Abstract
Naturally brewed soy sauce was spray-dried using a pilot-scale spray-dryer with two-fluid atomization nozzle. Maltodextrin (DE 10) was incorporated into liquid soy sauce as a drying aid, to form a uniform liquid of 40% w/v. The influence of spray-drying parameters including inlet drying air temperature, feed flow rate, and atomization air flow was examined. The product yield, bulk density, cohesiveness and particle size of the spray-dried powders were found to be influenced by the spray-drying conditions.
Extremely cohesive soy sauce powders were obtained when the air-drying temperature reached 180°C and above. Moreover, surface NaCl concentration of the soy sauce powders was shown to have insufficient expression in comparison with that in bulk. Surface NaCl content was also found to increase with increasing drying temperature.
La salsa de soja elaborada de forma natural fue desecada mediante la utilización de un pulverizador de secado a escala piloto con una boquilla de atomización de dos fluidos. Se incorporó maltodextrina de DE 10 en la salsa de soja líquida como agente secador auxiliar, para formar un líquido uniforme de 40% (w/v). Se examinaron la influencia de los parámetros de secado por pulverización, además de la temperatura del aire de secado de entrada, el nivel del flujo de alimentación y el flujo de aire de atomización. Se encontró que el rendimiento del producto, la densidad aparente, la cohesión y el tamaño de las partículas de los polvos desecados por pulverización fueron influenciados por las condiciones de secado por pulverización.
Se obtuvieron unas salsas de soja en polvo extremadamente cohesivas cuando la temperatura del aire de secado llegó a 180°C y por encima. Además, la concentración de NaCl en la superficie de las salsas de soja en polvo mostró tener una expresión insuficiente, en comparación con la concentración de NaCl aparente. También se encontró que el contenido de sal en la superficie se incrementó con el aumento de temperatura del aire de secado.
1. Introduction
Commonly produced and consumed in East Asia, soy sauce is a traditional condiment usually used in liquid form. Soy sauce powder as a new type of soy sauce product was reported in the literature as early as the 1970s (Hamano & Sugimoto, Citation1978). The characteristics of spray-dried soy sauce powders were recently intensively investigated by our group and those produced by different carrier agents were characterized in regard to anti-caking (Wang, Jiang, & Zhou, Citation2013; Wang & Zhou, Citation2012, Citation2013, Citation2015). However, as a foundation for industrial scaling-up, the spray-drying conditions used for soy sauce powder production have rarely been investigated or published in the literature.
Spray-drying transforms feed from a liquid or slurry form to a dry powder (Goula & Adamopoulos, Citation2008a). Continuous operations, such as spray-drying, are preferred over batch processes because of their better efficiency and economy. However, protein hydrolysate foods including soy sauce are generally difficult to spray-dry because they stick on the wall of the dryer or form unwanted agglomerates in the dryer chamber and conveying system, resulting in a lower product yield and operating problems. The sticky behavior of sugar-rich foods is attributed to low-molecular sugars, and the problem of wall deposition can be reduced by the addition of drying aids or the use of dehumidified air (Adhikari, Howes, Wood, & Bhandari, Citation2009). Due to the high stickiness of soy sauce powders, their product yield is always a matter of concern as it is with the spray-drying of other sticky products (Bhandari, Datta, Crooks, Howes, & Rigby, Citation1997; Goula & Adamopoulos, Citation2008a, Citation2008b; Telang &Thorat, Citation2010). Although other types of carrier agent have been studied in regard to soy sauce powder production, maltodextrin remains a popular drying carrier due to its low cost and good reconstitution properties.
In addition to product yield, optimizing process variables to achieve required powder properties is another consideration in the spray-drying of soy sauce. The physicochemical properties of powders produced by spray-drying depend on process variables such as air-drying temperature and flow rate, feed flow rate, and atomization force. Therefore, it is important to study the influence of drying process conditions on the properties of soy sauce powders in order to achieve high process yield and desirable properties during handling, storage, and applications. The objective of this study was to determine the effects of drying temperature and air and atomization flow rates on the properties of spray-dried soy sauce with maltodextrin as carrier. The characterized properties included bulk density, particle size, and flow behavior. Besides, soy sauce as a solution contains skin-forming materials like carbohydrates for dry powders and it has high NaCl as well. According to Walton and Mumford (Citation1999), diffusive migration of NaCl to the powder surface can be controlled by selecting the appropriate air-drying temperature. As a study on the impact of spray-drying temperature, the morphology and surface chemical composition of soy sauce powders were also examined in this study.
