Rhizobium, Delftia, Pseudomonas, Serratia, and Chryseobacterium were recommended based on the results. Four isolates of Stenotrophomonas maltophilia, as well as different species of Burkholderia and Achromobacter and two isolates of Pseudomonas koreensis, were prominent. The nucleotide sequence data were submitted to the NCBI GenBank depository (MN527307-MN527329). Phylogenetic analysis was carried out post DNA sequence alignment. The evolutionary relationships thus obtained are represented in .
Figure 2. The phylogenetic tree of the 16S rRNA gene of selected diazotrophic rhizobacteria from sugarcane. The evolutionary relationship was inferred with the Neighbor-Joining method. The percentage of replicate trees in which the related taxa clustered together in the bootstrap test (1000 replicates). The evolutionary distances were computed using the Kimura 2-parameter. The analysis involved 46 nucleotide sequences. All positions containing gaps and missing data were removed. Evolutionary analyses were showed using MEGAX.
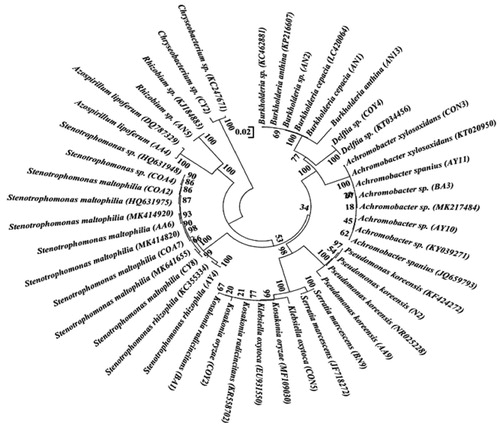
Detection and amplification of the nifH gene
The total genomic DNA extracted from the selected strains was used to amplify the nifH partial sequence PCR products of the appropriate size. Thirteen of the 23 strains were positive for the nifH gene amplification, yielding an end product of 360 bp length (A). Clone libraries for the nifH gene from the positive strains were established and identified using BlastN. It was observed that the sequences were associated with a partial nifH gene with a 93%–100% resemblance, and the NCBI GenBank accession numbers were MN535202- MN535214. The analysis for the nifH phylogenetic tree of the evolutionary relationship is shown in B.
Figure 3. (A) PCR-amplification of the nifH gene from genomic DNA. M; molecular size marker from 100 to 2000 bp. PC; is positive control (Klebsiella verticola) and NC; is a negative control. (B) The phylogenetic tree of nifH gene sequences of amplified diazotrophs strains was constructed by the Neighbor-joining method. Bootstrap values (%) based on 1000 resampled datasets are exposed at branch nodes. The analysis involved 26 nucleotide sequences. Strain Codes: N1, COY2; N2, COY4; N3, CON3; N4, AA4; N5, AN1; N6, AN2; N7, AN5; N8, BA1; N9, BA3; N10, CY2; N11, COA2; N12, COA4; N13, AY4.
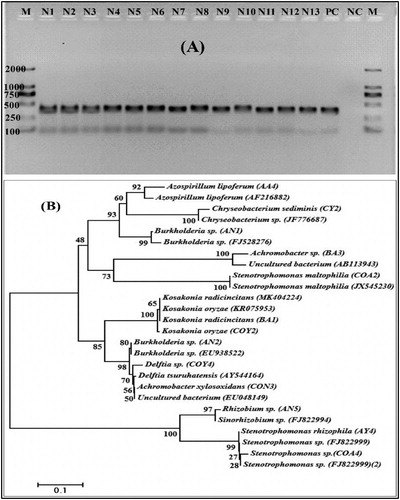
Metabolic profiling of K. radicincitans (BA1) and S. maltophilia (COA2) strains
Based on PGP, biocontrol, and nitrogenase activity results, two isolates K. radicincitans and S. maltophilia were selected for further analysis. Using the BIOLOG system, the metabolic potential of the selected strains was explained based on substrate utilization. The study helped to understand the use of carbon and nitrogen sources and the ability to withstand osmotic and pH stresses. Both the strains displayed a great use of carbon sources, inclusive of sugars, carboxylic acids, and amino acids. BIOLOG phenotype micro-array™ GNIII- carbon plate is divided into 10 groups i.e. carboxylic acids, esters and fatty acids, sugars, chemical sensitivity, acidic pH, sodium chloride, lactic acid, hexose- PO4, amino acid, hexose acid, and reducing sugar. The name and number of different carbon substrates utilized by both bacteria are depicted in & Table S2, showing almost similar substrate utilization patterns between these strains with only slight differences.
Figure 4. Metabolic studies of two most potent nitrogen-fixing K. radicincitans (BA1) and S. maltophilia (COA2) strains using the BIOLOG Phenotype Micro-Array™ plates GNIII-Carbon.
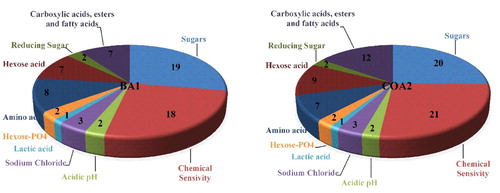
The heatmap is a way of representing the data in every 95 different substrates of three different parameters (nitrogen, pH, and osmolytes) in colored graphical form. The heat map showed relative abundance based on different substrates utilization in each well (total 95 wells). Clustering specifies the similarity of all 95 different substrates between the selected strains, while clustering displayed on the bottom of the figure depicts the similarity between the different Biolog plates ( & Table S3). The two strains were found to utilize amino acids as a desirable nitrogen source. L-amino acids, as sole sources of nitrogen, were used more than the D amino acids and other nitrogen sources, such as urea, thymidine, ammonia, and nitrite at almost similar rates. Combinations of two amino acids were also assessed for metabolic efficacy and it was observed that single, rather than dual amino acids were better utilized. Bacteria also tolerated a slightly acidic pH of 5.5, typical of the sugarcane fields, with S. maltophilia tolerating it better. pH studies in conjunction with amino acids revealed that the microbes could tolerate a pH of 4.5 in the presence of basic and neutral amino acids. Comparable results were observed in terms of osmotic stress tolerance, with S. maltophilia tolerating the stress better than K. radicincitans. Both bacteria demonstrated a moderate to high tolerance to osmotic stress induced by NaCl at 6%, both alone and in the presence of L amino acids, glutathione, and glycerol. Tolerance was also observed to osmotic stress induced by ammonium sulfate, sodium lactate, and sodium nitrate ().
Figure 5. Heatmap graph showing the colored graphical and hierarchical clustering of 95 different substrates utilization (Nitrogen-PM3B), and tolerance (Osmolytes-PM9 and pH-PM10) of K. radicincitans (BA1) and S. maltophilia (COA2) strains. Different colors with relative abundance showing quantitative differences in the growth of both strains.
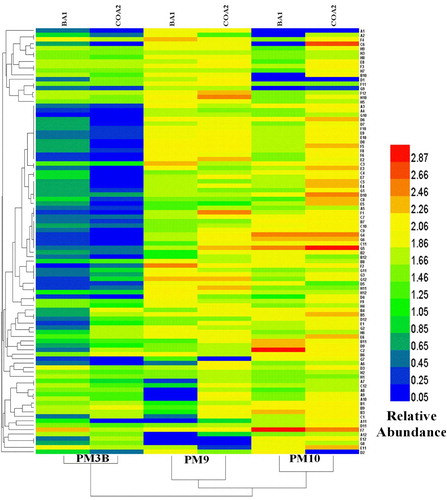
Colonization studies through CLSM and SEM
Morphology and colonization of two selected rhizobacteria K. radicincitans (BA1) and S. maltophilia (COA2) were examined in sugarcane cultivar GT11 with CLSM and SEM techniques. Scanning electron microscopy showing the rod-shaped morphology of both strains (A-B). Green fluorescence cells of both bacteria were detected in root and stem tissues of the sugarcane plant by CLSM and confirmed colonization (E-H & Figure S1). Scanning electron microscopy studies of the root tissues also confirmed the colonization efficiency of both strains in the sugarcane plant (C-D).
