ABSTRACT
Nitrogen is the primary limiting factor for crop yield formation. However, excessive application of nitrogen fertilizer not only leads to a decrease in nitrogen utilization efficiency, but also causes serious environmental problems. Therefore, how to balance environmental protection and crop yield when applying nitrogen fertilizer is an important challenge currently faced in agricultural production. Biochar, as a soil amendment, has been widely used in agricultural production. This study used ‘Longyan 101’ tobacco as the experimental material and explored the effects of nitrogen reduction treatment (reducing conventional nitrogen application by half) and combined application of biochar (20 kg/hm2) on tobacco photosynthesis under field experimental conditions. Transcriptome sequencing technology was used to study the changes in the expression levels of photosynthesis-related genes. The results indicate that: reducing the application of nitrogen fertilizer reduces the photosynthetic capacity of tobacco and inhibits its growth, while applying biochar can to some extent alleviate the adverse effects of insufficient nitrogen fertilizer. The results of this experiment have laid the foundation for the rational application of nitrogen fertilizer and the green development of tobacco agriculture.
1 Introduction
Nitrogen, as one of the essential nutrients for plants, participates in the biosynthesis of various metabolites and cellular components such as amino acids, chlorophyll, and nucleotides (Matiu et al. Citation2017), and is known as the ‘life element.’ The supply of nitrogen nutrients in agricultural production has become the primary limiting factor for crop growth and yield formation (Li et al. Citation2019). According to reports, approximately 85–90 million tons of nitrogen fertilizer are added to soil worldwide every year, but in fact, only 30–40% of nitrogen will be absorbed and utilized by plants (Curci et al. Citation2017). However, insufficient supply of nitrogen fertilizer can also lead to crop yield reduction. Therefore, the rational application of nitrogen fertilizer, especially studying the response mechanism of crops under reduced nitrogen fertilizer usage is crucial. At the same time, improving the utilization efficiency of nitrogen fertilizer and limiting its excessive use are important challenges for protecting the environment and crop production. Some studies have found that nitrogen deficiency can lead to a decrease in plant growth rate, causing yellowing of plant leaves, ultimately reducing leaf size and photosynthetic rate (Babst et al. Citation2019). In addition, under low nitrogen conditions, genes and enzymes related to photosynthesis can also be affected, leading to a decrease in photosynthesis (Zhao et al. Citation2011).
Biochar, as a soil amendment, has the potential to reduce soil greenhouse gas emissions and increase soil carbon sequestration (Shen et al. Citation2017), it has been promoted as an effective tool for mitigating climate change and has become a research hotspot in recent years. Currently, research has shown that the rational combination of biochar and nitrogen fertilizer has demonstrated its beneficial effects. Fang et al. (Citation2018) found that adding biochar to acidic soil increased nitrogen uptake by Brassica oleracea L. by 44–59%. Biochar also can affect soil nitrogen cycling by affecting the diversity, richness, and community structure of soil microorganisms (Liu et al. Citation2016). Overall, biochar amendments increase the effectiveness and retention of soil nitrogen, enhance soil water holding capacity, increase soil pH and cation exchange capacity, reduce soil bulk density, and promote the growth of beneficial microorganisms (Chen et al. Citation2019).
Photosynthesis is the starting source of energy conversion and is crucial for the growth and development of plants. Many studies have shown that nitrogen deficiency can hinder plant growth and development, reduce photosynthesis and leaf area, promote plant ageing, and ultimately lower plant productivity (Mu et al. Citation2016). Studies have shown that adding biochar to soil can promote the photosynthesis of tea (Camellia sinensis L.) (Li et al. Citation2021). Biochar also can increase plant photosynthesis by increasing soil humus and organic matter content, thereby affecting soil fertility and carbon dioxide supply (Liu et al. Citation2021), the reason may be that the porous structure of biochar can improve soil permeability and increase the specific surface area of the soil (Lin et al. Citation2018). In summary, the effects of biochar amendments on plant photosynthesis are also related to soil properties, cultivation methods, experimental methods, biochar application rates, biochar physicochemical properties, and plant types (Sarma et al. Citation2017). After adding biochar, these factors may affect soil nutrients and water use efficiency, thereby altering the physiological characteristics of plants. However, further research is needed on how the above factors affect the response of photosynthesis to biochar amendments (He et al. Citation2020).
Tobacco (Nicotiana L.) is an important economic crop, but some unreasonable planting systems, cultivation methods, and fertilization patterns, especially excessive reliance on fertilizers, have a significant impact on tobacco yield and quality (Liu et al. Citation2014). The reasonable application of nitrogen fertilizer is conducive to the normal growth of tobacco, improves its photosynthesis, increases the accumulation of nutrients, and greatly improves the quality and yield of tobacco. Under the trend of implementing green and clean production in tobacco and its products, in order to promote the green development of tobacco agriculture, biochar, as a soil amendment, has been widely used in agriculture and the environment, and has achieved positive effects (Qian et al. Citation2020). This article explores the effects of biochar application on photosynthesis in tobacco under nitrogen reduction conditions from the aspects of tobacco phenotype, photosynthetic performance, energy allocation, etc. The differential expression genes of tobacco after biochar application under nitrogen reduction conditions were analyzed by RNA-seq, and KEGG enrichment analysis was conducted. The relevant research results can lay a foundation for the rational application of nitrogen fertilizer, improvement of soil environment, and promotion of green development of tobacco agriculture.
2 Materials and methods
2.1 Overview of the experimental area and experimental design
The experimental site is located at the Binxi Experimental Field of Heilongjiang Tobacco Science Research Institute(N: 45°47′55″ E: 127°7′24″), the area has an altitude of 165 m, an average annual temperature of 3.9°C, and an average annual precipitation of 681 mm, belonging to the temperate continental monsoon season. The terrain and topography are hilly land, with soybeans as the previous crop. The soil in the experimental site is black soil, the pH of the tested soil is 6.52, the alkaline nitrogen content is 103.5 mg/kg, the effective phosphorus content is 21.9 mg/kg, the organic matter content is 4.15 g/kg, and the available potassium content is 149.6 mg/kg. The terrain and topography are hilly land, with soybeans as the previous crop.
The tested tobacco variety is ‘Longyan 101’ (WT), which is conventional sowing and greenhouse seedling cultivation. On May 10, 2023, when the seedlings grow to 6–7 leaves, they will be transplanted to the field with film covering, uncover the film around June 5th. Planting density: row spacing of 1.15 × 0.5 m, 17385 plants/hm2. This experiment is based on the local conventional fertilization standard of 2 kg of pure nitrogen per mu (Chinese unit of land measurement that is commonly 666.7 square metres) (N:P2O5:K2O = 1.2:1:2). Set a 1 kg reduction in nitrogen fertilizer application as the nitrogen reduction treatment (LN), with the same amount of phosphorus and potassium fertilizer as the conventional nitrogen application treatment. On the basis of conventional nitrogen fertilizer and nitrogen reduction, a biochar-based fertilizer of 20 kg/hm2 is used. Conventional application of chemical fertilizers, rice straw biochar is produced by Shenyang Longtai Biotechnology Co., Ltd. The pH value of the tested corn straw biochar is 10.53, with nitrogen (N), phosphorus (P2O5), potassium (K2O) content of 0.726%, 1.29%, 2.08%, and organic matter content of 37.4%, respectively. Apply once before tobacco transplanting in 2023. Application method of biochar: after dividing the experimental plot, the biochar is evenly spread on the surface of the test plot according to the amount of biochar used for each treatment. Artificial cultivation is used to fully mix it with the topsoil, and then small machinery is used to plow and mix it into the topsoil, with a plowing depth of about 20 cm.
