ABSTRACT
This study explores the potential feasibility of cultivating two determinate-type bell peppers, i.e. Hungarian Enigma Hot (HEH) and Hungarian Enigma Sweet (HES), in a ground bioregenerative life-support device. Fruits of the Hugarian Engima Sweet (HES) variety is advantageous for digestion due to it has thin cuticle and collenchyma at the same time the parenchyma of mesocarp of it is thin. β-carotene and caspanthin were the dominant carotenoids in both fruit varieties. Up to 55 tentative phytochemicals were not only in fruits but also in green juice processed from green leafy shoots. The green juice was also a rich source of protein (24–27% w/w) and a source of macro- and microelements. The findings of this study could hold valuable insights for the advancement of sustainable food production and resource utilization in space environments, with potential applications for long-duration space missions and beyond.
Key policy highlight
Two determinate-growth green pepper varieties with specific characteristics were grown in ground bioregenerative life-support research device
Fruits of the Hugarian Engima Sweet (HES) variety is advantageous for digestion as it has thin cuticle and collenchyma at the same time the parenchyma of mesocarp is thin
The green juice processed from green leafy shoots is a rich source of protein (24–27% w/w), luteins, flavonoids, phenolic acids and derivatives.
With the change in color of the fruits from green to red, β-carotene and capanthin as carotenoids showed the greatest increase.
Content of flavonoids and phenolic components, i.e. quercetin-3-O-d-glucopyranoside, rutin (quercetin-3-O-rutinoside), caffeic acid and ferulic acid showed a decreased trend during fruit ripening.
1. Introduction
We are currently entering a new era of Space exploration, with humans preparing to travel further from the Earth than ever before. The US National Aeronautics and Space Administration’s (NASA) Artemis program, in collaboration with the European (ESA), Canadian, and Japanese space agencies, proposes to build an orbiting lunar Space station (Gateway) to support landing humans on the lunar surface by 2024 (Gateway Citation2021; Mortimer and Gilliham Citation2022). The need to understand how plants respond to off-Earth conditions has been recognized for over 80 years (Wheeler et al. Citation2003; Wheeler Citation2017). Aboard the ISS (International Space Station), the NASA Vegetable Production System (Veggie) and the Advanced Plant Habitat (APH) have been used to grow a range of plant species, including lettuce (Lactuca sativa), Zinnia elegans, radish (Raphanus sativus), dwarf wheat (Triticum aestivum), and others.
Such experiments have shown that the tested plant species can grow in microgravity and that the plants produced are safe to eat (Khodadad et al. Citation2020). To choose possible crops for the research on Bioregenerative Life Support Systems (BLSS), the crop production test in the Breadboard Project at the Kennedy Space Center (NASA) has identified the best-performing cultivars for 14 crops, which grew better in the biomass production chamber (Wheeler et al. Citation2003). For the same purpose, 13 factors that are associated with diet, nutrition, and system condition were considered. In China, over 70 crop species were analyzed step by step according to the criteria. Also, 14 crop candidates, including four food crops (i.e. wheat, rice, soybean, peanut) and seven vegetables (i.e. Chinese cabbage, lettuce, radish, carrot, tomato, squash, pepper), as well as Welsh onion, garlic, and coriander, have been selected for BLSS research in China (Chunxiao and Hong Citation2008). If humankind is ever to undertake long-term space missions and colonization, establishing an efficient space farming system would be essential for human survival in space. However, existing crops are not sufficiently cost-effective and productive for use on space farms (Mortimer and Gilliham Citation2022). This led to the proposal of a Crop Readiness Level (CRL) scale to evaluate the suitability of various crop species for growth in space. Crops grown in space-based in situ crop production systems need to be subjected to additional testing and analysis to account for unique environmental and operational challenges of space. The CRL scale concept ensures systematic and consistent development of crops for space-based applications (Romeyn et al. Citation2019).
Studies on edible produce for spaceflight are limited, and a significant knowledge gap remains to determine the best cultivars to obtain acceptable, nutritious pick-and-eat foods for consumption in the spaceflight environment. At the same time, reduced-area container gardening has increased in popularity, and numerous new commercial vegetable varieties that can be productive in space-constrained conditions are now available (Spencer and Hummerick Citation2019). The Whole-Body Edible and Elite Plant (WBEEP) strategy for space crop improvement relies on plant biotechnology. The WBEEP strategy aims to develop crops with more edible parts, richer nutrient content, higher yields, and higher mineral nutrient use efficiencies for space farms, as well as enhance the regenerative capacity of the carbon reduction cycle and photosynthesis (Liu et al. Citation2021).
Peppers (Capsicum sp.) are the third most consumed vegetable worldwide. Its importance is supported by the considerable biological value of its fruits (Krishna De Citation2003; Bosland and Votava Citation2012). It is well-known that peppers are exceptionally rich in vitamin C, carotene, and vitamin A. Among biological active compounds, however, the fruit of peppers also contains other nutritionally valuable minerals for the astronauts (Ca, Mg, Fe, and K), as well as vitamin K, phenolics, anthocyanin, lutein, and zeaxanthin (Spencer and Hummerick Citation2019). In addition, to consume pepper in a pick-and-eat salad crop system on ISS and future exploration flights, this vegetable has exceptional potential. During the last five decades, space and ground BLSS targeted to explore the biological potential of bell and spice peppers by NASA, ESA, and Chinese scientists (Johnson and Tibbitts Citation1968; Brown et al. Citation1974; Salisbury and Clark Citation1996a, Citation1996b; Chunxiao and Hong Citation2008; Patterson Citation2011; Patterson et al. Citation2012; Lunn et al. Citation2017; Douglas et al. Citation2021; Johnson et al. Citation2021; Maiwald et al. Citation2021; NASA and DLR Citation2021; Zeidler et al. Citation2021).
In 2021, NASA successfully cultivated peppers at the International Space Station in NASA’s Advanced Plant Habitat (APH) growth chamber for 137 days. It was the longest plant experiment to date in the orbital laboratory. The PH-04 experiment started on July 12, 2021, and concluded on November 26. This pepper was a mild heat Hatch variety called NuMex ‘Española Improved’ bred by the New Mexico State University in 1986. The crew on the station sampled their freshly harvested peppers and used them as a fresh ingredient to make tacos in space. The pepper samples were returned to the Earth for scientific analysis at NASA’s Kennedy Space Centre in Florida (Herridge Citation2021). It is important to mention that this great advancement has more than a decade of well-established preliminary space-pepper growing investigations carried out by NASA and the researchers at the German Aerospace Centre (DLR) (Graham and Wheeler Citation2016, Citation2017; Lunn et al. Citation2017; EDEN ISS Citation2018; Hollingsworth Citation2019; Spencer and Hummerick Citation2019; Zabel et al. Citation2020; Douglas et al. Citation2021).
From many aspects, improving volume utilization efficiency and diminishing the harvest index is crucial for successful pepper space plant cultivation (Graham and Wheeler Citation2016). According to these authors, the root restriction of pepper is also an inevitable consequence of spaceflight crop production, where growing volumes are extremely limited. The effects of root restriction need to be considered when interpreting spaceflight crop production data; however, the effects can also be taken advantage of in a properly managed system to improve volume use efficiency for food production while reducing the amount of inedible biomass, which must be dealt with as solid waste (Graham and Wheeler Citation2016). However, to obtain better volume utilization efficiency, there are some interesting genetic aspects for pepper space cultivation. One of them could be growing suitable dwarf pepper cultivars bred by breeding companies and/or selecting new ones.
In Hungary, the breeding, growing, and researching of determinate spicy paprika and bell pepper varieties have great tradition since the last five decades of the twentieth century. Here, special production methods, the short growing period, and the dense plant populations used in Hungary were responsible, among others, for the great success of determinate-type pepper varieties cultivated first under traditional hotbed growing facilities and later under heated and unheated plastic tunnels (Kormos and Kormos Citation1956; Angeli Citation1964, Citation1971; Moóor and Zatykóo Citation1995; Zatykó Citation2006; Csilléry et al. Citation2016). The biotechnology of pepper has great advances during the recent decades since the first steps were carried out at the beginning of the 1980-ies (Gunay and Rao Citation1978; Fári and Czakó Citation1981; Fári Citation1983, Citation1986; Mityko et al. Citation1995).
The general goals of our research is to explore the potential of some biotech methods available to produce novel Whole-Body Edible peppers (WBE). The specific objective was to investigate the biochemical values of the fruits and green biomass of the two dedicated pepper cultivars, including in vitamins, protein and macro-microelement content, also qualitative and quantitative changes of other phytonutrients with a view to their food consumption. In addition, the anatomical analysis of the fruit was considered important for digestibility.
2. Materials and methods
2.1. Experimental location and growth conditions
Hydroponic experiments were set up in the BIODROME research greenhouse of the University of Debrecen, Hungary, to compare two determinate-growth pepper varieties for their possible cultivation in airspace conditions. The two varieties are the Hungarian Enigma Hot (HEH), which is characterized by a hot fruit and Hungarian Enigma Sweet (HES), which is recognized for its sweet fruit. Both varieties were produced from determinate-growth Hungarian heirloom pepper cultivars bred in the eighties of the last century and maintained in our genebank collection. The HEH variety was further selected from the Budai csípős cultivar, while The HES variety was produced from the Tizenegyes.
For germination, seeds were sown in a propagation tray filled with a peat-free organic substrate mixture. When the first flower bud emerged, the plants were transferred to the GHE Aeroflow 10 hydroponic system (General Hydroponics Europe, FarmerTec.-Treibhaustechnik, Germany) and grown to fruit maturity stage (125 days, (A–F)). Each unit of the system consists of five pieces of 9 cm diameter plastic GHE pots sunk into a closed PVC channel. The green pepper seedlings were planted in 20 replicates per variety in 4 independent units. The units can be flexibly assembled horizontally. The pots were filled with a peat-free organic substrate mixture, supplemented with a high-capacity WaterWick absorbent cord (Visser Horti System, The Netherlands) ((A,B)). From the liquid medium contained closed channel, the nutrient availability to the plants was ensured by the WaterWick cord. Complementary 400 µmol/m2/sec LED lighting was provided by the HORTILED Multi 4DIM system (Hortilux Schréder, The Netherlands), with 12 h of light period per day.
Figure 1. Horizontally arranged GHE Aeroflow 10 hydroponic pepper cultivation system growing Hungarian Enigma Sweet (HES) pepper seedlings (A); Hydroponic-type root system of the 90-day-old Hungarian Enigma Sweet pepper complemented by WaterWick absorbent cord (B); 125-day-old Hungarian Enigma Sweet pepper plant showing the entire foliage and both, the substrate-type and the hydroponic-type root system (C); 125-day-old Hungarian Enigma Sweet pepper plant showing the entire foliage and both, the substrate-type and the hydroponic-type root system (D); Fruits of the Hungarian Enigma Sweet pepper at different maturity stage (E); Fruits of the Hungarian Enigma Hot (HEH) pepper at different maturity stage (F).
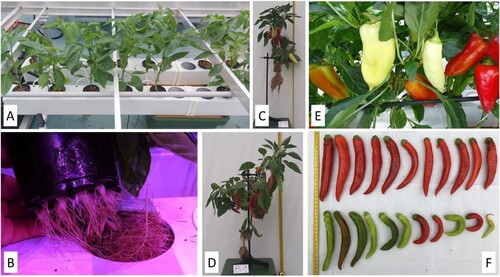
The composition of the liquid media was provided by Chili Focus, as a commercially available product recommended specifically for green peppers (Growth Technology Ltd., Taunton, Sommerset, UK). During the plant growth, the nutrient supply of the liquid culture medium was monitored every two days based on the EC (1.5–2.0 mS/cm) and pH measurements (pH 5.5).
2.2. Fruit harvest and green biomass processing
Plants were harvested at 125 days after seedling transplantation. Fruits of each plant were separately harvested and divided into three groups according to the fuit colors as follows: (i) green/pale green fruits (ripe), (ii) yellow fruits (medium ripe), and (iii) red fruits (fully ripe). The HEH variety belongs to the ‘Horn’ type of peppers, whose green-coloured fruit can be considered ripe ((F)). Similarly, the green-coloured fruit of the HES variety, belonging to the ‘Cecei’ type of peppers, can also be considered commercially ripe. It should be noted, however, that the green fruit of HES is pale green, almost white ((E)), and for clarity only, the green color is used consistently for both varieties.