Figure 6. Microscopic images showing the morphology and colonization of selected K. radicincitans (BA1) and S. maltophilia (COA2) strains in the sugarcane plant. (A-B) Scanning electron micrographs (SEM) showing rod-shaped morphology of BA1 and COA2 strains, (C-D) SEM showing BA1 and COA2 strains colonization in sugarcane root. (E-F) Confocal laser scanning micrographs (CLSM) showing inoculated GFP tagged BA1 strain colonization as a green dot in root and stem tissues of the sugarcane plant, and (G-H) CLSM showing inoculated GFP tagged COA2 strain colonization as green dots in root and stem tissues of the sugarcane plant.
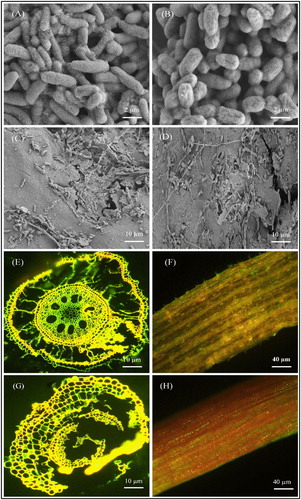
Estimation of stress-related enzymes in sugarcane
K. radicincitans (BA1) and S. maltophilia (COA2) strains increased the activity of SOD, CAT, PAL, CHI, and GLU enzymes in leaf and root tissues of sugarcane varieties (GT11 and GXB9) at 30, 60, and 90 days after inoculation as compared to control (). For SOD, maximum activity (414.6 U g−1 FW) was observed in leaf tissues at 90 days after inoculation of BA1 in GT11 cultivar in autoclaved soil (A-B). For root tissues, the highest SOD activity (1289.6 U g-1 FW) was obtained at 60 days in GXB9 inoculated with BA1 in non-autoclaved soil (A-B). For leaf CAT activity, a maximum increase in CAT activity (116.8 U g−1 FW) was observed in GT11 inoculated BA1 at 90 days in non-autoclaved soil (C-D). For root CAT activity, the maximum value (126.0 U g−1 FW) was observed in GXB9 inoculated with BA1 at 60 days in non-autoclaved soil (C-D). Maximum PAL activity (851.5 U g−1 FW) was observed in leaf (autoclaved soil) and root (890.6 U g−1 FW) (non- autoclaved soil) tissues of GXB9 cultivar inoculated BA1 at 60 days (E-F). Chitinase activity was highest in GXB9 cultivar at 30 days in the case of both leaf (1315.9 U g−1 FW) (non- autoclaved) and root (1575.9 U g−1 FW) (autoclaved) tissues after inoculation of strain BA1 (G-H). Whereas, GLU leaf activity (2957.5 U g−1 FW) was also elevated at 30 days and root (2574.7 U g−1 FW) GLU activity at 90 days after the inoculation of BA1 in GXB9 in autoclaved soil (I-J). While enzymes activity results suggested that the microbial strains were more effective in root tissues as compared to the leaf, and it also indicates that BA1 exerts a significant effect as compared to COA2 on the sugarcane cultivars.
Figure 7. Enzyme estimation in leaf and root tissues of two sugarcane cultivars GT11 and GXB9 inoculated with K. radicincitans (BA1) and S. maltophilia (COA2). (A) Superoxide dismutase, (B) Catalase, (C) Phenylalanine ammonia-lyase, (D) Chitinase, and (E) β-1-3-glucanase. All data points are the mean ± SE (n = 3). Different letters above the bar show a significant difference at p < 0.05.
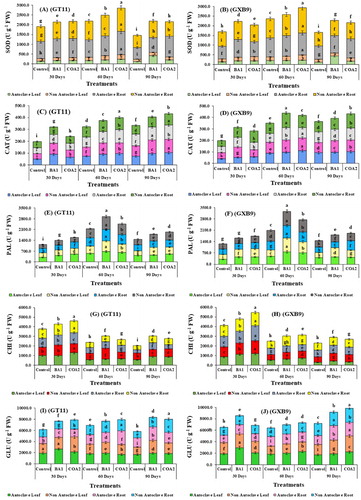
Expression of stress-related genes by qRT-PCR
Leaf tissues of the two sugarcane cultivars were used to study the expression of stress-related genes (SuSOD, SuCAT, SuPAL, SuCHI, and SuGLU) after inoculation with BA1 and COA2 strains using qRT-PCR. The results displayed a significant change in the expression levels in both the cultivars. The genes were expressed at a higher rate with the microbial inoculum, with expression levels highest for SuCHI for both cultivars, at 30 and 60 days, except for GT11 at 60 days (A-D). A reduction in the expression for SuCAT, SuSOD, and SuGLU was observed at 30 as compared to day 60 in the GT11 cultivar, while at the same time points, a significant increase was observed for SuPAL (A-B). Further, the expression of SuCHI remained constant. In GXB9 at 60 days, the gene expression of SuCAT, SuPAL, and SuCHI enzymes decreased as compared to day 30, while remaining constant for SuSOD and SuGLU (C-D). The SuCHI gene expression in GT11 and GXB9 increased during 30-days and 60-days planting, in both sugarcane cultivars (A-D).
Figure 8. qRT-PCR analysis of differentially expressed genes in leaf tissues of sugarcane cultivars (GT11 and GXB9) inoculated with K. radicincitans (BA1) and S. maltophilia (COA2). Data were standardized to the GAPDH expression level. All data points are the mean ± SE (n = 3). Different letters above the bar show a significant difference at p < 0.05.
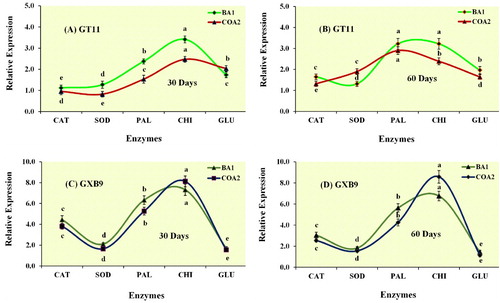
nifH gene expression
The two cultivars GT11 and GXB9, inoculated with the two isolated strains BA1 and COA2, were examined for the nifH gene expression at 30 and 60 days (A-B). Positive expression of the nifH gene was detected with qRT-PCR using the total RNA extracted from the foliate samples of the cultivars. After 30 days, the nifH gene expression was observed to be highest in GXB9 inoculated with BA1 and COA2 strains as compared to GT11, with the same pattern also observed at 60 days. Overall, BA1 showed more nifH gene expression in both cultivars as compared to the COA2 strain.
Figure 9. The expression of the nifH gene in two sugarcane cultivars GT11 and GXB9 with K. radicincitans (BA1) and S. maltophilia (COA2) strains inoculation by qRT-PCR. Data were normalized to the GAPDH expression level. All data points are the means ± SE (n = 3). Different letters above the bar show a significant difference at p < 0.05.
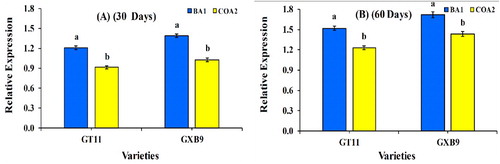
Determination of nitrogen fixation using the 15n isotope dilution technique
Nitrogen uptake and its concentration in the leaf, stem, and root tissues were determined using the 15N isotope dilution technique. Taking the average totals, the N-content showed an increase of 22%, 30%, and 25% in the GT11 cultivar inoculated with BA1 and a 24%, 28%, and 21% for the COA2 strain in the leaf, root, and stem tissues respectively (A). Whereas, the percentage of total N content in leaf, root, and stem tissues of GXB9 increased to 17%, 27%, and 11% when inoculated with BA1 and 15%, 20%, and 17% when inoculated with COA2 respectively, compared to the control (B).