2.2 Determination items and methods
2.2.1 Investigation of agronomic traits in tobacco
On July 15, 2023, tobacco plants were in a prosperous period, and their growth and other agronomic traits were measured. Root length is carefully excavated from the soil, and after cleaning the plants, the length from the lateral root to the longest root tip is measured. The height from the root to the highest leaf is taken as the plant height. Dry the washed root system with absorbent paper and weigh it as the fresh weight of the underground part, and then it was dried by 80°C to constant weight, which was used as underground biomass. The method for measuring fresh weight and biomass of aboveground parts is the same as that for underground parts.
2.2.2 Rapid chlorophyll fluorescence curve and fluorescence parameters
Select fully unfolded functional tobacco leaves from different strains under different treatments, and treat tobacco leaves with dark adaptation clips for 30 min. Use a multifunctional plant efficiency analyzer (M-PEA, Hansatech, UK) to measure the rapid chlorophyll fluorescence kinetics curve (OJIP curve), and repeat the measurement three times for each treatment. Apply 3500 during the measurement process μ Red light pulse of mol·m−2·s−1, record the chlorophyll fluorescence signal for 1 s. The time nodes of relative fluorescence intensity FO, FJ, FI and FP at O, J, I and P points are 0.02, 2, 30 and 1000 ms, respectively. According to the formulas VO-P = (Vt–VO)/(VP–VO), VO-J=(Vt–VO)/(VJ–VO)和VO-K=(Vt–VO)/(VK–VO), the OJIP curves are normalized to VO-P, VO-J and VO-K curves. The differences between all processed values and WT values are ΔVO-P, ΔVO-J and ΔVO-K, to analyze the variation range of each characteristic point on the curve. According to the calculation method of Strasser (Strassert et al. Citation1995) the JIP-test analysis of OJIP curve obtained the maximum photochemical efficiency (Fv/Fm) of PSII, photosynthetic performance index (PIABS) based on light absorption and other energy distribution parameters: absorbed light energy per unit reaction center (ABS/RC), energy per unit reaction center for electron transfer (ETO/RC), dissipated energy per unit reaction center (DIO/RC), absorbed energy per unit area (ABS/CSM), energy per unit area for electron transfer (ETO/CSM) and heat-dissipated energy per unit area (DIO/CSM).
2.2.3 Transcriptome analysis
Samples were taken from tobacco leaves of different strains grown under different treatments using a punch. The sampled leaves were completely unfolded in the middle of the plant, and the punch was used to avoid leaf veins. After sampling, the leaves were wrapped in tin foil and frozen in liquid nitrogen for 30 min before being stored at −80°C. The data were analyzed on the online platform of Majorbio Cloud Platform (www.majorbio.com), the main process of analysis includes: (1) Sequencing experiment process; (2) Quality control data statistics; (3) Differentially expressed gene screening; (4) Functional annotation statistics (Wang et al. Citation2021).
Based on the results of quantitative expression levels, analyze the differentially expressed genes (DEGs) between the two groups, and obtain the differentially expressed genes between the two groups using DESeq2 software. The filtering threshold is |log2FC| > 1 and P < 0.05. Kyoto Encyclopedia of Genes and Genomes (KEGG) pathway enrichment analysis: Using R language to write scripts for KEGG Pathway enrichment analysis of genes/transcripts in gene sets. KEGG pathway enrichment was determined at a P < 0.05 significance threshold.
2.2.4 Quantitative real-time PCR analysis
CTAB method for carrying tobacco leaf RNA and analyzing the mass of total RNA using NanoDrop (Thermo Fisher Scientific Inc). RNA with an OD260/280 value between 1.8 and 2.2 and OD260/230 value over 2.0 was reverse-transcribed into first-strand cDNA using the ReverTra Ace qPCR RT Master Mix with gDNA Remover kit. Real-time quantitative PCR was performed using SYBR Green Realtime PCR Master Mix (TOYOBO) according to the manufacturer's instructions. The gene-specific primers of the selected DEGs were designed using PRIMER 5 (). qRT-PCRs were performed on Roche 480 with three reactions for each biological replicate and a total of three biological replicates for each gene. The expression levels were calculated using the 2−ΔΔCT method, the gene L25 was used as an internal control (Xu et al. Citation2022).
Table 1. Primer pairs used in this study.
2.2.4 Data processing
The measured data were statistically analyzed by Microsoft Excel 2010 (Redmond, WA, USA) and SPSS (IBM, Inc., Armonk, NY, USA). The differences among different treatments were compared by one-way ANOVA. According to Duncan's multiple range test, P < 0.05 is a significant difference.
3 Results
3.1 Plant phenotype and biomass
As shown in (A,B), both nitrogen reduction treatment and application of biochar had no significant effect on the plant height and root length of tobacco (P > 0.05). In (C–F), the aboveground biomass, underground biomass and fresh weight of tobacco under the nitrogen reduction treatment were significantly lower than those under the normal nitrogen application condition. Under the normal nitrogen application condition, the application of biochar had no significant effect on the aboveground and underground biomass and fresh weight of tobacco, but under the nitrogen reduction treatment, the underground biomass of tobacco was significantly increased.
Figure 1. Effects of nitrogen reduction combined with Biochar on Plant height (A), Root length (B), Aboveground/Underground Biomass (C, D) and Aboveground/Underground fresh weight (E, F) of Tobacco plants. Notes: The addition of biochar to soil with reduced nitrogen levels is denoted as LN+C, no biochar is applied to nitrogen-reduced soils, which are abbreviated as LN-C. The addition of biochar to conventionally nitrogen fertilized soil is denoted as WT+C, no biochar is applied to conventional nitrogen fertilized soil is denoted as WT-C. The data in the figure are from three replicated (n = 11), and represent means ± standard error (SE). Different lowercase letters indicate significant differences (P < 0.05).
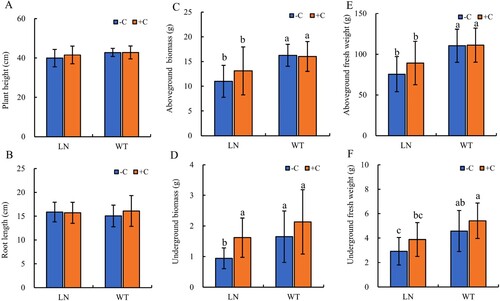
3.2 KEGG enrichment analysis of differentially expressed genes (DEGs) and DEGs under different treatments
Under conventional nitrogen application levels, a total of 207 differentially expressed genes (DEGs) were identified between biochar application and non-biochar application treatments, of which 180 were downregulated and 27 were upregulated ((A,B)). However, under nitrogen reduction conditions, the number of differentially expressed genes (DEGs) between biochar application and non-biochar application treatments was significantly lower than that of conventional nitrogen application treatments. Under the condition of no biochar application, a total of 1399 differentially expressed genes (DEGs) were identified between normal nitrogen fertilizer and nitrogen reduction treatments, of which 580 were upregulated and 819 were downregulated. These DEGs were significantly enriched in 14 KEGG pathways, including Carbon fixation in photosynthetic organisms (map00710) and Photosynthesis antenna proteins (map00196) ((H)). After applying biochar, the number of DEGs significantly decreased, and a total of 96 DEGs were identified. Compared with normal nitrogen application conditions, 76 DEGs were upregulated and 20 were downregulated after nitrogen reduction treatment ((C,D)), analysis of KEGG enrichment pathways with P < 0.05 reveals that these DEGs are significantly enriched in five pathways, including Carbon fixation in photosynthetic organizations (map00710) and Photosynthesis (map00195) ((I)). This indicates that reducing the application of nitrogen fertilizer will have a significant impact on the photosynthesis of tobacco, and biochar can effectively alleviate the impact of nitrogen fertilizer deficiency on tobacco plants. Under normal nitrogen application and simultaneous application of biochar, compared to reducing the addition of nitrogen fertilizer without biochar, 406 differentially expressed genes were upregulated and 443 differentially expressed genes were downregulated ((E)). A large amount of DEGs was significantly enriched in metabolic pathways related to photosynthesis, such as Photosynthesis-antenna proteins (map00196), Carbon fixation in photosynthetic organisms (map00710) and Photosynthesis (map00195) ((J)), that is to say, reducing the application of nitrogen fertilizer mainly affected the metabolic pathways such as light energy capture and photosynthetic electron transport in tobacco leaves.