The fresh weight of each fruit type was recorded per plant, and the fresh weight of leaves, stem, and roots per plant was measured. Harvested fruits were later lyophilized using the Alpha 1–4 LSC basic (Martin Christ Ltd., Germany) lyophilizer and then ground using a bead mill (model: EDW-50, Shanghai, China). Powdered fruits were kept in polyethylene bags in a −20°C freezer for further analyses. Harvested fresh green aboveground biomass (leafy shoots) of green peppers was mechanically pressed using a twin-screw juicer (Angel Juicer 5500, Angel Ltd, South Korea), producing a green liquid called ‘green juice’ and pressed cake called ‘fiber’. The green juice fraction was freeze-dried using Alpha 1–4 LSC basic (Martin Christ Ltd., Germany) liophylizer, and powdered using a bead mill (model: EDW-50, Shanghai, China), and then kept in polyethylene bags in −20°C freezer for future analyses.
2.3. Anatomical and biochemical characterization of green pepper varieties
2.3.1. Anatomical analysis of fruits
For the anatomical incestigations, two harvested fruits per plant were analyzed. Cuttings from the mid-third of the fruit were preserved in Strasbourger-Flemming solution (glycerol:water:alcohol 1:1:1). Sections were sectioned after paraffin embedding using a rotary microtome (Leica RM2255_Fully Automated Rotary Microtome, Leica Biosystems US). A section was placed in a 1:1 mixture of distilled water and sodium hypochlorite and was stained with toluidine blue stain. The cross-sections were examined with a light microscope (Zeiss Axioskop Light Microscope 2+, Göttingen, Germany) and digitized using Scope Photo 3.1 (ScopeTec) software and the selected anatomical features were measured. The relevant anatomical parameters measured were: cuticle thickness, cuticle and external wall of the epidermis together, epidermis thickness, hypoderma thickness, number of cell rows in hypoderma, mean cell wall thickness of hypodermis, mesocarp thickness, endocarp thickness. For all parameters 80 data were recorded.
2.3.2. Determination of total polyphenols (TPC) and flavonoids (TFC)
2.3.2.1. Sample preparation
Total polyphenols (TPC) and flavonoids (TFC) in green juices and fruits of the two green pepper varieties (HEH and HES) were extracted using methanol: distilled water (70:30) extract. Right after the fruit harvest and the initial pressing of the green biomass, the green juices and fresh fruits were promptly stored at −20°C for freezing. Subsequently, they underwent a freeze-drying process using a lyophilizer (an Alpha 1–4 LSC basic lyophilizer, Osterode am Harz, Germany). A 20 mg lyophilized sample was placed into a 1.5 mL Eppendorf tube, and 1 mL of extraction solution was added. Mixtures were vortexed for 2 min at room temperature and then incubated in an ultrasonic bath at room temperature for 60 min. Supernatants were separated by centrifuge at 10,000 rpm for 5 min at room temperature.
2.3.2.2. Measurements
Folin–Ciocalteu reagent was applied to measure TPC in supernatants as described by Singleton and Rossi (Citation1965). The absorbance of developed blue color was measured at 760 nm using the model UV-160A spectrophotometer (Shimadzu, Japan). A standard curve was constructed using various concentrations of gallic acid, specifically 0, 2.4, 4.8, 7.2, 9.6, 12.0, and 14.4 mg/L. The TPC concentration in the samples was then determined and reported as mg of gallic acid equivalent per g of dry weight (mg GAE/g DW). To create a blank sample, the plant sample's supernatant was replaced with 70% methanol. In cases where the absorbance reached approximately 2, dilution was performed.
A 500 µL of supernatants was used to quantify the TFC using aluminum chloride assay based on the method reported by Barna et al. (Citation2022). The absorbance of developed color was measured at 415 nm sing the model UV-160A spectrophotometer (Shimadzu, Japan). We generated a standard curve by employing different concentrations of rutin, namely 0, 2, 8, 14, 20, 26, and 32 mg/L. The TFC concentration in the samples was determined using the rutin standard and reported as mg of rutin equivalent per g of dry weight (mg RE/g DW). When the absorbance approached approximately 2, we conducted dilutions as necessary.
2.3.3. Determination of water and lipid soluble antioxidant capacity (Photochemiluminescence Assay)
This method was originally described by Popov and Lewin (Citation1994) and is distributed by Analytik Jena AG (Jena, Germany) as a complete system called Photochem®. The principles of the assay are described in detail by Nemes et al. (Citation2018), and can be used to measure hydrophilic and lipophilic antioxidants separately.
2.3.3.1. ACL: lipid-soluble antioxidant capacity
The lipid-soluble antioxidant capacity was measured with the ACL kit. The reaction solutions were prepared by mixing 2.3 mL Reagent 1 (100% methanol), 200 µL Reagent 2 (buffer solution), 25 µL Reagent 3 (photosensitizer and detection reagent), and 0–30 µL of Reagent 4 (calibration standard for quantification of lipophilic antioxidants in Trolox equivalents) or 10 µL of sample (beverage diluted with Reagent 1). After thorough mixing, the measurement was performed. The detector detected the current proportion to the generated luminescence as a function of the measurement time. The detector signal was followeed for 180 s and the results were expressed in µg trolox equivalent per g dry mass (Nemes et al. Citation2018).
2.3.3.2. ACW (water-soluble antioxidant capacity)
The ACW kit was used to measure the water-soluble antioxidant capacity. The reaction mixture was prepared from the following components: 1.5 mL of Reagent 1 (buffer solution pH 10.5), 1 mL of Reagent 2 (reaction buffer), 25 µL Reagent 3 (photosensitizer and detection reagent) and 0–30 µL of Reagent 4 (calibration standard for quantification of water-soluble antioxidants in ascorbic acid equivalents) or 10 µL of sample (beverage diluted with Reagent 1). The detector detected the current proportion to the generated luminescence as a function of the measurement time. The detector signal was followed for 250 s, and the results were expressed in µg ascorbic acid equivalent per g dry mass (Nemes et al. Citation2018).
2.3.4. Quantification of photosynthetic pigments
Following collection, the samples were promptly freeze-dried in small portions to prevent pigment degradation. A 10 mg of lyophilized sample was weighed into Eppendorf tubes and shaken thoroughly by adding 2 mL of 96% ethanol, and the samples were subjected to ultrasonic bath treatment to aid pigment extraction effectively. The samples were incubated in an ultrasonic bath for 1 h in the dark. After incubation, the samples were centrifuged at 13000 rpm for 2 min and the absorbance of the supernatant was measured at λ = 440, 480, 495, 649, 665. The resulting values were calculated with an appropriate formula as described by Kaszás et al. (Citation2018). Throughout this process, the samples were shielded from light and stored in darkness. Spectrophotometric measurements were conducted promptly after sample preparation. This method was chosen for its practicality, cost-efficiency, and expeditiousness.
2.3.5. Speciation of carotenoid derivatives
2.3.5.1. Sample preparation
A 50 mg of powdered sample was added to 2 × 1 mL of a 2:1:1:1 v/v% solvent mixture of dichloromethane: acetone: methanol. Digestion of the samples was performed with a bead homogenizer (600 rpm; 2x 30 sec) repeated twice, and then centrifuged at 7500 rpm for 5 min. The supernatants were evaporated to dryness using a rotary vacuum evaporator (Heidolph Instruments, Schwabach Germany). Then samples were dissolved in 300 µl carotenoid eluent (Izopropanol: Acetonitril: Metanol 55:35:10 v/v%) + 300 µL methanol. Before separation, samples were filtered through a 0.22 µm PTFE filter.
2.3.5.2. Measurements
A Waters Alliance liquid chromatograph consisting of an e2695 separation module and a photodiode array (PDA) detector (model 2998) (Waters, Milford, MA) was used for the quantitative analysis of carotenoids. Carotenoids were quantified using standard compounds: lutein (≥96.0%); β-Carotene (Tisztaság ≥99,7%); Zeaxanthin (≥95.0%); Violaxanthin (≥95.0%); Capsanthin (≥95.0%). All standards were purchased from Sigma-Aldrich (Darmstadt, Germany) or Cayman Chemical Co. (Michigan; United States) or Bio-Synthesis, Inc Lewisville, Texas, U.S. The HPLC instrument was operated using Empower 3 software (Waters). Separations were performed on a Waters X select C18 column (100 × 4.6 mm; 3.5 µm) using a gradient elution system with eluent A: methanol and eluent B: isopropanol: acetonitrile: methanol (55:35:10 v/v%). The flow rate was maintained at 1.0 ml/min. The gradient profile was: 0–15 min 100% A; 15–20 min 20% A – 80% B; 20–25 min 20% A – 80% B; 25–27 min 100% B; 27–30 min 100% B; 30–40 min 100% B. The limit of detection (LOD) and limit of quantifiaction (LOQ) parameters was not relevant based on the known spectral properties of the identified components and the concentration ratios in the sample.
2.3.6. Determination of total nitrogen, protein, and carbon contents
The Dumas method was employed to determine the nitrogen, protein, and carbon contents using an Elementar Vario Max Cube (Elementar Analysensysteme GmbH, Germany) (Cenci et al. Citation2021). In this method, 250 mg of the plant sample was placed in porcelain cartridges and combusted within the instrument's combustion tube, which was heated to 925°C, in an oxygen-rich environment, free of ambient air. The combustion process converted the nitrogen content into N2 and carbon content into CO2. The reduction tube of the apparatus further converted nitrogen oxides to N2. After combustion and reduction, CO2 was separately captured on adsorption columns. The instrument measured the amount of N2, and subsequently, after successive heating of the CO2 columns and desorption of the gases, the amount of carbon was determined. Helium served as the carrier gas during this process. The protein content was estimated by applying a nitrogen conversion factor of 4.4 (Mariotti et al. Citation2008).
2.3.7. Qualitative analysis of phytochemicals and quantitative analysis of vitamins
2.3.7.1. Sample preparation
Extraction of phytochemicals was carried out as described in section (2.4.2.) using hydro-alcoholic extraction of the freeze-dried samples. In brief, a 0.5 g of freeze-dried sample was weighed into a 15 mL centrifuge tube and 10 mL of extraction solvent (70% methanol) was added. After homogenization, the samples were shaken for 60 min. in an ultrasonic bath at room temperature. The extracts were then centrifuged for 5 min at 10,000 rpm. Then the hydroalcoholic extracts were filtered through a 0.22 µm PTFE syringe filter.
2.3.7.2. Measurments
The phytochemical composition was qualitatively analyzed using a UHPLC-ESI-ORBITRAP MS/MS hyphenated analytical system following the methods described in Kaszás et al. (Citation2020) and Domokos-Szabolcsy et al. (Citation2022). In brief, the UHPLC system (Dionex Ultimate 3000RS) was coupled to a Thermo Q Exactive Orbitrap hybrid mass spectrometer, which was equipped with a Thermo Accucore C18 analytical column (2.1 × 100 mm, 2.6 µm particle size). The mobile phase consisted of methanol (A) and water (B) (both acidified with 0.1% formic acid) and a 70-minute gradient program was employed, maintaining a flow rate of 0.2 mL/min. The gradient program was as follows: 0–3 min, 95% B; 3–43 min, 0% B; 43–61 min, 0% B; 61–62 min, 95% B; and 62–70 min, 95% B. The injection volume was 2 µL.
The Thermo Q Exactive Orbitrap hybrid mass spectrometer was furnished with electrospray ionization (ESI) source. The samples underwent separate measurements in both positive and negative ionization modes. Data collection and processing were conducted using Thermo Trace Finder 2.1 software, incorporating information from proprietary databases and online sources such as Metlin, Mass Bank of North America, and m/z Cloud. Following processing, the results underwent manual verification using Thermo Xcalibur 4.0 software. The LOD was 300 pg/mL and LOQ was 1 ng/mL.
Quantitative determination of vitamins was made taking into account the following selected standard compounds: nicotinic acid (≥95.0%); nicotinamide (≥99.5%); biotin (≥99.0%). All standards were purchased from Sigma-Aldrich (Darmstadt, Germany).