2. Materials and methods
2.1. Raw materials
Naturally brewed soy sauce was obtained from Kikkoman Pte. Ltd. (Singapore), with 10.3% protein, 8.1% carbohydrate, and 16.5% NaCl on a wet basis, giving a total solids content of 35%. Maltrin® M100 Maltodextrin was obtained from SUNTOP Pte. Ltd. (Singapore), with a DE value of 10.
2.2. Spray-drying and experimental design
For each sample, 400 g of maltodextrin were dissolved in 1 L of soy sauce to form a viscous fluid of 75% total solids. This solution was subsequently used as the feed for spray-drying. A pilot-scale spray-dryer (Mobile MinorTM, GEA, China) was used in the study. The dimensions of the drying chamber were 0.62 m cylindrical height, 0.80 m diameter, and 60° conical base. The feed solutions were fed to a two-fluid nozzle at the top of the spray-dryer using a peristaltic pump (Masterflex, USA). The spray-dryer was operated in a co-current air flow mode. The variables studied were: air temperature at 140°C, 160°C, 180°C, and 200°C, atomization air flow rate at 3, 4, 5, and 6 m3/h, and feed flow rate at 19, 27, 35, and 43 g/min. Dried powders were collected from the base of the cyclone separator of the dryer. All samples were produced in triplicate.
2.3. Product yield
The product yield was calculated as the ratio of the amount of powder collected after every spray-drying experiment to the initial amount of solids in the feed solution.
2.4. Moisture content analysis
About 1–2 g of each spray-dried sample were weighed and placed in an oven at 100°C for 24 h. The moisture content was measured in triplicate and expressed as percentage wet basis (i.e. 100 × g water/g wet material).
2.5. Bulk density
Bulk density was determined by gently pouring soy sauce powder into a 10 cm3 graduated cylinder, and was calculated as the ratio of the weight (g) of the sample contained in the cylinder to the volume occupied (i.e. 10 cm3) (Gallo, Llabot, Allemandi, Bucalá, & Piña, Citation2011).
2.6. Cohesion index
Powder cohesiveness was tested using a Powder Flow Analyzer (Stable Micro System, UK); 140 mL of powder was poured into a cylinder with gentle tapping and placed under the test system. A rotating blade made a downward and then an upward movement for three cycles, corresponding to three compaction and decompaction phases. A force–displacement curve was thus generated by the computer, exhibiting the force exerted on the cylinder bottom due to blade movement and powder displacement. A cohesion coefficient (g mm) was derived by the computer program through integrating the area under the curve during the decompaction cycle. The cohesion index (mm) was defined as the ratio of cohesion coefficient to sample weight. Powder flow behavior was then derived from the cohesion index ().
Table 1. Powder categorization scale based on the cohesion index (modified from Benkovic and Bauman, Citation2009).
Tabla 1. Escala de caracterización de los polvos basada en el índice de cohesión (modificado de Benkovic y Bauman, Citation2009).
2.7. Particle size analysis
Powder particle size distribution was measured in ethanol using a LA-950 V2 Laser Scattering Particle Size Analyzer (Horiba, Japan) at room temperature. The refractive index of ethanol was set at 1.36 and a stationary iteration number of 15 was used.
2.8. Scanning electron microscope–energy-dispersive X-ray spectrometer (SEM-EDS)
Particle surfaces were scanned and their elemental composition determined using a JEOL-JSM-6701 scanning electron microscope (SEM; JEOL, Tokyo, Japan) equipped with an energy-dispersive X-ray spectrometer. The samples were observed under magnification of 1000× by SEM. EDS was performed at an accelerating voltage of 15 kV, a working distance of 15 mm, and an acquisition time of 50 live seconds.
2.9. Statistical analysis
One-way ANOVA was conducted for determination of differences between samples using SPSS 17.0 software. Duncan’s test was employed. A probability level of p < 0.05 was considered to be significant for all statistical procedures.
