Abstract
Protein oxidation of peanut beverage and their stability during the storage were investigated. To further investigate the relationship between protein oxidation and peanut beverage stability, peanut protein isolate (PPI) was oxidized by peroxyl radicals derived from 2,2ʹ-azobis (2-amidinopropane) dihydrochloride (AAPH), and the emulsion properties stabilized by oxidized PPI were evaluated. During the storage, protein oxidation happened in the peanut beverage, accompanied by deteriorating their stability. Low storage temperature would lighten the oxidation extent and the peanut beverage instability. High roasting pretreatment of peanut kernels before making peanut beverage would enhance the protein oxidation. Emulsion stabilized by oxidized PPI had larger mean droplet size and lower surface charge. Microstructure of PPI stabilizing emulsion showed that emulsions stabilized by PPI of overoxidation underwent severe droplet aggregation during storage. Protein oxidation was an important factor influencing stability during peanut beverage storage.
El presente estudio investigó la oxidación experimentada por bebidas de cacahuate y la estabilidad de las mismas durante el almacenamiento. Con el objetivo de examinar a fondo la relación existente entre la oxidación proteica y la estabilidad de bebidas de cacahuate, se oxidó el aislado de proteína de cacahuate (PPI) a través de radicales de peroxil derivados de 2,2ʹ-azobis (2-amidinopropano) dicloridrato (AAPH); además, se estudiaron las propiedades de la emulsión estabilizada por PPI oxidado. Se constató que durante el almacenamiento se presentó la oxidación proteica de las bebidas de cacahuate, acompañada del deterioro de su estabilidad. Se concluyó que bajas temperaturas de almacenamiento ayudarían a reducir tanto el grado de oxidación como la inestabilidad de las bebidas de cacahuate y que la oxidación proteica aumentaría si los granos de cacahuate fueran tratados previamente con un tostado a altas temperaturas. Por otra parte, se observó que en la emulsión estabilizada por el PPI oxidado, las gotas presentaron un tamaño promedio más elevado y una carga superficial más baja. La microestructura de la emulsión estabilizadora del PPI demostró que las emulsiones estabilizadas por el PPI de sobre oxidación, desarrollaron una agregación de gotas muy elevada durante el almacenamiento. La oxidación proteica fue uno de los factores importantes que influyeron en el almacenamiento de las bebidas de cacahuate.
1. Introduction
Plant protein beverages as alternative sources of dietary protein are desirable in developing countries where cows’ milk may be costly, unavailable or not consumed due to dietary constraints or religion (Lee & Beuchat, Citation1992). Peanut beverage is easy to prepare and its amino acid composition meets people’s nutritional requirement (Howard, Hung, & McWatters, Citation2010; Jain, Yadav, Rajput, & Bhatt, Citation2013). Therefore, it has great potential as a dairy substitute or supplement.
Peanut beverage is a kind of emulsion. During their storage, instability would happen, even after homogenization and addition of stabilizers to improve the emulsion stability (Encarnacion & Rillo, Citation1982; Rubico, Resurreccion, Frank, & Beuchat, Citation1987). These defects are related to particles that are relatively larger than the surrounding medium that cause creaming or layering due to flocculation and coalescence (Rubico et al., Citation1987). In the peanut beverage, peanut protein not only serves as a nutritional source but also serves as an emulsifier, playing an important role in the emulsion stability. The emulsifying capability of proteins depends on their molecular structure and physicochemical characteristics (Keerati-u-rai, Miriani, Iametti, Bonomi, & Corredig, Citation2012; Vázquez-Ovando, Betancur-Ancona, & Chel-Guerrero, Citation2013).
