Abstract
Ostrich meat has received considerable attention in recent years due to its low fat, low sodium and collagen, and high polyunsaturated fatty acids and iron. It is recommended as an alternative to other types of red meat. In this study, functional characteristics of ostrich meat (M. iliofibularis) and beef (M. longissimus dorsi) during storage at 4°C for different lengths of time post-mortem were determined and compared. Significant decrease in Warner-Bratzler shear force in both meat types occurred during storage. Lightness (L*) of the two muscles decreased while redness (a*) and yellowness (b*) increased. The pH significantly decreased whereas buffering, emulsion, foaming and water holding capacities and emulsion stability, protein solubility increased during seven days postmortem. Aging had no effect on cooking loss. In conclusion, the results of this study showed that ostrich meat has superior functional properties in comparison to beef and these properties are more preserved than beef during aging.
La carne de avestruz ha recibido una importante atención en años recientes debido a su bajo contenido en grasa, en sodio, colágeno, alto contenido en ácidos grasos poliinsaturados y hierro. Se recomienda como alternativa a otros tipos de carne roja. En este estudio se determinaron y compararon características funcionales de carne de avestruz (M. iliofibularis) y buey (M. longissimus dorsi) en almacenamiento a 4°C durante diferentes períodos de tiempo post-mortem. Una reducción significativa en fuerza de corte de Warner-Bratzler en ambos tipos de carne tuvo lugar durante el almacenamiento. La claridad (L*) de los dos músculos se redujo, mientras la rojez (a*) y el amarilleado (b*) incrementaron. El pH se redujo significativamente mientras que las capacidades reguladoras, de emulsión, espumado y retención de agua, estabilidad de emulsión y solubilidad de proteína aumentaron durante los siete días post-mortem. La maduración no tuvo efecto en la pérdida durante cocción. En conclusión, los resultados de este estudio mostraron que la carne de avestruz tiene propiedades funcionales superiores en comparación con la de buey y esas propiedades se conservan mejor que las de carne de buey durante el envejecido.
Introduction
Ostrich meat (Struthio camelus) is perceived and marketed as a healthy alternative to other types of red meats (Fisher, Hoffman, & Mellett, Citation2000). Ostrich meat products are being marketed in food market as steaks, sausage links, summer sausage, fillets, hamburger, pastrami and jerky (Jeffery, Citation1999). Several researchers have studied the nutritional value of ostrich meat indicating that it contains low collagen, saturated fatty acid and sodium, whereas its polyunsaturated fatty acid along with iron content is high (Balog & Almeida Paz, Citation2007; Hoffman, Citation2005; Hoffman, Muller, Cloete, & Bran, 2008; Paleari, Corsico, & Beretta, Citation1995; Polawska, Marchewka, Krzyzewski, Bagnicka, & Wojcik, Citation2011a; Polawska et al., Citation2011b; Sales & Oliver-Lyons, Citation1996). The studies also indicated that ostrich meat is characterized by a higher pH ( > 6.2) and darker visual color due to more pigment content, whereas its tenderness and cooking loss is as of beef.
Functional properties of meat proteins are important in food processing and food product formulation. These include water holding/oil binding capacity, emulsification, foam capacity and gelation. These properties are affected by the intrinsic factors of proteins such as molecular structure, amino acid sequence (hydrophobicity profile) and size. Proteins with high oil and water binding capacities are desirable for use in meat products while proteins with high emulsifying and foaming capacity are good for sausages, bologna and soups (Ahmedna, Prinyawiwatkul, & Rao, Citation1999). To the best of our knowledge, there is no report on the effect of aging on physico-chemical, functional properties of protein, and the ultrastructural patterns of ostrich meat. Since beef is extensively used in meat industry, the present work sets out to identify and compare the properties of ostrich meat and compare it to beef during aging. The aim of this research was to evaluate several physico-chemical, functional, ultrastracture, patterns of ostrich meat during aging at 4°C in comparison to beef.
Materials and methods
Sample preparation
Three male ostriches, from the subspecies S. camelus australis (Blue Neck) were slaughtered at the age 10–12 months (ca. 70 kg live weight) at a local abattoir in Shiraz (Fars, Iran). They were fasted for 24 h and then were slaughtered after electrical stunning by the Halal method (cutting of neck for exsanguinations). Muscles were separated after removing feather and skin. The fan fillets (M. iliofibularis) were obtained from the carcasses. Muscle (M. Longissimus dorsi) was obtained from three carcasses of 1.5-year-old male Swiss brown cattle. The meat samples were then packaged and kept at 4°C and at 4.5, 24, 72 and 168 h postmortem. At the time of experiments, samples were brought to room temperature, and then visible fat and connective tissues were removed and used for subsequent analysis.
Physico-chemical analysis
Parameters including moisture, ash, protein and fat contents were tested in triplicate and determined according to the AOAC (2003) methods. Factor 6.25 was used for conversion of nitrogen to total protein. pH was measured with a pH meter (Consort P507, Belgium) on a suspension obtained by blending 20 g sample with 20 ml deionized water for 2 min at 20°C. Collagen content of the raw meat was calculated from the hydroxy-proline content according to the method of Egan, Kirk and Sawyer (Citation1981).
Determination of cooking loss
Cooking loss was determined by placing weighed samples of approximately 100 g, sealed in plastic bags, kept in water bath at 75°C for 50 min followed by cooling, dry blotting, and weighing (Honikel, Citation1998). Cooking loss was calculated as follows:
Evaluation of tenderness
Tenderness was determined using raw ostrich meat and beef. Triplicate samples of 1.25 cm diameter were randomly removed for determining Warner-Bratzler shear force values. The samples were cut parallel to the muscle fiber direction in order to measure the influence of the myofibril proteins by Instron machine (Instron 1140). Maximum shear force values (N/1.25 cm diameter) were recorded for each example (repeated three times). The crosshead speed was 200 mm/min. The mean value was calculated for each individual meat (Honikel, Citation1998).