Figure 2. Effects of nitrogen and biochar treatment on volcano plot of DEGs (A–E) and KEGG pathways enrichment analysis of DEGs (F–J) in tobacco leaves. Note: The addition of biochar to soil with reduced nitrogen levels is denoted as LN+C, no biochar is applied to nitrogen-reduced soils, which are abbreviated as LN-C. The addition of biochar to conventionally nitrogen fertilized soil is denoted as WT+C, no biochar is applied to conventional nitrogen fertilized soil is denoted as WT-C.
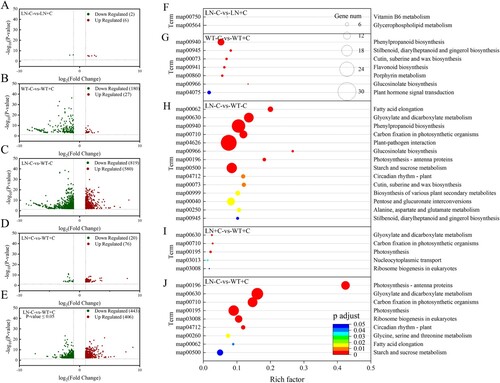
3.3 Chlorophyll fluorescence parameters
Under the condition of reducing the amount of nitrogen fertilizer, the relative fluorescence intensity (FP) of P point on the OJIP curve of tobacco leaves decreased significantly, which was 8.06% lower than that of normal application of nitrogen fertilizer, the addition of biochar has no significant effect on the relative fluorescence intensity under nitrogen reduction conditions ((A)). The VO-P, VO-J and VO-K curves are obtained by standardizing the original OJIP curve ((B)). Compared with normal nitrogen application conditions, the relative fluorescence intensity of J, K, and L points in tobacco leaves under reduced nitrogen conditions was significantly increased ((B–D)). The ΔVO-P, ΔVO-J and ΔVO-K curves were obtained by adding biochar with and without biochar under different nitrogen content ((E–G)). In the ΔVO-P curve, it was found that the relative fluorescence intensity at J point of tobacco treated with nitrogen reduction was significantly higher than that of normal nitrogen treatment ((E)), according to the quantitative results of VJ, the tobacco under nitrogen reduction treatment increased by 14.1% compared with that under normal nitrogen application ((J)). Similarly, the relative fluorescence intensity at K point of tobacco under the condition of reduced nitrogen in ΔVO-J curve was significantly higher than that of normal nitrogen application ((F)). According to the quantitative results of VK, tobacco under reduced nitrogen was 14.9% higher than that of normal nitrogen application ((K)). Under the condition of reducing nitrogen in ΔVO-K curve, the relative fluorescence intensity of tobacco leaves at L point was significantly higher than that of normal nitrogen application ((G)). According to the quantitative results of VL, tobacco under nitrogen reduction treatment increased by 11.6% compared with normal nitrogen application ((L)). Under the condition of nitrogen reduction, the addition of biochar had no significant effect on Fv/Fm ((H)), but the PIabs value of tobacco leaves with biochar was slightly higher than that without biochar ((I)).
Figure 3. Chlorophyll fluorescence curves and parameters in tobacco leaves under nitrogen and biochar treatment. Note: The addition of biochar to soil with reduced nitrogen levels is denoted as LN+C, no biochar is applied to nitrogen-reduced soils, which are abbreviated as LN-C. The addition of biochar to conventionally nitrogen fertilized soil is denoted as WT+C, no biochar is applied to conventional nitrogen fertilized soil is denoted as WT-C. The data in the figure are from three replicated (n = 11), and represent means ± standard error (SE). Different lowercase letters indicate significant differences (P < 0.05).
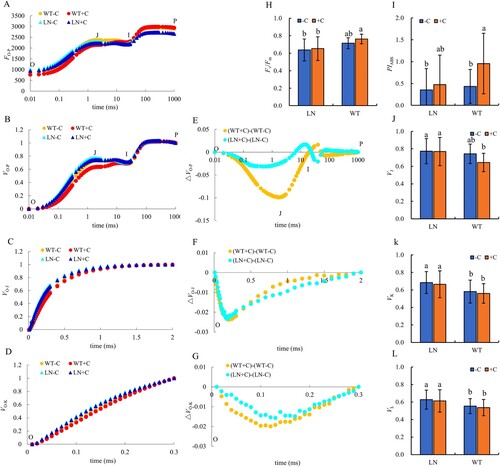
3.4 Energy distribution parameters of optical system
Under normal application of nitrogen fertilizer and nitrogen reduction treatment, biochar did not show significant effects on the energy allocation parameters of tobacco leaves ((A–F)). Compared with normal nitrogen application, the ABS/RC and DIo/RC of tobacco leaves after nitrogen reduction treatment showed the same trend, with a significant increase in ABS/RC and DIo/RC (P < 0.05) ((A,C)), while ETo/RC showed no significant change (P > 0.05) ((B)). However, after nitrogen reduction treatment, the ABS/CSm and ETo/CSm of tobacco leaves decreased compared to normal nitrogen application conditions, and only reached significant levels in tobacco treated with biochar, reducing by 8.83% (P < 0.05) and 40.6% (P < 0.05) respectively ((D,E)), while DIo/CSm increased, significantly increasing by 29.7% (P < 0.05) in tobacco treated with biochar ((F)). After reducing the nitrogen fertilizer content in the soil during tobacco growth, the application of biochar can relatively alleviate the imbalance of PSII energy allocation parameters caused by low nitrogen conditions, and to some extent promote the proportion of PSII reaction centers absorbing light energy for photochemical reactions.
Figure 4. Effect of nitrogen and biochar treatmeat on ABS/RC (A), ETo/RC (B), DIo/RC (C), ABS/CSm(D), ETo/CSm (E), and DIo/CSm (F) in tobacco leaves. Note: The addition of biochar to soil with reduced nitrogen levels is denoted as LN+C, no biochar is applied to nitrogen-reduced soils, which are abbreviated as LN-C. The addition of biochar to conventionally nitrogen fertilized soil is denoted as WT+C, no biochar is applied to conventional nitrogen fertilized soil is denoted as WT-C. The data in the figure are from three replicated (n = 11), and represent means ± standard error (SE). Different lowercase letters indicate significant differences (P < 0.05).