Peanut beverage is also a lipid-enriched system. Lipids are sensitive to oxidation and lead to rancid flavor resulted in shortening shelf life. Lipid oxidation will also produce lipid peroxidation-derived free radicals as well as lipid hydroperoxides and reactive aldehydes, which can interact with the proteins, leading to protein oxidation (Refsgaard, Tsai, & Stadtman, Citation2000). The protein can be attacked directly by reactive oxygen species or indirectly by reaction with the by-products of lipid peroxidation, resulting in a number of changes in amino acid residue side chains and protein polypeptide backbone (Shacter, Citation2000). Therefore, conformation of protein under oxidative stress would change, which has also been observed by many former studies (Wu, Wu, & Hua, Citation2010; Wu, Zhang, Kong, & Hua, Citation2009; Ye, Liao, Zhao, & Sun, Citation2013). As protein emulsifying properties are closely related with its structure, protein oxidation would affect its emulsifying properties. Wu, Hua, Lin, and Xiao (Citation2011) noticed that oxidation would deteriorate the gelling properties of soy protein isolate. Chen, Zhao, Sun, Ren, and Cui (Citation2013) reported that oxidation had both positive and negative effects on the emulsifying properties of soy protein isolate depending on the oxidation extent. Srinivasan and Hultin (Citation1997) found that oxidation might have positive effect on the emulsifying and gelling properties of cod proteins. However, the former studies used the simulated reactive oxygen species (ROS)-generating systems to trigger protein oxidation and then investigated the conformation and functionalities change of oxidized protein. So, it is necessary to evaluate the effect of protein oxidation in a real lipid-enriched system on its characteristics. In addition, heat treatment could also induce the peanut protein conformational changes and oxidation. Damame et al. (Citation1990) found that the dry roasting and oil roasting of peanut kernels could significantly decrease methionine and tryptophan and increase soluble proteins. However, Neucere et al. (Citation1969) found that total soluble protein was decreased considerably after dry roasting. Roasting temperature played an important role in these changes.
The objective of this study was to evaluate the relationship between peanut beverage stability and protein oxidation. Effects of roasting and storage temperature on peanut beverage stability were investigated. As peanut beverage is a relatively complex food system, in order to further confirm their relationship, a simulated oxidizing system triggered by 2,2ʹ-azobis (2-amidinopropane) dihydrochloride (AAPH) was also used. Peanut protein is oxidized by AAPH-derived peroxyl radicals, and the properties of emulsion stabilized by AAPH pretreated peanut protein isolate (PPI) were investigated. The emulsion properties were characterized by mean droplet size, ζ-potential and microstructure.
2. Materials and methods
2.1. Samples and materials
Peanut kernels (thousand grain weight 1.02 ± 0.12 kg) were purchased from the local supermarket (PARKnSHOP, Tianhe District, Guangzhou, China). They were vacuum packed and stored at 4°C before used. Low temperature defatted peanut flour (below 60°C during the process) was purchased from Tianshen Bioprotein Co., Ltd. (Linyi, Shandong, China). AAPH was purchased from Sigma-Aldrich (St. Louis, MO). All other chemicals were of analytical reagent grade.
2.2. Peanut beverage preparation
Peanut kernels (800 g) were subjected to dry roasting in hot air oven under 100°C and 140°C for 35 min, respectively. Samples without roasting were used as control. Then they were mixed with deionized water [the ratio of water to dry kernels was 10:1 (w/w)] and incubated at 65 ± 5°C for 8 h, drained and rinsed with deionized water for three times. After that, they were ground with deionized water [the ratio of water to dry kernels was 10:1 (w/w)], using a high speed blender (BL021, Zhongshan Haipan Electrical Co., Ltd., Zhongshan, China). The peanut kernel slurry was passed through a colloid mill (JTM60, Shenzhen LET Co., Ltd., Shenzhen, China). After that, the slurry was filtered through a muslin cloth to separate the peanut pulp from the raw peanut beverage, and their protein content was determined by using Kjeldahl method (AOAC Methods, Citation1990). The protein content in all samples were then adjusted to 1% (w/v) diluted with deionized water and mixed with 7% (w/v, final concentration) sugar, 0.1% (w/v, final concentration) combined emulsifying stabilizing agent (xanthan and guar gum, 3:1) and 0.05% salt. Xanthan and guar gum (food grade) were kindly donated by Rhodia Group (Shanghai, China). The peanut beverage was then homogenized with APV-1000 homogenizer (APV Gaulin, Abvertslund, Denmark) twice at 25 MPa. The homogenized filtrate was poured into 100-mL glass jar (more than 60 jars) and sealed with crown cap. Finally, the packaged samples were sterilized in the autoclave (121°C, 15 min). The sterilized peanut beverage was stored at 25°C (30 jars) and 4°C (30 jars), respectively.
