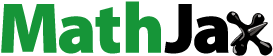
ABSTRACT
Proteolysis is specific for each type of cheese and might be affected by heat treatment. Studies have shown the ability of cheeses to produce bioactive peptides with antioxidant or anti-hypertension bioactivities, which have been related to ripening time or activity of starter cultures, however, little is known about their production in fresh cheeses. Our objective was to evaluate antioxidant and angiotensin-converting enzyme inhibitory (ACEI) activities in fresh goat cheeses elaborated without starters using pasteurized and raw milk from different seasons. Proteolysis was measured by acid-soluble nitrogen, non-protein nitrogen and ethanol-soluble nitrogen indexes, using the Kjeldahl method and reverse phase high performance liquid chromatography (RP-HPLC). Each fraction’s antioxidant and ACEI activities were measured. Analysed cheeses showed high biological activities and slight differences between them were associated with heat treatment. Our results suggested that fresh goat cheese had important biological activity due to peptides present originally in the milk or released by rennet action during cheese manufacture.
RESUMEN
La proteólisis es específica para cada tipo de queso y puede ser afectada por el tratamiento térmico. Durante ésta, pueden liberarse péptidos con actividad antioxidante o antihipertensiva asociados a la maduración o al uso de cultivos iniciadores. Poco se conoce acerca de su producción en quesos frescos. Nuestro objetivo fue evaluar las actividades antioxidantes y de inhibición de la enzima convertidora de angiotensina (ACEI) en quesos frescos de cabra. Se analizaron quesos elaborados sin cultivos iniciadores utilizando leche pasteurizada y cruda de diferentes estaciones. La proteólisis fue evaluada por índices de nitrógeno utilizando el método de Kjeldahl y por RP-HPLC. Las actividades antioxidante y ACEI para cada fracción nitrogenada fueron evaluadas. Se observaron altas actividades biológicas con ligeras diferencias asociadas al tratamiento térmico. Nuestros resultados sugieren que el queso fresco de cabra posee importante actividad biológica debido a los péptidos presentes originalmente en la leche o liberados por acción del cuajo.
Introduction
Bioactive peptides are defined as specific protein fragments that have a positive impact on body functions or conditions and could ultimately influence health (Kitts & Weiler, Citation2003). Their functionality is due to its amino acid composition and sequence, which generally consists of 3–20 amino acid residues per molecule (Hajirostamloo, Citation2010). Depending on the amino acids sequence, these peptides can exhibit one or diverse activities, including immunomodulatory, antimicrobial, antioxidant, antithrombotic, hypocholesterolemic and antihypertensive activity (Korhonen & Pihlanto, Citation2006)
Cheese contains numerous bioactive peptides released during proteolysis occurred in fermentation, ripening or even during digestion (Gupta, Mann, Kumar, & Sangwan, Citation2009, Citation2013; Hernández-Ledesma, Del Mar Contreras, & Recio, Citation2011; Théolier, Hammami, Fliss, & Jean, Citation2014).
The proteolysis process is different depending on the type of cheese due to differences in manufacturing practices, ripening time, etc., resulting in a unique peptide profile characteristic of each variety of cheese. However, in general, proteolysis can be summarized as follows: initial hydrolysis of caseins catalysed primarily by residual coagulant, and to a lesser extent by plasmin and perhaps cathepsin D and other somatic cell proteinases, resulting in the formation of large and intermediate-sized peptides, which are subsequently degraded by the coagulant and enzymes from the starter and nonstarter flora of the cheese. The secondary proteolysis (in ripened cheeses) generates small peptides and free amino acids resulting from the action of bacterial proteinases and peptidases (Fox & McSweeney, Citation1996). Some of these peptides exhibit biological activities as antioxidants (Ahmed, El-Bassiony, Elmalt, & Ibrahim, Citation2015; Abadia-Garcia et al., Citation2013; Timón, Parra, Otte, Broncano, & Petrón, Citation2014), and anti-hypertensive (Gupta et al., Citation2013; Ong, Henriksson, & Shah, Citation2007; Torres-Llanez, González-Córdova, Hernandez-Mendoza, Garcia, & Vallejo-Cordoba, Citation2011) between others.
Antioxidant peptides derived from milk are composed of 5–11 amino acids, including in the sequence hydrophobic amino acids, proline, histidine, tyrosine or tryptophan. Antioxidant activity of these peptides seems to be inherent to protease specificity (Pihlanto, Citation2006).