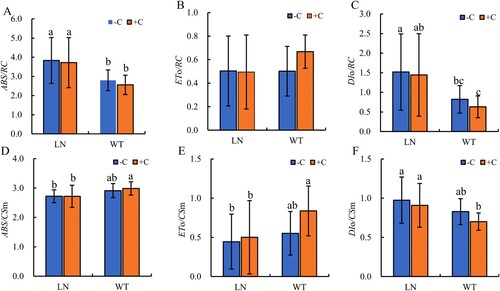
3.5 Expression of key genes in photosynthetic antenna proteins
It can be clearly seen from that a total of 14 genes encoding photosynthetic antenna proteins, including 5 LHCI and 9 LHCII, were identified in this study with significant changes in expression. Compared with normal nitrogen application, the expression of tobacco-related genes decreased except Lhcb2 (Nitab4.5-0004124g0010) ((I)). Among them, the expression of 3 LHCI (2 Lhca3 and 1 Lhca5) ((B, C, E)) and 5 LHCII (1 Lhcb3, 2 Lhcb5 and 2 Lhcb6) ((J, L-O)) genes reached a significant down-regulation level. Under nitrogen reduction treatment, compared to not applying biochar, applying biochar upregulated the expression levels of relevant genes in tobacco, but only Lhca5 ((E)) gene expression reached a significant upregulation level.
Figure 5. The key genes expression of photosynthetic-antenna proteins in tobacco leaves under nitrogen and biochar treatmeat. Abbreviations: Lhca2: light-harvesting complex I chlorophyll a/b binding protein 2; Lhca3: light-harvesting complex I chlorophyll a/b binding protein 3; Lhca4:light-harvesting complex I chlorophyll a/b binding protein 4; Lhca5:light-harvesting complex I chlorophyll a/b binding protein 5; Lhcb2: light-harvesting complex II chlorophyll a/b binding protein 2; Lhcb3: light-harvesting complex II chlorophyll a/b binding protein 3; Lhcb5: light-harvesting complex II chlorophyll a/b binding protein 5; Lhcb6:light-harvesting complex II chlorophyll a/b binding protein 6. Notes: The data in the figure are from three biological repetitions (n = 3) means ± standard error (SE). Different lowercase letters indicate significant differences (P < 0.05).
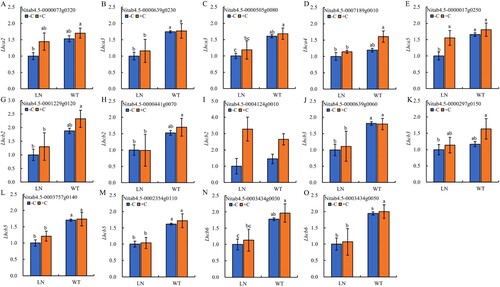
3.6 Expression of genes related to photosynthetic electron transfer
From , it can be seen that a total of 13 genes related to photosynthetic electron transfer have undergone significant changes in expression (P < 0.05). Compared with normal nitrogen application conditions, nitrogen reduction treatment downregulated the expression of photosynthetic electron transfer related genes in the PSII and PSI reaction centers of tobacco leaves. Except for the oxygen releasing complex (OEC) related protein PsbP ((G)), PsaG, and PsaN ((K,M)), The expression levels of genes related to 7 PSII reaction centers (2 PsbA, 2 PsbC, 2 PsbD, and 1 PsbQ) ((A–F, H)) and 3 PSI reaction centers (PsaA, PsaF, and PsaH) ((I, J, L)) were significantly downregulated (P < 0.05). Under nitrogen reduction conditions, compared to not applying biochar, the addition of biochar resulted in a downregulation of the expression levels of PsbC ((C)) and PsaF ((J)) genes, while the expression levels of most genes showed an upward trend.
Figure 6. The key genes expression of photosynthetic electron transport in tobacco leaves under nitrogen and biochar treatmeat. Notes: The data in the figure are from three biological repetitions (n = 3) means ± standard error (SE). Different lowercase letters indicate significant differences (P < 0.05).
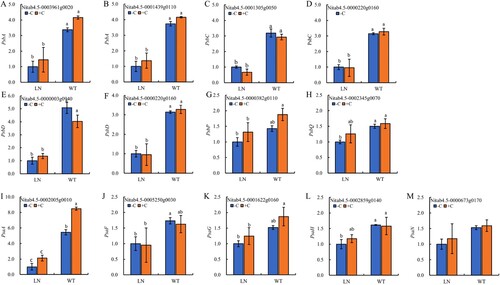
3.7 Calvin cycle-related gene expression levels
From , it can be seen that a total of 13 Calvin cycle-related genes were identified with significant changes in their expression levels. Compared with normal nitrogen application, the expression levels of Calvin cycle-related genes in tobacco were all downregulated under nitrogen reduction conditions ((A–M)), with 11 genes (5 rbcL, 4 rbcS, and 2 GAPA) showing significant downregulation levels (Figure (A-E, H-M)). Under nitrogen reduction conditions, compared to not applying biochar, applying biochar upregulated the expression of almost all Calvin cycle-related genes in tobacco.
3.8 qRT-PCR quantitative validation
In order to further analyze the expression patterns of photosynthesis-related genes in tobacco leaves under nitrogen reduction treatment, nine genes were randomly selected from antenna protein, electron transfer, and Calvin cycle-related genes, and qRT-PCR quantitative validation was performed on tobacco leaves under nitrogen reduction conditions. As shown in , the relative expression levels in qRT-PCR were consistent with TPM in RNA-seq, which verified the reliability of our transcriptome data.
Figure 8. Verification of the RNA-seq expression by qRT-PCR. Notes: The statistical values of RNA-seq are indicated by lowercase letters, and the statistical values of qRT-PCR are indicated by uppercase letters. The data in the figure are from three biological repetitions (n = 3) means ± standard error (SE). P < 0.05 was a significant difference.
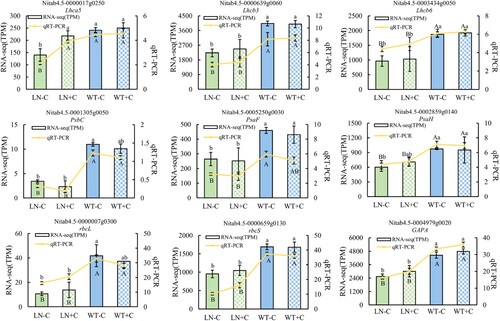
4. Discussion
Nitrogen, also known as the ‘life element’ in the plant kingdom, is one of the essential elements for the growth and development of green plants. The supply of nitrogen nutrients in agricultural production has become the primary limiting factor for crop growth and yield formation (Li et al. Citation2019). Under low nitrogen conditions, plants usually change their morphology, physiological changes, and molecular level to adapt to changes in nitrogen content both internally and externally. Zhang et al. (Citation2017) found that the growth parameters of Fagopyrum tataricum L., such as plant height, leaf area, and biomass, significantly decreased under low nitrogen stress, while the main root length and root crown ratio showed an increasing trend. Moreover, there were differences in the response of plant growth and development among different genotypes under low nitrogen stress. Low nitrogen stress can have a significant impact on the morphological characteristics of plant roots, and the roots will also exhibit adaptive mechanisms based on changes in soil nitrogen, such as changing the root diameter, number of root hairs, and branching of the roots (Jia et al. Citation2020). Biochar is a carbon rich substance produced through pyrolysis. Due to its low density and large surface area, biochar has been explored as an organic amendment to improve soil quality, enhance soil water holding capacity, and promote plant growth and crop productivity (Lehmann et al. Citation2011; Zhang, Lin, et al. Citation2016). In this study, the plant height and root length of tobacco did not show significant changes after nitrogen reduction treatment, while the aboveground and underground biomass and fresh weight significantly decreased. However, the application of biochar resulted in a significant increase in the underground biomass of tobacco plants under nitrogen reduction treatment. The findings indicate that biochar has the potential to enhance tobacco root development in order to alleviate the negative impacts of low nitrogen levels. More and more evidence suggests that biochar stimulates root growth, which is beneficial for root morphology development, including increasing root biomass (RB), root volume, surface area, root density, and root length to obtain more nutrients and water, promote plant photosynthesis and growth (Joseph et al. Citation2010; Makoto et al. Citation2010; Lehmann et al. Citation2011; Bruun et al. Citation2014).