2.3. PPI preparation
Defatted peanut flour was mixed with 20-fold deionized water, and the pH of the dispersion was adjusted to 8.0 with 2 M NaOH. The mixture was gently stirred at 25°C for 2 h and then centrifuged at 8000 g for 30 min at 20°C. The supernatant was adjusted to pH 4.5 with 2 M HCl and then centrifuged at 5000 g for 20 min at 20°C. The precipitate was then redissolved in fivefold deionized water and adjusted to pH 7.0 with 2 M NaOH. This solution was freeze-dried to produce PPI.
2.4. PPI oxidation
Oxidized PPI was prepared according to the method described by Wu et al. (Citation2009). PPI dispersion (25 mg/mL containing 0.5 mg/mL sodium azide, suspended in 10 mM sodium phosphate buffer, pH 7.4) was mixed with a serial concentration of AAPH and then incubated by continuous shaking under air at 37°C in dark for 24 h. The final concentration of AAPH was 0, 0.04, 0.2, 0.5, 1, 3 and 5 mM. The reaction was stopped by immediately cooling the solution to 4°C by ice-bathing and then centrifuged at 8000 g for 15 min at 4°C. The supernatant was dialyzed against deionized water at 4°C for 72 h to remove residual AAPH and salt. Oxidized PPI was obtained by freeze-drying and stored at 4°C until use.
2.5. Extraction of peanut protein from peanut beverage
Protein from lipid-enriched system was extracted by using the method described by Cucu, Devreese, Mestdagh, Kerkaert, and De Meulenaer (Citation2011). Peanut beverages were defatted four times with technical hexane to remove free lipids. Then the defatted fractions were collected and precipitated with trichloroacetic acid (10% final concentration) on ice. After centrifugation, the precipitated proteins were finally redissolved in 2% sodium dodecyl sulfate and the pH was adjusted to approximately 10 with 10 M NaOH to facilitate redissolving the pellet. Undissolved particles were removed by centrifugation at 10,000 g for 10 min. The clear supernatant was immediately used for the determination of protein carbonyl group content.
2.6. Protein carbonyl measurement
Protein carbonyl was detected by using the method described by Oliver, Ahn, Moerman, Goldstein, and Stadtman (Citation1987) with slight modification (Sun, Cui, Zhao, Zhao, & Yang, Citation2011). Protein concentration of PPI was determined by Biuret method using bovine serum albumin as a standard. The results were expressed as nanomole of carbonyl groups per milligram of soluble protein with molar extinction coefficient of 22,000 M−1 cm−1.
2.7. Intrinsic fluorescence emission spectra of peanut beverage
Intrinsic emission fluorescence spectra of peanut beverage were evaluated using the fluorescence spectrophotometer (F7000, HITACHI, Tokyo, Japan) according to the method described by Estévez, Kylli, Puolanne, Kivikari, and Heinonen (Citation2008) with minor modifications. Peanut beverage was diluted by 10 mM phosphate buffer (pH 7.0). The diluted peanut beverages were excited at 290 nm, and emission spectra were recorded from 300 to 400 nm at a constant slit of 5 nm for both excitation and emission. The maximum emission intensity of the spectra was recorded for analysis. The results were expressed as fluorescence arbitrary units.
2.8. Determination of mean droplet size
Droplet size distribution of peanut beverage and emulsion were determined using a Malvern Mastersizer 2000 (Malvern Instruments, Worcestershire, United Kingdom). The mean droplet size was characterized in terms of the volume mean diameter (d4,3) and surface area mean diameter (d3,2). The d4,3 and d3,2 calculations were done by using the Malvern Mastersizer 2000 software version 5.60 supplied by the manufacturer (Malvern Instruments Ltd.).
