ABSTRACT
Natural killer (NK) cells are a population of innate type I lymphoid cells essential for early anti-viral responses and are known to modulate the course of humoral and cellular-mediated T cell responses. We assessed the role of NK cells in allogeneic CD8 T cell-mediated responses in an immunocompetent mouse model across an MHC class I histocompatibility barrier to determine its impact in therapeutic clinical interventions with polyclonal or monoclonal antibodies (mAbs) targeting lymphoid cells in transplantation. The administration of an NK cell depleting antibody to either CD8 T cell replete or CD8 T cell-depleted naïve C57BL/6 immunocompetent mice accelerated graft rejection. This accelerated rejection response was associated with an in vivo increased cytotoxic activity of CD8 T cells against bm1 allogeneic hematopoietic cells and bm1 skin allografts. These findings show that NK cells were implicated in the control host anti-donor cytotoxic responses, likely by competing for common cell growth factors in both CD8 T cell replete and CD8 T cell-depleted mice, the latter reconstituting in response to lymphopenia. Our data calls for precaution in solid organ transplantation under tolerogenic protocols involving extensive depletion of lymphocytes. These pharmacological biologics with depleting properties over NK cells may accelerate graft rejection and promote aggressive CD8 T cell cytotoxic alloresponses refractory to current immunosuppression.
Introduction
Natural killer (NK) cells are a population of granular lymphoid cells phenotypically characterized by expression of NK1.1 or DX5 and the lack of CD3 and T cell receptor in C57BL/6 mice (B6 mice).Citation1, Citation2 These bone marrow-derived innate cells have evolved to attack and kill viral infected and transformed cells in a non-MHC restricted manner without the need of prior sensitization. A balance of signals orchestrated through inhibitory and activating receptors on NK cells interacting with MHC class I and non-MHC molecules expressed on target cells triggers NK cell function. This array of germline-encoded inhibitory and activating receptors detects alterations in the molecular composition of the target cells due to infection, malignant transformation or cellular stress, instructing NK cells to respond to altered self.Citation1,Citation3–Citation5
NK cells are also very sensitive to changes on MHC class I expression. Thus, cells expressing MHC class I mismatched molecules or autologous cells lacking self-MHC class I molecules or cells in which self-MHC class I molecules are down-regulated (missing self-theory) become targets for NK cell-mediated cytolysis.Citation5–Citation7 Moreover, lethally irradiated F1 hybrid mice spontaneously reject parental bone marrow cells (BMCs) or hematopoietic cells, a phenomenon against the laws of transplantation named “hybrid resistance”, first described by Snell et al., and then confirmed by others.Citation8–Citation11
Compelling evidence points to NK cells as regulators of both humoral and cellular immune responses in viral infections. In different experimental settings, antibody-mediated NK cell depletion modulates the course of CD8 T cell responses by increasing CD8 T cell priming in the acute phase of viral infection and enhancing memory responses characterized by an increase of central memory T cells (CD44hi CCR7+ CD62L+).Citation12,Citation13 Similarly, depletion of NK cells leads to increased numbers of T follicular helper cells (Tfh) and germinal center B cells, resulting in more sustained antibody responses after acute and chronic viral infections.Citation14,Citation15
The role of NK cells in transplant rejection and tolerance is controversial and paradoxical, meaning that they can promote either rejection or tolerance. NK cells reject allogeneic bone marrow cellsCitation2,Citation16 and participate in the rejection of solid organs.Citation17 NK cells also promote cardiac allograft vasculopathy (CAV) when parental heart grafts are placed into F1 recipients, in which NK cells are the only active mechanism of rejection.Citation18 NK cells may also contribute to prolong graft survival and transplant tolerance by eliminating donor-derived antigen-presenting cells (APC) and limiting the direct pathway of antigen presentation and T cell priming in draining lymph nodes.Citation19,Citation20
NK cells can also modulate CD8 T cell-mediated graft rejection downregulating CD8 T cell homeostatic proliferation in response to lymphopenia by competing for common growth factors, such as interleukin (IL)-15.Citation21 Moreover, the population of NK cells expressing low rather than high levels of CD27 has been shown to promote allograft survival by limiting the homeostatic proliferation and expansion of interferon-γ–producing memory CD8+ T cells under costimulatory blockade.Citation22
In this study, our aim was to address whether NK cells could modulate CD8 T cell-mediated response in an immunocompetent mouse model of alloreactivity across an MHC class I barrier in which rejection is mainly mediated by CD8 T cells and determine its effect in therapeutic interventions involving depletion of NK and/or CD8 T cells.Citation23–Citation25 Herein, we provide evidence that the removal of NK cells (CD3−DX5+ population and within this, the DX5+NKp46+ subpopulation) accelerated skin graft rejection in immunocompetent B6 recipients in both CD8 T cell replete and CD8 T cell-depleted recipients. These findings revealed that NK cells regulate allogeneic CD8 T cell responses to skin allografts when stimulated through the direct pathway of antigen presentation not only in immunocompetent recipients, but also in CD8 T cell-depleted recipients.
Results
Naïve NK cells regulate CD8 T cell-mediated rejection of allogeneic hematopoietic target cells in vivo in immunocompetent recipients
To determine whether NK cells regulate the course of graft rejection in immunocompetent mice, we chose an experimental mouse model in which donor and recipient differed in Kb allele (bm1 donor to B6 recipient).Citation26 In this transplant setting, alloreactive effector CD8 T cells activated through the direct pathway of antigen presentation are the main mediators of rejection.Citation23
In order to confirm the role of CD8 T cells in targeting bm1 alloantigen, carboxyfluorescein succinimidyl ester (CFSE)-labeled bm1 splenocytes were adoptively transferred intravenously (i.v.) into B6 recipients that were depleted of either NK cells or CD8 T cells. In un-manipulated hosts, there was a gradual clearance of donor bm1 cells (); however, in mice depleted of CD8 T cells no cytolytic activity against bm1 target cells was observed 5 days post-transfer (). The cytotoxic activity in this group eventually began to recover in both spleen () and in peripheral lymph nodes (pLNs) (), which was likely due to the declining antibody concentration in the periphery and reconstitution of the CD8 T cell compartment through thymic output and peripheral homeostatic proliferation in response to lymphopenia. Interestingly, the depletion of NK cells led to an increased clearance of CFSE-labeled bm1 cells at day 5 after adoptive transfer compared to isotype-treated control in spleen and pLNs () such that bm1 cells were practically undetectable by day 15 in either spleen () or pLNs ().
Figure 1. Host NK cells regulate CD8 T cell-mediated cytotoxic responses in vivo against bm1 hematopoietic target cells.
Naïve B6 mice were treated at day 0 and day 7 with 1 mg/mouse of isotype control (red bars), anti-NK1.1 antibody (black bars) or anti-CD8 antibody (blue bars). The same day, the three groups of mice were injected with CFSE-labeled female bm1 splenocytes (15 × 10Citation6 splenocytes). The absolute number of bm1 hematopoietic cells remaining in spleen and peripheral lymph nodes (pLNs) (inguinal plus axillary and brachial) was evaluated at day 5, 9 and 15 after cell transfer. Results are expressed as mean ± SD and the data comes from a pool of two experiments with three mice per experimental group.*, p < 0.05; **, p < 0.005; ***, p < 0.0005 were considered statistically significant.
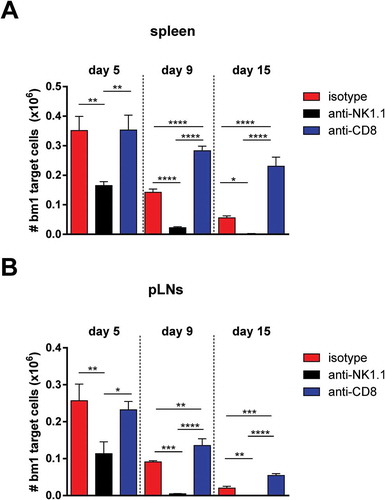
Taken together, these data confirm that CD8 T cells are the major immune cell population involved in the recognition and clearance of cells bearing the KbmCitation1 alloantigen in this setting. Furthermore, consistent with other studies, NK cells appeared to hamper cytotoxic T lymphocyte (CTL)-mediated clearance of bm1 cells, suggesting a role for NK cells in modulating CD8 T cell responses to alloantigen. These findings prompted us to analyze in more detail the role of NK cells in a more relevant mouse model of skin allotransplantation across an MHC class I mismatched barrier in immunocompetent recipients.
