Abstract
Gelatins with differences in biological origin (bovine, porcine, fish and poultry) and Bloom strength (from 100 to 270 °Bloom) were studied to investigate their characteristics in accordance with their intrinsic properties. Statistical differences were found in most of the parameters evaluated (°Bloom, intrinsic viscosity [η], pH, isoelectric point [IEP], and specific charge quantity). The [η] indicated hydrodynamic volume degree of the macromolecules at dilute concentrations of gelatin. Meanwhile, the gel strength was dependent on the intermolecular hydrogen bonding. °Bloom and intrinsic viscosity showed very poor correlation (R2 < 0.4). Samples with similar °bloom showed very different intrinsic viscosities. The acid-extracted gelatins exhibited high specific charge quantity (> 0.15 meq/g) and IEP (8.0) compared to the bovine alkali extracted sample (< 0.04 meq/g). Electro kinetic characteristics of gelatine molecules appeared to obey the differences due to extraction method during manufacture rather than source.
Gelatinas provenientes de diferentes orígenes biológicos (bovino, porcino, pescado y ave) y valor Bloom (de 100 a 270) fueron estudiadas para investigar sus características en relación a sus propiedades intrínsecas. Se encontraron diferencias significativas en la mayoría de los parámetros evaluados (°Bloom, viscosidad intrínseca [η], pH, punto isoeléctrico [IEP] y cantidad de carga específica). La [η] indicó el volumen hidrodinámico de las moléculas de las soluciones diluidas de gelatina, mientras que la firmeza del gel fue dependiente de los enlaces intermoleculares de hidrógeno. El valor de °Bloom y viscosidad intrínseca mostró una correlación muy deficiente (R2 < 0,4). Las muestras con valores similares de °Bloom mostraron una viscosidad intrínseca muy diferente. Las gelatinas que fueron extraídas en medio ácido exhibieron altas cantidades de carga específica (> 0,15 meq/g) y IEP (8,0) en comparación con la de bovino, la cual fue extraída en medio alcalino (< 0,04 meq/g). Las características electrocinéticas de la molécula de gelatina fueron influenciadas mayormente por el método de extracción en comparación con el origen de donde provenían.
Introduction
Gelatin has been used for a long time as a biological adhesive. Today gelatin is one of the most popular biopolymers for the manufacture of a range of products. It is widely used in the pharmaceutical industry for the manufacture of hard and soft gelatin capsules, coating tablets, and microcapsules (Hussain & Maji, Citation2008; Reich, Citation1995), and in the photography industry, where high-quality gelatin is utilized for the coating of photographic paper (Attridge, Citation1992; Kuge, Arisawa, Aoki, & Hasegawa, Citation2004). In the food industry, it is used for a wide range of applications. Gelatin involves several factors that define its structure and properties. For example, the source of collagen from where gelatin was extracted reflects differences in the thermal stability of gelatin (melting temperature). Another important factor that influences changes on the chemical composition of gelatin is the method of extraction, which could be acid or alkaline (Hinterwaldner, Citation1977). This action results in partial cleavage of the collagen cross-linked structure. The hydrolysis is known commonly as “the conditioning process” (Schrieber & Gareis, Citation2007). It defines the characteristics of gelatin during conversion of collagen to gelatin. In this way, gelatin characteristics are dependent on different parameters such as: acid/alkali extraction, source and thermal treatment parameters and differences in bloom strength (Veis, Citation1964).
The traditional sources of gelatin include bovine and porcine, typically from bones and skin, respectively. However, the requirements for certain groups of people to provide gelatins with halal and kosher characteristics, have led researchers to find gelatins from alternative sources (Cole, Citation1999). Hence, both poultry and fish sources have been proposed to satisfy those groups (Choi & Regenstein, Citation2000; Karim & Bhat, Citation2009). However, less is known about the impact of these alternative gelatins related to its intrinsic properties.