2.9. ζ-Potential measurement
The ζ-potential of emulsion was measured using the Zetasizer Nano-ZS instrument. Sample was diluted (50 μL in 5 mL of 10 mM sodium phosphate buffer, pH 7.0) and injected into a folded capillary cell with electrodes at either end to which a potential was applied. The cell was placed in a cell chamber controlled at 25°C, and the velocity of the charged droplet movement toward the oppositely charged electrode was measured. The ζ-potential was then calculated from the droplet velocity using Dispersion Technology Software version 4.20.
2.10. Microstructure of emulsion
Images of the emulsion were observed after emulsion preparation within 24 h using a CX31-12C04 microscope (Olympus Co., Tokyo, Japan) according to the method described by Sun, Zhou, Sun, and Zhao (Citation2013). Samples of the emulsion were taken from the emulsion as a whole after carefully blending. A drop of the nondiluted emulsion was placed on a microscope slide that was then covered with a coverslip and visualized at a magnification of 400×. The images were captured by a charge-coupled device camera (Olympus Co., Tokyo, Japan) connected to the microscope, and the photographs of the emulsions were recorded for analysis.
2.11. Statistical analysis
Statistical calculations were performed using the statistical package SPSS 17.0 (SPSS Inc., Chicago, IL, USA) for one-way analysis of variance. Student–Newman–Keuls test was used for comparison of mean values among treatments and to identify significant differences (p < 0.05) among treatments. All the data were expressed as means ± standard deviations of triplicate determinations (n = 3).
3. Results and discussion
3.1. Oxidation characterization of peanut beverage
Total protein carbonyl group content was used to evaluate the peanut protein oxidation in peanut beverage. Carbonyl derivatives form by oxidation of some amino acid residues and oxidative cleavage of the peptide backbone under oxidative stress (Berlett & Stadtman, Citation1997). Effects of roasting temperature, storage temperature and storage time on total carbonyl group content of peanut beverage are given in . Results indicated that carbonyl group content gradually increased with both the increasing roasting temperature and the time of storage. At 25°C, the carbonyl group content of nonroasting sample was 1.44 nmol/mg after 1 day storage, while that of roasting sample at 140°C was 1.68 nmol/mg. With increasing storage time, carbonyl group content increased significantly (p < 0.05). At 4°C storage temperature, samples after roasting still had higher carbonyl group level, and with the increasing storage time, carbonyl group content also increased (p < 0.05). Notably, the overall carbonyl group level was significantly lower (p < 0.05) than that at 25°C. These results indicated that protein oxidation occurs during the storage of peanut beverage. The oxidation extent would be influenced by the storage conditions. Lower temperature can weaken the oxidation process during the storage, while the storage time would increase the oxidation extent of peanut protein. For the sample of 140°C roasting temperature at 30 days, the oxidation extent of peanut protein at 4°C is 20.94% less than that at 25°C storage temperature.
Table 1. Effects of storage temperature and roasting temperature on the carbonyl group content of peanut beverage during storage.
Tabla 1. Efectos de la temperatura de almacenamiento y de la temperatura de tostado en el contenido del grupo de carbonilo de las bebidas de cacahuate durante el almacenamiento.
In order to further characterize the oxidation status, intrinsic fluorescent spectra of peanut beverage were investigated. This method had been used to investigate the oxidation status of oil-in-water emulsions stabilized by different proteins (Chen et al., Citation2013; Viljanen, Kivikari, & Heinonen, Citation2004). Results indicated that with the increasing roasting temperature and storage time, fluorescent intensity gradually decreased (). At 25°C, the fluorescent intensity of nonroasting sample was 701.7 after 1 day of storage, while that of roasting sample at 140°C was 457.8. The decrease (p < 0.05) in peanut beverage fluorescence was observed in all samples during storage. At 4°C storage temperature, similar phenomenon was observed. Notably, the overall fluorescent intensity at 4°C except roasting sample at 140°C was significantly higher (p < 0.05) than that at 25°C. Many former studies had confirmed that oxidation would result in decrease in fluorescent intensity (Chen et al., Citation2013; Viljanen et al., Citation2004). The gradual and remarkable decrease in the fluorescence intensity during storage of peanut beverage indicates the gradual structural unfolding of proteins (Sun, Zhao, Yang, Zhao, & Cui, Citation2011). The oxidation patterns of myofibrillar protein in oil-in-water emulsions resulted in the loss of tryptophan fluorescence (Estévez et al., Citation2008). The decrease in the fluorescence intensity during peanut beverage storage was a direct consequence of the oxidative degradation of tryptophan. Therefore, these results further proved the former investigation that peanut protein might be oxidized during storage of peanut beverage. And its oxidation status was affected by roasting temperature and the storage temperature. Protein oxidation would cause their conformation modification, which would have great influence on their functionalities. Peanut protein would play an important part in the stability of peanut beverage as it was in part used as an emulsifier in this system.