2.3.8. Quantitative analysis of phenolic compounds
2.3.8.1. Sample preparation
A 0.5 g of freeze-dried sample was weighed into a 15 ml centrifuge tube and 10 ml of extraction solvent (60% methanol, 39% water, and 1% formic acid) was added. After homogenization, the samples were shaken for 30 min. The extracts were then centrifuged for 10 min at 3000 rpm and 4°C. During the measurements the supernatant fraction was analyzed. Each sample was prepared in three repeats. The quantification was performed by matrix-matched calibration.
2.3.8.2. Measurement setup
Separations were performed using a Phenomenex Kinetex EVO C18 100 × 2.1 mm, 100A, 2.6 µm column with reversed phase stationary phase (Phenomenex, Inc California, USA) and a corresponding SecurityGuard ULTRA Cartridges for UHPLC EVO C18 for 2.1 mm ID Columns. Water containing 0.1% v/v formic acid (‘eluent A’) and UHPLC-MS grade acetonitrile (‘eluent B’) were used as eluents at a flow rate of 0.5 mL/min. The column temperature was 30°C. The injected sample volume was 10 µL. For the analysis of polyphenols, gradient elution with binary mobile phases was used. The gradient profile was: 0 min 95% A – 5% B; 4,0 min 40% A – 60% B; 4,1 min 0% A – 100% B; 5,1 min 0% A – 100% B. A tandem (QQQ) mass spectrometer equipped with an ESI ion source was used as a detector. The measurements were performed in dMRM (Dynamic Multiple Reaction Monitoring) mode. Quantitative determination of phenolic compounds was made taking into account the following selected standard compounds: 5-CQA ((≥98%),); caffeic acid (≥98%); p-coumaric acid; ferulic acid (USP reference standard); rutin (≥94%); sinapic acid (≥98%); quercetin-3-O-d glucopyranoside (≥98.0%); luteolin (≥98%); apigenin (≥95.0%). All standards were purchased from Sigma-Aldrich (Darmstadt, Germany). The signal-to-noise ratio method was used to determine the LOD and LOQ, namely a comparison was made between the signals from sample components of low known concentrations prepared in the pepper matrix and the blank signal in the pepper matrix. The baseline, before and after the given component on the chromatogram, was used as a blank signal. The signal-to-noise ratio was 3:1 for LOD and 10:1 for LOQ (). The UHPLC-ESI-MS/MS method was validated as described by Matkovits et al. (Citation2023).
Table 1. Limit of detection (LOD) and limit of quantification (LOQ) values for polyphenols in green pepper.
2.3.9. Macro- and micronutrients measurement
A 1 g of freeze-dried plant sample was weighted in digestion tube to which 10 cm3 HNO3 69% (VWR International Ltd., Radnor, PA, USA) was added and digested for 30 min at 60°C in a Labor MIM OE 718/A electric block digestion unit. After cooling of the samples, 10 cm3 H2O2 is added and the samples are further digestied for 90 min at 120°C. After digestion and cooling of them, the volume of the digested liquids were competed to 50 cm3 using ultrapore water (Milli Q two-level water purification system, Millipore S.A.S, Molsheim, France), and then filtered using MN 640 W filter paper. The macro- and microelement contents of digested samples were measured by inductively coupled plasma optical emission spectrometry (ICP-OES; Thermo Scientific iCAP 6300 Dual view). The instrument parameters together with the performance characteristics (limit of detection, accuracy, repeatability, stability) were described in detail in Soós et al. (Citation2019).
2.4. Statistical analysis
Data analysis was performed using Microsoft Excel 2016 and the SPSS 25.0 software package (SPSS Inc., Chicago, IL, USA). One-way ANOVA was conducted independently for both the green juice and fruits of pepper varieties and n = 3–10. Post-hoc tests, specifically Tukey's test, were utilized to compare means, and significant differences were determined at the p ≤ 0.05 level. The data were presented as mean ± standard deviation. Data of anatomical analysis were evaluated by independent sample t-test (p ≤ 0.05) and n = 80.
3. Results
3.1. Fresh weight of fruits, leaves, stem, and roots of pepper varieties
The harvest date is set so that at least 80% of the fruit is ready for consumption ((E,F)). Based on these fresh weight of harvested fruits varied significantly between the two varieties of pepper (). The HES variety exhibited higher fresh weights of green and red fruits as well as the total fruit yield than the HEH. For instance, fresh weights of HES’ green, yellow, red, and total fruits were 103.6, 61.9, 217.8, and 383 g/plant, respectively. The yield of red HES fruits was significantly higher in the same age individuals compared with HEH fruits. Otherwise, HEH variety revealed similar fresh weights of green leaves and stem as the HES, recording 100 and 66 g/plant while HES had 97.5 and 57 g/plant, respectively. When the yield of the total fruits and stem are evaluated in relation to each other, the results show that the ratio of fruits: stem is 1.55: 1 in case of HEH and 2.48: 1 for HES. The HES variety showed higher root fresh weight (111.1 g/plant) than the HEH variety (87 g/plant).
Figure 2. Fresh weight (g/plant) of fruits (green, yellow, and red), leaves, stem, and roots of two green pepper varieties, i.e. Hungarian Enigma Hot (HEH) and Hungarian Enigma Sweet (HES). Different letters on the bars of the one parameter are significant according to the Tukey’s test (P ≤ 0.05). Data are Means ± SD and n = 10.
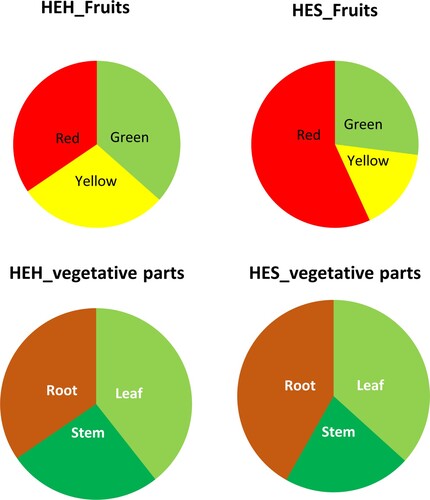
3.2. Fruit pericarp anatomy of green pepper variteies
The main structural characteristics of the pericarp ((A,D)) of both cultivars (HES, HEH) are the same. The exocarp (outer epidermis) is monolayered and covered by a continuous layer of cuticle. The outer cell walls of the epidermis are thicker than the other cell walls. The cuticle layer of the HEH is significantly thicker (P < 0.001), as is the combined thickness of cuticle and outer cell wall (P = 0.024). There was no significant difference in the thickness of the epidermis (P = 0.680). The mesocarp is composed of a subepidermal collenchyma (hypodermis) and a parenchyma with a large lumen of cells, the innermost cell line of which is composed of giant cells (Tiwary et al. (Citation2013) classify the giant cells as endocarp). The collenchyma is significantly thicker in HEH (P = 0.009), consisting of 4–6 thick-walled cell layers, in contrast to HES where only 2–4 cell layers are identified. Collenchyma is essentially a tangential thickening, with rare co-occurrence of tangential and angular collenchyma in HEH. Intercellularity is present between parenchyma cells, cell size does not change significantly towards the endocarp, vascular bundles are embedded between cells ((B,E)). Fine parenchyma bridges are wedged between giant cells. The parenchyma is more than twice as thick as in HES (). The total thickness of the mesocarp is significantly greater than in HEH (4.48 mm vs. 2.06 mm; P < 0.001), mainly due to the high number of cell rows and the thickness of the cell wall. The endocarp (inner epidermis) is monolayered and significantly thicker than in the HES (P = 0.011). It contains two cell types: scherenchymatic cells (adjacent to the giant cells) and parenchymatic cells (adjacent to the parenchymal bridges) ((C,F)).
Figure 3. Fruit pericarp anatomy in two Capsicum annuum cultivars ‘Hungarian Enigma Sweet' (A,B,C) and ‘Hungarian Enigma Hot’ (D,E,F) based on light microscopy in transverse section. Pericarp transverse sections (A-D), Exocarp and collenchyma (hypodermis) (B-E), Giant cells and single layer endocarp (C-F). ex-exocarp, co-collenchyma, p-parenchyma, gc-giant cell, en-endocarp, pc-parenchymatic cells in endocarp, sc-schlerenchymatic cells in endocarp. Scale bars: A 500 μm, B 200 μm, C-D 50 μm, E-F 100 μm.
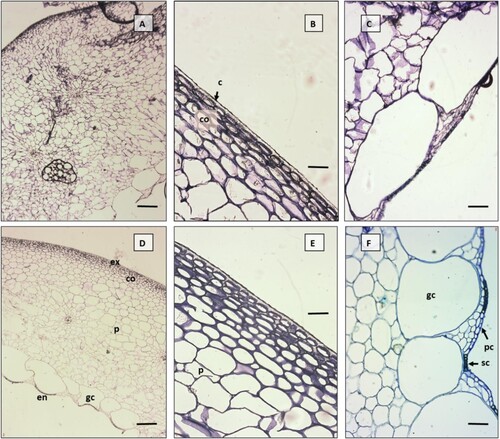
Table 2. Histological observations of fruits pericarp of two Capsicum annuum cultivars, i.e. Hungarian Enigma Sweet (HES) and Hungarian Enigma Hot (HEH).
3.3. Non-enzymatic antioxidant capacity in green juice and fruits of pepper varieties
The non-enzymatic antioxidant capacity varied significantly according to plant variety, plant organs and colors of fruits (). Overall, green juices of HEH and HES exhibited higher total polyphenol content (TPC) than fruits. Fruits of HES displayed higher TPC than HEH; however, red and yellow fruits revealed higher TPC than green fruits in both varieties. Green juice of HEH and HES varieties exhibited the highest content of TPC of 33.3 and 33.5 mg/g, followed by yellow (32.6 mg/g) and red (31.8 mg/g) fruits of HES, and green fruits of HEH had the lowest TPC (24.9 mg/g). Likewise, the total flavonoid content (TFC) peaked in green juices compared to fruits. However, the HES's green juice showed higher TFC (43.19 mg g−1) than the HEH's green juice (28.91 mg/g). Fruits of HEH, regardless of their ripening stage, had higher TFC than HES fruits. The TFC of HEH fruits decreased as the fruits ripened, where the green fruits had the highest TFC while the red fruits possessed the lowest.
Figure 4. Non-enzymatic antioxidant capacity in green juice and fruits (green, yellow, and red) of two pepper varieties, i.e. Hungarian Enigma Hot (HEH) and Hungarian Enigma Sweet (HES), (A) total polyphenol content (TPC); (B) total flavonoid content (TFC); (C) water-soluble antioxidant capacity (ACW); and (D) lipid-soluble antioxidant capacity (ACL). Different letters on the same bars are significant according to the Tukey’s test (P ≤ 0.05). Data are Means ± SD and n = 3.
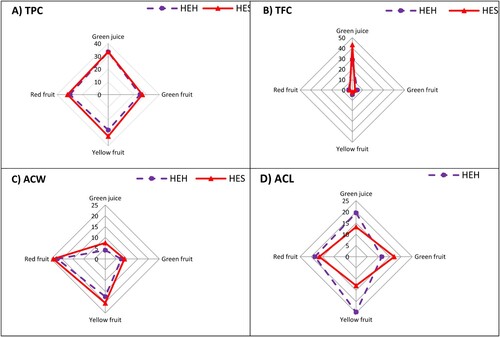
Unlike TPC and TFC, the water-soluble antioxidant capacity (ACW) was higher in pepper fruits than the green juices. Furthermore, HES’s fruits, regardless of their ripening stage, displayed higher ACW contents than the HEH’s fruits. Noticeably, the ACW content increased as the fruits of HEH and HES ripened. Red fruits of HES had the highest ACW content (24.03 µg/mg ascorbic acid), followed by HEH’s red fruits (22.19 µg/mg ascorbic acid). The green juice of HES revealed higher ACW content (7.45 µg/mg ascorbic acid) than the HEH’s green juice (4.08 µg/mg ascorbic acid). On the other hand, the lipid-soluble antioxidant capacity (ACL) peaked in fruits and green juice of HEH variety, where the highest ACL content (24.7 µg/mg trolox) corresponded to the HEH’s yellow fruits (24.70 µg/mg trolox), followed by HEH’s green juice (19.56 µg/mg trolox). The green and red fruits of HES exhibited higher ACL contents (17.00 and 16.40 µg/mg trolox, respectively) than the HES's green juice (13.27 µg/mg trolox), while yellow fruits of HES had the lowest ACL content.