NK cell depletion accelerates skin graft rejection across an MHC class I histocompatibility barrier in immunocompetent mice
We designed an experimental approach to evaluate the relevance of the above mentioned observations and to assess the outcome of skin graft survival in the context of immunosuppressive depleting regimens targeting either CD8 or NK cells. This reflects a particular scenario of clinical interventions such as, for example, immunosuppressive regimens based on thymoglobulin or anti-CD52 antibody therapy (alemtuzumab) with depleting activity on lymphoid cells.Citation27,Citation28
As shown in , depletion of NK cells at the time of transplantation accelerated bm1 skin graft rejection in B6 recipients (median survival time (MST): 12 days) compared to isotype-treated controls (MST: 15 days) (p < 0.0001). This suggests that NK cells regulate CD8 T cell-mediated rejection in immunocompetent recipient mice. Interestingly, depletion of NK cells together with CD8 T cells also significantly accelerated bm1 skin graft rejection in B6 immunocompetent recipients compared to CD8 T cell-depleted B6 recipients (MST: 20 days versus 26 days, p < 0.0001) ().
Figure 2. Depletion of NK cells accelerates bm1 skin allograft rejection in both CD8 T cell replete and CD8 T cell-depleted B6 recipients.bm1 skin allografts placed on B6 recipients were followed for 40 days after transplantation. C57BL/6 recipient mice were treated at day 0 and day 7 with 1 mg of either isotype control or anti-NK1.1 depleting antibody (A), or with anti-CD8 mAb or anti-CD8 mAb plus anti-NK1.1 mAb (B). The survival curve was plotted following the Kaplan–Meier life table method and the statistical analysis was performed using the long-rank test. The survival curve is the global result of a pool of 6 experiments. Visual macroscopic inspection of the bm1 skin grafts (C) and hematoxylin and eosin (H/E) staining of skin tissue sections (D) of the four experimental groups are depicted at day 13 and day 21 post-Tx. bm1 skin graft rejection occurred at day 13 post-Tx in NK cell depleted recipients and at day 21 post-Tx in CD8/NK cell depleted recipients few days in advance than in isotype control or anti-CD8 T cell depleted mice, respectively. Severe rejection of donor bm1 tail skin was accompanied with necrosis of donor epithelium and intense dermal mononuclear infiltration. Black dotted ellipse highlights massive necrosis of skin epithelium and dermis underneath of donor bm1 skin graft in the tissue section. Statistical significance is indicated as follows: *p < 0.05, **p < 0.005, ***p < 0.0005, and ns, non-significant.
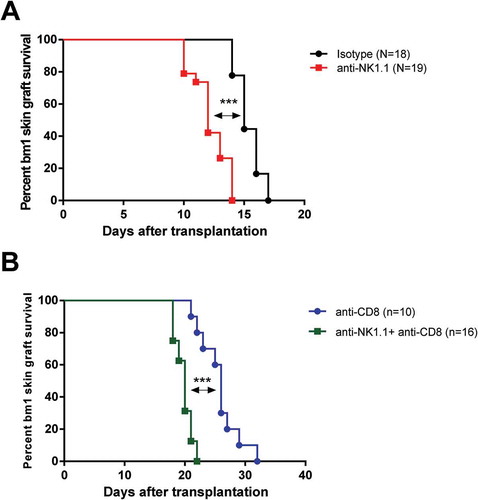
Figures 2C and 2D show representative macroscopic and microscopic photographs of skin bm1 allografts at day 13 and day 21 post-transplantation (post-Tx, respectively. Bm1 skin allografts transplanted to B6 recipients were rejected with an MST of 12 days in NK cell-depleted mice whereas bm1 grafts remained intact in isotype control-treated mice at this time, although they were eventually rejected (MST: day 15 post-Tx). Macroscopic signs of rejection and histopathological lesions were clearly detectable in NK cell-depleted recipients and in anti-NK1.1 plus anti-CD8 T cell-depleted B6 mice, at days 13 and day 21 post-transplantation, respectively (Figures 2C and 2D).
Moreover, our work confirms previous evidences supporting the lack of alloantibody response against H-2KbmCitation1 class I conformational epitopes in C57BL/6 recipients,Citation23 as either no detectable Tfh expansion (CD4+CXCR5+PD-1+) cells or germinal center formation (B220+GL7+Fas+) or host anti-donor humoral response was seen, as shown in supplementary Figure 1.
Overall, the data indicate that naïve or homeostatically dividing NK cells regulate the alloreactive cytotoxic response of CD8 T cells in CD8 T cell replete and CD8 T cell-depleted mice, respectively.
NKT cell and NK cell expansion in draining lymph nodes is associated with ongoing alloreactive CD8 T cell-mediated responses and graft rejection
We first monitored the effectiveness of the depleting NK cell therapy at day 13 and day 21 post-Tx, which corresponded with the time points around which rejection occurred in NK cell-depleted and in CD8/NK1.1 cell-depleted B6 mice, respectively. The population of NKT cells (DX5+ CD3+), a priori susceptible to depletion by anti-NK1.1 antibody, did not show any significant reduction in cell number compared to isotype-treated control group in either draining or non-draining lymph nodes at any of the two time points evaluated (day 13 post-Tx, , upper left panel and day 21 post-Tx, , upper right panel). It is noteworthy that NKT cells increased significantly in draining compared to non-draining pLNs at day 13 post-Tx in isotype-treated mice close to rejection and in NK cell-depleted mice undergoing rejection, respectively (, upper left panel). There was also a significant increase in NKT cell numbers at day 21 post-Tx in CD8 T cell-depleted mice near the time of rejection and in CD8/NK1.1 cell-depleted mice undergoing rejection (, upper right panel). However, there was no significant difference in the number of NKT cells between treatment groups in either non-draining or draining pLNs at day 13 and day 21 after Tx (, upper left and right panels). These data show that NKT cells expand in the draining pLNs during graft rejection.
Figure 3. NKT cell and NK cell expansion in draining pLNs during the course of graft rejection in both CD8 T cell replete and CD8 T cell-depleted recipients.Non-draining and draining axillary and brachial pLN from each experimental group of bm1 skin-grafted B6 recipients were collected at two time points after transplantation. The absolute number of NKT (DX5+CD3+) cells, non-T NK cells (DX5+CD3− cells and DX5+NKp46+ cells) was quantified at day 13 (left side panel) and day 21 (right side panel) post-Tx. Results are displayed as mean ± SD. Statistical significance and p value was calculated using unpaired Student’s t test for the comparison of means between draining versus non-draining pLN in each experimental group. One way ANOVA was applied for the comparison of means among experimental groups within non-draining or draining pLNs. The following criterion of statistical significance was used: *, p < 0.05; **, p < 0.005; ***, p < 0.0005. These plots display data pooled from three independent experiments with three mice per group.
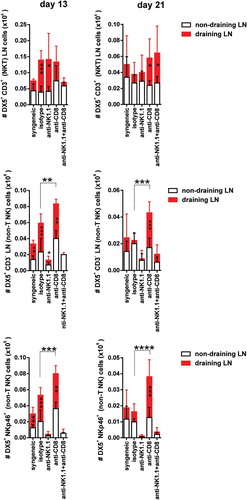
NK cells (DX5+ CD3−) exhibited a significant reduction in cell numbers in draining pLNs after depletion with anti-NK1.1 mAb compared to isotype control at day 13 and day 21 post-Tx, but did not completely eliminate this cell population (, middle left and right panels). The most sensitive NK cell population to antibody-mediated depletion was, however, the NK cell population co-expressing DX5 and NKp46 surface markers. Once eliminated from the periphery at day 13 post-Tx (, lower left panel), the rate of repopulation was slow and the absolute counts were still profoundly reduced at day 21 post-Tx (, lower right panel).
Both subsets of NK cells (DX5+CD3− and DX5+NKp46+) expanded in draining compared to non-draining pLNs in isotype-treated control at day 13 after transplantation. NK cell numbers also increased significantly, probably as a result of active proliferation or recruitment in draining compared to non-draining pLNs at day 13 and day 21 post–Tx in CD8 T cell-depleted mice (, middle and lower left and right panels) Furthermore, the number of NK cells was increased in the draining pLNs of CD8 T cell-depleted mice compared to the isotype-treated group at both day 13 post-Tx (, middle and lower left panels) and at day 21 post-Tx (, middle and lower right panels).