RNA-seq as a widely used tool for studying molecular regulatory mechanisms and searching for stress response genes (Que et al. Citation2014) it has been used to explore molecular mechanisms under low nitrogen stress in various plants (Liu et al. Citation2020). For example, Quan et al. (Citation2019) revealed the molecular mechanism of two Xizang wild barley under slow nitrogen deficiency conditions, and identified candidate genes related to low nitrogen tolerance. The research results of Liang (Liang and He Citation2018) indicate that the secondary metabolite anthocyanins accumulated in Arabidopsis thaliana L. under low nitrogen stress help it resist low nitrogen stress. KEGG enrichment analysis is an effective tool for understanding the main functions and regulatory pathways of DEGs in RNA sequencing data (Liu et al. Citation2022). Nitrogen plays a crucial role in the extensive metabolism and biochemical processes within plants, directly and effectively affecting the formation of chlorophyll and the assimilation of photosynthesis (Luo et al. Citation2013). From the transcriptomic results of this study, it can be inferred that under reduced nitrogen conditions, DEGs significantly enriched in KEGG pathways related to photosynthesis, including Carbon fixation in photosynthetic organizations (map00710) and Photosynthesis antenna proteins (map00196), compared to normal nitrogen levels. Moreover, under nitrogen reduction conditions, the KEGG pathway significantly enriched by DEGs between biochar application and non biochar application also includes processes such as Carbon fixation in Photosynthetic organizations (map00710) and Photosynthesis (map00195). Therefore, the enrichment results of DEGs indicate that not only is the photosynthesis process of tobacco sensitive to low nitrogen treatment, but the utilization of biochar had a significant impact on the regulation of genes associated with photosynthesis in tobacco.
Chlorophyll fluorescence analysis is considered a powerful tool for studying plant photosynthetic responses (Goltsev et al. Citation2016), and is a sensitive method for detecting and quantifying changes in plant photosystem caused by abiotic stress (Kan et al. Citation2017). Fv/Fm represents the maximum efficiency of PSII light energy utilization, reflecting the structural and functional integrity of the optical system PSII (Caffarri et al. Citation2014). A lower Fv/Fm value indicates a certain percentage of PSII reaction centers are damaged or inactivated, which is a common phenomenon in stressed plants (Baker and Rosenqvist Citation2004). PIABS is a performance index based on the absorption of light energy, which is more sensitive to environmental factors and can more accurately reflect the state of plant photosynthetic mechanisms. In our study, the trends of Fv/Fm and PIABS were consistent, both decreasing under nitrogen reduction treatment. However, the application of biochar alleviated the decrease in Fv/Fm and PIABS. This indicates that biochar can maintain high PSII photochemical activity in tobacco under nitrogen reduction treatment and improve plant light energy utilization efficiency. The original OJIP curve is largely influenced by the environment, so we standardize the OJIP curve so that all OJIP curves have a common starting point and a common ending point. The results showed that nitrogen reduction treatment significantly increased the relative variable fluorescence of tobacco seedlings at 2 ms (time point J) on the standardized O-P curve (VJ), 0.3 ms (time point K) on the standardized O-J curve (VK), and 0.15 ms (time point L) on the standardized O-K curve (VL). However, the application of biochar reduced the amplification of tobacco plants. The relative fluorescence intensity at point J is a marker of PSII electron receptor side damage, and an increase in VJ indicates that electron transfer from QA to QB in the photosynthetic electron transport chain is blocked under stress, resulting in an increase in QA-/QA ratio (Zhang, Zhong, et al. Citation2016, Citation2020). The increase in K-point relative variable fluorescence is considered a key indicator of PSII donor side oxygen release complex (OEC) photoinhibition damage (Devadasu et al. Citation2021). Bertamini (Bertamini and Nedunchezhian Citation2003) found that the donor side of PSII is more easily inhibited under pressure, mainly due to the reduction of 33 kDa hydrolyzed complex proteins. The activity of OEC is often inhibited under pressure, resulting in hindered electron transfer from the electron donor side to the electron acceptor side (Bertamini and Nedunchezhian Citation2003). Proteins PsbO, PsbP, and PsbQ are important components of the PSII oxygen-releasing complex (OEC). The L-point is an indicator of the energy connectivity between the fluorescent antenna and the PSII RC unit (Strasser et al. Citation2004). In addition, the increase in VL is mainly related to the damage to the thylakoid membrane in chloroplasts and the dissociation of thylakoids (Ye et al. Citation2013). The results of this study showed that the expression of PsbQ-related genes in tobacco leaves was downregulated after nitrogen reduction treatment. This indicates that nitrogen reduction treatment can hinder the function of the donor side OEC of tobacco PSII and the electron transfer from the receptor side QA to QB, while the application of biochar can stabilize the structure of the thylakoid membrane and alleviate the inhibition of PSII's photosynthetic electron transfer, which is consistent with the changes in Fv/Fm and PIABS. Studies have shown that under low nitrogen stress, the excitation energy absorbed by the cytochrome center is dissipated, leading to a decrease in PSII photochemical efficiency (Chow Citation2012). CURCI et al. (Curci et al. Citation2017) also found that under low nitrogen stress, most genes involved in photosynthesis and photorespiration in wheat (Triticum aestivum L.) were downregulated, including the photosystem (PSI), photosystem (PSII), ATP synthase, and Rubisco (ribulose-1,5-bisphosphonate carboxylase/oxygenase). In this study, the expression of photosynthetic electron transfer related genes in the PSII and PSI reaction centers of tobacco leaves showed a downward trend under nitrogen reduction conditions. After applying biochar, most of the photosynthetic electron transfer-related genes in the PSII and PSI reaction centers of tobacco leaves showed an upregulation trend, indicating that biochar can improve nitrogen utilization efficiency and alleviate the adverse effects of insufficient nitrogen fertilizer on photosynthesis in tobacco. Khan et al. (Khan et al. Citation2020) also demonstrated that the application of straw biochar significantly promoted soil chemical properties, nitrogen use efficiency, photosynthesis, and the growth of Brassica campestris L. Applying only nitrogen fertilizer resulted in poor growth of rapeseed, decreased nitrogen use efficiency, and soil acidification. However, the application of biochar can compensate for the adverse effects of excessive nitrogen fertilizer through the absorption and utilization efficiency of nutrients by rapeseed.
In this study, 5 PSI light-harvesting antenna proteins (LHCI) and 9 PSII light-harvesting antenna protein (LHCII) related genes in the photosynthetic antenna protein (map00196) pathway were also negatively affected by nitrogen reduction treatment. LHCI and LHCII are encoded by Lhca and Lhcb genes, respectively. They belong to the chlorophyll a/b binding protein (LHC) family and can bind chlorophyll a and b with other pigment molecules to capture and transport light energy (Ziehe et al. Citation2018). After nitrogen reduction treatment, the expression levels of a large number of photosynthetic antenna protein-coding genes in tobacco leaves LHCII and LHCI were significantly downregulated. The nitrogen supply and distribution within leaves have a significant impact on photosynthesis by regulating the biosynthesis of PSI, PSII, LHCs, Cyt-b6f, ATP synthase, and photosynthetic enzymes (Mu and Chen Citation2021). In C3 plants of the evergreen deciduous oak genus (Quercus L.), with a decrease in nitrogen supply, the content of Rubisco, bioenergy proteins, and light-harvesting proteins decreases, resulting in a decrease in photosynthetic rate (Tazoe et al. Citation2006). In rice (Oryzasativa L.), the content of light-harvesting protein, Rubisco, and cell wall decreases with decreasing nitrogen supply (Li et al. Citation2013; Zhong et al. Citation2019). This proves that nitrogen reduction treatment may reduce the photosynthetic rate by reducing the content of light-harvesting proteins in tobacco plants. After applying biochar, the expression of most key genes of photosynthetic antenna proteins showed an upregulation trend, and the expression levels of Lhca5 genes located at the PSI reaction center reached a significant upregulation level. This indicates that biochar can alleviate the expression of light-harvesting antenna protein-related genes in tobacco leaves under low nitrogen conditions, promote tobacco's ability to capture and absorb light energy, improve tobacco's energy supply, and enable better growth.