3.4. Vitamin contents in green juice and fruits of pepper varieties
The ripening stage of pepper fruit, pepper variety, and plant tissue affected the contents of vitamins. Among the water-soluble vitamins, the quantitative changes of nicotinic acid, nicotinamide and biotin were measured. Biotin was below the detection limit (<1 µg/g) in all samples tested therefore is not shown. The concentration of nicotinic acid in the fruits varied between 3.94 and 6.64 µg/g. For HEH, a significant decrease was observed in the nicotinic acid changes as the fruit ripened from green to red, while for the HES variety the results hesitated ((A)). Nicotinamide was measured at much lower concentrations (0.62 - 1.09 µg/g) regardless of cultivars than nicotinic acid. For all varieties, the nicotinamide concentrations hesitated in fruits from green to red ripening phase ((B)). The nicotinamide contents in green juices of both varieties were much lower than those reported for fruits; however, the nicotinic acid content in green juice was higher than in fruits. Green juice of HEH variety displayed higher nicotinamide contents than the HES variety, whereas nicotinic acid in green juice of HES was higher than in HEH. The highest nicotinic acid, nicotinamide contents were 8.44 and 1.32 µg/g respectively, and corresponded to green juice of HES, yellow fruits of HEH, and green fruits of HEH.
Figure 5. Contents of: (a) nicotinic acid (µg/g), (b) nicotinamide (µg/g), and (c) vitamin C (mg/g) in green juice and fruits (green, yellow, and red) of two pepper varieties, i.e. Hungarian Enigma Hot (HEH) and Hungarian Enigma Sweet (HES), (A) nicotinic acid; (B) nicotinamide; and (C) vitamin C. Different letters on the same bars are significant according to the Tukey’s test (P ≤ 0.05). Data are Means ± SD and n = 3.
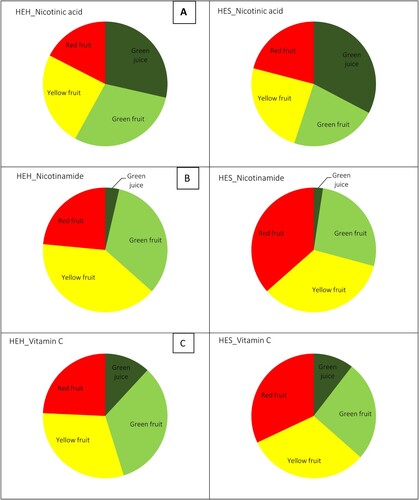
3.5. Photosynthetic pigments in fruits and green juices of pepper varieties
The chlorophyll a content decreased significantly as the fruits of HEH or HES ripened (). Green fruits exhibited the highest chlorophyll a content, while the red ones had the lowest. Overall, fruits of the HEH variety revealed higher chlorophyll a contents than the HES variety. For instance, green fruits of HEH and HES had chlorophyll a contents of 0.773 and 0.210 mg/g, respectively. Also, significantly higher chlorophyll a contents were measured in green juice compared to the fruits. Although HEH’s fruits revealed higher chlorophyll a contents than HES’s fruits, the green juice of HES showed significantly higher chlorophyll a content than HEH. The chlorophyll a contents in green juices of HEH and HES were 7.389 and 7.906 mg/g.
Table 3. Photosynthetic pigments (mg/g DW) in green juice and fruits (green, yellow, and red) of two pepper varieties, i.e. Hungarian Enigma Hot (HEH) and Hungarian Enigma Sweet (HES).
Similarly, green fruits had the highest chlorophyll b contents in both varieties, while the red fruits had the lowest values. Also, green juices exhibited higher chlorophyll b contents than fruits. Fruits of HEH had higher chlorophyll b contents than the HES, whereas HES’ green juice displayed higher chlorophyll b content than the HEH. The chlorophyll b content in HEH’s green fruits was 0.675 mg/g and dropped to 0.325 mg/g after turning fruits into red. Likewise, the chlorophyll b content of HES fruits reduced from 0.253–0.151 mg/g up on the fruit ripening (change from green to red). On the other side, the chlorophyll b content in the green juice of HES was 6.222 mg/g, which was significantly higher than HEH (5.708 mg/g). The different carotenoid types were measured separately in green juices processed from green leaf shoots. Among the separated carotenoid forms, lutein was the most abundant (997–1065 µg/g DW), regardless of the cultivar (). Along with it, the lutein content of HES was significantly higher compared to HEH. Capsanthin and β-Cryptoxanthin were present in negligible amounts.
3.6. Carotenoids speciation
Of the seven quantified carotenoid derivatives, only lutein and β-carotene were detected in all three colors of the two pepper varieties (). Among these, β-carotene showed a clear marked increase in fruits turned the color from green to red regardless of cultivars. While the lutein content was found to be hesitated in green, yellow and red fruits for both HEH and HES. Violaxanthin was quantified in red fruits of both varieties as well as green fruits of HES. Capsanthin was identified in yellow and red fruits of both varieties only. Zeaxanthin was found in yellow fruits of HEH and HES only ranged between 0.34–0.99 mg/g. Similarly, α-carotene was measured only in yellow fruits of HES. Only, β-cryptoxanthin appeared in red fruits of HES (1.99 mg/g). Overall, HES variety showed more carotenoid derivatives than the HEH (). In general, red fruits had higher capsanthin contents than yellow fruits; however, HEH showed higher contents than the HES. Overall, the content of lutein, β-carotene, violaxanthin, capanthin and α-carotene together showed an increasing trend in the fruits during ripening.
Table 4. Carotenoid speciation (mg/g DW) in fruits (green, yellow, and red) of two pepper varieties, i.e. Hungarian Enigma Hot (HEH) and Hungarian Enigma Sweet (HES).
3.7. Total nitrogen, protein, and carbon contents in fruits and green juices of pepper varieties
Overall, fruits and green juices of the HES variety displayed higher nitrogen content than their correspondents of the HEH (). Moreover, nitrogen content significantly diminished as the fruits ripened, where the green fruit exhibited the highest nitrogen content, and red fruits had the lowest nitrogen content. Green juices had about 3 times higher nitrogen content than fruits. The highest nitrogen content in green juice (6.25%) corresponded to the HES, whereas the highest nitrogen content in fruits (2.04%) corresponded to the green fruits of the HES variety.
Figure 6. (#A) Total nitrogen content; (B) total protein content; and (C) carbon content in green juice and fruits (green, yellow, and red) of two pepper varieties, i.e. Hungarian Enigma Hot (HEH) and Hungarian Enigma Sweet (HES). Different letters on the same bars are significant according to the Tukey’s test (P ≤ 0.05). Data are Means ± SD and n = 3.
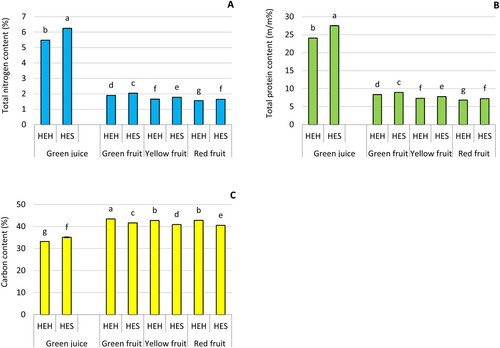
Consistent with the nitrogen content, the HES cultivar had a higher total protein content in fruit and green juice than HEH. During the fruits ripening the total protein content significantly decreased. For insatnce, green fruits of HES had a protein content of 8.96%, while red fruits dropped down to 7.25%. Similarly, green and red fruits of HEH possessed protein contents of 8.36% and 6.85%, respectively. The green juices had about 3 times higher protein contents than fruits. The protein contents in the green juice of HEH and HES were 34.26% and 39.07% ().
The carbon content in pepper fruits slightly decreased as the fruits ripened, regardless of the pepper variety (). Also, green juices displayed significantly lower carbon content than fruits. The highest carbon content was 43.4% and corresponded to the green fruits of HEH, while the lowest carbon content in fruits (40.5%) corresponded to the red fruits of HES. The green juices of HEH and HES were 33.20% and 35.11%, respectively.
3.8. Phytochemical profile of fruits and green juices of pepper cultivars
For both pepper cultivars, the phytochemical composition of the green biomass originated green juice and the (underripe) green and (ripe) red fruits was measured by UHPLC-ESI-MS. Up to 55 tentative phytochemicals were revealed, based on specific retention time, accurate mass, isotopic distribution, and fragmentation pattern. The identified compounds belonging to several metabolite types including vitamins, flavonoids, phenolic acids and derivatives, phenolamides/alkylamides, sesquiterpenes, fatty acid/dicarboxylic acids and other metabolites (). Most phytochemical components were detectable both in green juice and in the different ripened fruits. However, there were some specific compounds detected only in green juice, such as apigenin-O-pentosylhexoside, cosmozine (apigenin-7-O-glucoside) and chrisoeryol (3'-methoxy-4’.5.7-trihydroxyflavone), which belong to the family of falvonoids and zizybeoside I which is an organic oxygen compounds.
Table 5. Tentative identificated phytochemicals of green pepper varieties.
In contrast, capsaicinoids were mainly found in fruits such as nordihydrocapsaicin, dihydrocapsaicin, homocapsaicin, homodihydrocapsaicin and ω-hydroxycapsaicin. Green pepper cultivar-specific phytochemicals were also found. Thus, 4 tricoumaroylspermidine isomers were only revealed in the Hungarian Enigma Hot (HEH) variety.
Among the chemical groups, the class of alkylamides/phenolamides proved to be the most diverse, with 20 compounds identified. Apart from capsaicinoides, the hydroxycinnamic acid derivative linked to an aliphatic polyamine via an amide bond was the most common form (). In addition, 13 and 12 compounds of the widely distributed flavonoids and phenolic acids in the plant kingdom have been identified. The quantity of several of these compounds have been quantified ().
Table 6. Concentrations of some nutritionally important phytochemicals (µg/g DW) detected in green juice and fruits (green, yellow, and red) of two pepper varieties, i.e. Hungarian Enigma Hot (HEH) and Hungarian Enigma Sweet (HES).
3.9. Quantitative changes of phytochemicals in fruits and green juices of pepper cultivars
Fruits and green juices of the two green pepper cultivars exhibited several nutritionally important phytocompounds, which their concentrations varied significantly according to the ripening stage, plant variety, and plant tissues (). Nine key phytochemicals – known for their valuable nutritional properties – were quantified in the fruits and green juices of the two green pepper varieties, i.e. HEH and HES.
Several quantified phytochemicals in fruits, regardless of the pepper variety, decreased as the fruits ripened. For instance, significant decreases in quercetin-3-O-d-glucopyranoside, rutin (quercetin-3-O-rutinoside), caffeic acid and ferulic acid were realized in fruits at different stages of ripening. However, no trend-like changes in other phytochemical components such as p-coumaric acid and synaptic acid were observed in relation to fruit ripening.
To compare the two cultivars of green juices, markedly differences could be realized. Indeed, neochlorogenic acid (5-O-caffeoylquinic acid), p-coumaric acid, luteolin (3’.4’.5.7-tetrahydroxyflavone) and apigenin were found in 9- to 10-fold higher in green juice of HES compared to the HEH cultivar. It is noteworthy that the content of luteolin (7.29 ± 0.16 µg/g), apigenin (4.12 ± 0.26 µg/g), neochlorogenic acid (24.84 ± 1.10 µg/g) and p-coumaric acid (11.24 ± 0.57 µg/g) is much higher in HES green juice than in any of the fruits at the developmental stage.