Figure 10. Estimation of nitrogen fixation in sugarcane cultivars (GT11 and GXB9) inoculated with K. radicincitans (BA1) and S. maltophilia (COA2) diazotrophs using 15N abundance and 15N dilution methods. The columns represent the mean values ± SE (n = 3) of the data for each treatment. Different letters above the bar indicate a significant difference at p < 0.05.
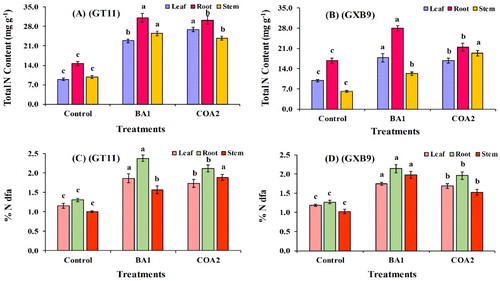
Additionally, nitrogen derived from the atmosphere was determined for the cultivars using 15N labeled ammonium sulfate-standard nitrogen fertilizer commonly used for agricultural purposes. Compared to the control, the 15N concentrations were higher among the cultivars grown under laboratory conditions with the bacterial inoculums. Greenhouse studies revealed a superior BNF compared to the control in the sugarcane cultivars. The GT11 cultivar demonstrated a higher nitrogen uptake with BA1 than COA2 inoculum in leaf and root tissues, while for COA2 inoculum nitrogen uptake was increased in the stem. The root showed a higher percentage of nitrogen uptake when inoculated with both strains (C). The GXB9 cultivar showed a higher uptake when inoculated with BA1 and COA2 as compared to the control. Similar to GT11, BA1 demonstrated a higher nitrogen fixation than COA2 in the root than in the stem and leaf samples. In this cultivar, the leaf sample showed a similar result with both strains. Therefore, both strains (BA1 and COA2) were observed to fix nitrogen on a higher scale than the control (D).
Physiological parameters
A strong influence of the K. radicincitans and S. maltophilia strains was observed in the sugarcane cultivars grown with autoclaved and non-autoclaved soil. All growth parameters were investigated at different time intervals (30, 60, and 90 days), presented in . The results of chlorophyll content were noted in special products analysis division (SPAD) units at all different time points. Both sugarcane cultivars inoculated with the strains showed improvement in plant height, root weight, plant weight, leaf surface area, and chlorophyll contents at 30, 60, and 90-days days in both types of soil as compared to control. Overall, K. radicincitans showed a better result as compared to S. maltophilia. (). These results suggest that these strains are effective in both autoclaved and non-autoclaved soil, and can be used as a biofertilizer for sugarcane growth enhancement.
Table 4. The effect of K. radicincitans (BA1) and S. maltophilia (COA2) diazotrophs inoculation on the growth of sugarcane cultivars (GT11 and GXB9) under greenhouse condition.
Discussion
The harmful effects of chemical fertilizers have led to the development of alternative, environmentally friendly biotechnology tools to be implemented globally in sustainable agricultural practices (Dobbelaere et al. Citation2003). Rhizosphere soil bacteria have been recognized for their ability to fix nitrogen, various PGP attributes, as well as the biocontrol activity that inhibits host plant pathogens and improves plant resistance towards various environmental stresses recommending them to be used as potential biofertilizers (Somers et al. Citation2004; Kang et al. Citation2007; Bhattacharyya and Jha Citation2012; Carvalho et al. Citation2014; Rafikova et al. Citation2016; Benito et al. Citation2017). In this study, diazotrophs from the sugarcane rhizosphere soil were isolated, identified, and examined for their ability to fix nitrogen and to promote plant growth with their attributes. Among all, BA1 and COA2 bacterial isolates, identified as K. radicincitans and S. maltophilia, respectively, were found to have a better influence on the sugarcane cultivars for growth promotion, in comparison with other isolates.
Phosphate solubilization and IAA production are considered vital PGP traits (Zeng et al. Citation2016; Verma et al. Citation2017). Phosphorous is an important nutrient that promotes plant growth but the phosphorus in soil is not readily available for plants (Wang et al. Citation2009). Microorganisms are known to solubilize phosphorous into a readily available form for the plants and reduce the need for phosphate fertilizers (Lavakusha et al. Citation2014). In the current study, the potential candidates K. radicincitans and S. maltophilia showed a high to moderate ability to solubilize phosphate. Microbial IAA production allows the rhizobacteria to overcome the host defense mechanisms while simultaneously promoting plant growth via cell enlargement and root initiation (Cherif-Silini et al. Citation2016). It also acts as a signal for colonization in the host plant, and IAA-producing bacteria can colonize better with the host plant than non-producers (Etesami et al. Citation2015). Of the identified isolates, K. radicincitans demonstrated significant IAA production over other isolates. The ability of IAA production indicates the potential application of these isolates as growth regulators. Another, important PGP trait is siderophore production by PGPR, which is used as iron sources by the host plants and improved plant growth by increasing the accessibility of Fe adjacent to the root. Siderophore-producing bacteria also act as biocontrol agents by limiting the growth of plant pathogens (Schwyn and Neilands Citation1987; Arora et al. Citation2013). Of the isolated bacteria, 78% demonstrated low to high levels of siderophore production.
HCN is a volatile, secondary metabolite produced by several PGPR. HCN production by PGPR is used as a biocontrol mechanism to protect plants from a fungal pathogen’s attacks (Olanrewaju et al. Citation2017). Based on several past studies PGPR strains, namely Rhizobium, Pseudomonas, Alcaligenes, Bacillus, and Aeromonas, have shown HCN production (Ahmad et al. Citation2008; Singh et al. Citation2013, Citation2020; Das et al. Citation2017; Li et al. Citation2017). In this study, strains which tested positive for HCN production also plays an important role against sugarcane pathogens. Ammonia production by PGPR accomplishes numerous biological roles such as suppress the growth of plant pathogens, prevent infection, and disease manifestations (Kumar and Singh Citation2001; Cakmak et al. Citation2010). This ability has been successfully demonstrated among 70% of the isolated strains suggesting their potential use as biocontrol agents. The isolates were screened for their antagonistic potential against S. scitamineum and C. paradoxa pathogens cause smut and pineapple diseases of sugarcane and reducing sugarcane yield and production (Viswanathan and Rao Citation2011). Here, 35% of the isolates showed antagonism against both C. paradoxa and S. scitamineum, with a high activity observed with K. radicincitans. A similar study also reported Pseudomonas, Bacillus, and Enterobacter strains isolated from the sugarcane rhizosphere showed biocontrol activity against S. scitamineum and C. paradoxa pathogens (Li et al. Citation2017; Guo et al. Citation2020; Singh et al. Citation2020).
Rhizobacteria that can synthesize ACC deaminase, use ACC as a nitrogen source, prevent the production of ethylene, and help the cultivars withstand stress (Sarkar et al. Citation2018). Apart from imparting stress tolerance, ACC deaminase encourages the growth of roots in the developing young saplings of sugarcane cultivars. Hence, the development of a healthier root system results in the development of a healthy shoot system (Zahir et al. Citation2003; Glick et al. Citation2007). Synthesis of ACC deaminase, however, is substrate-dependent (Sarkar et al. Citation2018). Based on the observations from bacterial growth inoculated on medium added with ACC as the sole nitrogen source an isolate identified as a member of the genus Bacillus, Pseudomonas, Enterobacter demonstrated high ACC deaminase activity while the others showed moderate activity (Li et al. Citation2017; Guo et al. Citation2020; Singh et al. Citation2020).