Evaluation of color
The color of samples was evaluated using a modified method of Yam and Papadakis (Citation2004). High resolution pictures of the samples were taken separately by a digital camera. Resolution, contrast and lightness of all images were set to 3000 dpi, 62% and 62%, respectively. The pictures were saved in JPEG format and analyzed, quantitatively using the Adobe Photoshop 8 software and the color parameters of L* (lightness), a* (redness–greenness) and b* (blueness–yellowness) values were determined in the lab mode of the software.
Myofibril fragmentation index (MFI)
MFI is used for evaluation of the degree of the tenderness. MFI was determined using a slightly modified version of the procedure of Culler et al. (Citation1978). 4 g of minced muscle was homogenized for 30 s in 10 vol (v/w) of a 4°C isolating medium consisting of 100 mM KCl, 20 mM potassium phosphate, 1 mM EDTA, 1 mM MgCl2, and 1 mM sodium azide. The homogenate was centrifuged at 1000 g for 15 min and the supernatant was decanted. The sediment was resuspended in 10 vol (v/w) of isolating medium using a stirring rod, sedimented again at 1000 × g for 15 min (Gallenkamp, UK) and the supernatant was then removed. The sediment was resuspended in 2.5 vol (v/w) of isolating medium and passed through a polyethylene strainer (18 mesh) to remove connective tissue and debris. An additional 2.5 vol (v/w) was used to facilitate passage of myofibrils through the strainer. Protein concentrations of the suspensions of myofibrils were determined by the biuret method (Gornall, Bardawill, & David, Citation1949). Briefly, 0.25 ml of each suspension was placed into glass tubes and 0.75 ml MFI buffer was added, and finally 4 ml biuret reagent was added and vortexed. Glass tubes were placed in the dark at room temperature for 30 min. The absorbance was read at 540 nm using a Labomed UVD-3200 spectrophotometer (Culver City, CA). Bovine serum albumin (BSA) was used to establish a standard curve. An aliquot of the myofibril suspension was diluted in isolating medium to a protein concentration of 0.5 ± 0.05 mg/ml. The diluted myofibril suspension was measured immediately at 540 nm. Absorbance was multiplied by 200 to give a MFI.
Nitrogen solubility index
Nitrogen solubility index (NSI) was determined using a modification of the procedure of Inklaar and Fortin (Citation1969). Ten grams of minced meat samples was added to l0 ml 5% NaCl and homogenized for 1 min. This was followed by centrifugation at 12,000 × g for 10 min. The nitrogen content of the supernatant was determined by the standard micro-Kjeldahl procedure (AOAC, 2003). NSI was calculated as follows:
Emulsifying capacity
Emulsifying capacity (EC) was determined using a slightly modified version of the procedure of Turgut (Citation1984). Fifty grams of ground meat samples was added to 200 ml 1.0 M NaCl solution in a blender (Seward Medical, London, UK). The mixture was blended for 60 s at 7000 rpm. A 12.5 g portion of the cold (4°C) meat slurry, 37.5 ml of cold (4°C) 1.0 M NaCl solution and 50 ml of corn oil were combined using a deep glass beaker immersed in an ice bath. After blending for 30 s at 9700 rpm, additional corn oil was introduced at a rate of 1 ml/s. Temperature of the oil was maintained at 23°C. When the emulsion breaks, there is an increase in its resistance which is measured by a digital ohmmeter (DT-9205AL, Japan) and this stops further oil being added. The results were expressed in volume of emulsified oil for 100 g sample (%). The results were the averages of three replicates.
Emulsion stability
Emulsion stability (ES; %) was determined as described by Turgut (Citation1984). ES is the time required for a stable emulsion to break (Saffle, Christian, Carpenter, & Zirkle, Citation1967). An emulsion was prepared by the EC technique with the addition of 100 ml oil to obtain a stable emulsion. The emulsion was blended for 30 s after the addition of the last drop of oil. The mixture was transferred to a Gerber cheese centrifuge tube, applying low vacuum. For optimum phase separation the filled tubes were centrifuged (Gallenkamp, England) twice at 2000 rpm at 70°C for 15 min. The amount of separated water was measured and used for ES calculation according to the following equation:
Water holding capacity
Water holding capacity (WHC) was determined by the method of Jauregui and Regenstein (1981) with some modifications. Two grams of meat samples waswrapped with filter paper number one (2.5 × 2.5 cm) and transferred to 2 ml Eppendorf tube and centrifuged (Gallenkamp, England) at 14,000 rpm for 20 min at 4°C. The meat cake was carefully removed with forceps and weighed. The water contents of meat samples were determined by measuring the weight loss produced in samples of known weight before centrifuging. WHC values represent the percentage of water retained in the meat cake.