3.10. Macro and microelement contents in fruits and green juices of pepper varieties
Several macro and microelements have been identified in the green juices and fruits of the two green pepper varieties (). The following elements were quantified in g/kg, K, P, Ca, Mg, and S, while Na, Zn, Fe, Mn, Mo, Cu, Sr, Ti, Al, B, Cd, Co, Cr, and Pb were detected in mg/kg. All the determined elements, except for the Na, revealed higher concentrations in the green juices than in fruits. Concentrations of K, P, Ca, Mg, and S in green juices were 4, 2, 24, 6, and 2-fold higher than in fruits, respectively. The K content in fruits was independent of the ripening stage; however, fruits of HEH recorded slightly higher values than the HES. The green fruits of both varieties displayed higher P contents than the yellow fruits, and red fruits had the lowest P contents; moreover, both varieties had the same P concentrations in fruits. Both varieties exhibited the same Ca contents in fruits; however, green fruits revealed the highest Ca contents, followed by red fruits, and yellow fruits showed the lowest values. The Mg and S concentrations in fruits did vary between HEH and HES, where they recorded the same values; yet, Mg and S concentrations were ripening stage-dependent as they slightly decreased as the fruits ripened. Green juices of both varieties displayed the same concentrations of P, Ca, and S; nevertheless, HEH’s green juice revealed higher K content, while Mg content was significantly higher in the HES’s green juice than the HEH’s green juice.
Table 7. Concentration of macro and microelements in green juice and fruits (green, yellow, and red) of two pepper varieties, i.e. Hungarian Enigma Hot (HEH) and Hungarian Enigma Sweet (HES).
The yellow fruits displayed higher Na contents than the green and red fruits, which had almost the same Na concentrations. The green and yellow fruits of HEH and HES showed higher Zn concentrations than the red fruits. Red fruits of HEH had slightly higher Zn content than the HES’s red fruits. Fe content significantly reduced as the fruits ripened, where the red fruits possessed the lowest Fe content. Fruits of HEH exhibited slightly higher Fe contents than the HES’s fruits. Similar results were reported for Mn, Mo, Cu, Sr, Al, Ti, and B. In fruits, Cd, Co, Cr, and Pb concentrations were very low, regardless of the ripening stage and pepper variety. The green juice of HES revealed significantly higher contents of Zn, Fe, Mo, Cu, Ti, Al, and B than the HEH’s green juice, whereas Mn and Cr were significantly higher in the HEH’s green juice.
4. Discussion
Nutrition is a critical aspect of space exploration, and astronauts face several unique challenges when it comes to maintaining a healthy diet during missions. These challenges stem from the microgravity environment, limited food storage, long-duration missions, and the need to provide balanced nutrition to support overall health and performance (Tang et al. Citation2021). Spacecraft have limited storage space, so the food that astronauts bring must be highly nutrient-dense and lightweight. Foods also need to be shelf-stable and resistant to spoilage over extended periods (Spencer and Hummerick Citation2019). Traditional preservation methods like refrigeration or freezing are not feasible due to the lack of these facilities in space. Therefore, astronauts have conducted experiments to cultivate plants in space stations for food purposes. While these experiments have not yet fully replaced the need for resupplying food from Earth, they are an important step toward developing sustainable food production systems for future long-duration missions, such as those to the Moon, Mars, and beyond.
These experiments aim to study the feasibility of growing fresh, nutritious crops in space and to understand the challenges associated with space (Heiney Citation2019). From this aspect cultivation speices such as pepper has gained an extraordinary attention due to its high nutrient content. Moreover, peppers possess a dual characteristic of being both vibrant and zesty, imparting an added burst of flavor to space cuisine, counteracting occasional blandness resulting from altered taste perceptions in microgravity environments (Pinto et al. Citation2016).
In this study, two determinate-growth green pepper varieties with specific characteristics were evaluated (HEH and HES). Determinate-growth peppers are genetically programmed to decrease growth when flower buds start developing and a trait that is advantageous in space environment. As mentioned in the Materials and Methods part the Hungarian Enigma Hot (HEH) variety is ‘horn’ type pepper. The HEH was selected from ‘Budai csípős’ determinate-growth Hungarian heirloom cultivar. The other observed variety is the Hungarian Enigma Sweet (HES) of the ‘Cecei’ type. This variety was obtained by individual selection from ‘Tizenegyes’ determinate-growth Hungarian heirloom cultivar.
Both pepper variteties were grown in GHE Aeroflow 10 hydroponic system as a ground bioregenerative life-support research facility. Under these conditions, both the HEH and HES varieties were harvested at the same time, on day 125, when at least 80% of the fruits were at consumable ripening stage. In general, the fruit of the ‘Horn’ and ‘Cecei’ varieties, ranging in color from green to yellow to red, are used both for direct consumption and in the canning industry. For this reason, biochemical evaluation of fruits of all three colors was carried out.
The ‘Cecei’ type, to which belongs the HES variety, typically has a thick pericarp and turn to red easily. This was indicated by our results as the red fruits yield at the time of harvest was significantly higher than that of the HEH variety. The anatomical analysis confirmed that the pericarp of the HES fruit is juicy and fleshy due to the thicker parenchyma of the mesocarp and the high number of cell lines. However, the collenchyma, which provides firmness, is thinner than in HEH, as is the cuticle. Together, these characteristics are advantageous from the aspects of digestibility.
Besides fruits, consuming leaf proteins can be an advantage for astronauts for several reasons, especially in the context of space exploration and long-duration missions. Space nutrition must fulfill the daily protein, fat, and sugar needs of individuals, along with essential inorganic elements, trace elements, fat-soluble vitamins, and a variety of water-soluble vitamins. The nutritional requirements for space travel are determined by the World Health Organization (WHO) based on the daily necessities of people on Earth. Consequently, it is recommended that the macronutrient composition include a minimum of 15% protein, 30% lipids, and 55% carbohydrates on average (Trumbo et al. Citation2002; Cooper et al. Citation2011). Leafy greens and other plant-based protein sources offer a range of benefits that contribute to the overall health and well-being of astronauts. Leafy greens contain protein, albeit in smaller amounts compared to animal sources. Including plant-based proteins diversifies the protein sources available to astronauts and can contribute to meeting their protein needs. Leafy greens are also rich in essential nutrients such as vitamins (e.g. vitamin C, vitamin K, folic acid), minerals (e.g. Fe, Ca, Mg), and dietary fiber (Rainey Citation2015). Also space travel exposes astronauts to higher levels of ionizing radiation, which can potentially affect their health, including their nutritional needs. Antioxidant-rich foods and supplements may be necessary to counteract some of the harmful effects of radiation. Leafy greens are abundant in antioxidants and phytochemicals, which have been associated with various health benefits, including reducing inflammation and lowering the risk of chronic diseases. These compounds may help mitigate the effects of radiation and oxidative stress that astronauts may encounter during space missions (Thuphairo et al. Citation2019). Consuming 100 g of fresh bell pepper is sufficient to provide a person with the daily recommended vitamin C requirement (Zhuang et al. Citation2012). The green pepper is interesting because it is not only the fruit that is valuable but also the green leafy shoots. However, the use of green biomass of sweet pepper as food is a neglected area in the literature. For this reason, in present work, special attention was paid to explore the biochemical values of green juice processed from green biomass.
Like other photosynthetic organisms, carotenoids were also represented in the green juices and fruits of HEH and HES. They play a crucial role in photosynthesis, photoprotection, phytohormone synthesis, and stabilization of free radicals (Rosas-Saavedra and Stange Citation2016). At the same time, carotenoids are also essential from human health. They are confirmed to have antioxidant and anticarcinogenic activities and act as precursors of other vital compounds, such as vitamin A and retinoic acid (Eggersdorfer and Wyss Citation2018). Regardless of the cultivars, the total amount of carotenoids increased as the fruits turned from green to red. However, there is a significant difference in the extent of change in individual carotenoid compounds between HEH and HES. β-carotene was more abundant in green and yellow fruits of HEH than in HES. Red-colored casphanthin was below the detection limit in green fruits and followed the trend of quantitative change of β-Carotene in yellow fruits. It was almost 2-times higher in the HEH. This could explain that the carotenoid profile changes differently during ripening depending on the genotype, although it is generally associated with an increase in total carotenoid content, which could be up to 10–90 times higher (Antonio et al. Citation2018). As mentioned above, the green HES fruit was pale green, almost white, and the attached photos support this visual evidence ().
In the red ripe fruits, β-Carotene represented 56% of the total carotenoids in the case of the HES cultivar, while in the HEH, it represented 43%. In comparison, there is not much difference, but in absolute terms, this implies almost double the amount (10.57 mg/g) in HES and (5.33 mg/g) in HEH. The β-carotene has a protective effect against sunburn and is the precursor of retinal and vitamin A (Anaya-Esparza et al. Citation2021). Interestingly, β-cryptoxanthin was only detected in the red HES fruit at 1.99 mg/g. It is noteworthy that β-cryptoxanthin may play a role in bone homeostasis by altering gene expression of proteins involved in bone formation and mineralization of osteoblast cells. The hot HEH cultivar had 42% of this red pigment, while the sweet HES cultivar had only 24%. In red Lamuyo-type sweet peppers, capsanthin was the most abundant carotenoid (9667 μg/100 g) (Rodríguez-Rodríguez et al. Citation2020). Kim et al. (Citation2021) compared 11 varieties with capsantin ranging from 89 to 110 μg/ g, and the lutein was found to be 12.5 ug/g. Lutein, zeaxanthin, and the meso-zeaxanthin isomer are found in the human retina. They are concentrated in the macula and are known as macular pigment (Eggersdorfer and Wyss Citation2018). It is well-known that solar and cosmic radiations are special environmental hazards that cause cataracts and retinal degeneration, in the prevention of which lutein and zeaxanthin play an essential role. Rodríguez-Rodríguez et al. (Citation2020) measured 1202 μg/100 g lutein. In our finding much higher lutein (250–260 μg/ g) measured in both cultivars, We found much higher lutein content (250–260 μg/g) in both varieties, which was still a significant decrease compared to the lutein content of green and yellow fruits.
Green juice is also a rich source of free-form carotenoids. Of these, lutein was the predominant form, regardless of HEH or HES; however, zeaxanthin was also detected at lower concentrations.
Green peppers contain a wide range of valuable metabolites, too. Many of these have already been identified, but the focus has been mainly on measuring the fruit. However, the present study also paid attention to exploring metabolites in green biomass, as well as to the traits of pepper fruits potentially suitable for the BLSS system. The hydro-alcoholic solvent mixture successfully extracted polar and semi-polar metabolites of green pepper samples using the UHPLC-ESI-MS technique.{Citation}
In phenolamides/ alkylamides as the chief metabolite group, 22 metabolites were identified in green juices and fruits (). In detail, the hydroxycinnamic acid amines are widely distributed in specific plant metabolites (Roumani et al. Citation2020). From these cis/trans-caffeoylputrescines, cis/trans-coumaroylputrescines, cis/trans-feruloylputrescines, and trans-feruloyltyramine were presented in green juice and fruits of HEH and HES. Similarly, Evidente and Masi (Citation2021) described the natural bioactive compounds, i.e. N-trans-feruloyltyramine, N-cis-coumaroyltyramine, and N-trans-coumaroyltyramine, from root of pepper. The bioactivities of phenolamides have been known for several decades and are now mainly of interest in an ethnopharmacological context. Masi et al. (Citation2021) confirmed the selective toxicity of N-p-coumaroyltyramine to A431 and HeLa cancer cells, respectively. Other pharmacological properties, such as the protective effects of phenolamides against metabolic syndrome and neurodegenerative diseases have also been confirmed (Roumani et al. Citation2020). Besides these, the hydroxycinnamate-tyramine conjugates play key role in plant defense against attacks by plant pathogens and herbivores (Pearce et al. Citation1998). Alkylamides are known to be present in the Solanaceae family (Elufioye et al. Citation2020). The pungent and tingling sensations of different plant organs are attributed to alkylamides, such as the capsaicinoids. The group of capsaicinoids was identified by positive ionization in the fruits of both hot and sweet pepper varieties. Also, Kelebek et al. (Citation2020) identified capsaicinoids by LC-DAD/ESI-MS-MS using positive ionization, but they only found capsaicin and dihydrocapsaicin in sweet Capia and hot Aleppo varieties. In the latter, higher concentrations were measured. In the present study, the HEH cultivar displayed higher capsaicinoids contents than the HES.