On the other hand, anti-hypertensive activity of peptides is given through the inhibition of angiotensin-converting enzyme (ACE), preventing the conversion of angiotensin I to angiotensin II, which induces the release of aldosterone, increasing sodium concentration and blood pressure; and inactivating the vasodilator bradykinin, protecting the body against cardiovascular disease (Hernández-Ledesma, Amigo, Ramos, & Recio, Citation2004; Jäkälä & Vapaatalo, Citation2010). ACE inhibitory peptides are generally short chain peptides, often carrying polar amino acid residues, such as proline (Espejo-Carpio, De Gobba, Guadix, Guadix, & Otte, Citation2013; Meisel, Citation2004).
Bioactivity of peptides might be susceptible to structural and chemical modifications of proteins during food processing, such as heat treatment (Hayaloglu & Brechany, Citation2007; Korhonen & Pihlanto, Citation2003) and by geoclimatic conditions, such as environmental temperature and wheatear (Lacroix, Verret, & Paquin, Citation1996; Ng-Kwai-Hang, Hayes, Moxley, & Monardes, Citation1984).
In recent decades, the interest of food as agents for prevention or cure in chronic diseases had increased. Although many studies have been conducted for the determination of peptides biological activity generated during cheeses ripening, little work has been done on the production of theses peptides in fresh cheeses. Since fresh cheeses represent a high market especially in the Latin American countries, identifying antioxidant and ACE inhibitory (ACEI) activity in these types of cheeses could provide a potential additional value for consumers because of its health benefits. Consequently, this study aimed to evaluate the production of bioactive peptides in experimental fresh goat cheeses prepared without starters, evaluating at the same time, the impact of milk pasteurization.
Materials and methods
Cheese manufacture
Goat milk was provided by artisanal producers from the region of Coatepec, Veracruz, Mexico (at 1200 m above sea level). From a herd of 28 goats, 10 healthy animals were selected according to their lactation period. From the whole morning milking batch (without colostrum), 4 l samples were taken and transported immediately in order to make cheeses. Sampling was carried out in three different periods of the year, November, January and March (N, J and M, respectively), 2012–2013. Thus, a total of 12 l of milk were used to cheese manufacture. The selected period of sampling had medium values for higher temperatures (~22–27°C), lower temperatures (~9–12°C), and total precipitation (~55–90 mm) of the year, that let us control the goats feeding and living conditions. Milk samples (4 l for period of the year) were split in two, 2 l were kept raw and 2 l were pasteurized at 63°C for 30 min. Cheeses were made with the two kinds of milks to obtain, on average, eight raw (NP) and eight pasteurized cheeses (P) for each one of the three milking periods. Thus, a total of 48 cheeses were produced.
Experimental mini cheese production was performed at the Universidad Veracruzana according to the method reported by Scholz (Citation1997) with slight modifications. Milk was heated to achieve 30–33°C followed by the addition of 10 ml/l rennet (Coagulmex, strength 1:10,000, Grupo Maphsa, Xalapa, Mexico). Coagulation took place at 38 ± 1°C for 50 min. Curd was cut into 1 cm3 cubes and whey separation was held for 20–40 min. Afterward, cheeses were moulded in plastic moulds (119 mm × 64 mm × 34 mm) allowing natural whey drainage by gravity at 4°C during 24 h. Cheeses of 9.9−16.5 g were obtained and stored at 4°C. After 6 days of storage, 4 raw and 4 pasteurized fresh cheeses of each milking period (November, January and March), in a total amount of 12 raw and 12 pasteurized cheeses, were randomly selected to be transported and analysed in the Tecnológico de Monterrey facilities.
Proteolysis assessment
Samples were separated in raw and pasteurized cheeses. Raw cheeses from the same season were mixed to obtain three samples of raw cheeses corresponding to different periods of milking (November, January and March). Same was made for pasteurized cheeses. Finally, a total of six samples of raw and pasteurized fresh goat cheeses produced in Veracruz at different periods of the year were analysed. Proteolysis of each sample was measured by following different nitrogenous fractions characteristics. For that, 20 g of each sample were homogenized with 20 ml of distilled water 5 min at 15,500 rpm using an Ultraturrax IKA T18 Basic (IKA instruments, Germany). Then, samples were incubated for 1 h in a water bath at 40°C and centrifuged (Hermle, Z 383 K Wehingen, Germany) at 4°C for 50 min and 4000 × g. The fat upper layer was removed and samples were re-suspended to obtain a non-fat cheese homogenate (NFCH). An aliquot of NFCH was used to quantify the total nitrogen with Kjeldahl method. The sample was kept at −80°C until fractionation.