Our data highlighted that NK cells increased in cell numbers after CD8 T cell depletion, taking advantage of the open space left by CD8 T cells, preferentially in draining pLNs where the allogeneic immune response is occurring.
Globally, these findings are in favor of the notion that NK cells compete with CD8 T cells for space in pLNs and exploit their niche. Moreover, NK cells, and in particular NKp46 expressing cells, represent the most likely effector innate cells involved in the regulation of allogeneic CD8 T cell-mediated responses stimulated through the direct pathway of antigen presentation.
Effective CD8 T cell depletion and CD4 and CD8 peripheral expansion of naïve and memory type T cells in draining lymph nodes
We next evaluated the effectiveness of CD8 T cell-specific depletion with anti-CD8 mAb treatment.Citation29 This depleting therapy was very effective because the absolute cell counts dropped profoundly after the administration of two doses of anti-CD8 depleting antibody, as assessed in draining and non-draining lymph nodes at day 13 post-Tx (, upper left panel). The absolute counts of CD8 T cells were still very low at day 21 post-Tx (, upper right panel), although an incipient recovery was already detectable at that time point, which was significantly higher in draining than in non-draining pLNs. Despite the low number of CD8 T cells observed at day 21 post-Tx, the frequency of alloreactive cells recognizing bm1 histoincompatible antigens was sufficient to initiate skin graft rejection in NK/CD8 cell-depleted B6 mice (), whereas the presence of NK cells delayed rejection of bm1 skin grafts in CD8 T cell-depleted mice ().
Figure 4. Expansion of CD4 T cells and CD8 T cells in draining pLNs of B6 mice after skin transplantation across an MHC class I barrier.
Non-draining and draining axillary and brachial pLNs from each experimental group of bm1-skin grafted B6 recipients were collected at two time points after transplantation.The absolute number of CD8 T cells (upper panels) and the absolute number of CD4 T cells (lower panels) at day 13 and day 21 post-Tx is depicted in draining and non-draining lymph nodes. Results are displayed as mean ± SD. Statistical significance and p value was calculated using unpaired Student’s t test for the comparison of means between draining versus non-draining pLN in each experimental group. The following criterion of statistical significance was used: *, p < 0.05; **, p < 0.005; ***, p < 0.0005. These plots display data pooled from three independent experiments with three mice per group.
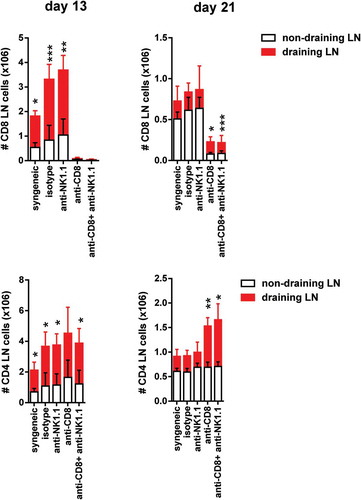
A significant peripheral expansion of CD8 T cells was found in draining compared to non-draining pLNs in isotype-treated and anti-NK1.1-treated groups at day 13 post-Tx (, upper left panel). CD8 T cells also expanded significantly at day 21 post-Tx in draining compared to non-draining pLNs in CD8 T cell-depleted and CD8/NK1.1 cell-depleted mice (, upper right panel).
We then analyzed the peripheral clonal expansion of CD4 T cells in non-draining versus draining pLNs. We included CD4 T cells in the analysis despite the fact that CD4 T cell depletion alone does not change the course of allograft rejection in this mouse model. However, it is known that depletion of CD4 T cells synergizes with depletion of CD8 T cells and prolongs graft survival beyond that observed in CD8 T cell-depleted mice, a clear indication that CD4/CD8 T cell collaboration is necessary to a certain extent for effective CD8 T cell-mediated rejection in this model.Citation23,Citation24 The absolute number of CD4 T cells increased in draining pLNs of all experimental groups compared to non-draining pLNs at day 13 post-Tx (, lower left panel). CD4 T cells also expanded significantly after CD8 T cell depletion or in CD8/NK1.1 cell depleted mice (, right panel). These observations suggest that CD4 T cells were also active during graft rejection in draining pLNs of rejecting mice.
To assess the effect of NK cell depletion on the adaptive immune response to skin allografts, the number of alloreactive CD4 and CD8 effector and central memory T cells was determined. A significant increase in the number of CD4 and CD8 T effector memory (TEM, CD44high CD62Llow) and T central memory (TCM, CD44high CD62Lhigh) cells in isotype and anti-NK1.1-treated mice was observed at day 13 post-Tx in draining pLNs compared to non-draining pLNs (). Alloreactive CD4 T cells and CD8 T cells as well as TEM and TCM cells counts increased significantly in draining compared to non-draining pLNs in isotype and NK1.1-treated groups at day 13 post-Tx and also in CD8 and CD8/NK cell depleted recipient at day 21 post-Tx (, ).
Figure 5. Increased of CD8 T cells and CD4 memory type T cell numbers in draining pLNs of B6 mice after skin transplantation across an MHC class I barrier.The absolute number of CD8 and CD4 TEM cells at day 13 post-Tx (upper left panel) (A) and day 21 post-Tx (upper right panel) (B) and the absolute number of CD8 and CD4 TCM cells at day 13 post-Tx (lower left panel) (A) and day 21 post-Tx (lower right panel) (B) is depicted in non-draining and draining lymph nodes. Results are displayed as mean ± SD. Statistical significance and p value was calculated using unpaired Student’s t test for the comparison of means between draining versus non-draining pLN in each experimental group. The following criterion of statistical significance was used: *, p < 0.05; **, p < 0.005; ***, p < 0.0005. These plots display data pooled from two independent experiments.
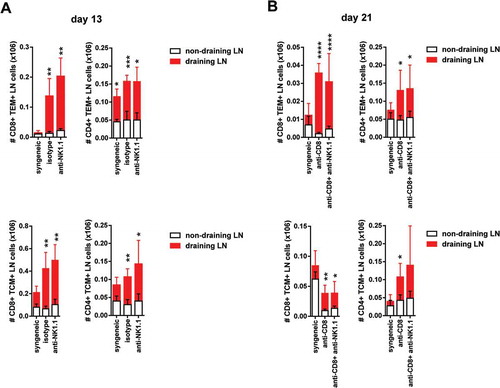
This peripheral increase of CD4 and CD8 TEM and TCM cell numbers suggest an active process of clonal expansion of both cell populations in response to donor bm1 antigens and T cell differentiation towards memory cells with cytotoxic function.
Enhanced in vivo cytotoxic activity against bm1 target cells after NK cell depletion in naïve immunocompetent mice and in CD8 T cell-depleted recipients after skin graft rejection.
To evaluate the degree of sensitization of B6 recipients after exposure to allogeneic bm1 skin grafts at the time of rejection, an in vivo cytotoxic assay was implemented. Thus, at day 13 post-Tx, NK cell-depleted B6 recipients efficiently killed CFSE-labeled allogeneic bm1 splenocytes in the spleen in 24 hours, whereas the other groups did not exhibit any remarkable cytotoxic activity (, upper left panel). In the peripheral lymph nodes, however, isotype-treated control mice showed evidence of presensitization and cytotoxic activity to some extent, although they rejected a few days later (, upper right panel).
Figure 6. NK cell depletion augmented in vivo CTL activity of skin-grafted sensitized allogeneic CD8 T cells against bm1 hematopoietic target cells.bm1 skin-grafted B6 recipients were challenged with CFSE-labeled target bm1 cells at day 13 (time of rejection for NK cell-depleted group) and day 21 (time of rejection for CD8/NK cell-depleted group) after Tx. The killing of bm1 hematopoietic target cells was evaluated as the total cell counts of bm1 cells remaining in spleen (A) and pLNs (B) 24 hours after i.v. injection of 15 × 10Citation6 of CFSE-labeled bm1 target splenocytes at day 13 and day 21 after Tx. Results are displayed from one representative experiment out of two with three mice for each experimental group. Results are displayed as mean ± SD. *, p < 0.05; **, p < 0.005; ***, p < 0.0005 were considered statistically significant.