After phenolamides/alkylamides, flavonoids and their glycoside derivatives were the most abundant metabolite group in the observed varieties. Among the 13 flavonoids, primarily apigenin, quercetin, and luteolin aglycones and their derivatives with hexoside moieties could be identified similarly to those described in the literature (Morales-Soto et al. Citation2013; Kelebek et al. Citation2020; Darko et al. Citation2022). According to Antonio et al. (Citation2018), quercetin and luteolin may represent ∼41% of the total flavonoids in Capsicum. Interestingly, the apigenin-O-pentosylhexoside, cosmosin (apigenin-7-O-glucoside), and chrysoeriol (3'-methoxy-4’.5.7-trihydroxyflavone), which belong to the Flavones, were not found in the fruits, only in the green juice from green biomass. The quantitative changes of flavonoids during fruit ripening show a contradictory profile compared to carotenoids and capsaicinoids (Antonio et al. Citation2018). This is confirmed by the signifiant increase of most carotenoid forms, while flavonoids remained unchanged or decreased. Indeed, rutin was 6.809 µg/g DW in the underripe green fruits of the HEH variety, while 3.656 µg/g DW was in the ripe red fruits. Given the widely studied/confirmed biological and human health properties of rutin, this should be emphasized (Damin et al. Citation2019).
Considering the quantitative changes in apigenin and luteolin, green juice was found to be more valuable than fruit, especially in the HES variety (.). In addition, a similar tendency was apparent for neochlorogenic acid and p-coumaric acid, which belong to the phenolic acids. These results also confirm the importance of using green biomass as food. The processing of green plant biomass from different origins and the use of valorized products for food purposes is of increasing importance due to the valuable proteins and other phytochemical components they contain. From this approach evaluating the green juice of green peppers was found that broccoli green juice has lower apigenin (0.15 µg/g DW) and luteolin (0.25 µg/g DW) content, while leaf protein concentrate from alfalfa green biomass is richer in apigenin (11.25 - 25 µg/g DW) but luteolin is similar (0.84 - 2.45 µg/g DW) (Domokos-Szabolcsy et al. Citation2022; Kovács et al. Citation2023). In addition to groups containing a larger number of metabolites, we were able to identify one sesquiterpene. A sesquiterpene co-metabolite, Canusesnol I, was detected only in the green juice of HEH. Previously, Kawaguchi et al. (Citation2004) identified 10 canusesnols (A - J) from the stems and roots of pepper.
Furthermore, up to our knowledge, this is the first identification of zizybeoside [M−H]− ion at m/z 431.15534 from green biomass of green pepper. Zyzylbeoside I belongs to a class of organooxygen compounds known as o-glycosyl compounds, which are quite rare in plants. Space nutrition must fulfill the daily protein demand given its essential importance. Consequently, it is recommended that the macronutrient composition include a minimum of 15% protein.
This paper searches beyond the feasible double benefits of green pepper cultivation as a possible and promising model of a nutrient-dense diet approach. The nutritional value of pepper fruits is well-known; however, fresh green leaves of pepper proved their substantial value. Ripe pepper fruits generally contain low crude protein contents. In the present study, however, relatively high crude protein contents in pepper fruits were noticed, which significantly depended on ripening stage of fruits and plant cultivar ((B)). Overall, green fruits showed the highest crude protein contents of 8.36–8.96% DW, while red fruits displayed the lowest crude protein contents of 6.85–7.25% DW. These values are relatively higher than those documented by Rosa-Martínez et al. (Citation2021), who reported that the crude protein content in ripe fruits of 10 pepper varieties ranged between 0.65% and 1.70% FW with an average of 1.13% FW. Nevertheless, Guilherme et al. (Citation2020) revealed higher crude protein contents in ripe red fruits than the green fruits of pepper, where crude protein contents in red and green fruits were 11.9 and 11.7% DW, respectively. Similarly, the crude protein in ripe fruits of two Spanish pepper varieties varied between 11.4% and 12.0% (Bernardo et al. Citation2008), whereas fruits of three Ethiopian pepper varieties had a crude protein ranged between 8.7% and 11.8% (Esayas et al. Citation2011). Also, a study on variations among 12 pepper cultivars reported that the crude protein contents in red fruits significantly varied from 10.3% to 18.5% DW (Kim et al. Citation2019).
The average recommended daily intake of protein (g/kg of body weight) is 56 g for adult male of 70 kg and 46 g for adult female of 57 kg (Meyers et al. Citation2006). In the present study, consuming 100 g of red and green fruits of HES variety provided males with 12.9% and 16.0%, respectively, and females with 15.8% and 19.5%, respectively, of their daily protein requirements. On the other hand, consuming 100 g of red and green fruits of HEH variety provided males with 12.2% and 14.9%, respectively, and females with 14.9% and 18.2%, respectively, of their daily protein requirements. However, a study reported lower sharing of daily recommended protein intake up on consuming 100 g of pepper fruits, which provided male and female with 2.0% and 2.4%, respectively, of their daily recommended intake of protein (Rosa-Martínez et al. Citation2021). Likewise, consuming 100 g tomato and eggplant were found to fulfill about 0.7–0.9% and 2.7–3.2% of daily recommended intake of protein for male and female, respectively (Rosa-Martínez et al. Citation2021). These results confirm that the fruits of HEH and HES could share higher portion of daily recommended intake of protein for humans than other vegetables such as tomato and eggplant.
The crude protein contents in leaves of six different pepper varieties were largely varied from 1.2–3.5% DW (Amaechi et al. Citation2021). However, green juices obtained by mechanical pressing of green leaves of HEH and HES varieties exhibited higher crude protein contents of 24.1% and 27.5% DW, respectively ((B)). This could be a promising alternative to providing astronauts with an adequate portion of their daily protein needs. For instance, consuming 100 g of HEH and HES’s green juices can supply male astronauts with about 43.0% and 49.2%, respectively, and female astronauts with 52.4% and 59.9%, respectively, of their daily recommended protein intake.
Mineral composition of pepper fruits and leaves can vary depending on factors such as the specific pepper variety, growing conditions, ripening stage, and soil composition. The mineral content in pepper fruits includes elements such as K, Mg, Ca, P, and trace amounts of other minerals like Fe and Zn (Pinto et al. Citation2016). In the present study, K was the most abundant element in fruits, regardless of the ripening stage, and green juices. Also, the mineral composition varied according to the ripening stage of fruits as well as pepper variety (). The mineral composition of sweet pepper fruits slightly changed according to the ripening stage (i.e. green and red). Green fruits exhibited higher K, Zn, and Cu contents than the red fruits, while Ca, P, Fe, Mn, and S contents were higher in the red fruits. Overall, the contents (mg/kg DW) of mineral elements in green and red fruits were as follows: K (35500–32700), Ca (1009–1041), P (1528–1704), Fe (64.7–73.6), Zn (25.0–18.6), Cu (11.6–7.94), Mn (21.3–22.5), and S (1518–1566), respectively (Bernardo et al. Citation2008). In the present study, the mineral composition (mg/kg DW) in pepper fruits varied as follows: K (21500–23100), Ca (400–700), P (3400–4000), Mg (1100–1400), Na (82.3–113.7), S (1100–1300), Fe (52.1–65.6), Zn (10.4–17.5), Mn (16.3–23.0), and Cu (6.6–8.9). However, lower contents of minerals were reported in ripe fruits of 10 pepper varieties, where the contents of macro and micro-elements (mg/kg FW) considerably varied, recording K (616–1364), Na (14–53), Mg (79–189), Ca (74–167), P (311–584), Fe (2.91–7.95), Zn (1.39–3.93), and Cu (0.35–1,40) (Rosa-Martínez et al. Citation2021). Bell peppers (paprika) are characterized by low protein content but they contain appreciable amounts of several minerals such as K, Na, Mg, Ca, and P (Anaya-Esparza et al. Citation2021), which substantially supply humans with indispensable nutrients. Likewise, in their comparative study on five dwarf pepper varieties, Spencer and Hummerick (Citation2019) reported that K was the highest mineral measured in the ripe fruits, followed by Mg and Ca, respectively, while Fe was below the detectable limit. Among the five cultivars, the contents of K, Ca, and Mg (g/kg DW) ranged between 17.5–20.5, 0.99–1.54, and 0.57–2.01, respectively. Contents of macro and microelements in red fruits of 12 different pepper cultivars significantly differed. Also, K was the most abundant macroelement, followed by P, Mg, and Ca, respectively. The variations in elemental composition (mg/kg DW) were as follows: K (20210–64000), Na (132–478), Mg (960–2300), Ca (184–1640), P (2090–4120), Fe (41–107), Zn (120–457), Cu (3.3–55.0), S (974–7120), and Mn (3.2–17.4) (Kim et al. Citation2019).
Pepper leaves includes several macroelements such as K, Ca, Mg, P, and trace minerals like Fe, Zn, Cu, Mn, and Mo (Pinto et al. Citation2016). Several macro and microelements have been detected in leaves of the six pepper varieties, from which K was the highest, followed by Na, Mg, Ca, P, Fe, and Zn was the lowest. The concentrations of these elements (mg/kg DW) significantly varied among pepper varieties; for instance, K (735–952), Na (755–874), Mg (345–745), Ca (313–433), P (239–450), Fe (27.2–57.7), and Zn (13.7–24.7) (Amaechi et al. Citation2021). In the current study, green juices of the fresh biomass of the two pepper varieties (i.e. HEH and HES) revealed appreciable amounts of many macroelements with concentrations even higher than those reported in pepper fruits. The most abundant element was K, followed by Ca, Mg, and P, respectively, and considerable amounts of several microelements such as S, Fe, Na, Zn, Cu, Mn, and Mo ().
The daily recommended dose (mg per day) for adult (male of 70 kg or female of 57 kg) of some minerals is as follows: K (4700), Ca (1000), Mg (400), P (700), Na (1500), Zn (11), Fe (8), and Cu (0.9) (Meyers et al. Citation2006). An astronaut can get about 91%, 62%, 112%, 53%, 0.28%, 63%, 123%, and 65%, of the daily recommended dose of K, Ca, Mg, P, Na, Zn, Fe, and Cum respectively, by consuming 50 g DW of green juices of HEH and HES varieties (on average). These results clearly proved the marked nutritional value of the tested two uncommercial pepper varieties (HEH and HES) and their possible cultivation in space, providing astronauts with appreciable amounts of their needed nutrients.
While leafy greens have several advantages, it's important to note that space environments come with unique challenges, such as limited space, resources, and access to sunlight for plant growth. Researchers and space agencies continue to explore innovative ways to cultivate and incorporate fresh produce like leafy greens into astronaut diets to maximize their nutritional benefits and support the health and performance of astronauts during space missions. Ultimately, addressing the nutritional challenges posed by limited storage space and the need for balanced nutrition is crucial for ensuring the health, well-being, and optimal performance of astronauts during space missions.
5. Conclusion
The phytochemical composition revealed significant differences when comparing the two dedicated cutlivars. The results disclose the importance of cultivars in metabolites of green pepper. It can be concluded that the Whole-Body-Edible peppers (WBE) conception achieved in BLSS system may works due to the valuable phytonutrients, metabolites. Together with this, further biochemical analyses should be performed to exclude the presence of antinutritional substances (such as cyanides). Summarizing the above investigations, it seems that to get special and WBE space pepper cultivars with appropriate volume utilization efficiency, and/or to develop special space cultivation protocols for peppers, it would be one of the forthcoming research ideas to further studies. We strongly believe that the use of our HEH and HES pepper varieties studied by this experiment can be a successful starting point in the future, i.e. for special WBE space pepper breeding programs. We also think that to achieve these goals, the involvement of different biotechnological methods as well as close international scientific cooperation will also be promising opportunities. These future researches will not only offer some useful food safety solutions for the forthcoming Moon and Mars missions, however, i.e. for the development of advanced new terrestrial vertical pepper cultivation systems linked closely to the building-integrated horticulture.
Acknowledgements
This research has been implemented with the TKP2021-EGA-20 support provided from the National Research, Development and Innovation Fund of Hungary, financed under the TKP2021-EGA funding scheme. The present work is also supported by the TKP2020-NKA-04; DE-SPACE project which is co-financed by National Research, Development and Innovation Fund of Hungary. The authors would like to thank Dr. Judit Remenyik and Dr. Erzsébet Szőllősi for their help in carotenoid measurements.
Disclosure statement
No potential conflict of interest was reported by the author(s).
Additional information
Notes on contributors
Éva Domokos-Szabolcsy
Éva Domokos-Szabolcsy, Associate professor at the University of Debrecen. She focuses on isolation and characterization of leaf protein from different green sources, including biomass plants and agro-wastes. She is also interested in studying the effect of several nanoparticles on plant growth and protein patterns in plants.