Crude nitrogenous fractions: acid soluble nitrogen (ASN) at pH 4.6, non-protein nitrogen (NPN) and 70% ethanol soluble nitrogen (EtOH-SN) were prepared, as described by Guerra-Martínez, Montejano, and Martín-del-Campo (Citation2012). NFCH was diluted with a solution of NaCl (9 g/l) to achieve a final concentration of 0.1-g cheese m/l (CMS).
For NPN, 20 ml of CMS were mixed with 20 ml of trichloroacetic acid (240 g/l) and incubated 1 h at 25°C. The soluble fraction was separated by centrifugation 10 min at 7°C and 4000 × g. The supernatants were stored at −80°C until nitrogen content analyses.
For the determination of ASN, 10 ml of the 0.1 g/ml of CMS were adjusted to pH 4.6 with HCl 1 M and incubated in a water bath for 20 min at 25°C. The soluble fraction was separated by centrifugation for 45 min at 7°C and 4000 × g. The supernatants were stored at −80°C until nitrogen content analyses.
EtOH-SN was prepared by adding ethanol (KARAL, Leon, Gto, Mexico) to 2 ml of ASN to achieve a 700 ml/l EtOH final concentration, and incubated in a water bath 24 h at 25°C, the soluble fraction was separated by centrifugation for 10 min at 6°C and 4000 × g. The precipitates, corresponding to the high-molecular weight peptides (EtOH-NSN) and the supernatant (EtOH-SN), were stored at −80°C until nitrogen content analyses.
Nitrogen content in all the obtained fractions was evaluated with Kjeldahl method according to the AOAC. Proteolysis index for each nitrogenous fraction was obtained by dividing the respective nitrogen content over total nitrogen content. All analyses were performed in duplicate.
Determination of biological activity
Antioxidant activity evaluation
The method described by Olvera-García, Cardador-Martínez, and Martín Del Campo (Citation2015) was used to assess the 2,2- diphenyl-1-picrylhydrazyl (DPPH) radical scavenging activity of ASN and NPN fractions. Briefly, 0.02 ml of each fraction (ASN and NPN) were added to a 96-well flat-bottom plates containing 0.22 ml of DPPH• solution (125-µM DPPH• in 800 ml/l methanol). Samples were prepared in triplicate. The plate was covered, left in the dark at room temperature 90 min before reading in a visible–UV microplate reader (X Mark Microplate Reader, Bio-Rad Laboratories, Inc. Japan) using a 520-nm filter. Resulting data are expressed as a percentage of DPPH•·discoloration.
Determination in vitro of ACEI activity
ACEI activity was determined by the reverse phase high performance liquid chromatography (RP-HPLC) method of Wang et al. (Citation2013) based on the liberation of hippuric acid (HA) from hippuryl-histidyl-leucine (HHL) catalysed by ACE.
Briefly, HHL and ACE (from porcine kidney, 0.5 U) were dissolved in 100-mM borate buffer (pH 8.3) supplemented with 300-mM NaCl at a concentration of 3 mM and 0.1 U/ml, respectively. All reagents came from Sigma–Aldrich, St. Louis, MO, USA.
Aliquots of 25 µl of the EtOH-SN and EtOH-NSN fractions, were pre-incubated with 25 µl of ACE at 37°C for 10 min, and then 25 µl of HHL were added and incubated at 30°C for 30 min. Reaction was stopped by adding 83.5 µl of HCl 0.1 M.
Separations were conducted by RP-HPLC using an Agilent Technologies 1200 series (Palo Alto, CA, USA) coupled with a diode array detector (DAD), at 25°C using a flow rate of 0.5 ml/min in a column Zorbax Eclipse XDB-C18 (5 µm, 4.6 µm i.d. × 150 mm, Agilent, USA). Injected volume was 5 µl. Solvents were as follows: (A) 0.5 ml/l TFA in HPLC-grade water. (B) Acetonitrile. The ratio of solvents A/B was 7:3. Detection was set at 226 nm in the DAD and data were acquired with Chemstation software. HA and HHL were used as reference standards to identify the peaks in the chromatogram.
The extent of ACE inhibition in the samples was calculated by Equation (1):
where
A blank of HHL, treated as previously specified but with buffer instead of sample and without ACE (thus with the maximum HLL peak), was used as equivalent of 100% ACEI activity.