Buffering capacity
Buffering capacity (BC) was calculated for each increment of acid and base for titration as described by Hill, Irvine and Bullock (Citation1985). Samples were homogenized (Silverston, England), and then 10 g aliquots was weighed out and separately homogenized with l0 ml distilled water. The homogenates were titrated using 0.1 N HCL or 0.l N NaOH. The homogenates were stirred during titration. Titrations were carried out at room temperature. BC was calculated as follows:
Foaming capacity
Foaming capacity (FC) was determined in triplicate using the method described by Makri, Papalamprou and Doxastakis (Citation2005) with slight modification. FC was expressed as percent volume increase due to foam as compared to the initial volume. Twenty grams of ground meat was mixed with 500 ml deionized water in Mixture Blend (Homex, GTM-8305G, Germany) for 3 min using a high-speed (on speed 3), poured in to 2000 ml (V1) graduated cylinders, and the volume of foam (V2) was immediately recorded at 0 min (initial volume) and 10 min, 30 min and 60 min (final volume) by the following equation:
Determination of myofibril and sarcoplasmic proteins
Myofibril and sarcoplasmic proteins were isolated by the method of Rathgeber, Boles and Shandi (Citation1999). Two phosphate buffers were used for extracting sarcoplasmic and myofibril proteins: buffer A: 15.6 mM Na2HPO4, 3.5mM, KH2PO4 (ionic strength, μ = 0.05, pH = 7.5) and buffer B: 0.45 M KCl, 5.6 mM Na2HPO4, 3.5 mM KH2PO4 (μ = 0.5, pH = 7.5). All experiments were conducted at 4°C. One gram of minced meat was homogenized (Silverstone, England) with 20 ml buffer A (low ionic strength) for 10 s at 20,000 rpm. The homogenate was centrifuged (Gallenkamp, England) at 16,000 × g for 15 min. The precipitate was again extracted by the same procedure. These two supernatants were combined and used as the sarcoplasmic protein fraction. The residue from the above was homogenized with 20 ml buffer B (high Ionic strength, μ = 0.5) and centrifuged. The precipitate was homogenized and centrifuged again. Both supernatants were combined and used as myofibrils proteins fraction. Protein content of the supernatants was determined by biuret methods using BSA as standard (Gornall et al., Citation1949).
Transmission electron microscopy (TEM)
Muscle tissues were cut into 1-mm cubes and fixed in 2.5% glutaraldehyde (pH = 7.4) at 4°C (Forsmann, Citation1969). After repeated sodium cacodylate buffer washes, the tissues were postfixed for 2 h in 2% osmium tetroxide (pH = 7.3). The tissues were then dehydrated through series of ethyl alcohols and cleared in propylene oxide, embedded in Spurr resin and polymerized at 60°C for 24–48 h. Sections were cut using ultra microtome equipped with diamond knife. Semi thin sections (1 μm) were cut as above, stained with 1% toluidine blue using in light microscopy. Ultra thin sections (70 nm) were collected on uncoated copper grids and stained with uranyl acetate and then with lead citrate (Harris, Citation1991). They were examined with a Philips CM-10 electron microscope.
Statistical analysis
Triplicate collection data were used for the analysis. All data were statistically analyzed by SPSS/PC software (version 17). One-way analysis of variance (ANOVA), independent-sample, and paired Student t-tests were used for comparison of the means.
Results and discussion
Chemical analyses
shows the results of chemical analyses of the meat samples. Protein content of ostrich meat was 22.4%, close to the values reported by Berge, Lepetit, Renerre and Touraille (Citation1997) (21.0%), Majewska, Jakubowska, Ligocki and Tarasewicz (Citation2009) (20.8%) and Paleari et al. (Citation1995) (22.2%), and was slightly higher than that of beef. (21.73). Ostrich meat had four times less fat and significantly higher moisture than beef (p < 0.05). The lipid content of meat is of particular importance from the nutritional point of view as it carries out vitamins and is a source of energy. Therefore, ostrich meat might be considered to be superior to beef in this regard and is a more attractive meat to be included in the diet of people who are concerned about fat content in their diet. The content of collagen in ostrich meat found in this study (0.44%) was close to that reported by Sales and Oliver-Lyons (Citation1996). Generally, ostrich meat is characterized by a low connective tissue content associated with its low mean collagen content in comparison with beef (Sales & Oliver-Lyons, Citation1996). The collagen content of beef found in this study was 1.21%.
Table 1. Chemical composition of ostrich muscle (M. iliofibularis) compared with cattle (M. longissimus dorsi), (mean ± SD) on three runs.
Tabla 1. Composición química de músculo de avestruz (M. iliofibularis) comparado con buey (M. longissimus dorsi), media ± desviación estándar de tres réplicas.
In the present study, no significant differences were observed between ostrich meat and beef in myofibrillar and sarcoplasmic proteins contents (p > 0.05). According to Wilson, Bray and Phillips (Citation1954), calves store more collagen in their tissue than old cows. In general, young animals do not present problem because of the small quantities of collagen in tissues. As the animal ages, the meat gets tough because of the stabilization of collagen molecules (Shimokomaki, Lourenco Soares, & Iouko Ida, 2010).
pH
There was a significant decrease in pH in M. iliofibularis of ostrich meat during seven days of post-mortem (p < 0.05) while the pH of M. longissimus dorsi of beef did not significantly change during the same time period (). Still ostrich meat has significantly higher pH than beef at all storage temperatures.
Table 2. Physical parameters variation in ostrich meat (M. iliofibularis) and cattle meat (M. logissimus dorsi) during storage time at 4°C, (mean ± SD).
Tabla 2. Variación de parámetros físicos en carne de avestruz (M. iliofibularis) y buey (M. logissimus dorsi) durante tiempo de almacenamiento a 4°C (media ± desviación estándar).
Another major difference between the meats of two species in terms of pH was the ultimate pH; where ostrich meat showed a higher value than that of beef (5.85 vs. 5.48). Ostrich meat is often classified as an intermediate meat type because of its pH ranging between normal (pH > 5.8) and extremely dark, firm and dry (pH < 6.2), also known as DFD (Sales & Mellett, Citation1996). Ostrich meat is characterized by high ultimate pH, as measured 24 h post-mortem (Azad & Akter, Citation2005). The difference in pH values found in our study is also reported by other researchers (e.g. Bingol & Ergun, Citation2011; Fernandez-Lopez, Sayas-Barbera, Munoz, Sendra, Navarro, & Perez-Alvarez, Citation2008; Leygonie, Britz, & Hoffman, Citation2011a; Seydim, Acton, Hall, & Dawson, Citation2006) and is probably related to the difference in chemical composition between the meat of two species, including lipids, minerals and amino acids and specially in compounds such as creatine, which is relatively high in ostrich meat (Azad & Atker, 2005). Muscle glycogen undergoes glycolysis, producing lactic acid, which promotes rapid pH drop. Meat quality traits related to post-mortem muscle pH drop are color, moisture content, and shelf life. Difficulties in pre-slaughter handing cause animals to experience stress which results in glycogen depletion and a high postmortem pH of meat (Lawrie, Citation1998). This situation is generally true for ostrich before and during slaughtering. However, the rest of glycogen is regularly converted to ATP under anaerobic condition so that pH is decreased over the time although with a different rate than that of beef. The pH decline of meat is generally related to glycogen content of the muscle at slaughter time (Immonen & Puolanne, Citation2000), where lower glycogen content may result in a decreased rate of glycolysis; hence, a slower accumulation of lactic acid and a slower rate of post slaughter pH decline.