Tarek Alshaal
Tarek Alshaal, Assistant professor at the Kafrelsheikh University and senior research fellow at the University of Debrecen. His research interests are soil fertility, plant nutrition, soil-plant-microbes interactions, role of nano-sized elements in enhancing plant growth, particularly under abiotic stress.
Judit Koroknai
Judit Koroknai, Departmental engineer at the University of Debrecen. As a research assistant, for a long time, she has been involved in the breeding and rearing procedures of various peppers, also in the research of the possibilities of using the pepper crop and biomass.
Szilvia Kovács
Szilvia Kovács, Assistant lecturer at the University of Debrecen. She carries out a wide range of basic research on plant organs and tissues, which provide excellent support and complement the results of applied plant breeding/gardening, plant breeding, variety comparison experiments (cuticle thickness, epidermis thickness, glandular hairs, number of stoma, etc). Recently, she is exploring the practical applications of the so-called press fibers as industrial by-products (food, feed, horticultural product development), an integral part of which is the analytical investigation of plant cell walls in different plant species (especially the measurement of lignocelluloses_lignin, hemicelluloses). Her interest is focused on product development in the field of fiber enrichment, where new perspective plant species are tested for food (pasta, biscuits, cheese, meat), feed, growing media or other purposes.
Csaba Tóth
Csaba Tóth, Departmental engineer at the University of Debrecen. He carries out work related to growing green pepper under greenhouse conditions and developing new systems for hydroponics.
Gábor Csilléry
Gábor Csilléry, PepGen Ltd. 1114 Budapest, Bartók B. 42. Hungary. His research interests are Capsicum species, interspecific hybrids, resistance, mutants.
Zsuzsa Jókai
Zsuzsa Jókai, Associate professor at Institute of Food Science and Technology of Hungarian University of Agriculture and Life Sciences. Her main areas of research are mercury speciation analysis, quality assurance of speciation analytical methods, uncertainty estimation, analysis of volatile organic compounds of microorganisms by GC-MS, element analysis of environmental-, food- and water samples using the ICP-OES technique, development of a yeast-based nutritional supplement for zinc and polyphenol supplementation by applying SEC-ICP-MS technique, investigation of polyphenol profile of foods by UHPLC-ESI-MS/MS. She has authored approximately 71 publications, with 13 papers published in D1, Q1 and Q2 journals, the number of independent citations is 170.
Anna Matkovits
Anna Matkovits, PhD student at Institute of Food Science and Technology of Hungarian University of Agriculture and Life Sciences. Her main research interests are investigation of Hungarian acacia honey, determination of polyphenol profile by UHPLC-ESI-MS/MS, sugar profile by HPLC-RI, applying of non-destructive technology (NIR) to detect honey adulteration, determination of botanical origin of honey samples. She has authored 5 publications, with 2 papers published in Q1 journals; the number of independent citations is 5.
Péter Makleit
Péter Makleit, works at the Department of Applied Plant Biology, Institute of Crop Sciences, University of Debrecen, AGTC, Debrecen, Hungary. His research interests are plant-environment interactions, secondary metabolites, abiotic and biotic stress.
Szilvia Veres
Szilvia Veres, Full professor at the University of Debrecen. Her research field is plant physiology/ecophysiology. Examination of the relationships between nutrient supply and photosynthesis as a function of environmental factors. Furthermore, the investigation of environmentally friendly nutrient supply options along abiotic conditions. Study of the components of nitrogen utilization efficiency, its environmental dependence, and its relationship with photosynthetic activity. Furthermore, improving the components of plant water utilization efficiency.
Miklós Gábor Fári
Miklós Gábor Fári, Professor emeritus at the University of Debrecen. His main research areas and achievements are the following: cell-, tissue culture of temperate and tropical horticultural crops. Automation of micropropagation technique of plant and design plant bioreactors. Genetic transformation of horticultural crops (carrot, eggplant, Capsicums, etc.). In vitro haploid of vegetable crops (Capsicums). Isolation of leaf protein concentrates - LPC from green biomass (grasses and alfalfa). Father of the patented Artificial Plant Ovary System (APO, 2009). In collaboration with Prof. L. Márton (University of South Carolina, USA), Prof. Fári is the inventor of the very first synthetic plant production technology based on sustainable somatic embryogenesis of Arundo donax L.
References
- Amaechi NC, Udeogu E, Okoronkwo CU, Irondi CP. 2021. Nutritional and phytochemical profiles of common pepper (Capsicum spp.) foliage consumed as leafy vegetables in Southeast Nigeria. Food Res. 5:136–144. doi:10.26656/fr.2017.5(5).675.
- Anaya-Esparza LM, Mora ZVL, Vázquez-Paulino O, Ascencio F, Villarruel-López A. 2021. Bell peppers (Capsicum annum L.) losses and wastes: source for food and pharmaceutical applications. Molecules. 26:5341. doi:10.3390/molecules26175341.
- Angeli L. 1964. The improvement of bounchy-type paprika. 1:67–79.
- Angeli L. 1971. The use of fasciculate form (determinate habit) in the breeding of new Hungarian pepper varieties. Torino, Italy; p. 150–155.
- Antonio AS, Wiedemann LSM, Veiga Junior VF. 2018. The genus Capsicum: a phytochemical review of bioactive secondary metabolites. RSC Adv. 8:25767–25784. doi:10.1039/C8RA02067A.
- Barna D, Alshaal T, O Tóth I, Cziáky Z, Fári MG, Domokos-Szabolcsy É, Bákonyi N. 2022. Bioactive metabolite profile and antioxidant properties of brown juice, a processed alfalfa (Medicago sativa) by-product. Heliyon. 8:e11655. doi:10.1016/j.heliyon.2022.e11655.
- Bernardo A, Martínez S, Álvarez M, Fernández A, López M. 2008. The composition of two Spanish pepper varieties (Fresno de la Vega and Benavente-Los Valles) in different ripening stages. J Food Qual. 31:701–716. doi:10.1111/j.1745-4557.2008.00229.x.
- Bosland PW, Votava EJ. 2012. Peppers: vegetable and spice capsicums, 2nd ed. Cambridge, MA: CABI.
- Brown AH, Chapman DK, Liu SWW. 1974. A comparison of leaf epinasty induced by weightlessness or by clinostat rotation. BioScience. 24:518–520. doi:10.2307/1296888.
- Cenci IDO, Guimarães BP, Amabile RF, Ghesti GF. 2021. Comparison between barley malt protein quantification methods. Food Science and Technology. 41(suppl 1):213–217. http://doi.org/10.1590/fst.13920.
- Chunxiao X, Hong L. 2008. Crop candidates for the bioregenerative life support systems in China. Acta Astronaut. 63:1076–1080. doi:10.1016/j.actaastro.2008.02.003.
- Cooper M, Douglas G, Perchonok M. 2011. Developing the NASA food system for long-duration missions. J Food Sci. 76:R40–R48. doi:10.1111/j.1750-3841.2010.01982.x.
- Csilléry G, Timár Z, Zatykó L, Márkus F. 2016. Genetic study of the super fasciculate plant-sfx mutant. In: Alain Palloix, editor. Proceedings Of Xvith Eucarpia Capsicum And Eggplant Working Group Meeting In Memoriam. Kecskemét, Hungary; p. 407–410.
- Damin FM, Meinhart AD, Caldeirão L, Filho M, da Silva LC, Constant L, Wagner R. 2019. Determination of rutin in fruits and vegetables in natura. J Food Nutr Res. 58:328–338.
- Darko E, Hamow KA, Marček T, Dernovics M, Ahres M, Galiba G. 2022. Modulated light dependence of growth, flowering, and the accumulation of secondary metabolites in chilli. Front Plant Sci. 13:801656. doi:10.3389/fpls.2022.801656.
- Domokos-Szabolcsy É, Elhawat N, Domingos GJ, Kovács Z, Koroknai J, Bodó E, Fári MG, Alshaal T, Bákonyi N. 2022. Comparison of Wet fractionation methods for processing broccoli agricultural wastes and evaluation of the nutri-chemical values of obtained products. Foods. 11:2418. doi:10.3390/foods11162418.
- Douglas GL, Wheeler RM, Fritsche RF. 2021. Sustaining astronauts: resource limitations, technology needs, and parallels between spaceflight food systems and those on earth. Sustainability. 13:9424. doi:10.3390/su13169424.
- EDEN ISS. 2018. Ground demonstration of plant cultivation technologies and operation in space [accessed 2023 Aug 28] https://eden-iss.net/.
- Eggersdorfer M, Wyss A. 2018. Carotenoids in human nutrition and health. Arch Biochem Biophys. 652:18–26. doi:10.1016/j.abb.2018.06.001.
- Elufioye TO, Habtemariam S, Adejare A. 2020. Chemistry and pharmacology of alkylamides from natural origin. Rev Bras Farmacogn. 30:622–640. doi:10.1007/s43450-020-00095-5.
- Esayas K, Shimelis A, Ashebir F, Retta N, Tilahun B, Haki GD. 2011. Proximate composition, mineral content and antinutritional factors of some capsicum (Capsicum annum) varieties grown in Ethiopia. Bull Chem Soc Eth. 25. doi:10.4314/bcse.v25i3.68602.
- Evidente A, Masi M. 2021. Natural bioactive cinnamoyltyramine alkylamides and co-metabolites. Biomolecules. 11:1765. doi:10.3390/biom11121765.
- Fári M. 1983. Embryo culture: an efficient technique in interspecific hybridization and in breeding of pepper (Capsicum). p. 31–37.
- Fári M. 1986. Pepper (Capsicum annuum L.). In: Bajaj YPS, editor. Crops I. Berlin: Springer; p. 345–362.
- Fári M, Czakó M. 1981. Relationship between position and morphogenetic response of pepper hypocotyl explants cultured in vitro. Sci Hortic. 15:207–213. doi:10.1016/0304-4238(81)90028-5.
- Gateway. 2021. Gateway | NASA. [accessed 2023 Aug 28]. https://www.nasa.gov/gateway/overview.
- Graham T, Wheeler R. 2016. Root restriction: a tool for improving volume utilization efficiency in bioregenerative life-support systems. Life Sci Space Res (Amst). 9:62–68. doi:10.1016/j.lssr.2016.04.001.
- Guilherme R, Reboredo F, Guerra M, Ressurreição S, Alvarenga N. 2020. Elemental composition and some nutritional parameters of sweet pepper from organic and conventional agriculture. Plants. 9:863. doi:10.3390/plants9070863.
- Gunay AL, Rao PS. 1978. In vitro plant regeneration from hypocotyl and cotyledon explants of red pepper (capsicum). Plant Sci Lett. 11:365–372. doi:10.1016/0304-4211(78)90024-X.
- Heiney A. 2019. Growing plants in space. In: NASA. [accessed 2023 Aug 28]. http://www.nasa.gov/content/growing-plants-in-space.
- Herridge L. 2021. Chile peppers start spicing up the Space Station. In: NASA. [accessed 2023 Sep 20]. http://www.nasa.gov/feature/chile-peppers-start-spicing-up-the-space-station.
- Hollingsworth S. 2019. Analysis of seed sanitization, rapid freezer proficiency, and Veg-03 water verification.
- Johnson CM, Boles HO, Spencer LE, Poulet L, Romeyn M, Bunchek JM, Fritsche R, Massa GD, O’Rourke A, Wheeler R. 2021. Supplemental food production with plants: a review of NASA research. Front Astron Space Sci. 8:734343. doi:10.3389/fspas.2021.734343.
- Johnson SP, Tibbitts TW. 1968. The liminal angle of a plagiogeotropic organ under weightlessness. BioScience. 18:655–661. doi:10.2307/1294318.
- Kaszás L, Alshaal T, El-Ramady H, Kovács Z, Koroknai J, Elhawat N, Nagy É, Cziáky Z, Fári M, Domokos-Szabolcsy É. 2020. Identification of bioactive phytochemicals in leaf protein concentrate of Jerusalem artichoke (Helianthus tuberosus L.). Plants. 9:889. doi:10.3390/plants9070889.
- Kaszás L, Kovács Z, Nagy E, Elhawat N, Abdalla N, Domokos-Szabolcsy E. 2018. Jerusalem artichoke (Helianthus tuberosus L.) as a potential chlorophyll source for humans and animals nutrition. EBSS. 2:1–20. doi:10.21608/jenvbs.2018.2942.1022.