Table 2. Effects of storage temperature and roasting temperature on the fluorescent intensity of peanut beverage during storage.
Tabla 2. Efectos de la temperatura de almacenamiento y de la temperatura de tostado en la intensidad fluorescente de las bebidas de cacahuate durante el almacenamiento.
3.2. Stability of peanut beverage
Droplet size is closely related with the stability of emulsion system. Therefore, the mean droplet size d4,3 and d3,2 were used to characterize the stability of peanut beverage in this work. Results indicated that droplet size is significantly affected by both roasting temperature and storage time. As shown in , on the whole, the d4,3 and d3,2 values of peanut beverage remained relatively constant when it was stored under 4°C. With the increasing storage time, the d4,3 and d3,2 values increased significantly (p < 0.05). Kiokias, Reiffers-Magnani, and Bot (Citation2004) and Korhonen, Hellen, Hirvonen, and Yliruusi (Citation2001) found that storage time and conditions of emulsions could affect their mean droplet size. Results in this work were in agreement with the reports that low temperature storage could be beneficial for emulsion stability. In addition, with the increasing roasting temperature, the d4,3 and d3,2 values did not change along with the total protein carbonyl group content and fluorescence intensity. It might be because the total soluble protein decreased considerably after dry roasting and protein conformational changes (Neucere et al., Citation1969). Emulsion droplets depend on protein molecular structure and physicochemical characteristics (Keerati-u-rai et al., Citation2012).
Table 3. Effects of storage temperature and roasting temperature on the mean droplet size of peanut beverage during storage.
Tabla 3. Efectos de la temperatura de almacenamiento y de la temperatura de tostado en el tamaño promedio de las gotas de las bebidas de cacahuate durante el almacenamiento.
Wu et al. (Citation2009, Citation2010) reported that protein oxidation would decrease the surface hydrophobicity of soy protein isolate. In general, protein with relative high surface hydrophobicity would have better emulsifying properties. Ye et al. (Citation2013) found that protein oxidation would induce the peanut protein conformational changes. Therefore, during storage the peanut beverage, instability might be caused by protein oxidation even though rarely studies had reported the relationship between emulsion stability and oxidized protein.
3.3. Emulsifying characteristics of oxidized PPI
In order to further confirm that oxidation had effect on the peanut beverage stability, it is necessary to find a relatively simple system to generate the peanut protein oxidation and then investigate the properties of emulsion stabilized by oxidized peanut protein. In this work, PPI was incubated with AAPH, which would generate peroxyl radicals, inducing the protein oxidation.