Nitrogenous fractions analysis by reverse phase HPLC
Nitrogenous fractions ASN, NPN, EtOH-SN and EtOH-NSN were analysed by RP-HPLC. ASN and NPN were analysed directly. For EtOH-SN, 2 ml were concentrated until dryness in a speed-vac (Thermo, Asheville, USA) and then solubilized in 1 ml of 700 ml/l ethanol. EtOH-NSN precipitated was solubilized in 0.5 ml of 50 µM of Tris–HCl (Sigma–Aldrich, St Louis, MO, USA) and 1 µM of EDTA (Sigma–Aldrich, St Louis, MO, USA). All samples were filtered through a 0.45-µm filter (PTFE Syringe Filter, Agilent Technologies, Germany) before injection.
RP-HPLC was performed using an Agilent Technologies 1200 series (Palo Alto, CA, USA) coupled with a DAD. Separations were conducted at 25°C using a flow rate of 0.75 ml/min in a column Zorbax Eclipse XDB-C18 (5 µm, 4.6 µm i.d. × 150 mm, Agilent, USA).
RP-HPLC system and the column used were previously described. Separations were conducted at 25°C using a flow rate of 0.75 ml/min. Solvents used were: (A) 100 ml/l acetonitrile and 0.5 ml/l TFA in HPLC-grade water and (B) 600 ml/l acetonitrile and 0.5 ml/l TFA in HPLC-grade water. Samples were eluted initially with 100% A for 10 min, then with a linear gradient from 0% to 49% B over 98 min and 50% to 80% B until 108 min, followed by a linear gradient from 80% to 100% B over 5 min and maintained at 100% B for 5 min. Volume injected was 10 µl. The DAD was set at 215 nm. Data acquisition was performed with Chemstation software (B.04.03, Agilent, USA). From the obtained chromatograms, all the peaks were integrated and codified according to their retention time for data analysis.
The chromatograms were analysed following the same criteria of Gonzalez De Llano, Polo, and Ramos (Citation1995) who separated the peaks integrated area in two groups, hydrophilic (HI) and hydrophobic (HO) peptides and evaluated its ratio (HO/HI). Considering that most of the free AA (except Phe and Trp) eluted in the first 10 min, the HI portion consisted in those peaks between 10 and 35 min, and the group of HO were those from 35 to 120 min. The HO/HI ratio was obtained by dividing the total area of the peaks in the HO portion by the total area of the peaks in the HI portion of the chromatogram.
Statistical analysis
All statistical analyses were performed by using the Statistica software v 12 (Statsoft, Inc., Tulsa, OK, USA). Data were grouped in sets as follows: (a) nitrogenous indexes, (b) antioxidant activity (AA) in the ASN and NPN fractions, (c) ACEI activity in EtOH-SN and EtOH-NSN, (d) ASN peptides peaks area, (e) NPN peptides peaks area, (f) EtOH-SN peptides peaks area, (g) EtOH-NSN peptides peaks area and (h) each nitrogenous fraction of HI and HO peptides.
For each fraction’s peptide profile, peaks were coded according to the fraction and retention time, and percentage of individual area recorded. Then for the global analysis of HO, HI peptides and HO/HI ratio, the total of peak areas of each group were used directly as variables.
All the data sets mentioned above were subject to analysis of variance (ANOVA) to evaluate if there were significant differences (p < 0.05) between cheeses. In addition, for biological activities, the fraction was also considered as a factor. After, the Fisher least square difference (LSD) test was conducted for all the variables that showed differences between cheeses. In addition, principal components analysis (PCA) was applied to (b) and (c) data sets together as well as for (h) data set (mentioned earlier).
Results and discussion
Proteolysis assessment
Total nitrogen and crude nitrogenous fractions (ASN, NPN and EtOH-SN) were used to measure the primary proteolysis in fresh goat cheeses. The mean values obtained were as follows: total nitrogen, 20.5 ± 9.07 g/kg dry matter; ASN, 0.28 ± 0.22; NPN, 0.20 ± 0.24 and EtOH-SN, 0.12 ± 0.08. These variations observed in nitrogen index were given by a combination of season and heat treatment, and can be attributed to differences in the milk from the different batches. However, ANOVA showed no significant differences between seasons or process for any of the evaluated index (p > 0.05). These results suggest that tested fresh goat cheeses were not different among them and, therefore, could be considered as a homogenous batch.
Low NPN values could be explained by the lack of starter culture in our cheeses, which are considered the main agents for NPN production (Fox & McSweeney, Citation1996). The ASN values obtained were characteristic of fresh cheese because according to El Galiou, Zantar, Bakkali, and Laglaoui (Citation2013), ASN reflects the extent of proteolysis, which increases during ripening.