Cooking loss
During storage, cooking loss values slightly decreased from 35.45 at 4.5 h postmortem to 33.07 at 168 h in ostrich and from 34.68 to 33.21 in beef (p < 0.05). There was no significant difference between ostrich meat and beef at each of the four aging stages (). Cooking losses are negatively correlated with pH value (Purchas, Citation1990). Yanar and Yetim (Citation2001) reported that in both longissimus and semimembranosus muscles cooking loss decreased from 29.9% on the first day of aging to 26.8% after seven days. Davis, Huffman and Cordray (Citation1975) reported that aging in general reduced cooking losses. Therefore, pH will also influence the percentage of the cooking loss of meat. The lower percentage of cooking loss of ostrich is due to the higher pH of this meat.
Shear force
Analysis of shear force values for post-mortem aging () showed that the integrity of the tissues significantly decreased. Aging improved tenderness in both types of meats. Muscles held for seven days were more tender than those held for one day post-slaughter. As a general rule, increasing the aging time will increase tenderness (Devine & Graafhuis, Citation1995). Tenderization is primarily due to post-mortem proteolysis mediated by proteolytic enzymes such as calpains and lysosomal proteases (Koohmaraie, Citation1994). Improvement occurs as a consequence of the degradation of muscle structure that reduces myofibrillar strength (Ouali, Citation1990) and could facilitate the release of meat juice during chewing. Tenderness and pH showed similar trend over the time such that the increase in muscle pH resulted in increase in tenderness whereas cooking losses are negatively correlated with pH (Purchas, Citation1990; Thomas, Gondoza, Hoffman, Oosthuizen, & Naude, Citation2004). Meat was softened during aging () because Warner-Braztler shear force (WBSF) in beef tended to decrease in raw meat. In beef, WBSF was always significantly greater than that of ostrich (). This variability might be related to the differences in proteolytic systems between two species (Caballero et al., Citation2007).
Color
Color, another important parameter of meat, was found to be comparable in ostrich and beef (). There was a significant difference between a* value (redness) in ostrich meat and beef during storage (p < 0.05) and ostrich meat always showed a higher value. There is also a significant difference between lightness in ostrich and beef at 4.5, 72 and 168 h post-mortem (p < 0.05). However, b value was higher than that of beef. Perez-Alvarez, Fernandez-Lopez, Sayas-Barbera and Cartagena-Gracia (Citation1998) reported that the meat with higher content of connective tissue showed higher b values as it was evidenced in this study by higher collagen content of beef (). Mellet and Sales (Citation1997) have also reported a negative correlation between yellowness and tenderness. The darker color of a meat sample is usually related to higher ultimate pH; however, the higher value might be due to higher pigment content of ostrich meat. The difference between the color of ostrich meat and beef is probably related to the different protein profiles, including myoglobin content (Vallejo-cordoba, Rodríguez-ramírez, & González-córdova, Citation2010) According to Lombardi-boccia, Martinez Dominguez and Aguzzi (Citation2005), ostrich meat contains higher iron than beef (2.57 vs. 1.8 mg Fe/100g).
Myofibrillar fragmentation index
MFI significantly increased (p < 0.05) in ostrich meat and beef from one to seven days post-mortem at 4°C (). MFI is a useful indicator of the extent of proteolysis showing both rupture of the I-band and breakage of myofibril linkages (Taylor, Geesink, Thompson, Koohmaraie, & Gol, Citation1995). Data were in agreement with results reported by Soltanizadeh, Kadivar, Keramat, & Fazilati, Citation2008). One possible cause of this variability (between species) could be the difference in the enzyme content and more likely the enzyme/inhibitor ratio, a parameter reflecting the efficiency of the proteolytic process which varies among species (Caballero et al., Citation2007). Comparing the results obtained from shear force also indicated that by increase in MFI, shear force decreased.
NSI
Protein solubility increased during the storage time (p < 0.05) in two species (). There were significant differences between ostrich meat and beef at each of the four aging times (p < 0.05). NSI value in ostrich meat was higher than that of beef (p < 0.05). The increase in solubility of total proteins during storage could be due to the proteolytic activities resulting in shorter peptides and free amino acids (Azad & Akter, Citation2005; Montero, GO´ Mez-guille´n, & Borderias, Citation1996; Zhang, Farouk, Young, Weliczko, & Podmore, Citation2005). It is generally accepted that proteolysis of the myofibrillar structure is an important factor in protein solubility and meat tenderness and that 90% or more of the observed proteolytic activity during the first 7–10 days of postmortem storage at 2–4°C can be attributed to the calpains (Lee, Santé-Lhoutellier, Vigouroux, Briand, & Briand, Citation2008; Sazili, Parr, Sensky, Jones, Bardsley, & Buttery, Citation2005).
Table 3. Functional parameters variation in ostrich meat (M. iliofibularis) and beef (M. logissimus dorsi) during storage time at 4°C, (mean ± SD).
Tabla 3. Variación de parámetros funcionales en carne de avestruz (M. iliofibularis) y buey (M. logissimus dorsi) durante tiempo de almacenamiento a 4°C (media ± desviación estándar).