Results indicated that with the increasing concentration of AAPH, d4,3 and d3,2 values of emulsion stabilized by PPI increased as a whole (). Incubating with 5 mM AAPH resulted in approximately 6.36-fold increase of d4,3, which indicated lower emulsion stability. It was noteworthy that the values of d3,2 decreased (p < 0.05) at 0.04 mM. The d4,3 values also decreased but not significantly (p > 0.05). Effect of protein oxidation on their emulsifying properties might be governed by their molecular structure and physicochemical characteristics. Oxidative characteristics of the AAPH-pretreated PPI had been reported by our former study (Ye et al., Citation2013). The investigation noticed that by incubating with increasing AAPH concentration, PPI oxidation extent enhanced, accompanied by a decreasing surface hydrophobicity and formation of soluble aggregates. High-pressure homogenization during emulsion preparation could lead to the diversity of structure flexibility of the aggregates that could easily attach to the interface and form a thicker adsorbed layer, resulting in changes in particle sizes of the emulsions (Yuan, Ren, Zhao, Luo, & Gu, Citation2012). Homogenization would have less effect on highly oxidized soy protein isolate due to their nonflexible structure (Chen et al., Citation2013). The net surface charge of emulsion particle in solution also affected their particle sizes (). Different molecular structures and net surface charge of native and oxidized peanut protein would have effects on their structure rearrangement on the oil–water interface, resulting in their different emulsifying capabilities.
Table 4. Effects of AAPH oxidation on the mean droplet size of emulsion stabilized by PPI.
Tabla 4. Efectos de la oxidación de aaph en el tamaño promedio de las gotas de la emulsión estabilizada por el ppi.
Figure 1. ζ-Potential of emulsion stabilized by PPI incubated with increasing concentration of AAPH for 24 h at 37°C.Note: Columns with different letters (a-c) are significantly different (p < 0.05).
ζ-Potencial de la emulsión estabilizada por el PPI incubado con una creciente concentración de AAPH durante 24 h a 37°C.
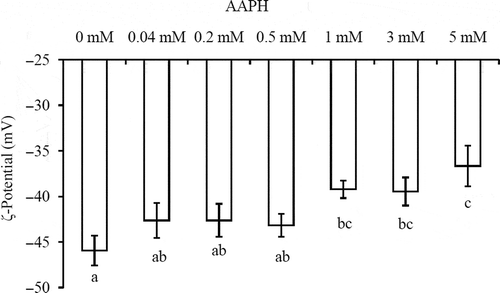
ζ-Potential as an indicator of the surface charge property of particle in solution is another important index of the emulsion properties. As shown in , ζ-potential of emulsion decreased with the emulsion stabilized by PPI incubated with increasing AAPH concentration. Higher surface charge would enhance the electrostatic repulsive force between emulsion droplets to overcome the attractive interaction like van der Waals and hydrophobic attraction (Chen et al., Citation2013; Kim, Decker, & McClements, Citation2002). Therefore, the lower surface charge of emulsion stabilized by PPI with higher oxidation extent indicated a worse emulsion stability. In order to further characterize their stability, the microstructure of the emulsion stabilized by oxidized PPI was also investigated (). The emulsion showed significant structural difference as a function of AAPH concentration. Droplet coalescence and flocculation gradually appeared in the emulsion stabilized by PPI with increasing oxidation extent. These observations were well in accordance with the results of emulsion mean droplet size and ζ-potential.
Figure 2. Microstructure of the emulsions stabilized by PPI incubated with increasing concentration of AAPH for 24 h at 37°C.
Microestructura de las emulsiones estabilizadas por el PPI incubado con una creciente concentración de AAPH durante 24 h a 37°C.
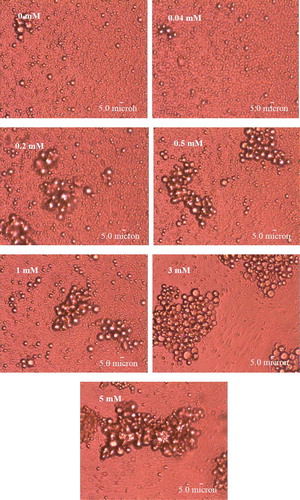
The observations proved that the emulsion stabilized by oxidized PPI would have lower stability. Therefore, the peanut beverage instability during storage would partly attribute to the peanut protein oxidation.
4. Conclusions
Results from this investigation showed that high roasting pretreatment would induce peanut protein oxidation. Protein oxidation that occurred during storage affected the peanut beverage stability. Further investigation using the simulated oxidation system indicated that peanut protein oxidation would induce the instability of emulsion. The stability of peanut beverage during storage was in part related with protein oxidation. Further work will be carried out to protect peanut protein from oxidation during industry process.