3. Results and discussion
3.1. Product yield
The product yield varied in a range 37.06%–62.45% (). Reduced product yield was obtained with an increase in inlet temperature from 160°C to 200°C. A higher product yield was reported previously in the literature with a reduction in feed flow rate (Gallo et al., Citation2011; Telang & Thorat, Citation2010). An increase in inlet air temperature was reported to increase product yield, while a further temperature increase caused particle surfaces to heat beyond their glass transition temperature and thus become sticky, reducing product yield (Telang & Thorat, Citation2010). Similar effects were found in our study when different inlet air temperatures and feed flow rates were examined. However, no influence of atomization air flow on product yield was found. Poor product yield would be expected at low atomization pressure, since the reduced drying rate can result in greater wall deposition (Telang & Thorat, Citation2010).
Table 2. Influence of process operating conditions on product yield.
Tabla 2. Influencia de las condiciones de los procesos operativos en el producto final.
3.2. Bulk density
As shown in , the bulk density decreased as the drying inlet air temperature increased. An opposite effect was found when the feed flow rate increased (). Bulk density was reduced when air flow rate was increased to 4 m3/h, but did not change thereafter ().
Figure 1. Bulk density of the soy sauce powder under different drying conditions. (a*: Feed flow rate 27 g/min, atomization air flow rate 4 m3/h; b*: inlet drying temperature 160°C, atomization air flow rate 4 m3/h; c*: feed flow rate 35 g/min, inlet air temperature 160°C.)
Figura 1. Densidad aparente de las salsas de soja en polvo bajo diferentes condiciones de secado (a*: Nivel del flujo de alimentación 27 g/min, flujo de aire de atomización 4 m3/h; b*: Temperatura de secado de entrada 160°C, flujo de aire de atomización 4 m3/h; c*: Nivel del flujo de alimentación 35 g/min, temperatura del aire de entrada 160°C).
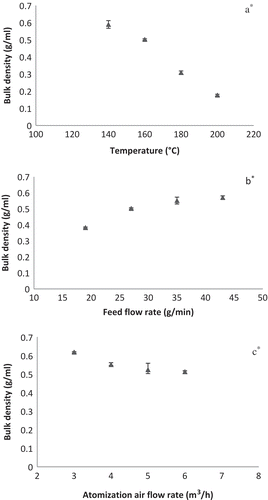
According to Walton (Citation2000), increasing air-drying temperature or decreasing feed flow rate generally resulted in a decrease in bulk density and there was a greater tendency for particles to be hollow. This could have resulted from a higher evaporation rate (Goula & Adamopoulos, Citation2008a) or a lower residual moisture content (Chengini & Ghobadian, Citation2005), which may be the reason why bulk density decreased dramatically as the inlet air-drying temperature increased.
3.3. Particle size distribution
shows the particle size distribution of the powder particles spray-dried under different operating conditions. As shown in , increasing inlet air temperature resulted in increased particle size. Increasing air-drying temperature established particle structure early and prevented shrinkage during drying, resulting in larger particles (Nijdam & Langrish, Citation2006). Decreasing feed flow rate can increase mass transfer rate during spray-drying and was expected to generate enlarged particles (Reiniccius, Citation2001), but the influence of feed flow rate was not unambiguously shown in our study. Increasing feed flow rate can cause collision and subsequent fusion of small drops and thus increased particle size (Al-AshenL, Jumah, Banat, & Hammad, Citation2003).
Figure 2. Particle size distribution of soy sauce powder under different drying conditions. (a*: Feed flow rate 27 g/min, atomization air flow rate 4 m3/h; b*: inlet drying temperature 160°C, atomization air flow rate 4 m3/h; c*: feed flow rate 35 g/min, inlet air temperature 160°C.)
Figura 2. Distribución del tamaño de las partículas de las salsas de soja en polvo bajo diferentes condiciones de secado (a*: Nivel del flujo de alimentación 27 g/min, flujo de aire de atomización 4 m3/h; b*: Temperatura de secado de entrada 160°C, Flujo de aire de atomización 4 m3/h; c*: Nivel del flujo de alimentación 35 g/min, temperatura del aire de entrada 160°C).
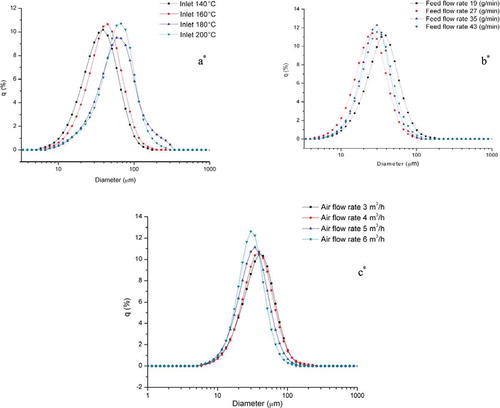
shows the effect of atomization air flow rate on particle size distribution. A higher atomization flow rate produced smaller droplets during the atomization process and thus the size of the powders produced after drying showed a clear correlation with atomization air flow rate. Results were reported on the influence of atomization wheel speed on powder particle size using rotary nozzles by Chegini and Ghobadian (Citation2005) and Pisecky (Citation1978). Using a two-fluid atomization nozzle, similar effects were found in our study.