Under adverse conditions, plants usually adapt to environmental changes by adjusting their energy distribution (Misra et al. Citation2001). Under nitrogen reduction conditions, the light energy absorption and distribution parameters of the PSII reaction center in tobacco leaves also undergo significant changes. The results showed that the absorption of light energy ABS/CSm per unit area showed a decreasing trend, but the absorption of light energy ABS/RC per unit reaction center significantly increased. This may be due to the enhanced activity of antenna pigments in each reaction center, which serves as a mechanism in response to nitrogen reduction treatment. This indicates that in the case of insufficient nitrogen fertilizer, the number of active reaction centers per unit area of tobacco leaves decreases, forcing the remaining reaction center activity to increase. Research has found that under stress, some PSII reaction centers become inactive, and the function of antenna pigments in the remaining active reaction centers increases to ensure energy supply (Dernetriou et al. Citation2007). Our findings align with those of previous studies. In addition, when nitrogen fertilizer is insufficient, the ETo/CSm of tobacco seedling leaves significantly decreases, while DIo/RC and DIo/CSm increase, indicating that under nitrogen reduction treatment, the energy absorbed by tobacco leaves for electron transfer decreases, while the energy used for heat dissipation increases. This plays a positive role in reducing excess excitation energy in PSII and protecting the electron transfer chain (Appenroth et al. Citation2001). Studies have shown that under low nitrogen stress, the excitation energy absorbed by the cytochrome center is dissipated, leading to a decrease in PSII photochemical efficiency (Chow Citation2012). Therefore, nitrogen reduction treatment leads to a decrease in the utilization efficiency of light energy in tobacco leaves. When the activity of PSII reaction centers weakens, light energy is mainly dissipated through heat and fluorescence. However, under nitrogen reduction conditions, the application of biochar reduced the various energy allocation parameters of tobacco leaves. This indicates that the application of biochar can enhance the PSII photochemical activity of tobacco leaves and adapt to nitrogen fertilizer deficiency by optimizing the allocation and utilization of light energy, especially by increasing the proportion of light absorbed by PSII reaction centers in tobacco leaves under low nitrogen conditions, thereby improving the photosynthetic capacity of tobacco leaves.
Rubisco is a key photosynthetic enzyme responsible for CO2 fixation. It is the most abundant protein in leaves, but it is an inefficient carboxylase because it is easily inactivated due to the loss of carbamoylation and extreme inhibition by sugar phosphate inhibitors (Spreitzer and Salvucci Citation2002). There are results indicating that due to the high nitrogen input in Rubisco, the photosynthetic efficiency of plants may be limited under low nitrogen conditions as Rubisco activity decreases (Tantray et al. Citation2020). Meanwhile, the research results of Li et al. (Citation2013) also indicate that under nitrogen-deficient conditions, the inhibition of nitrogen assimilation leads to a decrease in chlorophyll content and Rubisco activity, which is the main reason for the decrease in photosynthetic rate. Our research results showed that under nitrogen reduction conditions, the expression levels of Calvin cycle-related genes in tobacco leaves were all down regulated. Among them, the expression levels of genes encoding rbcL, rbcS, and GAPA proteins were significantly down regulated, indicating that under nitrogen deficiency conditions, Rubisco activity would decrease due to insufficient nitrogen supply, thereby hindering carbon assimilation and further reducing photosynthetic rate. After applying biochar, the expression levels of Calvin cycle-related genes showed an upward trend. Related studies have shown that biochar can enhance plant photosynthesis by increasing soil humus and organic matter content (Liu et al. Citation2021).
5 Conclusion
This study showed that under nitrogen reduction conditions, the aboveground and underground biomass and fresh weight of tobacco plants significantly decreased compared to normal nitrogen application. The application of biochar alleviated the growth status of tobacco plants under nitrogen reduction conditions. The results of physiological and transcriptome data also showed that nitrogen reduction significantly affected the photosynthesis process of tobacco leaves. Nitrogen reduction treatment led to a decrease in the activity of photochemical reaction centers in tobacco leaves, hindered photosynthetic electron transfer, and downregulated expression levels of antenna proteins, photosynthetic electron transfer, and Calvin cycle-related genes. The application of biochar can to some extent alleviate the adverse effects of reducing nitrogen fertilizer application on tobacco growth and photosynthetic capacity, especially stabilizing the electron transfer and energy distribution ratio of PSII reaction centers in tobacco leaves, improving the energy supply of photochemical reaction processes, and promoting the growth inhibition of tobacco plants caused by nitrogen reduction. In summary, the application of biochar can alleviate the growth inhibition of tobacco plants under nitrogen reduction conditions, which is mainly related to its promotion of the photosynthetic function of tobacco leaves.
Disclosure statement
No potential conflict of interest was reported by the author(s).
Additional information
Funding
Notes on contributors
Congcong Cui
Cui Congcong is a Postgraduate Student in Northeast Forestry University. Her research interests lie in the area of Plant physiology and molecular biology.
Jiaqi Song
Jiaqi Song is a Postgraduate Student in Northeast Forestry University. His research interests lie in the area of Plant physiology and molecular biology.
Shixin Han
Shixin Han is a Junior Researcher in Heilongjiang Tobacco company Mudanjiang Tobacco company. His research interests lie in the area of Plant physiology and crop cultivation.
Jiechen Wang
Jiechen Wang is a PhD in Northeast Forestry University. Her research interests lie in the area of Plant physiology and molecular biology.
Guangxin Ji
Guangxin Ji is a Postgraduate Student in Northeast Forestry University. His research interests lie in the area of Plant physiology and molecular biology.
Zhe Zhang
Zhe Zhang is a Postgraduate Student in Northeast Forestry University. Her research interests lie in the area of Plant physiology and molecular biology.
Hongjiao Zhang
Hongjiao Zhang is a PhD in Northeast Forestry University. Her research interests lie in the area of Plant physiology and molecular biology.
Zhiying E
Zhiying E is a Junior Researcher in Mudanjiang Tobacco Science Research Institute. Her research interests lie in the area of Plant physiology and crop cultivation.
Ye Yuan
Ye Yuan is an Associate Researcher in Mudanjiang Tobacco Science Research Institute. His research interests lie in the area of Plant physiology and crop cultivation.
Huihui Zhang
Huihui Zhang is an Associate Professor in Northeast Forestry University. His research interests lie in the area of Plant physiology and molecular biology.
References
- Appenroth KJ, Stockel J, Srivastava A, Strasser RJ. 2001. Multiple effects of chromate on the photosynthetic apparatus of Spirodela polyrhiza as probed by OJIP chlorophyll a fluorescence measurements. Environ pollut (Barking, Essex: 1987). 115:49–64. doi:10.1016/s0269-7491(01)00091-4.
- Babst BA, Gao F, Acosta-Gamboa LM, Karve A, Schueller MJ, Lorence A. 2019. Three NPF genes in Arabidopsis are necessary for normal nitrogen cycling under low nitrogen stress. Plant Physiol Biochem. 143:1–10. doi:10.1016/j.plaphy.2019.08.014.