Calpains constitute a large family of intracellular Ca2+-dependent neutral cysteine proteinases that consists of at least three components: (1) the form of the proteinase that is fully active at micromolar concentration of calcium (μ-calpain, also called CDP-I or calpain-I), (2) the form of the proteinase that is fully active at millimolar concentration of calcium (m-calpain, also called CDP-II or calpain-II), and (3) calpastatin, which inhibits the activity of both μ and m-calpain and their respective calcium requirement (Ilian, Bekhit, & Bickerstaffe, Citation2004; Lee, Stevenson-Barry, Kauffman, & Kim, Citation2000).
The calpain system is highly sensitive to fluctuating levels of calcium ion, pH and temperature (Hwang, Devine, & Hopkins, Citation2003). A calcium-activated neutral proteinases (CANPs) and their endogenous specific inhibitor calpastatin were found in a wide variety of vertebrate and invertebrate tissues (Mkwetshana, Naudé, & Muramoto, Citation2002). Therefore, physiological condition of carcass immediately following slaughter and the storage conditions are expected to have profound effect on protein solubility and tenderness of the meat. Meats from different muscles of the same species and meat from different species exhibit significant variation in tenderness which appears to be the results of variation in the calpains activity (Dransfield, Citation1997; Gill, Hortós, & Sárraga, Citation1998; Koohmaraie, Whipple, Kretchmar, Crouse, & Mersmann, Citation1991; Shackelford, Koohmaraie, Miller, Crouse, & Reagan, Citation1991). It has been reported that ageing time has significant effects on calpain cathepsin activities and protein extractability (Gill et al., Citation1998). The μ-calpain and calpastatin has been purified and characterized from ostrich brain. The N-terminal sequence of μ-calpain showed 24% and 18% sequence identity with human and bovine μ-calpain, respectively. (Mkwetshana et al., Citation2002). The increase in solubility of proteins of ostrich meat observed in this study could be the result of activation of the putative muscle calpain system in this species which could be expected to be more active in the respective calpains in cattle, hence a higher NSI.
Emulsion capacity and emulsion stability
Emulsion capacity (EC) values of ostrich meat were significantly higher than those of beef (p < 0.05) during the seven-day post-mortem. At each of the four ageing times (p < 0.05), however, no significant differences were evidenced in each of the muscles (M. iliofibularis of ostrich and M. longissimus dorsi of beef) over aging time (p > 0.05) (). Usually two factors affect the emulsifying capacity of muscle; the amount of soluble protein available which is under the influence of pH, and the efficiency of the protein to emulsify fat (Saffle et al. Citation1967) that is mostly affected by the protein structures. In ostrich meat, the pH was shown to be significantly higher than that of the beef; therefore, it has a higher solubility and NSI. According to Sosulski and Fleming (Citation1977), emulsifying activity of proteins depends upon the hydrophilic–lipophilic balance of a matter that is highly controlled by pH.
Water holding capacity
Water holding capacity (WHC) increased significantly during the aging period in both meats (p < 0.05) as shown in . Although there was a significant difference between the meats so much so that WHC of ostrich was significantly higher than that of beef (p < 0.05), that could be due to higher ultimate pH, (6.0) in ostrich meat. Purchas (Citation1990) indicated that the greater the pH, the greater of the WHC.
Buffering capacity
There was a significant increase in buffering capacity (BC) of both ostrich meat and beef. During aging (p < 0.05), the amount of BC in ostrich was higher compared to that of beef (). Acidic buffering was 5.39 at 4.5 h postmortem and with upward trend to 6.68 in 168 h postmortem. There was an upward trend in BC of both meats during all the time. The compounds affecting the BC in the pH range 5.5–7.0 are the following: (1) phosphates having pk a values of 6.1–7.1; (2) histidyl imidazole residues of myofibrillar protein; and (3) the dipeptides carnosine and anserine (Puolanne & Kivikari, Citation2000). The principal difference in the BC of different types of muscles is due to the fact that white fibers have a higher content of histidine compounds when compared with red ones (Olsman & Slump, 1981).
Foaming capacity
As can be seen in , there was as remarkable increase in FS of both ostrich meat and beef after 10 min (p < 0.05). FC values in ostrich meat was higher than that of beef (p < 0.05). There were significant differences between the two types of meat after 10, 30 and 60 min in each of the four times of aging (p < 0.05), but after 30 min the foaming of the both kinds of meat did not increase significantly (p > 0.05).
Transmission electron microscopy
Qualitative changes in structure of M. iliofibularis and M. longissimus dorsi muscles of ostrich and beef which were investigated with TEM are shown in and . Two hours after slaughter, sarcomeres were characterized by regular structure of A and I-bands, M-lines and Z-disks. The myofibril lattice was more relaxed in muscles from beef than that of ostrich. Over the time, the space between myofibrils and sarcolemma and also between single myofibrils in muscle fibres increased during storage. On the other hand, structure of sarcomere underwent continuous degradation, and length of sarcomere increased due to enlargement of I-bands accompanied by Z-disk degradation. After 24 h, the partial disappearance of M-line and the degradation of Z-discs manifested by their disappearance in fragments of myofibrils were observed. During cold storage, the length of H-band increased more in the beef than the ostrich muscle (3.39 and 1.18%, respectively). An increase in meat tenderness occurs during cold storage and after rigor mortis, mostly due to degradation of inner- and extra-cellular structure of muscle fibres. According to Dransfield (Citation1997) and Taylor et al. (Citation1995), the first symptoms of changes in muscle fiber structure during aging can be shown after separation of sarcolemma from adjacent myofibrils. On the other hand, the increase in the I-band length of sarcomeres can be caused by displacement of actin from the actomyosin complex by p-tropomyosin accompanied by the weakening of the contractile proteins links (Takahashi, Hattori, & Kuroyanagi, Citation1995).
Figure 1. Transmission electron micrographs of beef (M. longissimus dorsi) and ostrich muscle (M. iliofibularis). Notes: (A) beef, 2 h after slaughter; (B) beef, 24 h after slaughter; (C) Ostrich, 2 h after slaughter; and (D) Ostrich, 24 h after slaughter.