McSweeney, Fox, Lucey, Jordan, and Cogan (Citation1993) found no significant differences in primary proteolysis between fresh cheeses made with raw milk or pasteurized milk, instead, they found that these differences in proteolysis appear and increase as ripening progressed. However, to our knowledge, no works have been done about the primary proteolysis in fresh cheeses; all the studies of proteolysis and the release of bioactive peptides have focused on maturated cheeses. Furthermore, the few works about fresh cheeses in this field have been done on cheeses prepared with starter cultures (Abadia-Garcia et al., Citation2013; Guerra-Martínez et al., Citation2012; Sánchez-Macías et al., Citation2011; Torres-Llanez et al., Citation2011), thus no comparison could be made with our results.
Biological activity of nitrogenous fractions
Antioxidant activity measure
NPN and ASN fractions were chosen to determinate the antioxidant activity, since they represent the medium and short chain peptide content together. NPN and ASN fractions of fresh goat cheese exhibited an important antioxidant activity. Significant differences were observed between fractions, where NPN presented a significant higher antioxidant activity (62.89% discoloration) compared to the ASN fraction (22.11%). No significant differences in antioxidant activity were found between cheeses in any of the evaluated fractions (p > 0.05).
It has been reported that this activity in cheeses depends mainly on ripening time (Gupta et al., Citation2009), however, our results suggest that the release of antioxidant peptides occurs not only during ripening but also during cheese making.
NPN and ASN differences in antioxidant activity might be related to the type of peptides present in each fraction. However, lower antioxidant activity in ASN fraction could also be due to the features of DPPH radical whose performance is highly dependent on the characteristics of the tested sample that limits its use for hydrophilic antioxidants, as previously observed by Aloğlu & Öner (Citation2011) and Meira et al. (Citation2012). Considering our results, further analyses with different radical scavenging methods are recommended to clarify them.
In vitro ACEI activity
EtOH-SN and EtOH-NSN fractions were chosen to evaluate the ACEI activity, since these fractions contain, respectively, the small- and medium-sized peptides fractioned from ASN. These, according to previous studies are the holders of ACEI activity (Korhonen & Pihlanto, Citation2003).
EtOH-SN and EtOH-NSN fractions showed ACEI activities ranging between 73.03% and 100%. ANOVA showed no significant differences between fractions, or cheeses (p > 0.05). Our results are consistent with those reported by Torres-Llanez et al. (Citation2011) who found values between 95.3% and 99.8% of ACEI activity in water extracts of Mexican fresh cheese. RP-HPLC obtained to quantify ACEI activity is shown in ,).
Figure 1. ACEI activity measurement by RP-HPLC. (a) Sample without ACE inhibitors. (b) EtOH-SN fraction of pasteurized fresh goat cheese. Decrease in HA production was related with ACEI activity. HA: Hippuric acid, HHL: Hippuryl-histidyl-leucine.
Figura 1. Actividad inhibidora de la enzima convertidora de angiotensina (ECA) medida por RP-HPLC. (a) Muestra sin inhibidores de ECA. (b) Fracción EtOH-SN de queso fresco pasteurizado de cabra. La disminución en producción de AH se relacionó con la actividad inhibitoria de la enzima. AH: Ácido hipúrico, HHL: Hipuril-histidil-leucina.
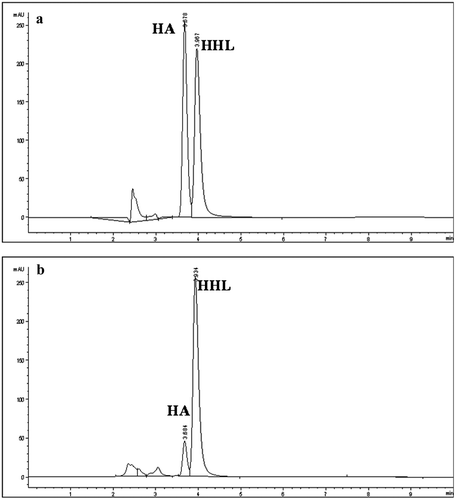
Although many studies have been carried out to measure the biological activity of cheeses with different ripening degrees, only few studies have focused on the evaluation of these activities in fresh cheeses (Paul & Van Hekken, Citation2011; Torres-Llanez et al., Citation2011). However, the few works done focused on this type of cheeses attribute the release of ACEI peptides to the starter cultures (Torres-Llanez et al., Citation2011). Nevertheless our fresh goats cheeses were fabricated without starter culture, we assume that the inhibitory compounds found in our cheeses were either present in the goat milk (Bezerra et al., Citation2013) or generated during cheese making by the action of coagulant (Silva, Pihlanto, & Malcata, Citation2006).