- Baker NR, Rosenqvist E. 2004. Applications of chlorophyll fluorescence can improve crop production strategies: an examination of future possibilities. J Exp Bot. 55:1607–1621. doi:10.1093/jxb/erh196.
- Bertamini M, Nedunchezhian N. 2003. Photoinhibition of photosynthesis in mature and young leaves of grapevine (Vitis vinifera L.). Plant Sci. 164:635–644. doi:10.1016/S0168-9452(03)00018-9.
- Bruun EW, Petersen CT, Hansen E, Holm JK, Hauggaard-Nielsen H. 2014. Biochar amendment to coarse sandy subsoil improves root growth and increases water retention. Soil Use Manag. 30:109–118. doi:10.1111/sum.12102.
- Caffarri S, Tibiletti T, Jennings RC, Santabarbara S. 2014. A comparison between plant photosystem I and photosystem II architecture and functioning. Curr Protein Pept Sci. 15:296–331. doi:10.2174/1389203715666140327102218.
- Chen WF, Meng J, Han XR, Lan Y, Zhang WM. 2019. Past, present, and future of biochar. Biochar. 1:75–87. doi:10.1007/s42773-019-00008-3.
- Chow F. 2012. Nitrate assimilation: the role of in vitro nitrate reductase assay as nutritional predictor.
- Curci PL, Cigliano RA, Zuluaga DL, Janni M, Sanseverino W, Sonnante G. 2017. Transcriptomic response of durum wheat to nitrogen starvation. Sci Rep. 7:14. doi:10.1038/s41598-017-01377-0.
- Dernetriou G, Neonaki C, Navakoudis E, Kotzabasis K. 2007. Salt stress impact on the molecular structure and function of the photosynthetic apparatus - The protective role of polyamines. Biochim Biophys Acta-Bioenerg. 1767:272–280. doi:10.1016/j.bbabio.2007.02.020.
- Devadasu E, Pandey J, Dhokne K, Subramanyam R. 2021. Restoration of photosynthetic activity and supercomplexes from severe iron starvation in Chlamydomonas reinhardtii. Biochim Biophys Acta-Bioenerg. 1862:13. doi:10.1016/j.bbabio.2020.148331.
- Fang M, Ren T, Lai X, Wang Z, Song T, Li J, Zhang G. 2018. Effects of peanut shell biochar on physico-chemical properties and greenhouse gas emission in fluvo-aquic soil and red soil. J Agro-Environ Sci. 37:1300–1310.
- Goltsev VN, Kalaji HM, Paunov M, Baba W, Horaczek T, Mojski J, Kociel H, Allakhverdiev SI. 2016. Variable chlorophyll fluorescence and its use for assessing physiological condition of plant photosynthetic apparatus. Russ J Plant Physiol. 63:869–893. doi:10.1134/S1021443716050058.
- He YH, Yao YX, Ji YH, Deng J, Zhou GY, Liu RQ, Shao JJ, Zhou LY, Li N, Zhou XH, Bai SH. 2020. Biochar amendment boosts photosynthesis and biomass in C3 but not C4 plants: a global synthesis. GCB Bioenergy. 12:605–617. doi:10.1111/gcbb.12720.
- Jia ZT, Giehl RFH, von Wirén N. 2020. The root foraging response under Low nitrogen depends on DWARF1-mediated brassinosteroid biosynthesis. Plant Physiol. 183:998–1010. doi:10.1104/pp.20.00440.
- Joseph SD, Camps-Arbestain M, Lin Y, Munroe P, Chia CH, Hook J, van Zwieten L, Kimber S, Cowie A, Singh BP, et al. 2010. An investigation into the reactions of biochar in soil. Aust J Soil Res. 48:501–515. doi:10.1071/sr10009.
- Kan X, Ren JJ, Chen TT, Cui M, Li CL, Zhou RH, Zhang Y, Liu HH, Deng DX, Yin ZT. 2017. Effects of salinity on photosynthesis in maize probed by prompt fluorescence, delayed fluorescence and P700 signals. Environ Exp Bot. 140:56–64. doi:10.1016/j.envexpbot.2017.05.019.
- Khan Z, Zhang KK, Khan MN, Fahad S, Xu ZH, Hu LY. 2020. Coupling of biochar with nitrogen supplements improve soil fertility, nitrogen utilization efficiency and rapeseed growth. Agronomy. 10:1661. doi:10.3390/agronomy10111661.
- Lehmann J, Rillig MC, Thies J, Masiello CA, Hockaday WC, Crowley D. 2011. Biochar effects on soil biota - a review. Soil Biol Biochem. 43:1812–1836. doi:10.1016/j.soilbio.2011.04.022.
- Li C, Yang W, Zhou B, Zhang Y, Lin Y, Xing S. 2021. Effects of biochar based fertilizer on soil nutrients, tea output and quality in an acidified tea field. Chin J Soil Sci. 52:387–397.
- Li DD, Tian MY, Cai J, Jiang D, Cao WX, Dai TB. 2013a. Effects of low nitrogen supply on relationships between photosynthesis and nitrogen status at different leaf position in wheat seedlings. Plant Growth Regul. 70:257–263. doi:10.1007/s10725-013-9797-4.
- Li MX, Xu J, Guo R, Liu Y, Wang SY, Wang H, Abd U, Shi LX. 2019. Identifying the metabolomics and physiological differences among Soja in the early flowering stage. Plant Physiol Biochem. 139:82–91. doi:10.1016/j.plaphy.2019.03.012.
- Li Y, Ren BB, Ding L, Shen QR, Peng SB, Guo SW. 2013b. Does chloroplast size influence photosynthetic nitrogen use efficiency? PLoS One. 8:e62036. doi:10.1371/journal.pone.0062036.
- Liang J, He JX. 2018. Protective role of anthocyanins in plants under low nitrogen stress. Biochem Biophys Res Commun. 498:946–953. doi:10.1016/j.bbrc.2018.03.087.
- Lin QY, Zhang L, Riaz M, Zhang MY, Xia H, Lv B, Jiang CC. 2018. Assessing the potential of biochar and aged biochar to alleviate aluminum toxicity in an acid soil for achieving cabbage productivity. Ecotoxicol Environ Saf. 161:290–295. doi:10.1016/j.ecoenv.2018.06.010.
- Liu DP, Li MX, Liu Y, Shi LX. 2020. Integration of the metabolome and transcriptome reveals the resistance mechanism to low nitrogen in wild soybean seedling roots. Environ Exp Bot. 175:104043. doi:10.1016/j.envexpbot.2020.104043.
- Liu H, Zhou Q, Li J, Zhang L, Zhang M, Sun M, Liu Z, Chen J. 2016. Effect of biochar application amount on the soil improvement and the growth of flue-cured tobacco. J Nucl Agric Sci. 30:1411–1419.
- Liu LY, Teng K, Fan XF, Han C, Zhang H, Wu JY, Chang ZH. 2022. Combination analysis of single-molecule long-read and Illumina sequencing provides insights into the anthocyanin accumulation mechanism in an ornamental grass, Pennisetum setaceum cv. Rubrum. Plant Mol Biol. 109:159–175. doi:10.1007/s11103-022-01264-x.
- Liu X, Wu P, Li C, Wang Y, Zhang S, Chen J. 2021. Effects of biochar and reduced nitrogen application on photosynthetic characteristics of sugar beet under saline-alkali stress. J Northwest A & F Univ Nat Sci Ed. 49:108.