Materials and methods
Gelatin
Gelatins from different sources were obtained as powders with an average 10% water content dry basis. describes the sources, key and supplier details of the materials investigated. FG-150 and PG-240 samples were kindly donated by Healan Co. (UK), FG-260 and PG-190 samples were donated by Rousselot (France) and the rest of samples were obtained from Sigma chemical (UK).
Table 1. Sources of gelatin investigated.
Tabla 1. Orígenes de gelatinas investigadas.
Titrants used
The determination of the isoelectric point (IEP) in anionic gelatin, hydrochloric acid 0.1N was applied. Thus, cationic gelatin was titrated with sodium hydroxide 0.1N. The cationic polydiallyl-dimethylammonium chloride (Poly-DADMAC) 0.001N and anionic sodium polyethylene sulfonate (PES-Na) 0.001N were standard polyelectrolytes used to measure the charge of the gelatins.
Water content determination
Gelatin in powder form was placed in a convection oven at 105°C for 24 h until constant weight was reached.
Three replicates were considered and the average was taken as the final result.
Bloom strength
The bloom strength test was carried out in accordance with the British Standard Method (British Standard-757, Citation1975).
Intrinsic viscosity [η] and molecular weight (Mw)
The intrinsic viscosity [η] was determined by using a capillary viscometry technique (Harding, Citation1997). Since [η] is a limiting value at an infinite dilution, it is a parameter which directly reflects the molecular properties of the solute (Hiemenz, Citation1984). For molecules which can exist with a variety of molecular weights, the relation of [η] and Mw can be described with Mark–Hownik–Kuhn–Sakurada (MHKS) relation (Harding, Citation1998). Therefore, the calculations of Mw were carried out by using the [η].
Results and discussions
Intrinsic viscosity [η] – molecular weight (Mw) relationship
For macromolecules that can exist with a variety of molecular weights such as gelatin, the relationship between [η] and Mw represents one of the most important properties (Schrieber & Gareis, Citation2007; Tanford, Citation1961). shows as an example the [η] of B-225 gelatin obtained by double extrapolation of the ηred/c and ln ηred/c to zero concentration. It was found that the viscosity and gel formation did not have a linear correlation with each other.
Figure 1. Double extrapolation of ηred/c and ln ηred/c to zero for intrinsic viscosity [η] of B–225.
Figura 1. Doble extrapolación de ηred/c y ln ηred/c a cero para la viscosidad intrínseca [η] de B–225.
![Figure 1. Double extrapolation of ηred/c and ln ηred/c to zero for intrinsic viscosity [η] of B–225. Figura 1. Doble extrapolación de ηred/c y ln ηred/c a cero para la viscosidad intrínseca [η] de B–225.](/cms/asset/13237533-08a7-4a95-ad4f-6e0d59cee18c/tcyt_a_658441_f0001.gif)
shows that gelatins with the same gel strength such as A-100 and B-100 had different intrinsic viscosities. The same pattern applies for A-160 and FG-150. However, samples of the same source showed that the higher the viscosity, the greater the gel strength as can be seen in samples PG-190 and PG-240. These results suggested that these functionalities and the performance of the gelatin may be dominated by different properties of the molecular weight fractions within the gelatin.
Figure 2. Relationship between intrinsic viscosity and gel strength in gelatins from different sources. Arrows indicate the increment of gel strength as the viscosity increase within sources.
Figura 2. Relación entre viscosidad intrínseca y firmeza de gel en gelatinas de diferentes orígenes. Las flechas indican el incremento de firmeza de gel conforme la viscosidad incrementa en cada origen.