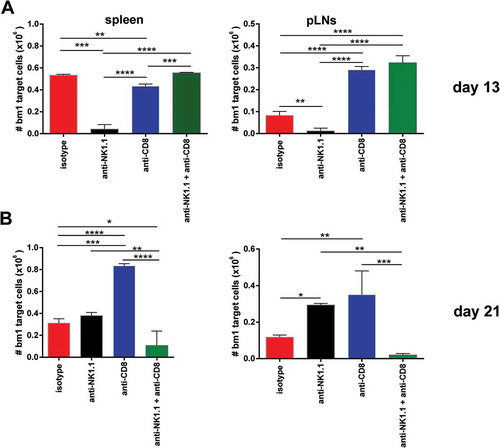
At day 21 post-Tx, NK/CD8 cell-depleted B6 recipients showed strong sensitization with high cytotoxic activity against bm1 target cells in spleen compared to CD8 T cell-depleted B6 mice (, lower left panel). Some residual cytotoxic activity was still present in sensitized isotype-treated B6 mice and NK cell-depleted mice that had already rejected their grafts two weeks previously. This observed cytotoxic activity in the spleen was similar and correlated well to that recorded in peripheral lymph nodes (, lower right panel).
We performed an experiment at the early time point after bm1 skin grafting to assess the frequency of bm1 antigen specific CD8 T cells in draining compared to non-draining lymph nodes using the CD107a degranulation assay.Citation30 Depletion of NK cells led to a modest increase of the frequency of CD8 T cells expressing CD107a responding to alloantigen compared to isotype-treated mice. This slight increase in CD8+CD107a+ T cells detected in draining lymph nodes is in line with the claim of a low frequency of T cells responding to bm1 antigens ().
Figure 7. NK cell depletion increases donor alloantigen specific CD8+ T cells expressing CD107a.At the time of rejection initiation in NK-cell depleted mice, 1.5 × 10Citation5 cells from non-draining and draining pLNs (axillar and brachial) were isolated from syngeneic or allogeneic bm1 skin grafted treated either with isotype control or anti-NK1.1 antibody. Then, syngeneic and allogeneic lymphocytes were re-stimulated in vitro with 0.5 × 10Citation5 allogeneic syngeneic control and bm1 allogeneic mature BM-DC per well for 1 hour in the presence of PE-conjugated isotype control or PE-conjugated anti-CD107a mAb. Finally, the Golgi plug monensin was added and incubated 4 more hours. This figure is a representative experiment of two performed with similar results using one mouse/experimental group/experiment.
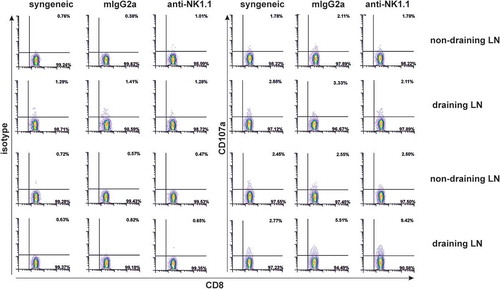
Globally, these data reinforce the notion that NK cells modulate CD8 T cell cytotoxic responses against class I MHC mismatched target cells because, in their absence, cytotoxic responses were more aggressive.
Discussion
The purpose of this work was to assess the role of host NK cells in the regulation of CD8 T cell mediated responses stimulated through the direct pathway of antigen presentation.Citation23–Citation25,Citation31 In this mouse model, alloreactive host CD8 T cells recognize directly unprocessed MHC class I mismatched KbmCitation1 antigen, whereas alloreactive host CD4 T cells are activated by donor and host dendritic cells (DCs) that shared MHC class II presenting donor KbmCitation1-derived peptides through the indirect pathway of antigen presentation.Citation25 The frequency of alloreactive CD4 T cells recognizing allogeneic peptides is very low, which is translated into limited IL-2 help to alloreactive CD8 T cells.Citation31 bm1-specific CD4+ T helper cells can see a peptide derived from the KbmCitation1 molecule encompassing the three mutations when presented by MHC class II molecules on donor/host APCs. Despite this low frequency of alloreactive CD4 T cells, they can synergize with alloreactive CD8 T cells contributing to some extent to the process of rejection across an MHC class I mismatched barrier.Citation23,Citation24,Citation31 In this experimental approach, the host anti-donor humoral immune response against bm1 alloantigens does not contribute to the rejection response because MHC class I KbmCitation1 in B6 mice does not trigger an alloantibody response. This was in line with an absolute lack of germinal center formation and CD4 Tfh cell expansion observed in this setting (supplementary Figure 1).
Herein, we provide evidence that innate NK cells play an essential role in delaying CD8 T cell alloreactive responses as depletion of NK cells accelerates bm1 skin graft rejection in CD8 T cell replete and in CD8 T cell-depleted B6 immunocompetent mice.
This immune regulatory behavior of NK cells controlling CD8 T cell-mediated immunity may have evolved to prevent aggressive cytolytic responses against infected cells in the acute phase of viral infection to attenuate excessive tissue damage and the subsequent immunopathology. Most of the preliminary evidence on the role of NK cells regulating anti-viral CD8 T cell responses come from the LCMV model in which using different strains of virus and dosing, it is possible to recreate models of acute infection and chronic infection.Citation32,Citation33 NK cells can directly target CD8 T cells for elimination in a perforin- NKG2D-dependent manner or indirectly through the removal of mature DCs or viral-infected APCs, thus limiting costimulatory signals leading to attenuation of CD4 T cell help to CD8 T cells.Citation34 In the absence of NK cells, viral infection triggers CD8 T cell priming that enhances memory T cell responses, leading to an increase of the frequency of antigen-specific T cells. T cell differentiation is biased towards central memory T cells.Citation13 NK cells can act as rheostats, regulating CD4 T cell help to anti-viral CD8 T cells for the control of viral persistence and the fatal consequences of an intense acute immune response to viral infection, preventing the associated immunopathology.Citation35
In the field of transplantation, previous reports have provided some preliminary evidences on the modulatory role of NK cells in allogeneic CD8 T cell responses. Zecher et al. showed that the adoptive transfer of syngeneic T cell-enriched splenocytes into immunodeficient Rag2 gamma chain KO (NK cell deficient) recipients repopulate the peripheral CD8 T compartment quicker and 8-fold more CD8 T cells were recovered compared to Rag KO (NK cell sufficient) recipients. Similar results were observed in wild type mice depleted of both CD4/CD8 T cells compared to NK/T-cell depleted WT mice.Citation21 As opposed to Zecher et al., we could not prove that the removal of NK cells conferred an advantage to CD8 T cells to recover quicker and to a greater extent. In our transplant setting, CD8 T cells recovered after depletion to the same extent in NK sufficient as in NK-cell depleted mice, meaning that NK cell depletion did not alter the recovery of the total CD8 T cell numbers. Instead, NK cells exploited the CD8 T cell niche and expanded at day 21 post-Tx intensively in non-draining and draining lymph node, particularly in CD8 T cell-depleted mice. Interestingly, NK cells also expanded significantly at day 13 post-Tx in draining pLNs of isotype-treated control despite the presence of normal CD8 T cell numbers.
To reconcile these results and to account for the quicker recovery of CD8 T cells in immunodeficient Rag 2 KO mice and also in CD4 and CD8 T cell-depleted immunocompetent recipients, one can postulate that CD8 T cells recovering under homeostatic conditions in response to lymphopenia repopulate a peripheral compartment in which CD4 T cells were also depleted, whereas in our experiments, the CD4 T cell compartment was intact. It is known that, in the absence of either CD4 or CD8 T cells subpopulations, the open niche left by one population can be occupied by the other population and vice versa in such a manner that the total number of T cells remains similar to that present in normal mice. In other words, these two T cell populations share common resources and can partially invade each other’s niches. Cell loss of one cell type can be compensated by the other population.Citation36,Citation37
For the interpretation of our observations, we should consider two distinct scenarios because MHC class I mismatched skin grafts are rejected in our experimental setting in two different situations: CD8 T cell sufficient and CD8 T cell-depleted recipients undergoing homeostatic CD8 T cell repopulation.