Further PCA was applied to both antioxidant and ACEI activities as a unique data set. The factorial map shown in is defined by PC1 and PC2, which explain 61.85% and 29.99%, respectively, of total variance. PC1 grouped samples according to the heat treatment. Samples from pasteurized cheeses showed positive values while samples from raw cheeses showed negative values.
Figure 2. Antioxidant and ACEI activity. PCA plots of the first two principal components PC1 and PC2. (a) Factorial map. (b) Factor loading plots (PC1 and PC2). N: November, J: January, M: March. NP: Raw, P: Pasteurized.
Figura 2. Actividades antioxidante y ACEI. Gráficas de ACP para los dos primeros componentes principales PC1 y PC2- (a) Mapa factorial. (b) Cargas de los factores (PC1 y PC2). N: Noviembre, J: Enero, M: Marzo. NP: Cruda, P: Pasteurizada.
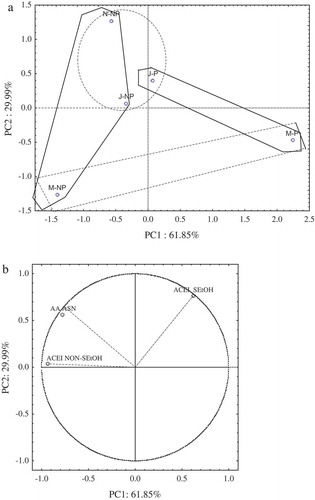
In factor loading plots [)], we observe that only ACEI in the EtOH-NSN fraction shows a strong negative correlation with PC1 (R = −0.93), whereas ACEI in the EtOH-SN fraction shows a positive correlation with PC2 (R = 0.76).
Differences observed between PCA and ANOVA could be attributed to the fact that PCA evaluates a global response and not individual factors.
Thus, our results reveal that tested fresh goat cheeses exerted important ACEI and antioxidant activity. This is in accordance with previous works of Silva et al. (Citation2006), where authors observed that water soluble extracts of 45 days raw and sterilized goat cheeses made with plant coagulant exhibited both biological activities. However, authors found higher ACEI activity in cheeses made from raw milk than in those made with sterilized one. The lack of differences observed in our results can be due to the fact that our tested cheeses were fresh and differences between raw and pasteurized cheeses are mainly observed throughout ripening, as previously discussed.
Nitrogenous fractions analysis by RP-HPLC
In order to observe the impact of pasteurization over the obtained PCA results, RP-HPLC of ASN, NPN, EtOH-SN and EtOH-NSN fractions were performed. For data analysis, we considered each peak as one compound. It is well known that pasteurization of milk prior to cheese making can affect the proteolysis, its effect over the proteolysis and the type of peptides produced in cheese has been previously reported (Albenzio et al., Citation2001; Kırmacı, Hayaloğlu, Özer, Atasoy, & Türkoğlu, Citation2014).
As a first approach, the nitrogenous fractions of each cheese were compared and noticeable differences were found between them. ASN and NPN fractions presented the most complexes profiles (207 and 120 peaks, respectively) while EtOH-SN and EtOH-NSN were simpler (43 and 22 peaks, respectively).
ANOVA and LSD tests showed that some peaks were statistically different between cheeses according to heat treatment. For ASN, three peptides with retention time (r.t.) at 2.05, 18.36 and 50.11 min showed significant differences (p = 0.048, 0.045 and 0.011, respectively) according to the heat treatment. NPN showed significant differences for one peptide r.t. at 49.27 min (p = 0.008). On the other hand, EtOH-SN and EtOH-NSN showed significant differences for two (r.t. 72.55 and 80.26 min) and one peptides (r.t. 18.86), respectively, depending on the heat treatment (p = 0.00013, 0.012 and 0.0004, respectively).
After each run, chromatograms were divided in HO and HI peptides to calculate the HO/HI ratio and compare more globally the peptide profiles between cheeses. Results are shown in . ASN was significantly different (p = 0.004) between pasteurized and raw cheeses only for HO peptides. Heat treatment increased the content of HO peptides (from an average of 11,821.93– 28,214.53) due probably to the effect of heat on the caseins, making them more fragile for a breakdown therefore increasing the hydrophobic peptides. Statistical analysis to the HO and HI peptides in NPN, EtOH-SN and EtOH-NSN profiles did not differentiate between cheeses. The breakdown of caseins with the increase of hydrophobic peptides had been reported by McSweeney et al. (Citation1993) in Cheddar cheeses.