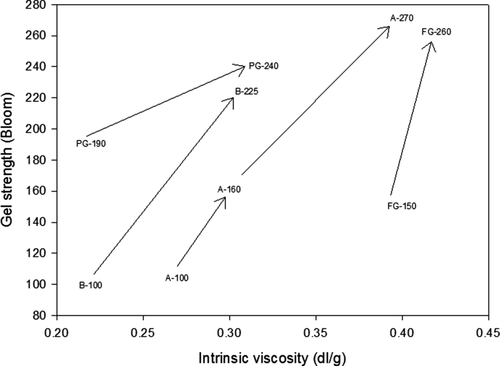
Other facts that need to be considered to explain why two gelatins possessing the same Bloom strength reflected differences in intrinsic viscosity could be related to hydrodynamic volume (Harding, Citation1997) and electro-viscous effects (Shaw, Citation1992). The shape of the molecule in the aqueous media is relevant to its intrinsic viscosity as well as its actual molecular weight.
The acid sequence of gelatin molecules determines its physico-chemical properties such as molecular mass distribution (Schrieber & Gareis, Citation2007). In the manufacturing of gelatin, the conversion of collagen to gelatin results in the creation of different collagen fragments due to the effects of acid or alkali as well as thermal treatment. Therefore, the molecular weight distributions and viscosity of gelatin are determined by the type and intensity of the hydrolysis procedure used. In the case of alkaline-conditioned gelatin such as B-100 and B-225, the major part of molecular weight distributions is in the region of 100,000 g mol−1, which corresponds to the alpha-chains. On the other hand, the rest of the samples evaluated in this work do not have such a pronounced peak, but rather a wider distribution.
Electro kinetic properties of gelatin
Gelatin has an amino acid composition based on the original collagen structure, rich in acid and basic functional groups with the addition of some α -amino and carboxyl groups (Eastoe & Leach, 1977). However, gelatins show different electro kinetic properties similar to polyampholytes (Veis, Citation1964), as the extraction process can alter the charged amino acids groups. For these reasons, the titration analysis for the estimation of charge becomes important in the characterization of gelatin.
For particle charge density (PCD) measurements, the calibration of titrants was carried out before starting the titrations of gelatin solutions.
shows the specific charge density of gelatins from different sources. It can be seen that the two groups were confirmed. Low charges were measured for the alkali-treated gelatins represented by the B-type gelatins. Acid-treated gelatins were observed to have high charge. The greater the distance between pH and IEP, the more the charge was measured.
Figure 3. Electro kinetics properties of gelatin solutions from different sources (20 g/L at 40°C). Specific charge quantity (a) and IEP (b) values are illustrated in the left and right plot, respectively. The measurements were taken at pH 4.5.
Figura 3. Propiedades electrocinéticas de soluciones de gelatina de diferentes orígenes (20 g/L a 40°C). Cantidad de carga específica (a) y IEP (b) los valores están ilustrados en el gráfico izquierdo y derecho, respectivamente. Las mediciones fueron realizadas a un pH de 4,5.
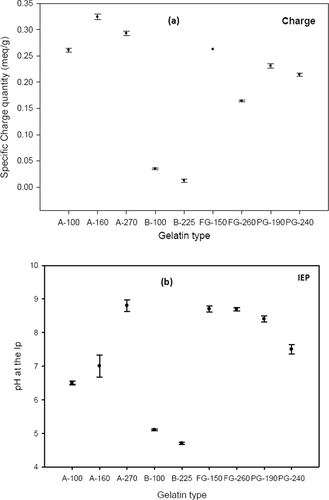
The electrostatic interaction between acid gelatins and polyanions created a complex (Bowman, Rubinstein, & Tan, Citation1997) between the negative charged anionic site of Pes-Na and the available positively charged groups of gelatin, such as amino group (Gillmor, Connelly, Colby, & Tan, Citation1999; Uhrich & Nawn, Citation1970). The addition of Pes-Na to acid-treated gelatin reversed its cationic charge.