- Kawaguchi Y, Ochi T, Takaishi Y, Kawazoe K, Lee K-H. 2004. New sesquiterpenes from Capsicum annuum. J Nat Prod. 67:1893–1896. doi:10.1021/np0305472.
- Kelebek H, Sevindik O, Uzlasir T, Selli S. 2020. LC-DAD/ESI MS/MS characterization of fresh and cooked Capia and Aleppo red peppers (Capsicum annuum L.) phenolic profiles. Eur Food Res Technol. 246:1971–1980. doi:10.1007/s00217-020-03548-2.
- Khodadad CLM, Hummerick ME, Spencer LE, Dixit AR, Richards JT, Romeyn MW, Smith TM, Wheeler RM, Massa GD. 2020. Microbiological and nutritional analysis of lettuce crops grown on the international space station. Front Plant Sci. 11:199. doi:10.3389/fpls.2020.00199.
- Kim E-H, Lee KM, Lee S-Y, Kil M, Kwon O-H, Lee S-G, Lee S-K, Ryu T-H, Oh S-W, Park S-Y. 2021. Influence of genetic and environmental factors on the contents of carotenoids and phenolic acids in red pepper fruits (Capsicum annuum L.). Appl Biol Chem. 64:85.
- Kim E-H, Lee S-Y, Baek D-Y, Park S-Y, Lee S-G, Ryu T-H, Lee S-K, Kang H-J, Kwon O-H, Kil M. 2019. A comparison of the nutrient composition and statistical profile in red pepper fruits (Capsicums annuum L.) based on genetic and environmental factors. Appl Biol Chem. 62:48. doi:10.1186/s13765-019-0456-y.
- Kormos J, Kormos J. 1956. Determinate growth in paprika. Növénytermelés. 5:1–10.
- Kovács Z, Soós Á, Kovács B, Kaszás L, Elhawat N, Razem M, Veres S, Fári MG, Koroknai J, Alshaal T, et al. 2023. Nutrichemical alterations in different fractions of multiple-harvest alfalfa (Medicago sativa L.) green biomass fortified with various selenium forms. Plant Soil. 487:173–195. doi:10.1007/s11104-023-05917-8.
- Krishna De A, editor. 2003. Capsicum: the genus Capsicum. 0 ed.. London, UK: CRC Press.
- Liu Y, Xie G, Yang Q, Ren M. 2021. Biotechnological development of plants for space agriculture. Nat Commun. 12:5998. doi:10.1038/s41467-021-26238-3.
- Lunn GM, Stutte GW, Spencer LE, Hummerick ME. 2017. Recovery of nutrients from inedible biomass of tomato and pepper to recycle fertilizer.
- Maiwald V, Vrakking V, Zabel P, Schubert D, Waclavicek R, Dorn M, Fiore L, Imhof B, Rousek T, Rossetti V, et al. 2021. From ice to space: a greenhouse design for moon or mars based on a prototype deployed in Antarctica. CEAS Space J. 13:17–37. doi:10.1007/s12567-020-00318-4.
- Mariotti F, Tomé D, Mirand PP. 2008. Converting nitrogen into protein—beyond 6.25 and Jones’ factors. Crit Rev Food Sci Nutr. 48:177–184. doi:10.1080/10408390701279749.
- Masi M, Koirala M, Delicato A, Di Lecce R, Mérindol N, Ka S, Seck M, Tuzi A, Desgagné-Penix I, Calabrò V, et al. 2021. Isolation and biological characterization of homoisoflavanoids and the alkylamide N-p-coumaroyltyramine from Crinum biflorum Rottb., an Amaryllidaceae species collected in Senegal. Biomolecules. 11:1298. doi:10.3390/biom11091298.
- Matkovits A, Nagy K, Fodor M, Jókai Z. 2023. Analysis of polyphenolic components of Hungarian acacia (Robinia pseudoacacia) honey; method development, statistical evaluation. J Food Compos Anal. 120:105336. doi:10.1016/j.jfca.2023.105336.
- Meyers LD, Hellwig JP, Otten JJ. 2006. Dietary reference intakes: the essential guide to nutrient requirements. Washington, DC: National Academies Press.
- Mityko J, Andrasfalvy A, Csillery G, Fari M. 1995. Anther-culture response in different genotypes and F1 hybrids of pepper (Capsicum annuum L.). Plant Breed. 114:78–80. doi:10.1111/j.1439-0523.1995.tb00764.x.
- Moóor A, Zatykóo L. 1995. Results of pepper breeding in Hungary. Acta Hortic. 88–91. doi:10.17660/ActaHortic.1995.412.8.
- Morales-Soto A, Gómez-Caravaca AM, García-Salas P, Carretero AS, Fernández-Gutiérrez A. 2013. High-performance liquid chromatography coupled to diode array and electrospray time-of-flight mass spectrometry detectors for a comprehensive characterization of phenolic and other polar compounds in three pepper (Capsicum annuum L.) samples. Food Res Int. 51:977–984. doi:10.1016/j.foodres.2013.02.022.
- Mortimer JC, Gilliham M. 2022. SpaceHort: redesigning plants to support space exploration and on-earth sustainability. Curr Opin Biotechnol. 73:246–252. doi:10.1016/j.copbio.2021.08.018.
- NASA, DLR. 2021. Showcase results of test run for plant cultivation on the moon and mars. In: SpaceQuip Journal. [accessed 2023 Aug 28]. https://www.spacequip.eu/2021/12/09/nasa-and-dlr-showcase-results-of-test-run-for-plant-cultivation-on-the-moon-and-mars/.
- Nemes A, Szőllősi E, Stündl L, Biró A, Homoki JR, Szarvas MM, Balogh P, Cziáky Z, Remenyik J. 2018. Determination of Flavonoid and proanthocyanidin profile of Hungarian sour cherry. Molecules. 23:3278. doi:10.3390/molecules23123278.
- Patterson RL. 2011. Description, operation and production of the South Pole food growth chamber (SPFGC) [master’s thesis]. Tucson: University of Arizona.
- Patterson RL, Giacomelli GA, Kacira M, Sadler PD, Wheeler RM. 2012. Description, operation and production of the South Pole food growth chamber. Acta Hortic. 589–596. doi:10.17660/ActaHortic.2012.952.75.
- Pearce G, Marchand PA, Griswold J, Lewis NG, Ryan CA. 1998. Accumulation of feruloyltyramine and p-coumaroyltyramine in tomato leaves in response to wounding. Phytochemistry. 47:659–664. doi:10.1016/S0031-9422(97)00620-1.
- Pinto CMF, Dos Santos IC, De Araujo FF, Da Silva TP. 2016. Pepper importance and growth (Capsicum spp.). In: Ramalho do Rêgo E, Monteiro do Rêgo M, Luiz Finger F, editors. Production and breeding of chilli peppers (Capsicum spp.). Cham: Springer International Publishing; p. 1–25.
- Popov IN, Lewin G. 1994. Photochemiluminescent detection of antiradical activity: II. Testing of nonenzymic water-soluble antioxidants. Free Radical Biol Med. 17:267–271. doi:10.1016/0891-5849(94)90082-5.
- Rainey K. 2015. Crew members sample leafy greens grown on space station. In: NASA. [accessed 2023 Aug 28]. http://www.nasa.gov/mission_pages/station/research/news/meals_ready_to_eat.
- Rodríguez-Rodríguez E, Sánchez-Prieto M, Olmedilla-Alonso B. 2020. Assessment of carotenoid concentrations in red peppers (Capsicum annuum) under domestic refrigeration for three weeks as determined by HPLC-DAD. Food Chem: X. 6:100092. doi:10.1016/j.fochx.2020.100092.
- Romeyn MW, Spencer LE, Massa GD, Wheeler RM. 2019. Crop readiness level (CRL): a scale to track progression of crop testing for space. Boston (MA: 49th International Conference on Environmental Systems.
- Rosa-Martínez E, García-Martínez MD, Adalid-Martínez AM, Pereira-Dias L, Casanova C, Soler E, Figàs MR, Raigón MD, Plazas M, Soler S, et al. 2021. Fruit composition profile of pepper, tomato and eggplant varieties grown under uniform conditions. Food Res Int. 147:110531. doi:10.1016/j.foodres.2021.110531.
- Rosas-Saavedra C, Stange C. 2016. Biosynthesis of carotenoids in plants: enzymes and color. In: Stange C, editor. Carotenoids in nature. Cham: Springer International Publishing; p. 35–69.
- Roumani M, Duval RE, Ropars A, Risler A, Robin C, Larbat R. 2020. Phenolamides: plant specialized metabolites with a wide range of promising pharmacological and health-promoting interests. Biomed Pharmacother. 131:110762. doi:10.1016/j.biopha.2020.110762.
- Salisbury FB, Clark MA. 1996a. Choosing plants to be grown in a controlled environment life support system (CELSS) based upon attractive vegetarian diets. Life Support Biosph Sci. 2:169–179.
- Salisbury FB, Clark MAZ. 1996b. Suggestions for crops grown in controlled ecological life-support systems, based on attractive vegetarian diets. Adv Space Res. 18:33–39. doi:10.1016/0273-1177(95)00859-D.
- Singleton VL, Rossi JA. 1965. Colorimetry of total phenolics with phosphomolybdic-phosphotungstic acid reagents. Am J Enol Vitic. 16:144–158. doi:10.5344/ajev.1965.16.3.144.
- Soós Á, Bódi É, Várallyay S, Molnár S, Kovács B. 2019. Mineral content of propolis tinctures in relation to the extraction time and the ethanol content of the extraction solvent. LWT. 111:719–726. doi:10.1016/j.lwt.2019.05.090.
- Spencer LE, Hummerick ME. 2019. Dwarf tomato and pepper cultivars for space crops. Boston (MA: 49th International Conference on Environmental Systems.
- Tang H, Rising HH, Majji M, Brown RD. 2021. Long-term space nutrition: a scoping review. Nutrients. 14:194. doi:10.3390/nu14010194.
- Thuphairo K, Sornchan P, Suttisansanee U. 2019. Bioactive compounds, antioxidant activity and inhibition of key enzymes relevant to Alzheimer’s disease from sweet pepper (Capsicum annuum) extracts. Prev Nutr Food Sci. 24:327–337. doi:10.3746/pnf.2019.24.3.327.
- Tiwari A, Vivian-Smith A, Ljung K, Offringa R, Heuvelink Ep. 2013. Physiological and morphological changes during early and later stages of fruit growth in Capsicum annuum. Physiol Plantarum. 147:396–406.
- Trumbo P, Schlicker S, Yates AA, Poos M. 2002. Dietary reference intakes for energy, carbohydrate, fiber, fat, fatty acids, cholesterol, protein and amino acids. J Am Diet Assoc. 102:1621–1630. doi:10.1016/S0002-8223(02)90346-9.
- Wheeler RM. 2017. Agriculture for space: people and places paving the way. Open Agric. 2:14–32. doi:10.1515/opag-2017-0002.
- Wheeler RM, Sager JC, Prince RP, Knott WM, Mackowiak C, Stutte G, Yorio NC, Ruffe LM, Peterson BV, Goins G. 2003. Crop production for advanced life support systems - observations from the Kennedy Space Center Breadboard Project.
- Zabel P, Zeidler C, Vrakking V, Dorn M, Schubert D. 2020. Biomass production of the EDEN ISS space greenhouse in Antarctica during the 2018 experiment phase. Front Plant Sci. 11:656. doi:10.3389/fpls.2020.00656.
- Zatykó L. 2006. Pepper (Capsicum annuum L.) breeding methods at the turn of the century. Acta Agron Hung. 54:179–202. doi:10.1556/AAgr.54.2006.2.7.
- Zeidler C, Woeckner G, Schöning J, Vrakking V, Zabel P, Dorn M, Schubert D, Steckelberg B, Stakemann J. 2021. Crew time and workload in the EDEN ISS greenhouse in Antarctica. Life Sci Space Res (Amst). 31:131–149. doi:10.1016/j.lssr.2021.06.003.
- Zhuang Y, Chen L, Sun L, Cao J. 2012. Bioactive characteristics and antioxidant activities of nine peppers. J Funct Foods. 4:331–338. doi:10.1016/j.jff.2012.01.001.