Elucidating the phenotypic profile allows for a comprehensive understanding of the variations in genotypes, stress responses, nutrient requirements, and environmental conditions for a microorganism (Mazur et al. Citation2013). Assessing each entity of a substrate group will aid in establishing the correlation of the metabolite and utilization potential of the strain. From this study, it can be interpreted that the strains could utilize a number of substrates, with K. radicincitans and S. maltophilia demonstrating a diversified metabolism. This means that these bacteria have a better chance of colonizing the root than other bacteria. However, it was also noted that the presence of amino acids influenced the utilization efficacy of the microbes (Chelius and Triplett Citation2000; Wielbo et al. Citation2007; Chojniak et al. Citation2015). Plant roots represent the most general way of entrance of endophytic bacteria into their host plant and created close and safe relations among bacteria and their host plants (Timmusk et al. Citation2005; Passari et al. Citation2015). This is the key difference between endophytes and rhizobacteria (Belbahri et al. Citation2017). Therefore, in this study, both selected DPGPR strains were genetically tagged with a GFP-pPROBE-pTetr-OT to understand the effect elicited by them on the sugarcane cultivars GT11 and GXB9. The level of colonization in the different plant components was also examined through CLSM (Lin et al. Citation2012; Jooste et al. Citation2019; Guo et al. Citation2020). These tools can also be effectively applied to determine a potent strain from isolates, or in identifying a better colonizer among different plant-associated bacteria. In this study, separate inoculations of plantlets with the individual strains in different soils (autoclave and non-autoclave) showed colonization in all the plant parts. Microscopy studies concluded that both microbes colonized in sugarcane efficiently like endophytes.
The ability of all isolated strains to reduce acetylene was observed from the ARA, suggesting that nitrogen-fixing rhizobacteria inhabit the sugarcane rhizosphere soil, niches that could potentially be applied to augment the nitrogen concentrations in soil in a readily available form. Several bacteria have been reported for their association with nitrogen fixation in sugarcane fields (Sevilla et al. Citation2001; Loiret et al. Citation2004; Baldani and Baldani Citation2005; Mehnaz et al. Citation2010; Lin et al. Citation2012). The same has also been observed in this study. Molecular studies on the selected isolates were conducted to determine the presence of nifH genes. Contrary to the result for the ARA, where all strains showed nitrogen-fixing capability, the nifH gene was detected in only 56% of the isolated strains. Characterization and amplification of nifH genes using degenerate primers is the primary and vital tool in determining the nitrogen fixation potential in bacteria (Zehr and Capone Citation1996; Rosado et al. Citation1998). Variations in the nucleotide sequences of the nifH gene may be present both between species and within the species, which could be the reason for the absence of amplification of the functional gene in other isolates. Studies have suggested that the absence of amplification does not suggest that the isolates do not have a nitrogen-fixing potential (Zehr et al. Citation2003).
Quantitative RT–PCR studies are sensitive and specific in the detection of mRNA and hence have been successfully exploited in studies of the nifH gene expression (Noda et al. Citation1999; Brown et al. Citation2003). The presence of the nifH gene is indicative of biological nitrogen fixation (Akter et al. Citation2014). Enterobacteriaceae and Xanthomonadaceae members have been reported for their efficacy at nitrogen fixation, however, systematic studies for the same are few. In the current study, the selected strains have shown significant nifH gene expression. After 30 days the expression was observed to be highest in GT11 variety inoculated with K. radicincitans and S. maltophilia strains. Both the 15N isotope and N balance studies have demonstrated that the Brazilian sugarcane cultivars could obtain over 30%–80% of nitrogen through BNF (Taulé et al. Citation2012; Urquiaga et al. Citation2012). Colonization of the bacteria in the roots, and inhabitation in the host as endophytes, have been attributed to this improvement (Boddey et al. Citation1995). It has also been observed that wild-type strains were effective at BNF, and sugarcane cultivars obtained their nitrogen from the atmosphere through the association of rhizobacteria (Sevilla et al. Citation2001). BNF quantification studies were carried out using the 15N isotope dilution method. While the quantification precision in this method is low, the results obtained were found to be more consistent in comparison with ARA (Montañez et al. Citation2009). We also observed that both strains were able to fix nitrogen in both the sugarcane cultivars, GT11, and GXB9.
Antioxidant enzymes are produced by plants under stress imparted by the reactive oxygen species. Interaction between PGPR and host plants has also been observed to increase the biosynthesis of defense-related molecules under normal conditions that can defend against further attacks by pathogens or other interactions between plant microbes (Cassán et al. Citation2014; Fukami et al. Citation2017; Bhattacharyya et al. Citation2020). The effect on the expression of these enzymes in the plantlets inoculated with the GFP tagged strains was assessed, resulting in enhanced expression of the antioxidant enzymes inoculated with the selected strains as compared to the controls. Antioxidant enzymes such as CAT, POD, SOD, CHI, and GLU, synthesized by the plants, reduced SOD into water and oxygen thereby enabling the plants to tolerate reactive oxygen species (ROS) induced stress (Bharti et al. Citation2016; Sarkar et al. Citation2018). SOD followed by CAT acts as the primary defense in oxidative stress where SOD converts the superoxide (O2-) into H2O2, which later is decomposed into water and oxygen by CAT. Variations in gene expression and physiology are indicative of mutual symbiosis of the host and microorganism (Brown et al. Citation2003; Paungfoo-Lonhienne et al. Citation2016). K. radicincitans (BA1) and S. maltophilia (COA2) inoculated sugarcane cultivars demonstrated an improved expression and augmented activity of the antioxidant enzymes.
Conclusions
To the best of our knowledge, this is the first systematic report on K. radicincitans (BA1) and S. maltophilia (COA2), as nitrogen-fixing PGPR associated with sugarcane. Both strains possessed important PGP attributes including antagonism against sugarcane pathogens, effective colonization, survival under various stresses as well as confirmed nitrogen fixation and defense-related gene expression in two sugarcane cultivars (GT11 and GXB9) under greenhouse conditions. It can be concluded that both strains have the potential to be applied as biofertilizers for sustainable agricultural practices for sugarcane cultivars and other crops, to improve environmental conditions and the state of the bio-agri industry. Further studies, in this field, could help ratify our findings.
Supplemental Material
Download MS Word (334 KB)Disclosure statement
No potential conflict of interest was reported by the author(s).
Additional information
Funding
Notes on contributors
Rajesh Kumar Singh
Rajesh Kumar Singh is a Postdoctoral researcher in Sugarcane Research Institute, Guangxi Academy of Agricultural Sciences, Nanning, Guangxi, China.
Pratiksha Singh
Pratiksha Singh is a Postdoctoral researcher in Sugarcane Research Institute, Guangxi Academy of Agricultural Sciences, Nanning, Guangxi, China.
Hai-Bi Li
Hai-Bi Li is a Research Assistant in Guangxi South Subtropical Agriculture Science Research Institute, Longzhou, Guangxi, China.
Dao-Jun Guo
Dao-Jun Guo is a Ph.D. student in College of Agriculture, Guangxi University, Nanning, China.
Qi-Qi Song
Qi-Qi Song is a Research Assistant in Guangxi Subtropical Crop Research Institute, Sugarcane Research Institute, Nanning, Guangxi–530001, China.
Li-Tao Yang
Li-Tao Yang is a former Professor in College of Agriculture, Guangxi University, Nanning, China.