Acknowledgements
The authors are grateful to the National High Technology Research and Development Program of China (863 Program) (No. 2013AA102201) and the National Natural Science Foundation of China (No. 31171783).
References
- AOAC Methods. (1990). Method 960.52. In K. Helirich (Ed.), Official methods of association of official analytical chemists international (15th ed.). Arlington, VA: Association of Official Analytical Chemists.
- Berlett, B. S., & Stadtman, E. R. (1997). Protein oxidation in aging, disease, and oxidative stress. Journal of Biological Chemistry, 272, 20313–20316. doi:10.1074/jbc.272.33.20313
- Chen, N., Zhao, M., Sun, W., Ren, J., & Cui, C. (2013). Effect of oxidation on the emulsifying properties of soy protein isolate. Food Research International, 52, 26–32. doi:10.1016/j.foodres.2013.02.028
- Cucu, T., Devreese, B., Mestdagh, F., Kerkaert, B., & De Meulenaer, B. (2011). Protein–lipid interactions during the incubation of whey proteins with autoxidizing lipids. International Dairy Journal, 21, 427–433. doi:10.1016/j.idairyj.2011.01.003
- Damame, S. V., Chavan, J. K., & Kadam, S. S. (1990). Effects of roasting and storage on proteins and oil in peanut kernels. Plant Foods for Human Nutrition, 40(2), 143–148. doi:10.1007/BF02193772
- Encarnacion, S. S., & Rillo, B. O. (1982). Improvement of quality characteristics of peanut milk. UP Home Economics Journal, 10, 43–61.
- Estévez, M., Kylli, P., Puolanne, E., Kivikari, R., & Heinonen, M. (2008). Fluorescence spectroscopy as a novel approach for the assessment of myofibrillar protein oxidation in oil-in-water emulsions. Meat Science, 80(4), 1290–1296. doi:10.1016/j.meatsci.2008.06.004
- Howard, B. M., Hung, Y. C., & McWatters, S. K. (2010). Analysis of ingredient functionality and formulation optimization of an instant peanut beverage mix. Journal of Food Science, 75(1), S8–S19. doi:10.1111/j.1750-3841.2009.01380.x
- Jain, P., Yadav, D. N., Rajput, H., & Bhatt, D. K. (2013). Effect of pressure blanching on sensory and proximate composition of peanut milk. Journal of Food Science and Technology-Mysore, 50(3), 605–608. doi:10.1007/s13197-011-0373-5
- Keerati-u-rai, M., Miriani, M., Iametti, S., Bonomi, F., & Corredig, M. (2012). Structural changes of soy proteins at the oil-water interface studied by fluorescence spectroscopy. Colloids Surfaces B: Biointerfaces, 93, 41–48. doi:10.1016/j.colsurfb.2011.12.002
- Kim, H. J., Decker, E. A., & McClements, D. J. (2002). Role of post adsorption conformation changes of β-lactoglobulin on its ability to stabilize oil droplets against flocculation during heating at neutral pH. Langmuir, 18, 7577–7583. doi:10.1021/la020385u
- Kiokias, S., Reiffers-Magnani, C. K., & Bot, A. (2004). Stability of whey-protein-stabilized oil-in-water emulsions during chilled storage and temperature cycling. Journal of Agricultural and Food Chemistry, 52(12), 3823–3830. doi:10.1021/jf035441r
- Korhonen, M., Hellen, L., Hirvonen, J., & Yliruusi, J. (2001). Rheological properties of creams with four different surfactant combinations-effect of storage time and conditions. International Journal of Pharmaceutics, 221(1–2), 187–196. doi:10.1016/S0378-5173(01)00675-5
- Lee, C., & Beuchat, L. (1992). Chemical, physical and sensory characteristics of peanut milk as affected by processing conditions. Journal of Food Science, 57, 401–405. doi:10.1111/j.1365-2621.1992.tb05503.x
- Neucere, N. J., Ory, R. L., & Carney, W. B. (1969). Effect of roasting on the stability of peanut proteins. Journal of Agricultural and Food Chemistry, 17(1), 25–28. doi:10.1021/jf60161a007
- Oliver, C. N., Ahn, B. W., Moerman, E. J., Goldstein, S., & Stadtman, E. R. (1987). Age-related changes in oxidized proteins. Journal of Biological Chemistry, 262, 5488–5491.