In CD8 T cell sufficient mice, at day 13 post-Tx, the mechanism of action of NK cells may be mediated through the elimination of donor-derived DCs migrating to draining lymph nodes. This effect would lead to attenuation of the direct pathway of antigen presentation as shown by others.Citation19,Citation20,Citation38 Yu et al., compared alloantigen-driven T cell homeostatic proliferation in lymphopenic environments of immunodeficient mice after allogeneic skin graft transplantation between Rag2 deficient (NK sufficient) and Rag2/gamma chain double-deficient (NK deficient) mice adoptively transferred with syngeneic T cells. These authors found that deficiency in NK cells increased T cell proliferation and accelerated allograft rejection, whereas in NK cell sufficient mice, NK cells induced their immunomodulatory function by killing donor-derived dendritic cells that migrated to secondary lymphoid organs, leading to enhanced allograft survival.Citation19 These findings are coincident with those reported by Laffont et al., who also provided evidence for the role of NK cells recruited to the draining lymph nodes in the inhibition of the alloreactive T-cell activation through a perforin-mediated killing of donor-derived allogeneic dendritic cells.Citation20
In CD8 T cell-depleted mice undergoing homeostatic T cell repopulation, at day 21 post-Tx, the role of donor derived DC is likely to be negligible because of the gradual decay of this passenger leukocyte population from the graft. In this scenario, NK cells may delay CD8 T cell-mediated rejection for their ability to compete with the alloreactive CD8 T cells dividing in recipients devoid of CD8 T cells. In favor of this notion is that NK cells expanded in draining pLNs of CD8 T cell-depleted recipients at that time point, competing with the alloreactive CD8 T cells proliferating in response to bm1 alloantigens and homeostatically to lymphopenia, as well as with other T cells of the non-alloreactive repertoire. NK cells and CD8 T cells respond to similar cytokines (IL-2 and IL-15) for peripheral expansion, and therefore cross-competition for these common resources cross-regulate the ability of each population to survive, differentiate and proliferate homeostatically in response to lymphopenia.Citation39–Citation41
Newly generated cells (recent thymic emigrants and peripheral cell division) compete in the immune system with resident cells for survival.Citation36 Sometimes one population prevents other population from occupying a particular niche and from exploiting the resources there (interference competition). In other occasions, and more often, different populations may have common needs for resources present in the niche, which is in limited supply (exploitation competition).Citation36 The CD8 T cell independent niche can, however, be invaded by other cells, such as NK cells, an example of exploitation competition, which is in agreement with our results. This NK cell exploitation of the CD8 T cell niche may account for the delay of graft rejection. Our data in this sense is in agreement with that reported by Zecher´s work using immunodeficient and T cell-depleted immunocompetent recipients who provided evidence in favor of the role of NK cells in modulating CD8 T cell responses, not by killing homeostatically proliferating T cells, but by competing for T cell growth factors.Citation21 Our findings suggest that this competition for common resources may provide an advantage to NK cells that would reduce the availability of these cytokines to the alloreactive repertoire of CD8 T cell reacting against bm1 antigens. Therefore, reaching the threshold frequency of alloantigen specific CD8 T cells necessary to initiate rejection would take longer and consequently graft rejection would be delayed.
An alternative means for the control of CD8 T cell responses is the direct killing of activated CD8 T cells upon upregulation of NKG2D ligands 24–48 hours. Rabinovich et al. proved that syngeneic IL-2 activated NK cells can detect altered self-structures on activated CD8 T cells, not present in resting T cells. These changes in the molecular composition of activated CD8 T cells make them susceptible to NK cell-mediated killing by a NKG2D- and perforin-dependent mechanism.Citation42
This in vitro NK cytolytic activity is unlikely to occur in vivo because there are mechanisms of shielding T cells to prevent their destruction by NK cells, as demonstrated on activated CD4 T cells expressing Qa-1 that interact with inhibitory NKG2A NK receptor. This interaction confers CD4 T cells with resistance to NK cell-mediated lysis.Citation43 Another example of this shielding applies also to CD8 T cells expressing CD48 that inhibit NK cells through interaction with 2B4 (CD244).Citation44,Citation45 We found no evidence that NK cells were regulating the size of the CD8 T cell pool, as shown in , by killing activated alloreactive CD8 T cells because CD8 T cell counts were not affected in the absence of NK cells.
Conversion from naïve to memory T cell phenotype occurs in response to rapid homeostatic T cell proliferation in immunodeficient mice adoptively transferred with syngeneic splenocytes or in T cell-depleted immunocompetent recipients.Citation46 Homeostatic proliferation in response to lymphopenia is driven by TCR interaction with peptide-MHC on APCs and cytokines, such as IL-7 and IL-15.Citation47 We found that the alloreactive CD8 T cell response in draining pLNs was associated with a significant conversion of naïve to memory type cells (central memory and effector memory) and increased proliferation in both CD8 T cell replete (only responding to bm1 antigens at day 13 post-Tx) and CD8 T cell depleted mice (responding to bm1 antigens and to lymphopenia at day 21 post-Tx). NK cell depletion did not modify significantly the rate of conversion or memory type cell counts.
Although a priori unexpected, no significant changes were seen in CD8 T cell numbers in the draining lymph nodes of grafts undergoing rejection. To account for this, one possibility is that depletion of NK cells led to only a modest increase of the frequency of CD8 T cells expressing CD107a responding to alloantigen compared to isotype-treated mice, and this subtle change was not reflected in the total CD8 T cell counts. Secondly, the time point at which the experiment was analyzed coincided with the initiation of graft rejection and part of the antigen-specific response may have already become effector and migrated to the graft as effector CTLs.
One caveat from the use of anti-NK1.1 antibody for NK cell depletion is that it may also deplete NKT cells. Therefore, modulation of the immune response after administering anti-NK1.1 mAb might be assigned in part to depletion of NKT cells, which are also known to exhibit immunoregulatory functions. However, we showed that NKT cells were not reduced upon anti-NK1.1 antibody administration, which supports a role for NK and not NKT cells in modulating rejection in this setting. NKT cell may have persisted despite anti-NK1.1 mAb administration owing to a rapid recovery through thymic output or peripheral reconstitution or because decreased susceptibility to depletion attributable to the fact that NKT cells express intermediate levels of NK1.1 receptor. This result is in agreement with a report using a transgenic mouse model expressing constitutively soluble anti-NK1.1 antibody (clone PK136). In this mouse model, NK cells were efficiently eliminated, whereas NKT cell numbers were maintained and not affected by the chronic presence of the antibody.Citation48
The DX5+CD3− population of NK cells also exhibited resistance to depletion to some degree because anti-NK1.1 depleting therapy did not achieve complete elimination of this population in pLNs. The resistance of NK cells (DX5+CD3−) as opposed to the susceptibility of NKp46 cells to depletion makes more likely that the latter is endowed with the capacity to modulate CD8 T cell-mediated allogeneic responses in this model. However, both NKp46 cells as well as NK cells (DX5+CD3−) exhibited a competitive advantage over CD8 T cells and exploited the CD8 T cell niche when proliferating in draining pLNs, particularly in CD8 T cell-depleted recipients in the close proximity to rejection.
Evidence supporting a role for NKT cells in allograft rejection is scarce, with most reports relating to their ability to support the induction of tolerance.Citation49 This NKT cell activation, along with proinflammatory molecules secreted during graft rejection, may enhance their functional activity in such a manner that can provide an alternative source of help to the rejection process, particularly when the major effector T cells, that is, CD8 T cells, are depleted. Similarly to NK cells, NKT cells expanded significantly in CD8 replete and CD8 depleted in NK sufficient mice in close proximity to rejection. The association of NKT cell expansion with ongoing rejection favors the notion that these cells may also play a role helping alloreactive CD8 T cells to compensate for their low frequency, which is insufficient for the initiation of graft rejection. Indeed, according to some reports NKT cells represent potent helper cells for DC-dependent CTL priming.Citation50
The simultaneous depletion of NK cells and T cells may be undesirable in transplant recipients because of homeostatic division in response to lymphopenia converts the naïve phenotype of T cells into memory-like phenotype.Citation46 These T cells repopulating the peripheral compartment precipitate allograft rejection and resist protocols for tolerance induction.Citation51 Our data calls for precaution in solid organ transplantation under tolerogenic protocols involving extensive depletion of lymphocytes or leukocytes with agents such as ATG or alemtuzumab (anti-CD52 mAb) with partial depleting properties over NK cells. The global immunotherapeutic interventions, particularly with polyclonal antibodies against lymphoid cells that target NK cells secondarily, may accelerate graft rejection and promote aggressive CD8 T cell cytotoxic responses when proliferating in response to lymphopenia because these emerging T cells proliferating under homeostatic conditions are refractory to current immunosuppression.
Material and methods
Mice
Eight- to 12-week-old female C57BL-6J (B6, H-2Kb, Janvier Labs) and B6.C-H-2bmCitation1-By mice (H2-KbmCitation1, hereafter bm1 mice) were bred in our facility and used for the experiments described in this work. The Animal Welfare Committee of the University of Alcala de Henares (Madrid) in accordance with the European Guidelines for Animal Care and Use of Laboratory Animals approved all experiments with rodents.