3.4. Cohesion index
As shown in , spray-dried soy sauce powders showed marked variation in cohesion index. Increasing inlet air temperature resulted in a dramatic increase in the cohesion index. According to the cohesion categorization scale in , extremely cohesive powders were produced when the drying inlet air temperature reached 180°C and above. By adjusting the feed flow rate, a faster drying rate resulted in an increased cohesion index on average. However, statistical analysis indicated that there was no significant difference among flow rates. Atomization air flow rate influences powder cohesiveness by changing particle size. In our study, an increased cohesion index was found when atomization air flow was increased to over 5 m3/h. However, further increase resulted in no significant increase in the cohesion index.
Table 3. Effect of drying conditions on cohesion index and moisture content of soy sauce powder.
Tabla 3. Efecto de las condiciones de secado en el índice de cohesión y porcentaje de humedad de las salsas de soja en polvo.
Powder cohesiveness was reported to be influenced by both particle size and moisture content. Larger particle size indicates that the powder flows more freely and is less cohesive (Buma, Citation1971; Landillon, Cassan, Morel, & Cuq, Citation2008; Teunou, Fitzpatrick, & Synnott, Citation1999). However, an opposite relationship between particle size and cohesion index was observed in our study: increased inlet air temperature resulted in increased particle size. However, at the same time, a much higher cohesion index was obtained. In addition, the moisture content of the powder ranged between 6.15% and 8.55%, unlikely to have any marked effect on powder cohesiveness.
In addition to moisture content and particle size, powder cohesiveness can also be influenced by many other factors such as surface asperity, surface free energy, hardness and elasticity, and even interstitial air (Chen, Jallo, Quintanilla, & Dave, Citation2010). Therefore, the parameter of cohesiveness is difficult to model and control. For example, an increase in drying air temperature resulted in reduced particle density and reduced crust thickness (Walton, Citation2000). This altered the hardness and elasticity of the particles and rendered them more cohesive through interparticle plastic deformation during a flow test (Medhe, Pitchumani, & Tomas, 2005)
3.5. Powder morphology
shows SEM micrographs of soy sauce powders produced under different inlet air temperatures. All spray-dried soy sauce powder particles were spherical with significant heterogeneity in size. This is in agreement with the results of particle size analysis described in Section 3.3. Powders dried under higher inlet air temperatures ( and ) had a relatively smoother surface than those dried under lower temperatures ( and ). During spray-drying, a higher drying air temperature can help droplets rapidly develop a dry, hard skin, preventing subsequent deflation and shrinkage. Morphological changes with respect to air-drying temperature were reported in the literature for spray-dried dairy powders (Nijdam & Langrish, Citation2006; Walton & Mumford, Citation1999).
Figure 3. SEM micrographs of soy sauce powder produced using different inlet air temperatures. (a: 200°C, b: 180°C, c: 160°C, d: 140°C, feed flow rate 27 g/min, and atomization air flow rate 4 m3/h.)
Figura 3. Micrografías SEM de las salsas de soja en polvo producidas utilizando diferentes temperaturas de aire de entrada (a: 200°C, b: 180°C, c: 160°C, d: 140°C,nivel del flujo de alimentación 27 g/min, aire de atomización 4 m3/h).
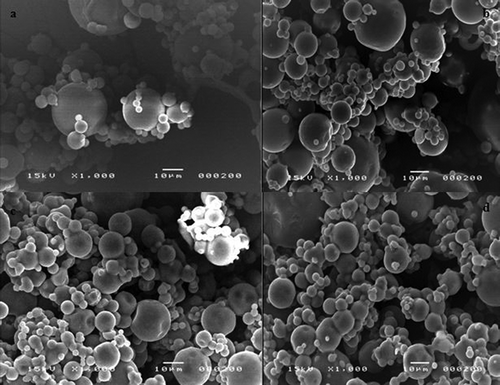
Micrographs of soy sauce powders spray-dried with variation in the feed flow rate are shown in . A relatively smoother surface was noted for powder particles dried under a reduced feed flow rate, which resulted from the increased drying rate. Atomization air flow was affected droplet size during atomization. In agreement with the findings from the particle size analyzer shown in , SEM micrographs verified that increasing atomization air flow resulted in decreased particle size for spray-dried soy sauce powder ().