Figura 1. Micrografía de transmisión de electrones de músculo de buey (M. longissimus dorsi) y de avestruz (M. iliofibularis). Notas: (A): buey, dos horas tras la matanza, (B): buey, 24 horas tras la matanza, (C): avestruz, dos horas tras la matanza, (D): avestruz, 24 horas tras la matanza.
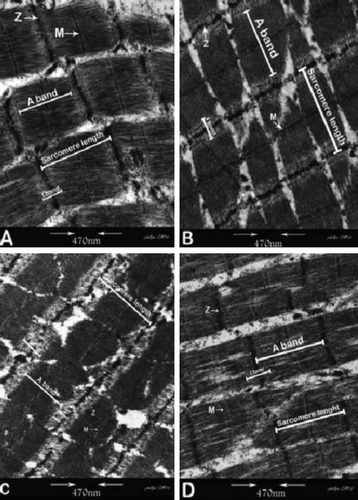
Table 4. Changes of sarcomere, A- and I-band M lines and Z-disks length of myofibrils from M. iliofibularis and M. longissimus dorsi muscles of ostrich meat and beef stored at 4°C during 2 and 24 h after slaughter, (mean ± SD).
Tabla 4. Cambios en longitud de sarcómero, banda A, banda I, línea M y discos Z de miofibrillas de músculo de M. iliofibularis y M. longissimus dorsi almacenados a 4°C durante dos y 24 horas tras la matanza (media ± desviación estándar).
Conclusion
It was found that physical and functional properties (WHC, NSI, FC, BC, EC, ES), of both types of meat were improved upon aging most probably due to increase in protein solubility at post-mortem. Ostrich meat has superior functional attributes in comparison to beef and might be a good source for production of meat like sausages. Functional properties and tenderness improved by aging for seven days at 4°C in muscles from ostrich and beef. 24 h after slaughter change in muscle fiber structure occurred. Taken together, the higher nutritional values and significantly better functional properties of ostrich meat as found in this study, compared to beef, make ostrich meat an attractive substitute for beef in meat industry.
Acknowledgment
This research was financially supported by grant numbers 89-GR-AGR-29 and 89-GR-VT-11 from Shiraz University Research Council. We also appreciate Dr Khaksar for his help in taking TEM.
References
- Ahmedna , M. , Prinyawiwatkul , W. and Rao , R.M. 1999 . Solubilized wheat protein isolate: Functional properties and potential food applications . Journal of Agricultural and Food Chemistry , 47 : 1340 – 1345 .
- AOAC International . 2003 . Official methods of analysis of AOAC international , 17th ed , Gaithersburg : AOAC International .
- Azad , M.A.K and Akter , S. 2005 . Influence of freezing time on quality of beef . Journal of Animal and Veterinary Advances , 4 : 424 – 426 .
- Balog , A. and Almeida Paz , I.C.L. 2007 . Ostrich (Struthio camelus) carcass yield and meat quality parameters, Brazil . Journal of Poultry Science , 9 : 215 – 220 .
- Berge , P. , Lepetit , J. , Renerre , M. and Touraille , C. 1997 . Meat quality traits in the emu (Dromaius novohollandaie) as affected by muscle type and animal age . Meat Science , 45 ( 209 )
- Bingol , E.B. and Ergun , O. 2011 . Effects of modified atmosphere packaging (MAP) on the microbiological quality and shelf life of ostrich meat . Meat Science , 88 : 774 – 785 .
- Caballero , B. , Sierra , V. , Oliván , M. , Vega-Naredo , I. , Tomás-Zapico , C. , Alvarez-García , O. and Coto-Montes , A. 2007 . Activity of cathepsins during beef aging related to mutations in the myostatin gene . Journal of Science of Food and Agriculture , 87 : 192 – 199 .
- Culler , R.D. , Parrish , F.C. , Smith , G.C. and Cross , H.R. 1978 . Relationship of myofibril fragmentation index to certain chemical, physical and sensory characteristics of bovine longissimus muscle . Journal of Food Science , 43 : 1177 – 1180 .
- Davis , C.A. , Huffman , D.L. and Cordray , J.A. 1975 . Effect of mechanical tenderization, aging and pressing on beef quality . Journal of Food Science , 40 : 1222 – 1224 .
- Devine , C.E. and Graafhuis , A.E. 1995 . The basal toughness of unaged lamb . Meat Science , 39 : 285 – 291 .
- Dransfield , E. When the glue comes unstuck . Paper presented at Proceedings of 43rd International Congress of Meat Science and Technology, Technol . pp. 52 – 63 . Auckland , , New Zealand
- Egan , H. , Kirk , R.D. and Sawyer , R. 1981 . Pearson's chemical analysis of foods , 8th ed , New York : Churchill Livingstone .
- Fernandez-Lopez , J. , Sayas-Barbera , E. , Munoz , T. , Sendra , E. , Navarro , C. and Perez-Alvarez , J.A. 2008 . Effect of packaging conditions on shelf-life of ostrich steaks . Meat Science , 78 : 143 – 152 .
- Fisher , P. , Hoffman , L.C. and Mellett , F.D. 2000 . Processing and nutritional characteristics of value added ostrich produce . Meat Science , 55 : 251 – 254 .
- Forsmann , W.G. 1969 . A method for in vivo diffusion tracer studies combining perfusion fixation with intravenous tracer injection . Histochemia , 20 : 277 – 286 .
- Gill , M. , Hortós , M. and Sárraga , C. 1998 . Calpain and cathepsin activities, and protein extractability during ageing of longissimus porcine muscle from normal and PSE meat . Food Chemistry , 63 : 385 – 390 .
- Gornall , A.G. , Bardawill , C.J. and David , M. 1949 . Determination of serum protein by means of the biuret reagent . Journal of Biological Chemistry , 177 : 751 – 766 .