The polyanions shifted the pH of gelatin to higher values. This end titration pH value was recorded as the IEP of the macromolecule (see b). Once the IEP was reached, the colloidal system was unstable (Junkers & Agfa-Gevaert, Citation1991). At this point, the mixture showed turbidity and the solubility was decreased, leading the complex Pes-Na–gelatin to phase separation at the end of titration. Gelatin molecule at its IEP shows a random coiled structure because of its neutral charge. As the pH value shift away from the IEP, the charge of the molecule will be changed consequently and the molecule unfolds (Schrieber & Gareis, Citation2007).
The electro kinetic properties of gelatin appear to obey the differences due to extraction method during manufacture, rather than differences in species, viscosity, and Bloom strength. The short times and the acid conditions used for the pig, poultry, and fish gelatin manufactured from their respective collagen sources resulted in retention of the amino acid side groups of the glutamine and asparagines groups during gelatin extraction (Clark & Courts, 1977).
The buffering effect of gelatin
The buffering effect of gelatin was investigated by dissolving gelatin powder A-270 and B-225 at 20 g/L in a range of acid/base solutions from 3 to 11 pH value.
After dissolving, swelling (overnight) and melting (50°C) of gelatin, the pH value was recorded at the beginning of the titration. shows the pH values after addition of gelatin. At the beginning of titration, both A-270 and B-225 were positioned at pH around 5.0, no matter what the original pH of the solution was. This phenomenon was interpreted as the buffer capacity of gelatin due to its amphoteric nature (Dobrynin, Colby, & Rubinstein, Citation2004; Kantor, Kardar, & Li, Citation1994; Veis, Citation1964). It was found that this buffer behavior resembles that of the weak acids such as acetic acid. The values at the end of titration, when zero charge point was reached, were observed near 9.0 for acid extracted gelatin A-270 and 4.7 for alkali processed B-225. These values are in good agreement with the literature (Clark & Courts, 1977).
Figure 4. Determination of IEP in gelatin solution dissolved at different pH value solutions. Key: left for acid-treated and right for alkali-treated.
Figura 4. Determinación de IEP en soluciones de gelatina disueltas a diferentes valores de pH. Clave: izquierda para las tratadas en ácido y derecha para las tratadas en álcali.
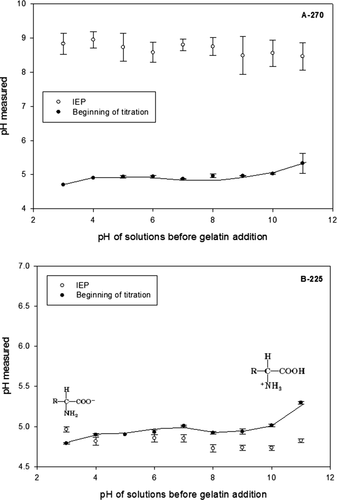
Bloom strength – molecular weight relationship
Stainsby (Citation1977a) found that the minimum Mw value needed to obtain a gelatin gel was 15,000 g mol−1 under the conditions used in this work. Above this value, the gel strength and physical properties of a gel improve until average molecular weights of 90,000 g mol−1.
shows the gel strength of gelatins from different sources as a function of Mw, which was calculated from intrinsic viscosity results. Gelatins with larger Mw values were thought to reduce these gel strength properties as is the case of gelatin B-225 which showed poorer gel strength when compared with low Mw samples such as A-270, FG-260, and PG-240. The viscosity determined the mobility of the gelatin molecules in the liquid, whereas the bloom strength described the structure formed by intermolecular hydrogen bonds.
Figure 5. Relationship between Mw and gel strength in gelatins from different sources. Arrows indicate gelatins with similar gel strength but different Mw. Dotted line shows gelatins with Mw values closer to 90,000 g mol−1.
Figura 5. Relación entre peso molecular y firmeza de gel en gelatinas de diferentes orígenes. Las flechas indican las gelatinas con similar firmeza de gel pero diferente peso molecular. Las líneas punteadas muestran las gelatinas con peso molecular cercano a 90,000 g mol−1.