Antibodies for in vivo use
Hybridoma cell lines secreting anti-NK.1.1 (clone PK136, mouse IgG2a) or anti-CD8 (clone 2.43, rat IgG2b) mAbs were grown in serum-free medium (SFM) (Thermo Fisher Scientific) supplemented with IgG-depleted fetal calf serum (FCS) (less than 1%) in spinner flasks. Citation29 Cell culture supernatants were pre-filtered, purified by affinity chromatography using a protein G-Sepharose column, dialyzed against phosphate-buffered saline (PBS) and subsequently filtered through 0.45-μm. Purified antibodies for in vivo use were stored frozen in PBS at a concentration of 1 mg/ml. Mouse IgG2a (clone MG2a-53) and rat IgG2b (RTK4530) were used as isotype control mAbs for in vivo use.
We established four different groups according to the following experimental set up: Group I: Isotype control, mixture of mouse IgG2a and rat IgG2b; Group II: Selective ablation of mouse NK cells with anti-NK1.1 mAb (clone PK136); Group III: Depletion of CD8 T cells with anti-CD8 mAb (clone 2.43) and Group IV: depletion of CD8 T cells and NK cells with anti-CD8 plus anti-NK1.1 mAb. All groups received 1 mg intraperitoneally of each mAb at the time of transplantation and at day 7 post-transplantation.
Antibodies for flow cytometry
The following list of flow cytometry antibodies were used for the staining of axillary and brachial non-draining and draining peripheral lymph node cell suspensions: CD3 (145-2C11, cat. #100,321), CD49b (DX5, cat. #108,908), CD8 (53–6.7, cat. #100,708), CD4 (GK1.5, cat. #100,432), CD62L (MEL-14, cat. #104,421), CD44 (IM7, cat. #103,030), CD335 (NKp46, 29A1.4, cat. #137,616)Citation52, B220 (RA3-6B2, cat. #103,206), CXCR5 (L138D7, cat. #145,504) and PD-1 (29F.1A12, cat. #135,218) mAbs were obtained from Biolegend. T and B activation antigen (GL7, cat. # 562,967) and Fas (15A7, cat. # 17–0951-82) were purchased from BD Biosciences and Thermo Fisher Scientific, respectively. All antibodies used in this study were properly titrated to determine optimal dilution to stain 0.5–1 × 10Citation6 cells per tube. Fc receptors were blocked by pre-incubating cell suspensions with 2 μg/ml of blocking anti-FcγR mAb (2.4G2) was produced and purified in house and used to reduce nonspecific binding before adding the specified biotin- or fluorochrome-labeled mAbs.Citation53 Biotinylated antibodies were detected with SA-Brilliant Violet 421. Dead cells and debris were excluded from acquisition by propidium iodide (PI). Flow cytometry acquisition was carried out on a Cyan 9 cytometer (Beckman Coulter, Miami, FL, USA) and data analysis was performed using WinList version 8.0 (Verity Software House, Topsham, ME, USA).
Allogeneic skin graft transplantation
Skin graft transplantation was performed following the protocol previously reported. Citation54,Citation55 Briefly, skin graft beds were prepared on the right lateral side of the thorax in the vicinity of the right axillary/brachial draining lymph nodes region of B6 recipient mice under ketamine-xylazine anesthesia. bm1 tail skin grafts were placed with four stitches and covered with terramycin ointment impregnated gauze, which was removed on day 8 post-transplantation (post-Tx). Signs of onset and progression of rejection, such as dryness, loss of hair, contraction, scaling, and necrosis were recorded by visual inspection for a period of time of 40 days, every day in the first 20 days and then every other day from day 20 to day 40 after transplantation. Skin grafts were considered fully rejected when complete necrosis of the skin graft was observed.
Host anti-donor specific antibody response
For the measurement of B6 host anti-donor KbmCitation1 humoral immune response, serum samples were collected from the different experimental groups at day 13 and 21 after bm1 skin grafting, then diluted at (1:10) and incubated for 1 hour with 0.5 × 10Citation6 donor type thymocytes. The extent of the host anti-donor isotype-specific humoral immune response was determined by evaluating the mean fluorescence intensity values of the staining. As positive control, Balb/c skin grafts were placed on B6 recipients and the presence of host anti-donor antibodies was measured at day 13 after transplantation.
Gm-csf-mediated bone marrow-derived dendritic cell differentiation
Syngeneic C57BL/6 (B6) and allogeneic bm1 bone marrow cells were harvested from tibiae and differentiated with 30 ng/ml murine GM-CSF (PeproTech), as previously described.Citation56,Citation57 Bone marrow-derived DC (BM-DC) were finally matured upon overnight exposure to 1 µg/ml LPS O111:B4 (Sigma-Aldrich).
Cd107a degranulation assay
1.5 × 10Citation5 of draining and non-draining (axillar and brachial) pLNs were isolated around day of rejection from B6 syngeneic or allogeneic bm1 skin grafted mice treated either with isotype control or anti-NK1.1 antibody and then were re-stimulated in vitro with 0.5 × 10Citation5 of either syngeneic (B6) or allogeneic bm1 mature BM-DC. PE-labelled isotype-matched control (clone Rtk-275, Biolegend) or PE-conjugated anti-CD107a mAb (clone 1D4B, Biolegend) were added to the cell culture and incubated for 1 hour at 37 ºC in 5% CO2 atmosphere. Stimulator and responder cells were incubated for four additional hours in the presence of Golgi plug 2 μM monensin and CD107a degranulation assay was used as a surrogate assay to monitor cytolytic function on donor CD8+ T cells. Citation30 For the gating strategy, a dump channel was set to include the following markers (CD19, CD11b and CD11c).
In vivo cytotoxic t lymphocyte (CTL) assay
Single-cell suspensions were prepared from spleens of bm1 female mice in RPMI 1640 complete medium as target cells for the in vivo CTL assay. Target cells were washed twice in D-PBS and labeled with CFSE (Molecular Probes) at 1 μM for 10 min at 37°C. Citation58 The reaction was stopped by adding two volumes of cold RPMI 1640 containing 10% FCS followed by two washes in D-PBS. 15 × 10Citation6 of CFSE-labeled bm1 target cells were i.v. injected to each group of mice at day 13 and day 21 post-skin graft transplantation. 24 hours later, recipient mice were euthanized and the specific killing of bm1 target cells was calculated as the absolute number of CFSE-labeled bm1 splenocytes remaining 24 hours after cell transfer in host spleen and peripheral lymph nodes (pLNs).Citation59
Histology
Allogeneic and syngeneic skin grafts samples from representative mice of each experimental group were fixed in 10% neutral-buffered formalin and conventionally processed. Fixed samples were dehydrated through a graded alcohol series before being embedded in paraffin wax following conventional processing for histological examination. Microtome Sections (4 µm thick) were cut, mounted on glass microscope slides and stained with hematoxylin and eosin. Finally, the slides were dehydrated and mounted for histological examination.
Statistical analysis
Collected data was organized in excel worksheets and Prism data tables. Results are expressed as mean ± SD or mean ± SEM. For the statistical analysis of normally distributed data, statistical significance between non-draining versus draining pLNs was calculated using two-tailed Student t test. For the comparison of more than 2 groups, one way ANOVA statistical test was applied. Adjustments for multiple comparisons were performed with the Tukey-Kramer comparison test. These statistical studies were performed under the conditions of independence of the data, normality test (Kolmorogov test) and equal variances among groups (Bartlett´s test). Parametric or non-parametric tests were applied accordingly.
Skin graft survival was calculated by using the Kaplan–Meier life table method and statistical analysis for the comparison of the survival curves was performed by the log rank (Mantel-Cox) test. The statistical analysis was performed using GraphPad Prism 6.0 software (Graphpad Software, Inc., La Jolla, CA). A value of p < 0.05 was considered statistically significant.
Sources of financial support
This work has been supported by grant FIS PI# 1300029 (Fondo de Investigaciones Sanitarias, Ministry of Health, Spanish Government and co-funded by European Union ERDF/ESF, “Investing in your future”), LE093U13 and Unit of Excellence Research UIC #012 (Department of Education of the Regional Government, Junta de Castilla y Leon) and Gerencia Regional de Salud (BIO/01/15) to JIRB, and by Miguel Servet National Grant (Health National Organization Research) CP12/03063, CPII17/00002 and FIS PI16/00002 (Instituto de Salud Carlos III and co-funded by European Union ERDF/ESF, “Investing in your future”), and Gerencia Regional de Salud GRS963/A/2014, GRS1142/A/2015 and GRS 1505/A/2017 to M.L.R.G.