- Harris , J.R. 1991 . Electron microscopy in biology, a practical approach , 91 – 97 . Oxford : Oxford University Press .
- Hill , A.R. , Irvine , D.M. and Bullock , D.H. 1985 . Buffer capacity of cheese whey . Journal of Food Science , 50 : 733 – 738 .
- Hoffman , L.C. A review of the research conducted on ostrich meat . Paper presented at Proceedings of the 3rd international ratite science symposium of the world's poultry science association and 12th world ostrich congress . pp. 107 – 119 . Beekbergen , , Netherlands : World Poultry Science Association .
- Hoffman , L.L.C. , Muller , M. , Cloete , S.W.P. and Brand , M. 2008 . Physical and sensory meat quality of South African Black ostriches (Struthio camelus var. domesticus), Zimbabwean Blue ostriches (Struthio camelus australis) and their hybrid . Meat Science , 79 : 365 – 374 .
- Honikel , K.O. 1998 . Reference methods for the assessment of physical characteristic of meat . Meat Science , 49 : 447 – 457 .
- Hwang , I.H. , Devine , C.E. and Hopkins , D.L. 2003 . The biochemical and physical effects of electrical stimulation on beef and sheep meat tenderness . Meat Science , 65 : 677 – 691 .
- Immonen , K. and Puolanne , E. 2000 . Variation of residual glycogen glucose concentration at ultimate pH values below 5.75 . Meat Science , 55 : 279 – 283 .
- Ilian , M.A. , Bekhit , A.E. and Bickerstaffe , R. 2004 . The relationship between meat tenderization, myofibril fragmentation and autolysis of calpain 3 during post-mortem aging . Meat Science , 66 : 387 – 397 .
- Inklaar , P.A. and Fortin , J. 1969 . Determining the emulsifying and emulsion stabilizing capacity of protein beef additives . Food Technology , 23 : 103 – 107 .
- Jeremiah , L.E. , Dugan , M.E.R. , Aalhus , J.L. and Gibson , L.L. 2003 . Assessment of the chemical and cooking properties of the major beef muscles and muscle groups . Meat Science , 65 : 985 – 992 .
- Jauregui , C.A. and Regenstein , J.M. 1981 . A simple centrifugal method for measuring expressible moisture, water binding properties of muscle food . Journal of Food Science , 46 : 1217 – 1273 .
- Jeffery , J. 1999 . “ Ostrich production. ” . In Extension veterinarian, Texas Agricultureal Extension Service , The Texas A&M University system . Retrieved from: http://gallus.tamu.edu/ratite/ostrich.html
- Koohmaraie , M. 1994 . Muscle proteinases and meat aging . Meat Science , 36 : 93 – 104 .
- Koohmaraie , M. , Whipple , G. , Kretchmar , D.H. , Crouse , J.D. and Mersmann , H.J. 1991 . Postmortem proteolysis in longissimus muscle from beef, lamb and pork carcasses . Journal of Animal Science , 69 : 617 – 624 .
- Lawrie , R.A. 1998 . Lawrie's meat science , (6th ed , Lancaster , PA : Technomic Publishing Inc .
- Lee , H.E. , Santé-Lhoutellier , V. , Vigouroux , S. , Briand , Y. and Briand , M. 2008 . Role of calpains in postmortem proteolysis in chicken muscle . Poultry Science , 87 : 2126 – 2132 .
- Lee , S. , Stevenson-Barry , J.M. , Kauffman , R.G. and Kim , B.C. 2000 . Effect of ion fluid injection on beef tenderness in association with calpain activity . Meat Science , 56 : 301 – 310 .
- Leygonie , C. , Britz , T.J. and Hoffman , L.C. 2011a . Oxidative stability of previously frozen ostrich Muscularis iliofibularis packaged under different modified atmospheric conditions . International Journal of Food Science & Technology , 46 : 1171 – 1178 .
- Leygonie , C. , Britz , T.J. and Hoffman , L.C. 2011b . Protein and lipid oxidative stability of fresh ostrich M. Iliofibularis packaged under different modified atmospheric packaging conditions . Food Chemistry , 127 : 1659 – 1667 .
- Lombardi-boccia , G. , Martinez Dominguez , B. and Aguzzi , A. 2005 . Total aspects of meat quality: Trace elements and B vitamins in raw and cooked meats . Journal of Food Composition and Analysis , 18 : 39 – 46 .
- Majewska , D. , Jakubowska , M. , Ligocki , M. and Tarasewicz , Z. 2009 . Physicochemical characteristics, proximate analysis and mineral composition of ostrich meat as influenced by muscle . Food Chemistry , 117 : 207 – 211 .
- Makri , E. , Papalamprou , E. and Doxastakis , G. 2005 . Study of functional properties of seed storage proteins from indigenous European legume crops (lupin, pea, broad bean) in admixture with polysaccharides . Food Hydrocolloid , 19 : 583 – 594 .
- Mellet , F.D. and Sales , J. 1997 . Tenderness of Ostrich meat . South African Journal of Food and Nutrition , 9 : 27 – 29 .
- Mkwetshana , N. , Naudé , R.J. and Muramoto , K. 2002 . The purification and characterization of μ-calpain and calpastatin from ostrich brain . The International Journal of Biochemistry & Cell Biology , 34 : 613 – 624 .
- Montero , P. , GO´ Mez-guille´n , M.C. and Borderias , J. 1996 . Influence of subspecies, season and stabilization procedures in gel-forming ability of frozen minced muscle of sardine (Sardina pilchardus) . Food Science & Technology International , 2 : 111 – 122 .
- Olsman , W.J. and Slump , P. 1981 . “ Methods of determination of connective tissue free muscle protein in meat products ” . In Developments of Meat Science , 195 – 240 . London : Elsevier .
- Ouali , A. 1990 . Meat tenderization: Possible causes and mechanisms. A review . Journal of Muscle Foods , 1 : 129 – 165 .