Table 1. Proportion of hydrophobic, hydrophilic peptides and ratio (HO/HI) in nitrogenous fractions of fresh goat cheese prepared with raw and pasteurized milk.
Tabla 1. Proporción de péptidos hidrofóbicos, hidrofílicos y relación (HO/HI) en las fracciones nitrogenadas de quesos frescos de cabra preparados con leche cruda y pasteurizada.
Further PCA was applied to ASN fraction [,)]. PCA factorial map [)] of the HO and HI peptides in the ASN fraction is defined by PC1 and PC2, explaining 67.61% and 29.07%, respectively, of the total variance. In , samples were classified in two groups according to heat treatment, where raw samples were in the positive values of the PC2 and pasteurized ones in the negative values.
Figure 3. HO and HI peptides in the ASN profile. PCA plots of the first two principal components PC1 and PC2. (a) Factorial map. (b) Factor loading plots (PC1 and PC2). N: November, J: January, M: March. NP: raw, P: pasteurized.
Figura 3. Péptidos HO y HI en el perfil de ASN. Gráficas de ACP para los dos primeros componentes principales PC1 y PC2- (a) Mapa factorial. (b) Cargas de los factores (PC1 y PC2). N: Noviembre, J: Enero, M: Marzo. NP: Cruda, P: Pasteurizada.
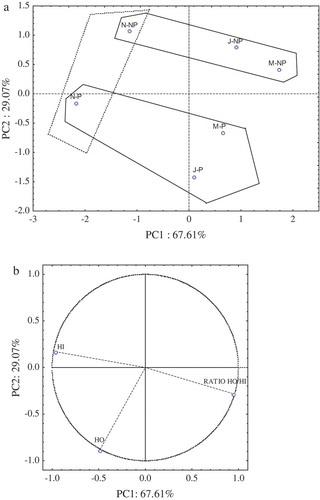
As shown in factor loading plot [)] the studied variables HI and Ratio HO/HI were strongly correlated with PC1 (R = −0.96 and 0.94 respectively).Whereas HO was the only variable correlated (R = −0.88) with PC2.
These results suggest that heat treatment influence significantly the type of peptides in the ASN fraction. This can be explained by the effect of pasteurization over the tertiary structure of caseins, producing denaturation through destabilization of hydrogen bonding and electrostatic interactions, while hydrophobic interactions are stabilized. This denaturation might further affect their enzymatic susceptibility, increasing the number of sites exposed to enzymatic attack, thus producing different peptides from a non-pasteurized milk (Hayaloglu & Brechany, Citation2007; Turgeon & Rioux, Citation2011). Despite some studies have been made to characterize the proteolysis by HPLC methods in ripened cheese, and even when heat treatment has already been probed to affect the proteolysis in cheeses, barely nothing has been published on the effect of heat treatment on the peptide profile of fresh cheeses. Therefore, the lack of published results makes it difficult to compare our findings with the literature. However, our results can be partially compared with previous works in the field of dairy protein degradation. Mullally, Mehra, and Fitzgerald (Citation1998) observed that after thermal denaturation of β-lactoglobulin, this protein was significantly more susceptible to enzymes. In addition, Rinaldi, Gauthier, Britten, and Turgeon (Citation2014) showed that high temperatures used during sterilization of the milk increases the pepsin susceptibility of β-lactoglobulin and α-lactoalbumin. Similar results were obtained by Dupont et al. (Citation2010) who observed that high temperatures used in sterilization of the milk produce extensive protein denaturation.
Thus, as observed in , heat treatment produced a change in the hydrolysis of the caseins affecting the production of HO peptides [)]. This HO variation further affects the ACEI activity in the EtOH-SN fraction that differentiates between NP and P cheeses [)]. Meanwhile, biological activity in NP cheeses seems to be more related to the HI peptides of the ASN fraction [ and )]. This is in agreement with previous results of our same research group for Cotija cheese (ripened cheese), where a high correlation was observed between HO peptides and ACEI activity; and HI peptides with the AA activity (Hernández Galán et al., Citation2016). However, further studies need to be carried out to understand the precise relationship between the type of peptides in a determined nitrogenous fractions and its biological activity.