This work has been partially funded by the National Network CIBER-ONC (oncology research) CB16/12/00480. P.S. is funded by grant 31003A-17-6256 of the Swiss National Science Foundation. We thank University of Leon for providing funding to cover publication expenses.
Abbreviations
CD | = | Cluster of differentiation |
NK | = | Natural killer |
TCR | = | T cell receptor |
MHC | = | Major histocompatibility complex |
Th | = | T helper |
pLNs | = | peripheral lymph nodes |
post-Tx | = | post-transplantation |
ILC | = | Innate lymphoid cells |
BMCs | = | Bone marrow cells |
Tfh | = | T follicular helper cells |
ILC | = | Innate lymphoid cells |
APC | = | Antigen-presenting cells |
Ig | = | Immunoglobulin |
SFM | = | Serum free medium |
mAb | = | Monoclonal antibody |
PI | = | Propidium Iodide |
CFSE | = | Carboxyfluorescein succinimidyl ester |
FCS | = | Fetal calf serum |
H/E | = | Hematoxylin/Eosin |
SD | = | Standard deviation |
SEM | = | Standard error of the mean. |
CAV | = | Cardiac allograft vasculopathy |
CTLA-4 | = | Cytotoxic T-Lymphocyte Antigen 4 |
Supplemental Material
Download TIFF Image (6.2 MB)Disclosure statement
No potential conflict of interest was reported by the authors.
Supplementary Material
Supplementary materials can be accessed here
References
- Lanier LL. 2005. Nk cell recognition. AnnuRevImmunol. 23:225–274.
- Manilay JO, Sykes M. 1998. Natural killer cells and their role in graft rejection. Curr Opin Immunol. 10(5):532–538.
- Herberman RB, Nunn ME, Holden HT, Lavrin DH. 1975. Natural cytotoxic reactivity of mouse lymphoid cells against syngeneic and allogeneic tumors. li. Characterization of effector cells. IntJCancer. 16(2):230–239.
- Kiessling R, Petranyi G, Klein G, Wigzel H. 1975. Genetic variation of in vitro cytolytic activity and in vivo rejection potential of non-immunized semi-syngeneic mice against a mouse lymphoma line. IntJCancer. 15(6):933–940.
- Ljunggren HG, Karre K. 1990. In search of the ‘missing self’: mhc molecules and nk cell recognition. ImmunolToday. 11(7):237–244.
- Velardi A, Ruggeri L, Mancusi A, Aversa F, Christiansen FT. 2009. Natural killer cell allorecognition of missing self in allogeneic hematopoietic transplantation: A tool for immunotherapy of leukemia. CurrOpinImmunol. 21(5):525–530.
- Yokoyama WM, Daniels BF, Seaman WE, Hunziker R, Margulies DH, Smith HR. 1995. A family of murine nk cell receptors specific for target cell mhc class i molecules. Semin Immunol. 7(2):89–101. doi:10.1006/smim.1995.0013.
- Snell GD, Jackson RB. 1958. Histocompatibility genes of the mouse. Ii. Production and analysis of isogenic resistant lines. J Natl Cancer Inst. 21(5):843–877.
- Cudkowicz G, Bennett M. 1971. Peculiar immunobiology of bone marrow allografts. Ii. Rejection of parental grafts by resistant f 1 hybrid mice. JExpMed. 134(6):1513–1528. doi:10.1084/jem.134.6.1513.
- Suzue K, Reinherz EL, Koyasu S. 2001. Critical role of nk but not nkt cells in acute rejection of parental bone marrow cells in f1 hybrid mice. EurJImmunol. 31(11):3147–3152.
- Kumar V, George T, Yu YY, Liu J, Bennett M. 1997. Role of murine nk cells and their receptors in hybrid resistance. CurrOpinImmunol. 9(1):52–56.
- Cook KD, Whitmire JK. 2013. The depletion of nk cells prevents t cell exhaustion to efficiently control disseminating virus infection. Journal Immunology (Baltimore, Md: 1950). 190(2):641–649. doi:10.4049/jimmunol.1202448.
- Soderquest K, Walzer T, Zafirova B, Klavinskis LS, Polic B, Vivier E, Lord GM, Martin-Fontecha A. 2011. Cutting edge: cd8+ t cell priming in the absence of nk cells leads to enhanced memory responses. Journal Immunology (Baltimore, Md: 1950). 186(6):3304–3308. doi:10.4049/jimmunol.1004122.
- Rydyznski C, Ka D, Ep K, Tr B, Se M, Mt M, Li C, Sutiwisesak R, Rm W, Sn W. 2015. Generation of cellular immune memory and b-cell immunity is impaired by natural killer cells. Nat Commun. 6:6375. doi:10.1038/ncomms7375.
- Cook KD, Kline HC, Whitmire JK. 2015. Nk cells inhibit humoral immunity by reducing the abundance of cd4+ t follicular helper cells during a chronic virus infection. J Leukoc Biol. 98(2):153–162. doi:10.1189/jlb.4HI1214-594R.
- George T, Yu YY, Liu J, Davenport C, Lemieux S, Stoneman E, Mathew PA, Kumar V, Bennett M. 1997. Allorecognition by murine natural killer cells: lysis of t-lymphoblasts and rejection of bone-marrow grafts. ImmunolRev. 155:29–40.
- Van Der Touw W, Bromberg JS. 2010. Natural killer cells and the immune response in solid organ transplantation. AmJTransplant. 10(6):1354–1358.
- Uehara S, Chase CM, Kitchens WH, Rose HS, Colvin RB, Russell PS, Madsen JC. 2005. Nk cells can trigger allograft vasculopathy: the role of hybrid resistance in solid organ allografts. JImmunol. 175(5):3424–3430. doi:10.4049/jimmunol.175.5.3424.
- Yu G, Xu X, Vu MD, Kilpatrick ED, Li XC. 2006. Nk cells promote transplant tolerance by killing donor antigen-presenting cells. JExpMed. 203(8):1851–1858. doi:10.1084/jem.20060603.
- Laffont S, Seillet C, Ortaldo J, Coudert JD, Guery JC. 2008. Natural killer cells recruited into lymph nodes inhibit alloreactive t-cell activation through perforin-mediated killing of donor allogeneic dendritic cells. Blood. 112(3):661–671. doi:10.1182/blood-2007-10-120089.
- Zecher D, Li Q, Oberbarnscheidt MH, Demetris AJ, Shlomchik WD, Rothstein DM, Lakkis FG. 2010. Nk cells delay allograft rejection in lymphopenic hosts by downregulating the homeostatic proliferation of cd8+ t cells. Journal Immunology (Baltimore, Md: 1950). 184(12):6649–6657. doi:10.4049/jimmunol.0903729.
- Lantow M, Eggenhofer E, Sabet-Baktach M, Renner P, Rovira J, Koehl GE, Schlitt HJ, Geissler EK, Kroemer A. 2015. Cd27low natural killer cells prolong allograft survival in mice by controlling alloreactive cd8+ t cells in a t-bet-dependent manner. Transplantation. 99(2):391–399. doi:10.1097/TP.0000000000000585.
- Auchincloss H Jr., Mayer T, Ghobrial R, Winn HJ. 1989. T-cell subsets, bm mutants, and the mechanisms of allogeneic skin graft rejection. ImmunolRes. 8(2):149–164.
- Rosenberg AS, Munitz TI, Maniero TG, Singer A. 1991. Cellular basis of skin allograft rejection across a class i major histocompatibility barrier in mice depleted of cd8+ t cells in vivo. JExpMed. 173(6):1463–1471. doi:10.1084/jem.173.6.1463.
- Le Moine A, Goldman M, Abramowicz D. 2002. Multiple pathways to allograft rejection. Transplantation. 73(9):1373–1381.
- Schulze DH, Pease LR, Geier SS, Reyes AA, Sarmiento LA, Wallace RB, Nathenson SG. 1983. Comparison of the cloned h-2kbm1 variant gene with the h-2kb gene shows a cluster of seven nucleotide differences. Proc Natl Acad Sci U S A. 80(7):2007–2011.