- Paleari , M.A. , Corsico , P. and Beretta , G. 1995 . The ostrich: Breeding, reproduction, slaughtering and nutritional value of the meat . Fleischwirtschaff , 75 : 1120 – 1123 .
- Perez-Alvarez , J.A. , Fernandez-Lopez , J. , Sayas-Barbera , M.E. and Cartagena-Gracia , R. 1998 . Caracterizacion de los parametro de color de diferentes materias mas usadas en la industrial carnica . Eurocarne , 63 : 115 – 137 .
- Polawska , E. , Marchewka , J. , Cooper , R.G. , Sartowska , K. , Pomianowski , J. , Jozwik , A. and Horbanczuk , J.O. … & . 2011b . The ostrich meat – an updated review. II. Nutritive value . Animal Science Papers and Reports , 29 : 89 – 97 .
- Polawska , E. , Marchewka , J. , Krzyzewski , J. , Bagnicka , E. and Wojcik , A. 2011a . The ostrich meat – an updated review. I. Physical characteristics of ostrich meat . Animal Science Papers and Reports , 29 : 5 – 18 .
- Purchas , R.W . 1990 . An assessment of the role of pH differences in determining the relative tenderness of meat from bulls and steers . Meat Science , 27 : 120 – 140 .
- Puolanne , E. and Kivikari , R. 2000 . Determination of post rigor meat . Meat Science , 56 : 7 – 13 .
- Rathgeber , B.M. , Boles , J.A. and Shandi , P.J. 1999 . Rapid postmortem pH decline and delayed chilling rate reduce quality of turkey breast meat . Poultry Science , 78 : 477 – 484 .
- Saffle , R.L. , Christian , J.A. , Carpenter , J.A. and Zirkle , S.B. 1967 . A rapid method to determine stability of sausage emulsion and the effects of processing temperature and humilities on various characteristics and emulsion . Food Technology , 21 : 784 – 788 .
- Sales , J. and Mellett , F.D. 1996 . Post-mortem pH decline in different ostrich muscles . Meat Science , 42 : 235 – 238 .
- Sales , J. and Oliver-Lyons , B. 1996 . Ostrich meat: A review . Food Australia , 48 : 504 – 511 .
- Sazili , A.Q. , Parr , T. , Sensky , P.L. , Jones , S.W. , Bardsley , R.G. and Buttery , P.J. 2005 . The relationship between slow and fast myosin heavy chain content, calpastatin and meat tenderness in different ovine skeletal muscles . Meat Science , 69 : 17 – 25 .
- Seydim , A.C. , Acton , J.C. , Hall , M.A. and Dawson , P.L. 2006 . Effects of packaging atmospheres on shelf-life quality of ground ostrich meat . Meat Science , 73 : 503 – 510 .
- Shackelford , S.D. , Koohmaraie , M. , Miller , M.F. , Crouse , J.D. and Reagan , J.D. 1991 . An evaluation of tenderness of the longissimus muscle of Angus by Hereford versus Brahman crossbred heifers . Journal of Animal Science , 69 : 171 – 177 .
- Shimokomaki , M. , Lourenco Soares , A. and Iouko Ida , E. 2010 . “ Protein and poultry meat quality ” . In Handbook of poultry science and technology, volume 2: Secondary processing , Edited by: Guerrero-Legarreta , Isabel and Hui , Y.H. 332 New York : John Wiley & Sons .
- Soltanizadeh , N. , Kadivar , M. , Keramat , J. and Fazilati , M. 2008 . Comparison of fresh beef and camel meat proteolysis during cold storage . Meat Science , 80 : 892 – 895 .
- Sosulski , F.W. and Fleming , S.E. 1977 . Chemical function and nutritional properties of sunflower protein products . Journal of the American Oil Chemists’ Society , 54 : 100A
- Takahashi , K. , Hattori , A. and Kuroyanagi , H. 1995 . Relationship between translocation of paratropomyosin and restoration of rigor-shortened sarcomeres during post-mortem ageing of meat—a molecular mechanism of meat tenderization . Meat Science , 40 : 413 – 423 .
- Taylor , R.G. , Geesink , G.H. , Thompson , V.F. , Koohmaraie , M. and Gol , D.E. 1995 . Is Z-disk degradation responsible for postmortem tenderisation? . Journal of Animal Science , 73 : 1351 – 1367 .
- Thomas , A.R. , Gondoza , H. , Hoffman , L.C. , Oosthuizen , V. and Naude , R.J. 2004 . The roles of the proteasome, and cathepsins B, L, H and D, in ostrich meat tenderization . Meat Science , 67 : 413 – 20 .
- Turgut , H. 1984 . Emulsifying capacity and stability of goat, water buffalo, sheep and cattle muscle proteins . Journal of Food Science , 44 : 168 – 171 .
- Vallejo-cordoba , B. , Rodríguez-ramírez , R. and González-córdova , R.F. 2010 . Capillary electrophoresis for bovine and ostrich meat characterisation . Food Chemistry , 120 : 304 – 307 .
- Wilson , G.D. , Bray , R.W. and Phillips , P.H. 1954 . The effect of age and grade on the collagen and elastin content of beef and veal . Journal of Animal Science , 13 : 826 – 831 .
- Yam , K.L. and Papadakis , S.E. 2004 . A simple digital imaging method for measuring and analyzing color of food surfaces . Journal of Food Engineering , 61 : 137 – 142 .
- Yanar , M. and Yetim , H. 2001 . The effects of aging period and muscle type on the textural quality characteristics of mutton . Turkish Journal of Veterinary and Animal Science , 25 : 203 – 207 .
- Zhang , S.X. , Farouk , M.M. , Young , O.A. , Weliczko , K.J. and Podmore , C. 2005 . Functional stability of frozen normal and high pH beef . Meat Science , 69 : 765 – 772 .