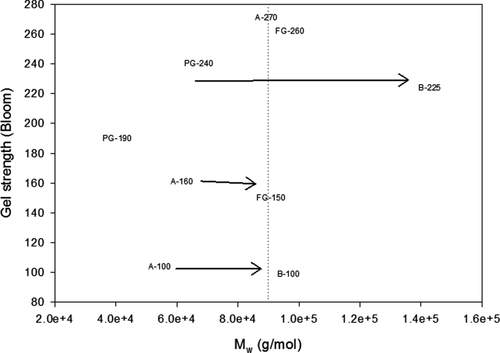
Johnston-Banks (Citation1990) states that low Mw gelatins are composed mainly of α -chains, while, high Mw gelatins are conformed mainly of α -chains. This could be the case for the pair A-100 and B-100, respectively. The presence of several polypeptide chains linked by covalent bonds (α -chains) in the B type gelatins could promote formation of hydrogen bonds (Veis, Citation1964), reducing the possibility of intermolecular hydrogen bond linking when the system was cooled. This resulted in a small number of links per unit volume in the network (low Bloom strength).
There was found a trend of increment of viscosity and therefore, Mw, as the gel strength increased within the sources. For example, pigskin gelatins A-100, A-160, and A-270. The behavior of this intrinsic property remained on gelatin when these pigskin biopolymers were converted to film and evaluated on its mechanical properties (Aguirre-Alvarez, Pimentel-González, Campos-Montiel, Foster, & Hill, Citation2011).
The tendency of increment of viscosity and bloom strength within the same source of gelatin is in good agreement with literature (Kasapis, Al-Marhoobi, & Mitchell, Citation2003). However, these previous works did not use gelatins from different sources. Additionally, the range of molecular weights investigated varied from 30,000 g mol−1 to 68,000 g mol−1. In accordance with Stainsby (Citation1977a), the larger the Mw, the higher the strength gel will be observed within the Mw range 15,000 g mol−1 to 90,000 g mol−1.
Conclusions
The intrinsic properties of gelatin from different origins were affected by parameters inherent to the manufacture of gelatin such as bloom strength, specific charge, IEP, and viscosity rather than origin. These results suggest that the bovine and pigskin gelatins can be replaced with those of fish and poultry. This interchange will depend on the expected characteristics of the final product such as stability, viscosity, color, and consistency.
Acknowledgments
The first author gratefully acknowledges the Universidad Autónoma del Estado de Hidalgo and Programa para el Mejoramiento del Profesorado (PROMEP) for their financial support.
References
- Aguirre-Alvarez, G., Pimentel-González, D.J., Campos-Montiel, R.G., Foster, T., and Hill, S.E., 2011. The effect of drying temperature on mechanical properties of pig skin gelatin films, CyTA – Journal of Food 9 (2011), pp. 243–249, doi: 10.1080/19476337. 2010.523902.
- Attridge, G., 1992. Color in photography, Journal of the Society of Dyers and Colourists 108 (1992), pp. 523–526.
- Bowman, W.A., Rubinstein, M., and Tan, J.S., 1997. Polyelectrolyte–gelatin complexation: Light-scattering study, Macromolecules 30 (1997), pp. 3262–3270.
- British Standard-757, 1975. United Kingdom Patent No.. British Standards; 1975.
- Clark, R., and Courts, A., 1977. "The chemical reactivity of gelatin". In: Ward, A.G., ed. The Science and Technology of gelatin. London: Academic Press; 1977. pp. 209–241.
- Cole, B., 1999. "Gelatin". In: Francis, F., ed. Encyclopedia of Food Sciences and Technology. New York: Wiley; 1999. pp. 1183–1188.
- Choi, S.S., and Regenstein, J.M., 2000. Physicochemical and sensory characteristics of fish gelatin, Journal of Food Science 65 (2000), pp. 194–199.
- Dobrynin, A.V., Colby, R.H., and Rubinstein, M., 2004. Polyampholytes, Journal of Polymer Science Part B – Polymer Physics 42 (2004), pp. 3513–3538.