Figure 4. SEM micrographs of soy sauce powder produced using different feed flow rates. (a: 19 g/min, b: 27 g/min, c: 35 g/min, d: 43 g/min; inlet air temperature 160°C, atomization air flow rate 4 m3/h.)
Figura 4. Micrografías SEM de las salsas de soja en polvo producidas utilizando diferentes niveles del flujo de alimentación (a: 19 g/min, b: 27 g/min, c: 35 g/min, d: 43 g/min, temperatura del aire de entrada 160°C, aire de atomización 4 m3/h).
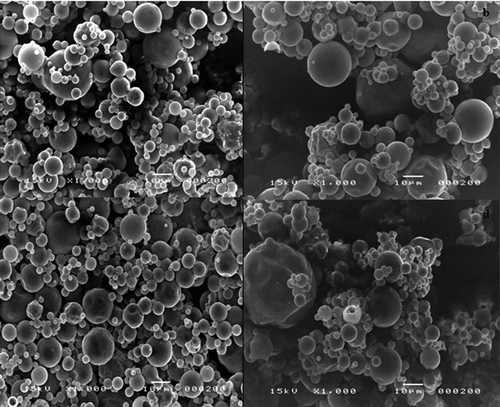
Figure 5. SEM micrograph of soy sauce powder produced using different atomization air flow rates. (a: 3 m3/h, b: 4 m3/h, c: 5 m3/h, d: 6 m3/h; inlet air temperature 160°C, feed flow rate 35 g/min.)
Figura 5. Micrografía SEM de las salsas de soja en polvo producidas utilizando diferentes flujos de aire de atomización (a: 3 m3/h, b: 4 m3/h, c: 5 m3/h, d: 6 m3/h, temperatura del aire de entrada 160°C, nivel del flujo de alimentación 35 g/min).
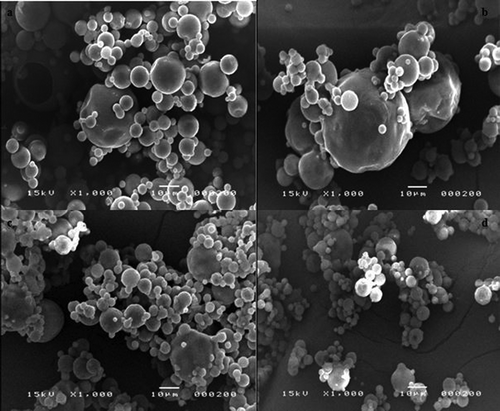
Besides, no significant amount of NaCl was found on the surface of the powder particles from SEM analysis results. After spray-drying, the salt particles were present as tiny, fine particles of irregular shape typical for crystal materials. They were reported to be distributed mainly on the inner surface of spray-dried powders formulated with NaCl (Chindapan, Devahastin, & Chiewchan, Citation2010; Walton & Mumford, Citation1999). According to Kim, Chen, and Pearce (Citation2003), during spray-drying there will be water diffusion towards the surface and solutes to the inner layer of droplets driven by concentration gradients. Components of lower molecular size will have much higher diffusivity and tend to be distributed on the inner layers of powders after spray-drying. In our study, the high diffusivity of NaCl as determined by its small molecular size might have resulted in its insufficient expression on the outermost surface of the powder particles, as shown by the SEM micrographs.
3.6. EDS analysis for elemental concentration
Soy sauce powders spray-dried under different inlet air temperatures were analyzed by EDS for surface atomic composition. EDS is not purely a surface analysis – it detects the elemental composition of bulk material in the near surface layer for about 1 µm (Cocke, Mollah, Parga, Hess, Ortega, Citation1992). As shown in , the crusts of the spray-dried soy sauce powders were composed mainly of carbohydrates and NaCl, although NaCl is not easily identifiable by SEM. Spray-drying temperature influences the subsurface NaCl concentration of particles. When air-drying temperature was increased from 140°C to 200°C, the mass of Na+ and Cl− together increased from 17.15 ± 1.48% to 22.65 ± 2.14%, which is close to the bulk NaCl concentration of 22.0% calculated by mass balance in the spray-drying process. Walton and Mumford (Citation1999) observed that NaCl distribution on the powder surface increased with air-drying temperature when drying a solution composed of NaCl and skimmed milk. The mechanism for increased NaCl distribution on the surface is still not clear, but one possible explanation could be rapid crust formation on the droplet surface with increased drying temperature, which could effectively reduce the diffusive migration of NaCl solute from the droplet surface to its inner layer. As a result, more NaCl crystals were present on the powder surface or within 1 µm of the surface layer after spray-drying.