Mukesh Kumar Malviya
Mukesh Kumar Malviya is a Postdoctoral researcher in Sugarcane Research Institute, Guangxi Academy of Agricultural Sciences, Nanning, Guangxi, China.
Xiu-Peng Song
Xiu-Peng Song is a Research Associate in Guangxi Key Laboratory of Crop Genetic Improvement and Biotechnology, Nanning, Guangxi, China.
Yang-Rui Li
Yang-Rui Li is a Professor and Head of Guangxi Key Laboratory of Sugarcane Genetic Improvement, Sugarcane Research Institute, Guangxi Academy of Agricultural Sciences, Nanning, Guangxi, China.
Notes
(+) = low activity; (++) = moderate activity; (+++) =strong activity; (-) = no activity. Phosphate and siderophore activity of the bacterial isolates was observed by 3 mm or greater clear zone of inhibition on suitable medium after 3–5 days. A; S. scitamineum, B; C. paradoxa. Antifungal activity was measured as a zone of inhibition after 3–5 days of incubation at 26 ± 2°C.
P-Tryptophan: Presence of tryptophan; A-Tryptophan: Absence of tryptophan. Means followed by the same letter within a row are not significantly different (P ≤ 0.05) according to Duncan’s Multiple Range Test.
Means followed by the same letter within a row are not significantly different (P ≤ 0.05) according to Duncan’s Multiple Range Test.
References
- Ahmad F, Ahmad I, Khan MS. 2008. Screening of free-living rhizospheric bacteria for their multiple plant growth promoting activities. Microbiol Res. 163:173–181.
- Akter Z, Pageni BB, Lupwayi NZ, Balasubramanian PM. 2014. Biological nitrogen fixation and nifH gene expression in dry beans Phaseolus vulgaris L. Can J Plant Sci. 94:203–212.
- Alexander A, Singh VK, Mishra A, Jha B. 2019. Plant growth promoting rhizobacterium Stenotrophomonas maltophilia BJ01 augments endurance against N2 starvation by modulating physiology and biochemical activities of Arachis hypogea. PLoS ONE. 149:e0222405.
- Arora NK, Tewari S, Singh R. 2013. Multifaceted plant-associated microbes and their mechanisms diminish the concept of direct and indirect PGPRs. In: NK Arora, editor. Plant microbe symbiosis; Fundamentals and advances. Springer; p. 411–449.
- Asis CA, Kubota M, Ohta H, Arima Y, Ohwaki Y, Yoneyama T, Tsuchiya K, Hayashi N, Nakanishi Y, Akao S. 2002. Estimation of the nitrogen fixation by sugarcane cultivar NiF-8 using 15N dilution and natural 15N abundance techniques. J Soil Sci Plant Nutr. 48(2):283–285.
- Baldani JI. 1984. Ocorrênciae caracterização de Azospirillum amazonense em comparação com outras espécies deste gênero em raízes de milho sorgo e arroz, Federal Rural University of Rio de Janeiro Seropédica RJ Brazil. MSc Thesis.
- Baldani JI, Baldani VLD. 2005. History on the biological nitrogen fixation research in graminaceous plants: special emphasis on the Brazilian experience. An Acad Bras Cienc. 77:549–579.
- Baldani VLD, Baldani JI, Olivares FL, Döbereiner J. 1992. Identification and ecology of Herbaspirillum seropedicae and the closely related Pseudomonas rubrisubalbicans. Symbiosis. 13:65–73.
- Belbahri L, Chenari Bouket A, Rekik I, Alenezi FN, Vallat A, Luptakova L, Petrovova E, Oszako T, Cherrad S, Vacher S, Rateb ME. 2017. Comparative genomics of Bacillus amyloliquefaciens strains reveals a core genome with traits for habitat adaptation and a secondary metabolites rich accessory genome. Front Microbiol. 8:1438.
- Benito P, Alonso-Vega P, Aguado C, Luján R, Anzai Y, Hirsch AM, Trujillo ME. 2017. Monitoring the colonization and infection of legume nodules by Micromonospora in co-inoculation experiments with rhizobia. Sci Rep. 7:11051.
- Berger B, Patz S, Ruppel S, Dietel K, Faetke S, Junge H, Becker M. 2018. Successful formulation and application of plant growth-promoting Kosakonia radicincitans in maize cultivation. Biomed Res Int. 6439481:8. doi:10.1155/2018/6439481.
- Bharti N, Barnawal D. 2018. Amelioration of salinity stress by PGPR: ACC deaminase and ROS scavenging enzymes activity. In: Singh AK, Kumar A, Singh PK, editors. PGPR Amelioration in sustainable Agriculture: Food Security and environmental management. Woodhead Publishing; p. 85–106.
- Bharti N, Pandey SS, Barnawal D, Patel VK, Kalra A. 2016. Plant growth promoting rhizobacteria Dietzia natronolimnaea modulates the expression of stress responsive genes providing protection of wheat from salinity stress. Sci Rep. 6:34768.
- Bhattacharyya C, Banerjee S, Acharya U, Mitra A, Mallick I, Halder A, Halder S, Ghosh A, Ghosh A. 2020. Evaluation of plant growth promotion properties and induction of antioxidative defense mechanism by tea rhizobacteria of Darjeeling India. Sci Rep. 10:15536. doi:10.1038/s41598-020-72439-z.
- Bhattacharyya PN, Jha DK. 2012. Plant growth-promoting rhizobacteria (PGPR): emergence in agriculture. World J Microbiol Biotechnol. 28:1327–1350.
- Boddey RM, de Oliveira OC, Urquiaga S, Reis VM, de Olivares EL, Baldani VLD, Dobereiner J. 1995. Biological nitrogen fixation associated with sugar cane and rice: Contributions and prospects for improvement. Plant Soil. 174:195–209.
- Bradford MM. 1976. A rapid and sensitive method for the quantitation of microgram quantities of protein utilizing the principle of protein-dye binding. Anal Biochem. 72:255-260.
- Brown MM, Friez MJ, Lovell CR. 2003. Expression of nifH genes by diazotrophic bacteria in the rhizosphere of short form Spartina alterniflora. FEMS Microbiol Ecol. 43:411–417.
- Bulgarelli D, Rott M, Schlaeppi K, Ver Loren van Themaat E, Ahmadinejad N, Assenza F, Rauf P, Huettel B, Reinhardt R, Schmelzer E, et al. 2012. Revealing structure and assembly cues for Arabidopsis root-inhabiting bacterial microbiota. Nature. 488(2):91–95.
- Cakmak I, Pfeiffer WH, Clafferty BM. 2010. Biofortification of durum wheat with zinc and iron. Cereal Chem. 87(1):10–20.
- Carvalho TLG, Balsemao-Pires E, Saraiva RM, Ferreira SG, Hemerly AS. 2014. Nitrogen signalling in plant interactions with associative and endophytic diazotrophic bacteria. J Exp Bot. 65:5631–5642. doi:10.1093/jxb/eru319.
- Cassán F, Vanderleyden J, Spaepen S. 2014. Physiological and agronomical aspects of phytohormone production by model plant-growth-promoting rhizobacteria PGPR belonging to the genus Azospirillum. J Plant Growth Regul. 33:440–459.
- Cavalcante VA, Döbereiner J. 1988. A new acid-tolerant nitrogen-fixing bacterium associated with sugarcane. Plant Soil. 108:23–31.
- Chelius MK, Triplett EW. 2000. Immuno localization of dinitrogenase reductase produced by Klebsiella pneumoniae in association with Zea mays L. Appl Environ Microbiol. 66:783–787.
- Chen HJ, Wu SD, Huang GJ, Shen CY, Afiyanti M, Li WJ, Lin YH. 2012. Expression of a cloned sweet potato catalase SPCAT1 alleviates ethephon-mediated leaf senescence and H2O2 elevation. J Plant Physiol. 169:86–97.