- Waldmann H. 2001. Therapeutic approaches for transplantation. CurrOpinImmunol. 13(5):606–610.
- Page EK, Dar WA, Knechtle SJ. 2012. Biologics in organ transplantation. TransplInt. 25(7):707–719. doi:10.1111/j.1432-2277.2012.01456.x.
- Ghobrial RR, Boublik M, Winn HJ, Auchincloss H Jr.. 1989. In vivo use of monoclonal antibodies against murine t cell antigens. Clin Immunol Immunopathol. 52(3):486–506.
- Betts MR, Brenchley JM, Price DA, De Rosa SC, Douek DC, Roederer M, Koup RA. 2003. Sensitive and viable identification of antigen-specific cd8+ t cells by a flow cytometric assay for degranulation. JImmunolMethods. 281(1–2):65–78.
- Ossevoort MA, De Bruijn ML, Van Veen KJ, Kast WM, Melief CJ. 1996. Peptide specificity of alloreactive cd4 positive t lymphocytes directed against a major histocompatibility complex class i disparity. Transplantation. 62(10):1485–1491.
- Oldstone MB. 2002. Biology and pathogenesis of lymphocytic choriomeningitis virus infection. Curr Top Microbiol Immunol. 263:83–117.
- Pallmer K, Oxenius A. 2016. Recognition and regulation of t cells by nk cells. Front Immunol. 7:251. doi:10.3389/fimmu.2016.00251.
- Andrews DM, Estcourt MJ, Andoniou CE, Wikstrom ME, Khong A, Voigt V, Fleming P, Tabarias H, Hill GR, Rg VDM, et al.. 2010. Innate immunity defines the capacity of antiviral t cells to limit persistent infection. J Exp Med. 207(6):1333–1343. doi:10.1084/jem.20091193.
- Waggoner SN, Cornberg M, Selin LK, Welsh RM. 2011. Natural killer cells act as rheostats modulating antiviral t cells. Nature. 481(7381):394–398. doi:10.1038/nature10624.
- Freitas AA, Rocha B. 2000. Population biology of lymphocytes: the flight for survival. AnnuRevImmunol. 18:83–111.
- Rocha B, Dautigny N, Pereira P. 1989. Peripheral t lymphocytes: expansion potential and homeostatic regulation of pool sizes and cd4/cd8 ratios in vivo. Eur J Immunol. 19(5):905–911. doi:10.1002/eji.1830190518.
- Gill RG. 2010. Nk cells: elusive participants in transplantation immunity and tolerance. Curr Opin Immunol. 22(5):649–654. doi:10.1016/j.coi.2010.09.005.
- Li XC, Demirci G, Ferrari-Lacraz S, Groves C, Coyle A, Malek TR, Strom TB. 2001. Il-15 and il-2: A matter of life and death for t cells in vivo. NatMed. 7(1):114–118.
- Sun JC, Lanier LL. 2011. Nk cell development, homeostasis and function: parallels with cd8(+) t cells. Nat Reviews Immunol. 11(10):645–657. doi:10.1038/nri3044.
- Fehniger TA, Cooper MA, Caligiuri MA. 2002. Interleukin-2 and interleukin-15: immunotherapy for cancer. Cytokine Growth Factor Rev. 13(2):169–183. doi:10.1016/S1359-6101(01)00021-1.
- Rabinovich BA, Li J, Shannon J, Hurren R, Chalupny J, Cosman D, Miller RG. 2003. Activated, but not resting, t cells can be recognized and killed by syngeneic nk cells. Journal Immunology (Baltimore, Md: 1950). 170(7):3572–3576.
- Lu L, Ikizawa K, Hu D, Werneck MB, Wucherpfennig KW, Cantor H. 2007. Regulation of activated cd4+ t cells by nk cells via the qa-1-nkg2a inhibitory pathway. Immunity. 26(5):593–604. doi:10.1016/j.immuni.2007.03.017.
- Sn W, Rt T, Pa M, Kumar V, Rm W. 2010. Absence of mouse 2b4 promotes nk cell-mediated killing of activated cd8+ t cells, leading to prolonged viral persistence and altered pathogenesis. J Clin Invest. 120(6):1925–1938. doi:10.1172/JCI41264.
- Crouse J, Bedenikovic G, Wiesel M, Ibberson M, Xenarios I, Von Laer D, Kalinke U, Vivier E, Jonjic S, Oxenius A. 2014. Type i interferons protect t cells against nk cell attack mediated by the activating receptor ncr1. Immunity. 40(6):961–973. doi:10.1016/j.immuni.2014.05.003.
- Goldrath AW, Bogatzki LY, Bevan MJ. 2000. Naive t cells transiently acquire a memory-like phenotype during homeostasis-driven proliferation. JExpMed. 192(4):557–564. doi:10.1084/jem.192.4.557.
- Boyman O, Purton JF, Surh CD, Sprent J. 2007. Cytokines and t-cell homeostasis. CurrOpinImmunol. 19(3):320–326.
- Yuan D, Bibi R, Dang T. 2004. The role of adjuvant on the regulatory effects of nk cells on b cell responses as revealed by a new model of nk cell deficiency. Int Immunol. 16(5):707–716. doi:10.1093/intimm/dxh071.
- Jukes JP, Wood KJ, Jones ND. 2007. Natural killer t cells: A bridge to tolerance or a pathway to rejection? Transplantation. 84(6):679–681. doi:10.1097/01.tp.0000280551.78156.ac.
- Stober D, Jomantaite I, Schirmbeck R, Reimann J. 2003. Nkt cells provide help for dendritic cell-dependent priming of mhc class i-restricted cd8+ t cells in vivo. Journal Immunology (Baltimore, Md: 1950). 170(5):2540–2548.
- Wu Z, Bensinger SJ, Zhang J, Chen C, Yuan X, Huang X, Markmann JF, Kassaee A, Rosengard BR, Hancock WW, et al. 2004. Homeostatic proliferation is a barrier to transplantation tolerance. NatMed. 10(1):87–92.
- Walzer T, Blery M, Chaix J, Fuseri N, Chasson L, Robbins SH, Jaeger S, Andre P, Gauthier L, Daniel L, et al. 2007. Identification, activation, and selective in vivo ablation of mouse nk cells via nkp46. Proc Natl Acad Sci U S A. 104(9):3384–3389. doi:10.1073/pnas.0609692104.
- Unkeless JC. 1979. Characterization of a monoclonal antibody directed against mouse macrophage and lymphocyte fc receptors. JExpMed. 150(3):580–596. doi:10.1084/jem.150.3.580.
- Rodriguez-Barbosa JI, Fernandez-Renedo C, Moral AMB, Buhler L, Del Rio ML. 2017. T follicular helper expansion and humoral-mediated rejection are independent of the hvem/btla pathway. Cell Mol Immunol. 14(6):497–510. doi:10.1038/cmi.2015.101.
- Del Rio ML, Cote-Sierra J, Rodriguez-Barbosa JI. 2011. Flt3l-mobilized dendritic cells bearing h2-kbm1 apoptotic cells do not induce cross-tolerance to cd8+ t cells across a class i mhc mismatched barrier. Transpl International: Official Journal Eur Soc Transplant. 24(5):501–513. doi:10.1111/j.1432-2277.2011.01220.x.
- Lutz MB, Kukutsch N, Ogilvie AL, Rossner S, Koch F, Romani N, Schuler G. 1999. An advanced culture method for generating large quantities of highly pure dendritic cells from mouse bone marrow. JImmunolMethods. 223(1):77–92.
- Del Rio ML, Jones ND, Buhler L, Norris P, Shintani Y, Ware CF, Rodriguez-Barbosa JI. 2012. Selective blockade of herpesvirus entry mediator-b and t lymphocyte attenuator pathway ameliorates acute graft-versus-host reaction. JImmunol. 188(10):4885–4896. doi:10.4049/jimmunol.1103698.
- Lyons AB. 2000. Analysing cell division in vivo and in vitro using flow cytometric measurement of cfse dye dilution. JImmunolMethods. 243(1–2):147–154.
- Del Rio ML, Kurtz J, Perez-Martinez C, Ghosh A, Perez-Simon JA, Rodriguez-Barbosa JI. 2011. B- and t-lymphocyte attenuator targeting protects against the acute phase of graft versus host reaction by inhibiting donor anti-host cytotoxicity. Transplantation. 92(10):1085–1093. doi:10.1097/TP.0b013e3182339d4a.