- Eastoe, J.E., and Leach, A.A., 1977. "Chemical constitution of gelatin". In: Ward, A.C., ed. The science and technology of gelatin. London: Academic Press Inc; 1977. pp. 73–105.
- Gillmor, J.R., Connelly, R.W., Colby, R.H., and Tan, J.S., 1999. Effect of sodium poly(styrene sulfonate) on thermoreversible gelation of gelatin, Journal of Polymer Science Part B – Polymer Physics 37 (1999), pp. 2287–2295.
- Harding, S.E., 1997. The intrinsic viscosity of biological macromolecules. Progress in measurement, interpretation and application to structure in dilute solution, Progress In Biophysics & Molecular Biology 68 (1997), pp. 207–262.
- Harding, S.E., 1998. "Dilute solution viscometry of food biopolymers". In: Hill, S.E., Lard, D.A., and Mitchell, J.R., eds. Functional properties of food macromolecules. Gaithersburg, Maryland: Aspen publishers Inc; 1998.
- Hiemenz, P.C., 1984. Polymer chemistry: The basic concepts. New York: Marcel Dekker, Inc; 1984.
- Hinterwaldner, R., 1977. "Technology of gelatin manufacture". In: Stainsby, G., ed. The science and technology of gelatin. London: Academic Press; 1977. pp. 315–461.
- Hussain, M.R., and Maji, T.K., 2008. Preparation of genipin cross-linked chitosan-gelatin microcapsules for encapsulation of Zanthoxylum limonella oil (ZLO) using salting-out method, Journal of Microencapsulation 25 (2008), pp. 414–420.
- Johnston-Banks, F.A., 1990. "Gelatine". In: Harris, P., ed. Food gels. London: Elsevier Applied Food Sciences; 1990. pp. 233–290.
- Junkers, G., and Agfa-Gevaert, A., 1991. Electrokinetic characterization of gelatin and its reaction with polyelectrolytes by a streaming current detector. Leverkusen, Germany: BTG Mutek GmbH; 1991.
- Kantor, Y., Kardar, M., and Li, H., 1994. Statistical-mechanics of polyampholytes, Physical Review E 49 (1994), pp. 1383–1392.
- Karim, A.A., and Bhat, R., 2009. Fish gelatin: properties, challenges, and prospects as an alternative to mammalian gelatins, Food Hydrocolloids 23 (2009), pp. 563–576.
- Kasapis, S., Al-Marhoobi, I.M., and Mitchell, J.R., 2003. Molecular weight effects on the glass transition of gelatin/cosolute mixtures, Biopolymers 70 (2003), pp. 169–185.
- Kuge, K., Arisawa, M., Aoki, N., and Hasegawa, A., 2004. Preparation of gold clusters in gelatin layer film using photographic film – 4: Effect of photographic materials, Imaging Science Journal 52 (2004), pp. 176–180.
- Reich, G., 1995. Effect of drying conditions on structure and properties of gelatin capsules – studies with gelatin films, Pharmazeutische Industrie 57 (1) (1995), pp. 63–67.
- Schrieber, R., and Gareis, H., 2007. Gelatine handbook. Theory and industrial practice. Germany: Wiley-VCH; 2007.
- Shaw, D., 1992. Introduction to colloid & surface chemistry. Butterworth: Heinemann; 1992.
- Stainsby, G., 1977a. "The gelatin gel and the sol–gel transformation". In: Ward, A.C., ed. The science and technology of gelatin. London: Academic Press Inc; 1977a. pp. 179–208.
- Tanford, C., 1961. Physical chemistry of macromolecules. New York, NY: John Wiley & Sons, Inc; 1961.
- Uhrich, C., and Nawn, G., 1970. Interactions of polyanions with gelatins, Photographic Science and Engineering 14 (1970), p. 178.
- Veis, A., 1964. The macromolecular chemistry of gelatin. London: Academic Press Inc; 1964.