Figure 6. Atomic mass of the crust of soy sauce powder under different drying inlet air temperatures. (C, carbon atoms; O, oxygen atoms; Na + Cl, sodium and chlorine atoms in total.)
Figura 6. Masa atómica de la corteza de las salsas de soja en polvo con diferentes temperaturas de aire de secado de entrada (C: átomos de carbono; O: átomos de oxígeno Na+Cl: átomos de sodio y cloro en total).
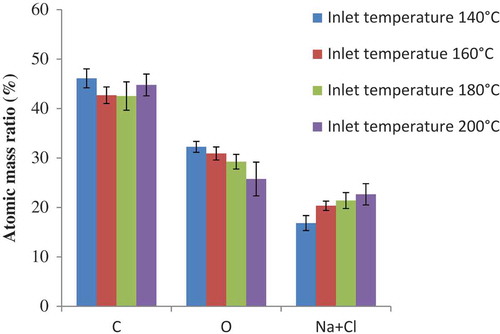
The finding of uneven distribution of salt on the powder surface might be of value in producing soy sauce powders with a stronger salty taste. Increased surface salt expression at higher drying temperatures enhances the likelihood of NaCl binding with the corresponding receptors on the taste buds of the tongue, thereby increasing the product’s salty taste and reducing the actual salt content required.
4. Conclusion
Extremely cohesive soy sauce powder was obtained when the air-drying temperature reached 180°C and above. This may have been due to elastic deformation of the flowing particles. Although salt crystals did not tend to be distributed on the outermost particle surface a significant presence was detected on the particle subsurface by EDS analysis. The impact of increased spray-drying temperature on surface salt expression was found to yield a powder with increased surface salt content.
Disclosure statement
No potential conflict of interest was reported by the authors.
Additional information
Funding
References
- Adhikari, B., Howes, T., Wood, B. J., & Bhandari, B. R. (2009). The effect of low molecular weight surfactants and proteins on surface stickiness of sucrose during powder formation through spray drying. Journal of Food Engineering, 94(2), 135–143. doi:10.1016/j.jfoodeng.2009.01.022
- Al-AshenL, S., Jumah, R., Banat, F., & Hammad, S. (2003). The use of experimental factorial design for analysing the effect of spray-dryer operating variables on the production of tomato powder. Transaction Institute Chemical Engineering, 81(2), 81–88. doi:10.1205/096030803322088215
- Benkovic, M., & Bauman, I. (2009). Flow properties of commercial infant formula powders. World Academy of Science, Engineering and Technology, 54, 495–499.
- Bhandari, B., Datta, N., Crooks, R., Howes, T., & Rigby, S. (1997). A semi-empirical approach to optimise the quantity of drying aids required to spray dry sugar-rich foods. Drying Technology, 15(10), 2509–2525. doi:10.1080/07373939708917373
- Buma, T. J. (1971). Free fat in spray-dried whole milk. 5. Cohesion. Determination, influence of particle size, moisture content and free-fat content. Netherlands Milk and Dairy Journal, 25, 107–122.