- Cherif-Silini H, Silini A, Yahiaoui H, Ouzari I, Boudabous A. 2016. A phylogenetic and plant-growth-promoting characteristic of Bacillus isolated from the wheat rhizosphere. Ann Microbio. 66:1087–1097.
- Chojniak J, Wasilkowski D, Płaza G, Mrozik A, Brigmon R. 2015. Application of biolog microarrays techniques for characterization of functional diversity of microbial community in phenolic-contaminated water. Int J Environ Res. 9:785–794.
- Compant S, Kaplan H, Sessitsch A, Nowak J, Ait Barka E, Clément C. 2008. Endophytic colonization of Vitis vinifera L, by Burkholderia phytofirmans strain PsJN: from the rhizosphere to in florescence tissues. FEMS Microbiol Ecol. 63(1):84–93.
- Das K, Prasanna R, Saxena AK. 2017. Rhizobia: a potential biocontrol agent for soilborne fungal pathogens. Folia Microbiol. 62(5):425–435.
- de-Freitas JR, Banerjee MR, Germida JJ. 1997. Phosphate solubilizing rhizobacteria enhance the growth and yield but not phosphorus uptake of Canola Brassica napus L. Biol Fert Soils. 24:358–364.
- Deng W, Wang Y, Liu Z, Cheng H, Xue Y. 2014. Hemi: a toolkit for illustrating heatmaps. PLoS ONE. 9(11):e111988.
- De-Souza R, Ambrosini A, Passaglia LMP. 2015. Plant growth-promoting bacteria as inoculants in agricultural soils. Genet Mol Biol. 38:401–419. doi:10.1590/S1415-475738420150053.
- Dey R, Pal K K, Bhatt D M, Chauhan S M. 2004. Growth promotion and yield enhancement of peanut Arachis hypogaea L, by application of plant growth promoting rhizobacteria. Microbiol Res. 159:371–394.
- Dobbelaere S, Vanderleyden J, Okon Y. 2003. Plant growth promoting effects of diazotrophs in the rhizosphere. Crit Rev Plant Sci. 22:107–149.
- Dobereiner J. 1992. History and new perspectives of diazotrophs in association with non-leguminous plants. Symbiosis. 13:1–13.
- Döbereiner J. 1997. Biological nitrogen fixation in the tropics: social and economic contributions. Soil Biol Biochem. 29(5-6):771–774.
- Edwards U, Rogall TH, Blocker H, Emde M, Bottger EC. 1989. Isolation and direct complete nucleotide determination of entire genes, characterization of a gene coding for 16S ribosomal RNA. Nucleic Acids Res. 17:7843–7853.
- Etesami H, Ali HA, Hosseini HM. 2015. Indole-3-acetic acid IAA production trait a useful screening to select endophytic and rhizosphere competent bacteria for rice growth promoting agents. MethodsX. 2:72–78.
- FAO. 2018. Top sugarcane production: Food and Agriculture Organization of the United Nations Rome: FAO.
- Felsenstein J. 1985. Confidence limits on phylogenies: an approach using the bootstrap. Evolution. 39:783–791.
- Fukami J, Ollero F J, Megías M, Hungria M. 2017. Phytohormones and induction of plant-stress tolerance and defense genes by seed and foliar inoculation with Azospirillum brasilense cells and metabolites promote maize growth. AMB Express. 7(1):153.
- Gaiero JR, McCall CA, Thompson KA, Day NJ, Best AS, Dunfield KE. 2013. Inside the root microbiome: bacterial root endophytes and plant growth promotion. Am J Bot. 100:1738–1750.
- Glick BR, Todorovic B, Czarny J, Cheng Z, Duan J, McConkey B. 2007. Promotion of plant growth by bacterial ACC deaminase. Crit Rev Plant Sci. 26:227–242.
- Glickmann E, Dessaux Y. 1995. A critical examination of the specificity of the Salkowski reagent for indolic compounds produced by phytopathogenic bacteria. Appl Environ Microbiol. 61:793–796.
- Guo D-J, Singh RK, Singh P, Li D-P, Sharma A, Xing Y-X, Song X-P, Yang L-T, Li Y-R. 2020. Complete genome sequence of Enterobacter roggenkampii ed5 a nitrogen fixing plant growth promoting endophytic bacterium with biocontrol and stress tolerance properties isolated from sugarcane root. Front Microbiol. 11:580081. doi:10.3389/fmicb.2020.580081.
- Hacquard S, Spaepen S, Garrido-Oter R, Schulze-Lefert P. 2017. Interplay between innate immunity and the plant microbiota. Annu Rev Phytopathol. 55(1):565–589.
- Han J, Sun L, Dong X, Cai Z, Sun X, Yang H, Wang Y, Song W. 2005. Characterization of a novel plant growth-promoting bacteria strain Delftia tsuruhatensis HR4 both as a diazotroph and a potential biocontrol agent against various plant pathogens. Syst Appl Microbiol. 28(1):66–76.
- Hardy RWF, Holsten RD, Jackson EK, Burns RC. 1968. The acetylene ethylene assay for N2 fixation: laboratory and field evaluation. Plant Physiol. 43:1185–1207.
- Hayat R, Ali S, Amara U, Khalid R, Ahmed I. 2010. Soil beneficial bacteria and their role in plant growth promotion: a review. Ann Microbiol. 60:579–598.
- Honma M, Shimomura T. 1978. Metabolism of 1-aminocyclopropane-1-carboxylic acid. Agric Biol Chem. 42:1825–1831.
- Jacobson CB, Pasternak JJ, Glick BR. 1994. Partial purification and characterization of 1-aminocyclopropane-1-carboxylate deaminase from the plant growth promoting rhizobacterium Pseudomonas putida GR12-2. Can J Microbiol. 40:1019–1025.
- Jain R, Chandra A, Venugopalan VK, Solomon S. 2015. Physiological changes and expression of SOD and P5CS genes in response to water deficit in sugarcane. Sugar Tech. 17:276–282.
- Jooste M, Roets F, Midgley GF, Oberlander KC, Dreyer LL. 2019. Nitrogen-fixing bacteria and Oxalis –evidence for a vertically inherited bacterial symbiosis. BMC Plant Biol. 19:441. doi:10.1186/s12870-019-2049-7.
- Kang SH, Cho HS, Cheong H, Ryu CM, Kim JF, Park SH. 2007. Two bacterial entophytes eliciting both plant growth promotion and plant defense on Pepper Capsicum annuum L. J Microbiol Biotechnol. 17(1):96–103.
- Krey T, Baum C, Ruppes L, Seydel M, Eichler Lobermann B. 2013. Organic and inorganic P sources interacting with applied rhizosphere bacteria and their effects on growth and P supply of maize. Commun Soil Sci Plan. 44(22):3205–3215.
- Kumar S, Stecher G, Tamura K. 2016. MEGA7: molecular evolutionary genetics analysis version 7,0 for bigger datasets. Mol Biol Evol. 33:1870–1874.
- Kumar V, Singh KP. 2001. Enriching vermicompost by nitrogen fixing and phosphate solubilizing bacteria. Bioresour Tech. 76:173–175.
- Lavakusha JY, Verma JP, Jaiswal DK, Kumar A. 2014. Evaluation of PGPR and different concentration of phosphorus level on plant growth: yield and nutrient content of rice Oryza sativa. Ecol Eng. 62:123–128.
- Lebeis SL. 2014. The potential for give and take in plant-microbiome relationships. Front Plant Sci. 5:287.
- Li HB, Singh RK, Singh P, Song QQ, Xing YX, Yang LT, Li YR. 2017. Genetic diversity of nitrogen-fixing and plant growth promoting pseudomonas species isolated from sugarcane rhizosphere. Front Microbiol. 8:1268.