- Refsgaard, H. H. F., Tsai, L., & Stadtman, E. R. (2000). Modifications of proteins by polyunsaturated fatty acid peroxidation products. Proceedings of the National Academy of Sciences of the United States of America, 97, 611–616. doi:10.1073/pnas.97.2.611
- Rubico, S. M., Resurreccion, A. V. A., Frank, J. F., & Beuchat, L. R. (1987). Suspension stability, texture, and color of high temperature treated peanut beverage. Journal of Food Science, 52, 1676–1679. doi:10.1111/j.1365-2621.1987.tb05904.x
- Shacter, E. (2000). Quantification and significance of protein oxidation in biological samples. Drug Metabolism Reviews, 32, 307–326. doi:10.1081/DMR-100102336
- Srinivasan, S., & Hultin, H. O. (1997). Chemical, physical, and functional properties of cod proteins modified by a nonenzymic free-radical-generating system. Journal of Agricultural and Food Chemistry, 45, 310–320. doi:10.1021/jf960367g
- Sun, W., Cui, C., Zhao, M., Zhao, Q., & Yang, B. (2011). Effects of composition and oxidation of proteins on their solubility, aggregation and proteolytic susceptibility during processing of Cantonese sausage. Food Chemistry, 124(1), 336–341. doi:10.1016/j.foodchem.2010.06.042
- Sun, W., Zhao, M., Yang, B., Zhao, H., & Cui, C. (2011). Oxidation of sarcoplasmic proteins during processing of Cantonese sausage in relation to their aggregation behaviour and in vitro digestibility. Meat Science, 88, 462–467. doi:10.1016/j.meatsci.2011.01.027
- Sun, W., Zhou, F., Sun, D.-W., & Zhao, M. (2013). Effect of oxidation on the emulsifying properties of myofibrillar proteins. Food and Bioprocess Technology, 6(7), 1703–1712. doi:10.1007/s11947-012-0823-8
- Vázquez-Ovando, A., Betancur-Ancona, D., & Chel-Guerrero, L. (2013). Physicochemical and functional properties of a protein-rich fraction produced by dry fractionation of chia seeds (Salvia hispanica L.). CyTA-Journal of Food, 11(1), 75–80. doi:10.1080/19476337.2012.692123
- Viljanen, K., Kivikari, R., & Heinonen, M. (2004). Protein−lipid interactions during liposome oxidation with added anthocyanin and other phenolic compounds. Journal of Agricultural and Food Chemistry, 52, 1104–1111. doi:10.1021/jf034785e
- Wu, W., Hua, Y., Lin, Q., & Xiao, H. (2011). Effects of oxidative modification on thermal aggregation and gel properties of soy protein by peroxyl radicals. International Journal of Food Science and Technology, 46, 1891–1897. doi:10.1111/j.1365-2621.2011.02698.x
- Wu, W., Wu, X., & Hua, Y. (2010). Structural modification of soy protein by the lipid peroxidation product acrolein. LWT-Food Science and Technology, 43, 133–140. doi:10.1016/j.lwt.2009.05.006
- Wu, W., Zhang, C., Kong, X., & Hua, Y. (2009). Oxidative modification of soy protein by peroxyl radicals. Food Chemistry, 116, 295–301. doi:10.1016/j.foodchem.2009.02.049
- Ye, L., Liao, Y., Zhao, M., & Sun, W. (2013). Effect of protein oxidation on the conformational properties of peanut protein isolate. Journal of Chemistry, 2013, 1–6.
- Yuan, B., Ren, J., Zhao, M., Luo, D., & Gu, L. (2012). Effects of limited enzymatic hydrolysis with pepsin and high-pressure homogenization on the functional properties of soybean protein isolate. LWT-Food Science and Technology, 46, 453–459. doi:10.1016/j.lwt.2011.12.001