- Chegini, G. R., & Ghobadian, B. (2005). Effect of spray drying conditions on physical properties of orange juice powder. Drying Technology, 23, 657–668. doi:10.1081/DRT-200054161
- Chen, Y., Jallo, L., Quintanilla, M. A. S., & Dave, R. (2010). Characterization of particle and bulk level cohesion reduction of surface modified fine aluminum powders. Colloids and Surfaces A: Physicochemical and Engineering Aspects, 361(1–3), 66–80. doi:10.1016/j.colsurfa.2010.03.015
- Chindapan, N., Devahastin, S., & Chiewchan, N. (2010). Effect of electrodialysis pretreatment on physicochemical properties and morphology of spray-dried-fish sauce powder. Journal of Food Engineering, 99(1), 31–39. doi:10.1016/j.jfoodeng.2010.01.027
- Cocke, D. L., Mollah, M. Y., Parga, J. R., Hess, T. R., & Ortego, J. D. (1992). An XPS and SEM/EDS characterization of leaching effects on lead- and zinc-doped Portland cement. Journal of Hazardous Materials, 30(1), 83–95. doi:10.1016/0304-3894(92)87076-R
- Gallo, L., Llabot, J. M., Allemandi, D., Bucalá, V., & Piña, J. (2011). Influence of spray-drying operating conditions on Rhamnus purshiana (Cáscara sagrada) extract powder physical properties. Powder Technology, 208, 205–214. doi:10.1016/j.powtec.2010.12.021
- Goula, A. M., & Adamopoulos, K. G. (2008a). Effect of maltodextrin addition during spray drying of tomato pulp in dehumidified air: I. Drying kinetics and product recovery. Drying Technology, 26(6), 714–725. doi:10.1080/07373930802046369
- Goula, A. M., & Adamopoulos, K. G. (2008b). Effect of maltodextrin addition during spray drying of tomato pulp in dehumidified air: II. Powder properties. Drying Technology, 26, 726–737. doi:10.1080/07373930802046377
- Hamano, M., & Sugimoto, H. (1978). Water sorption, reduction of caking and improvement of free flowingness of powdered soy sauce and miso. Journal of Food Processing and Preservation, 2(3), 185–196. doi:10.1111/j.1745-4549.1978.tb00557.x
- Kim, E. H. J., Chen, X. D., & Pearce, D. (2003). On the mechanisms of surface formation and the surface compositions of industrial milk powders. Drying Technology, 21, 265–278. doi:10.1081/DRT-120017747
- Landillon, V., Cassan, D., Morel, M. -H., & Cuq, B. (2008). Flowability, cohesive, and granulation properties of wheat powders. Journal of Food Engineering, 86, 178–193. doi:10.1016/j.jfoodeng.2007.09.022
- Nijdam, J. J., & Langrish, T. A. G. (2006). The effect of surface composition on the functional properties of milk powders. Journal of Food Engineering, 77, 919–925. doi:10.1016/j.jfoodeng.2005.08.020
- Pisecky, J. (1978). Bulk density of milk powders. Dairy Industries International, 2, 4–11.
- Reiniccius, G. A. (2001). Multiple-core encapsulation – the spray drying of food ingredients. In P. Vilstrup (Ed.), Microencapsulation of food ingredients (pp. 151–185). Surrey, BC: Leatherhead Publishing.
- Telang, A. M., & Thorat, B. N. (2010). Optimization of process parameters for spray drying of fermented soy milk. Drying Technology, 28, 1445–1456. doi:10.1080/07373937.2010.482694
- Teunou, E., Fitzpatrick, J. J., & Synnott, E. C. (1999). Characterisation of food powder flowability. Journal of Food Engineering, 39, 31–37. doi:10.1016/S0260-8774(98)00140-X
- Walton, D. E. (2000). The morphology of spray-dried particles. A qualitative view. Drying Technology, 18(9), 1943–1986. doi:10.1080/07373930008917822
- Walton, D. E., & Mumford, C. J. (1999). The morphology of spray-dried particles, the effect of process variables upon the morphology of spray-dried particles. Chemical Engineering Research and Design, 77(5), 442–460. doi:10.1205/026387699526296
- Wang, W., Jiang, Y., & Zhou, W. (2013). Characteristics of soy sauce powders spray-dried using dairy whey proteins and maltodextrins as drying aids. Journal of Food Engineering, 119(4), 724–730. doi:10.1016/j.jfoodeng.2013.06.047
- Wang, W., & Zhou, W. (2012). Characterization of spray-dried soy sauce powders using maltodextrins as carrier. Journal of Food Engineering, 109(3), 399–405. doi:10.1016/j.jfoodeng.2011.11.012
- Wang, W., & Zhou, W. (2013). Water adsorption and glass transition of spray dried soy sauce powders using maltodextrins as carrier. Food and Bioprocess Technology, 6(10), 2791–2799. doi:10.1007/s11947-012-0992-5
- Wang, W., & Zhou, W. (2015). Characterisation of spray dried soy sauce powders made by adding crystalline carbohydrates to drying carrier. Food Chemistry, 168, 417–422. doi:10.1016/j.foodchem.2014.07.065