- Li YR, Zhou XZ, Yang LT. 2015. Biological nitrogen fixation in sugarcane and nitrogen transfer from sugarcane to cassava in an intercropping system. Inter J Sci Nature. 6:214–218.
- Lin L, Guo W, Xing Y, Zhang X, Li Z, Hu C, Li S, Li Y, An Q. 2012. The actinobacterium Microbacterium sp, 16SH accepts pBBR1-based pPROBE vectors forms biofilms invades roots and fixes N2 associate d with micropropagated sugarcane plants. Appl Microbiol Biotechnol. 93:1185–1195.
- Livak KJ, Schmittgen TD. 2001. Analysis of relative gene expression data using real-time quantitative PCR and the 2−11Ct method. Methods. 25:402–408.
- Loiret FG, Ortega E, Kleiner D, Ortega-Rodés P, Rodés R, Dong Z. 2004. A putative new endophytic nitrogen-fixing bacterium Pantoea sp. from sugarcane. J Appl Microbiol. 97:504–511.
- Lorck H. 1948. Production of hydrocyanic acid by bacteria. Physiol Plant. 1:142–146.
- Mazur A, Stasiak G, Wielbo J, Koper P, Kubik-Komar A, Skorupsk A. 2013. Phenotype profiling of Rhizobium leguminosarum bv, trifolii clover nodule isolates reveal their both versatile and specialized metabolic capabilities. Arch Microbiol. 195:255–267.
- Mehnaz S, Baig DN, Lazarovits G. 2010. Genetic and phenotypic diversity of plant growth promoting rhizobacteria isolated from sugarcane plants growing in Pakistan. J Microbiol Biotechnol. 20:1614–1623. doi:10.4014/jmb.1005.05014.
- Montañez A, Abreu C, Gill PR, Hardarson G, Sicardi M. 2009. Biological nitrogen fixation in maize Zea mays L, by 15N isotope-dilution and identification of associated culturable diazotrophs. Biol Fertil Soils. 45:253–263.
- Niu JQ, Wang AQ, Huang JL, Yang LT, Li YR. 2015. Isolation characterization and promoter analysis of cell wall invertase gene SOCIN1 from sugarcane Saccharum spp. Sugar Tech. 17:65–76.
- Noda S, Ohkuma M, Usami R, Horikoshi K, Kudo T. 1999. Culture independent characterization of a gene responsible for nitrogen fixation in the symbiotic microbial community in the gut of the termite Neotermes koshunensis. Appl Environ Microbiol. 65:4935–4942.
- Olanrewaju OS, Glick BR, Babalola OO. 2017. Mechanisms of action of plant growth promoting bacteria. World J Microbiol Biotechnol. 33:197.
- Oliveira ALM, Urquiaga S, Dobereiner J, Baldani JI. 2002. The effect of inoculating endophytic N2 -fixing bacteria on micropropagated sugarcane plants. Plant Soil. 242:205–215.
- Park M, Kim C, Yang J, Lee H, Shin W, Kim S, Sa T. 2005. Isolation and characterization of diazotrophic growth promoting bacteria from rhizosphere of agricultural crops of Korea. Microbiol Res. 160:127–133.
- Passari AK, Mishra VK, Gupta VK, Yadav MK, Saikia R, Singh BP. 2015. In vitro and in vivo plant growth promoting activities and DNA fingerprinting of antagonistic endophytic actinomycetes associates with medicinal plants. PLoS ONE. 10:e0139468.
- Paungfoo-Lonhienne C, Lonhienne TGA, Yeoh YK, Donose BC, Webb RI, Parsons J, Liao W, Sagulenko E, Lakshmanan P, Hugenholtz P, et al. 2016. Crosstalk between sugarcane and a plant-growth promoting Burkholderia species. Sci Rep. 6:37389.
- Perin L, Martinez-Aguilar L, Paredes-Valdez G, Baldani JI, Estrada-de los Santos P, Reis VM, Caballero-Mellado J. 2006. Burkholderia silvatlantica sp, nov, a diazotrophic bacterium associated with sugarcane and maize. Int J Syst Evol Microbiol. 56:1931–1937.
- Pikovskaya RI. 1948. Mobilization of phosphorus in soil in connection with the vital activity of 804 some of the microbial species. Microbiologiya. 17:362–370.
- Poly F, Monrozier LJ, Bally R. 2001. Improvement in the RFLP procedure for studying the diversity of nifH genes in communities of nitrogen fixers in soil. Res Microbiol. 152:95–103.
- Rafikova GF, Korshunova T, Minnebaev LF, Chetverikov SP, Loginov ON. 2016. A new bacterial strain Pseudomonas koreensis IB-4 as a promising agent for plant pathogen biological control. Microbiol. 85:333–341.
- Reis VM, Olivares FL, de Oliveira ALM, dos Reis JFB, Baldani JI, Dobereiner J. 1998. Technical approaches to inoculate micro-propagated sugarcane plants with Acetobacter diazotrophicus. Plant Soil. 206:205–211.
- Remus R, Ruppel S, Jacob HJ, Hecht-Buchholz C, Merbach W. 2000. Colonization behaviour of two enterobacterial strains on cereals. Biol Fert Soils. 30(5-6):550–557.
- Robertson-Albertyn S, Alegria Terrazas R, Balbirnie K, Blank M, Janiak A, Szarejko I, Chmielewska B, Karcz J, Morris J, Hedley PE, et al. 2017. Root hair mutations displace the Barley rhizosphere microbiota. Front Plant Sci. 8:1094. doi:10.3389/fpls.2017.01094.
- Rosado AS, Duarte GF, Seldin L, Elsas JDV. 1998. Genetic diversity of nifH gene sequences in Paenibacillus azotofixans strains and soil samples analyzed by denaturing gradient gel electrophoresis of PCR amplified gene fragments. Appl Environ Microbiol. 64:2770–2779.
- Rosenblueth M, Martínez-Romero E. 2006. Bacterial endophytes and their interactions with hosts. Molecular Plant-Microbe Interactions®. 19:827–837.
- Sahoo RK, Ansari MW, Dangar TK, Mohanty S, Tuteja N. 2014. Phenotypic and molecular characterisation of efficient nitrogen-fixing Azotobacter strains from rice fields for crop improvement. Protoplasma. 251:511–523.
- Sahran BS, Nehra V. 2011. Plant growth promoting rhizobacteria: a critical review. Lif Sci Med Res. 21:1–30.
- Saitou N, Nei M. 1987. The neighbor-joining method: a new method for reconstructing phylogenetic trees. Mol Biol Evol. 4:406–425.
- Santi C, Bogusz D, Franche C. 2013. Biological nitrogen fixation in non-legume plants. Ann Bot. 111:743–767.
- Sarkar A, Ghosh PK, Pramanik K, Mitra S, Soren T, Pandey S, Mondal MH, Maiti TK. 2018. A halotolerant Enterobacter sp. displaying ACC deaminase activity promotes rice seedling growth under salt stress. Res Microbiol. 169:20–32.
- Schwyn B, Neilands JB. 1987. Universal chemical assay for the detection and determination of siderophores. Anal Biochem. 160:47–56.
- Sevilla M, Burris RH, Gunapala N, Kennedy C. 2001. Comparison of benefit to sugarcane plant growth and 15N2 incorporation following inoculation of sterile plants with Acetobacter diazotrophicus wild-type and Nif− mutant strains. Molecular Plant-Microbe Interactions®. 14:358–366.
- Silveira APD, Sala VMR, Cardoso EJBN, Labanca EG, Cipriano MAP. 2016. Nitrogen metabolism and growth of wheat plant under diazotrophic endophytic bacteria inoculation. Appl Soil Ecol. 107:313–319.