- Liu Y, Zhao Z, Fu X, Zhan E, Jiang Y, Huang W, Chen X, Li C, Zhao F, Yu H. 2014. Effects of adjusting fertilization on flue-cured tobacco growth and leaf quality in Zhaotong. Chin Tob Sci. 35:32–37.
- Luo J, Li H, Liu TX, Polle A, Peng CH, Luo ZB. 2013. Nitrogen metabolism of two contrasting poplar species during acclimation to limiting nitrogen availability. J Exp Bot. 64:4207–4224. doi:10.1093/jxb/ert234.
- Makoto K, Tamai Y, Kim YS, Koike T. 2010. Buried charcoal layer and ectomycorrhizae cooperatively promote the growth of Larix gmelinii seedlings. Plant Soil. 327:143–152. doi:10.1007/s11104-009-0040-z.
- Matiu M, Ankerst DP, Menzel A. 2017. Interactions between temperature and drought in global and regional crop yield variability during 1961-2014. PLoS One. 12:e0178339. doi:10.1371/journal.pone.0178339.
- Misra AN, Srivastava A, Strasser RJ. 2001. Utilization of fast chlorophyll a fluorescence technique in assessing the salt/ion sensitivity of Mung bean and Brassica seedlings. J Plant Physiol. 158:1173–1181. doi:10.1078/S0176-1617(04)70144-3.
- Mu XH, Chen QW, Chen FJ, Lixing YX, Mi GH. 2016. Within-Leaf nitrogen allocation in adaptation to Low nitrogen supply in maize during grain-filling stage. Front Plant Sci. 7:11. doi:10.3389/fpls.2016.00699.
- Mu XH, Chen YL. 2021. The physiological response of photosynthesis to nitrogen deficiency. Plant Physiol Biochem. 158:76–82. doi:10.1016/j.plaphy.2020.11.019.
- Qian ZZ, Tang LZ, Zhuang SY, Zou Y, Fu DL, Chen X. 2020. Effects of biochar amendments on soil water retention characteristics of red soil at south China. Biochar. 2:479–488. doi:10.1007/s42773-020-00068-w.
- Quan XY, Zeng JB, Chen G, Zhang GP. 2019. RDWN6XB, a major quantitative trait locus positively enhances root system architecture under nitrogen deficiency in rice. BMC Plant Biol. 19:12. doi:10.1186/s12870-018-1620-y.
- Que YX, Su YC, Guo JL, Wu QB, Xu LP. 2014. A global view of transcriptome dynamics during sporisorium scitamineum challenge in sugarcane by RNA-Seq. PLoS One. 9:e106476. doi:10.1371/journal.pone.0106476.
- Sarma B, Borkotoki B, Narzari R, Kataki R, Gogoi N. 2017. Organic amendments: effect on carbon mineralization and crop productivity in acidic soil. J Cleaner Prod. 152:157–166. doi:10.1016/j.jclepro.2017.03.124.
- Shen YF, Zhu LX, Cheng HY, Yue SC, Li SQ. 2017. Effects of biochar application on CO2 emissions from a cultivated soil under semiarid climate conditions in northwest China. Sustainability. 9:1482. doi:10.3390/su9081482.
- Spreitzer RJ, Salvucci ME. 2002. RUBISCO: structure, regulatory interactions, and possibilities for a better enzyme. Annu Rev Plant Biol. 53:449–475. doi:10.1146/annurev.arplant.53.100301.135233.
- Strasser RJ, Tsimilli-Michael M, Srivastava A. 2004. Analysis of the chlorophyll a fluorescence transient. Dordrecht: Springer Netherlands; p. 19:321–362. doi:10.1007/978-1-4020-3218-9_12.
- Strassert RJ, Srivastava A, Govindjee. 1995. Polyphasic chlorophyll a fluorescence transient in plants and cyanobacteria*. Photochem Photobiol. 61: 32–42. doi:10.1111/j.1751-1097.1995.tb09240.x.
- Tantray AY, Bashir SS, Ahmad A. 2020. Low nitrogen stress regulates chlorophyll fluorescence in coordination with photosynthesis and Rubisco efficiency of rice. Physiol Mol Biol Plants. 26:83–94. doi:10.1007/s12298-019-00721-0.
- Tazoe Y, Noguchi K, Terashima I. 2006. Effects of growth light and nitrogen nutrition on the organization of the photosynthetic apparatus in leaves of a C4 plant, Amaranthus cruentus. Plant Cell Environ. 29:691–700. doi:10.1111/j.1365-3040.2005.01453.x.
- Wang Y, Wang JC, Guo DD, Zhang HB, Che YH, Li YY, Tian B, Wang ZH, Sun GY, Zhang HH. 2021. Physiological and comparative transcriptome analysis of leaf response and physiological adaption to saline alkali stress across pH values in alfalfa (Medicago sativa). Plant Physiol Biochem. 167:140–152. doi:10.1016/j.plaphy.2021.07.040.
- Xu G, Guo W, Li Z, Wang C, Xu Y, Jin J, Zhou H, Deng S. 2022. Transcriptomic insights into the regulatory networks of chilling-induced early flower in tobacco (Nicotiana tabacumL.). J Plant Interact. 17:496–506. doi:10.1080/17429145.2022.2055175.
- Ye L, Shen W, Zheng B, Song T, Chen G, Lu C. 2013. Changes of photosynthetic membrane function and protein complexes in flag leaves of Liangyoupeijiu during leaf senescence. Acta Agron Sin. 39:2030–2038. doi:10.3724/SP.J.1006.2013.02030.
- Zhang C, Zhang Y, Lu Z, Liu L, Yang C. 2017. Effect of Low nitrogen stress on the seedling growth and root physiological traits of Fagopyrum tataricum cultivars with different low-N treatments. Acta Bot Boreali-Occidentalia Sin. 37:1331–1339.
- Zhang HH, Xu ZS, Guo KW, Huo YZ, He GQ, Sun HW, Guan YP, Xu N, Yang W, Sun GY. 2020. Toxic effects of heavy metal Cd and Zn on chlorophyll, carotenoid metabolism and photosynthetic function in tobacco leaves revealed by physiological and proteomics analysis. Ecotoxicol Environ Saf. 202:110856. doi:10.1016/j.ecoenv.2020.110856.
- Zhang HH, Zhong HX, Wang JF, Sui X, Xu N. 2016a. Adaptive changes in chlorophyll content and photosynthetic features to low light in Physocarpus amurensis Maxim and Physocarpus opulifolius “Diabolo”. PeerJ. 4:e2125. doi:10.7717/peerj.2125.
- Zhang YJ, Lin F, Wang XF, Zou JW, Liu SW. 2016b. Annual accounting of net greenhouse gas balance response to biochar addition in a coastal saline bioenergy cropping system in China. Soil Tillage Res. 158:39–48. doi:10.1016/j.still.2015.11.006.
- Zhao M, Sun J, Wang J, Xu H, Tang L, Chen W. 2011. Global genome expression analysis of photosynthesis-related genes under Low nitrogen stress in rice flag leaf. Sci Agric Sin. 44:1–8.
- Zhong C, Jian SF, Huang J, Jin QY, Cao XC. 2019. Trade-off of within-leaf nitrogen allocation between photosynthetic nitrogen-use efficiency and water deficit stress acclimation in rice (Oryza sativa L.). Plant Physiol Biochem. 135:41–50. doi:10.1016/j.plaphy.2018.11.021.
- Ziehe D, Dünschede B, Schünemann D. 2018. Molecular mechanism of SRP-dependent light-harvesting protein transport to the thylakoid membrane in plants. Photosynth Res. 138:303–313. doi:10.1007/s11120-018-0544-6.