- Singh RK, Kumar DP, Solanki MK, Singh P, Srivastava S, Srivastva AK, Kumar S, Kashayp PL, Singhal PK, Arora DK. 2013. Multifarious plant growth promoting characteristics of chickpea rhizosphere associated Bacilli help to suppress soil-borne pathogens. Plant Growth Regul. 73:91–101.
- Singh RK, Singh P, Li HB, Song QQ, Guo DJ, Solanki MK, Verma KK, Malviya MK, Song XP, Lakshmanan P, et al. 2020. Diversity of nitrogen fixing rhizobacteria associated with sugarcane A comprehensive study of plant-microbe interactions for growth enhancement in Saccharum spp. BMC Plant Biol. doi:10.1186/s12870-020-02400-9.
- Singh RK, Singh P, Li HB, Yang LT, Li YR. 2017. Use of nitrogen-fixing bacteria for plant growth and development in sugarcane. In: DP Singh, et al., editors. Plant-microbe interactions in agro-ecological perspectives. Singapore: Springer Nature; 3:35–59.
- Singh P, Song QQ, Singh RK, Li HB, Solanki MK, Malviya MK, Verma K K, Yang LT, Li YR. 2019. Proteomic analysis of the resistance mechanisms in sugarcane during Sporisorium scitamineum infection. Int J Mol Sci. 20:569. doi:10.3390/ijms20030569.
- Singh P, Song QQ, Singh RK, Li HB, Solanki MK, Yang LT, Li YR. 2018. Physiological and molecular analysis of sugarcane varieties-F134 and NCo310 during Sporisorium scitamineum interaction. SugarTech. 21:631–644.
- Smyth DR, Bowman JL, Meyerowitz EM. 1990. Early flower development in Arabidopsis. Plant Cell. 2:755–767.
- Sneath PHA, Sokal RR. 1973. Numerical taxonomy. San Francisco (CA): Freeman.
- Somers E, Vanderleyden J, Srinivasan M. 2004. Rhizosphere bacterial signalling: a love parade beneath our feet. Crit Rev Microbiol. 30:205–240.
- Song XP, Huang X, Mo FL, Tian DD, Yang LT, Li YR, Chen BS. 2013. Cloning and expression analysis of sugarcane phenylalanine ammonia-lyase PAL gene. Sci Agric Sin. 46:2856–2868.
- Su Y, Xu L, Fu Z, Yang Y, Guo J, Wang S, Que Y. 2014. Scchi, encoding an acidic class III chitinase of sugarcane, confers positive responses to biotic and abiotic stresses in sugarcane. Int J Mol Sci. 15:2738–2760. doi:10.3390/ijms15022738.
- Su YC, Xu LP, Xue BT, Wu QB, Guo JL, Wu LG, Que YX. 2013. Molecular cloning 840 and characterization of two pathogenesis related ß-13-glucanase genes ScGluA1 and ScGluD1841 from sugarcane infected by Sporisorium scitamineum. Plant Cell Rep. 32:1503–1519.
- Suckstorff I, Berg G. 2003. Evidence for dose dependent effects on plant growth by Stenotrophomonas strains from different origins. J Appl Microbiol. 95:656–663.
- Sundar AR, Barnabas EL, Malathi P, Vishwanathan RA. 2012. Mini-review on smut disease of sugarcane caused by sporisorium scitamineum. In: J. Mworia Rijeka, editor. Botany. In Tech Publisher; p. 109–128.
- Tamura K, Nei M, Kumar S. 2004. Prospects for inferring very large phylogenies by using the neighbor-joining method. Proc Natl Acad Sci USA. 101:11030–11035.
- Taulé C, Mareque C, Barlocco C, Hackembruch F, Reis VM, Sicardi M, Battiston F. 2012. The contribution of nitrogen fixation to sugarcane Saccharum officinarum L, and the identification and characterization of part of the associated diazotrophic bacterial community. Plant Soil. 356:35–49. doi:10.1007/s11104-011-1023-4.
- Timmusk S, Grantcharova N, Wagner GH. 2005. Paenibacillus polymyxa invades plant roots and forms biofilms. Appl Environ Microbiol. 71:7292–7300.
- Urquiaga S, Cruz KHS, Boddey RM. 1992. Contribution of nitrogen fixation to sugarcane nitrogen-15 and nitrogen balance estimates. Soil Sci Soc Am Proc. 56:105–114.
- Urquiaga S, Xavier RP, de Morais RF, Batista RB, Schultz N, Leite JM, Sa JME, Barbosa K P, de Resende AS, Alves BJR, Boddey RM. 2012. Evidence from field nitrogen balance and 15N natural abundance data for the contribution of biological N fixation to Brazilian sugarcane varieties. Plant Soil. 356:5–21.
- Venturi V, Keel C. 2016. Signaling in the rhizosphere. Trends Plant Sci. 21(3):187–198. doi:10.1016/j.tplants.2016.01.005.
- Verma R, Maurya BR, Meena VS, Dotaniya ML, Deewan P, Jajoria M. 2017. Enhancing production potential of cabbage and improves soil fertility status of Indo-Gangetic Plain through application of bio-organics and mineral fertilizer. Int J Curr Microbiol Appl Sci. 63:301–309.
- Viswanathan R, Rao GP. 2011. Disease scenario and management of major sugarcane diseases in India. Sugar Tech. 13:336–353. doi:10.1007/s12355-011-0102-4.
- Wang X, Wang Y, Tian J, Lim BL, Yan X, Liao H. 2009. Over expressing at PAP15 enhances phosphorus efficiency in soybean. Plant Physiol. 151:233–240.
- Wielbo J, Marek-Kozaczuk M, Kubik-Komar A, Skorupska A. 2007. Increased metabolic potential of Rhizobium spp. is associated with bacterial competitiveness. Can J Microbiol. 53:957–967.
- Wilson D. 1995. Endophyte: the evolution of a term and clarification of its use and definition. Oikos. 73(2):274–276. doi:10.2307/3545919.
- Xing YX, Wei CY, Mo Y, Yang LT, Huang SL, Li YR. 2016. Nitrogen-Fixing and plant growth-promoting ability of Two endophytic bacterial strains isolated from sugarcane Stalks. Sugar Tech. 18(4):373–379.
- Zahir ZA, Muhammad A, Frankenberger WTJ. 2003. Plant growth promoting rhizobacteria: applications and perspectives in agriculture. Adv Agron. 81:97–168.
- Zehr JP, Capone DG. 1996. Problems and promises of assaying the genetic potential for nitrogen fixation in the marine environment. Microb Ecol. 32:263–281.
- Zehr JP, Jenkins BD, Short SM, Steward GF. 2003. Nitrogenase gene diversity and microbial community structure: a cross-system comparison. Enviro Microbiol. 5:539–554.
- Zeng Q, Wu X, Wen X. 2016. Identification and characterization of the rhizosphere phosphate-solubilizing bacterium Pseudomonas frederiksbergensis JW-SD2 and its plant growth-promoting effects on poplar seedlings. Ann Microbiol. 66:1343–1354.
- Zewide I. 2019. Role of bio-fertilizer for maximizing productivity of selected horticultural crops. J Agri Res Adv. 0102:01–18.
- Zhang M, Govindaraju M. 2018. Sugarcane production in China. Sugarcane-Technol Res. doi:10.5772/intechopen.3:73113.
- Zhu B, Zhou Q, Lin L, Hu C, Shen P, Yang L, An Q, Xie G, Li Y. 2013. Enterobacter sacchari sp, nov, a nitrogen-fixing bacterium associated with sugar cane Saccharum officinarum L. Int J Syst Evol Microbiol. 63:2577–2582.