Conclusions
Although the studied goat cheese was fresh and fabricated without starter culture, antioxidant and ACE inhibitory activities associated with peptides were observed, implying that nitrogenous fractions of fresh goat cheese had biological activity derived from the naturally present milk proteins or released by the rennet used during cheese manufacture.
PCA results of biological activity grouped cheeses according with heat treatment. These could be related with the observed variation in the type of peptides (HO and HI) and the ratio between them in the ASN fraction. Further studies need to be carried out to identify the peptides associated with each biological activity.
Acknowledgement
Leticia Hernández-Galán would like to thank the Consejo Nacional de Ciencia y Tecnología CONACyT) for her Ph.D. scholarship (211892).
Disclosure statement
No potential conflict of interest was reported by the authors.
References
- Abadia-Garcia, L., Cardador, A., Martin Del Campo, S.T., Arviz, S.M., Castaño-Tostado, E., Regalado-Gonzalez, C., … Amaya-Llano, S.L. (2013). Influence of probiotic strains added to cottage cheese on generation of potentially antioxidant peptides, anti-listerial activity, and survival of probiotic microorganisms in simulated gastrointestinal conditions. International Dairy Journal, 33(2), 191–197. doi:10.1016/j.idairyj.2013.04.005
- Ahmed, A.S., El-Bassiony, T., Elmalt, L.M., & Ibrahim, H.R. (2015). Identification of potent antioxidant bioactive peptides from goat milk proteins. Food Research International, 74, 80–88. doi:10.1016/j.foodres.2015.04.032
- Albenzio, M., Corbo, M.R., Rehman, S.U., Fox, P.F., De Angelis, M., Corsetti, A.S., … Gobbetti, M. (2001). Microbiological and biochemical characteristics of Canestrato Pugliese cheese made from raw milk, pasteurized milk or by heating the curd in hot whey. International Journal of Food Microbiology, 67, 35–48. doi:10.1016/S0168-1605(00)00533-X
- Aloğlu, H.Ş., & Öner, Z. (2011). Determination of antioxidant activity of bioactive peptide fractions obtained from yogurt. Journal of Dairy Science, 94, 5305–5314. doi:10.3168/jds.2011-4285
- Bezerra, V.S., Campos, J.F., Da Silva, R.A., Porto, T.S., De Lima-Filho, J.S., & Porto, A.L.F. (2013). Biotechnological richness of the northeastern semi-arid region: Antioxidant activity of casein hydrolysates from Moxotó goat milk (Capra hircus Linnaeus, 1758) obtained by papain action. Food Science and Technology, 33(3), 513–520. doi:10.1590/S0101-20612013005000074
- Dupont, D., Mandalari, G., Molle, D., Jardin, J., Rolet-Repecaud, O., Duboz, G., … Mackie, A.R. (2010). Food processing increases casein resistance to simulated infant digestion. Molecular Nutrition and Food Research, 54, 1677–1689. doi:10.1002/mnfr.200900582
- El Galiou, O., Zantar, S., Bakkali, M., & Laglaoui, A. (2013). Lipolysis and proteolysis during the ripening of fresh Moroccan goats’ milk cheese. World Journal of Dairy & Food Sciences, 8, 201–206. doi:10.5829/idosi.wjdfs.2013.8.2.8140
- Espejo-Carpio, F.J., De Gobba, C., Guadix, A., Guadix, E.M., & Otte, J. (2013). Angiotensin I-converting enzyme inhibitory activity of enzymatic hydrolysates of goat milk protein fractions. International Dairy Journal, 32(2), 175–183. doi:10.1016/j.idairyj.2013.04.002
- Fox, P.F., & McSweeney, P.L.H. (1996). Proteolysis in cheese during ripening [review]. Food Reviews International, 12(4), 457–509. doi:10.1080/87559129609541091
- Gonzalez De Llano, D., Polo, M.C., & Ramos, M. (1995). Study of proteolysis in artisanal cheeses: High performance liquid chromatography of peptides. Journal of Dairy Science, 78, 1018–1024. doi:10.3168/jds.S0022-0302(95)76717-0
- Guerra-Martínez, J.A., Montejano, J.G., & Martín-del-Campo, S.T. (2012). Evaluation of proteolytic and physicochemical changes during storage of fresh Panela cheese from Queretaro, Mexico and its impact in texture. CyTA - Journal of Food, 10(4), 296–305. doi:10.1080/19476337.2011.653791
- Gupta, A., Mann, B., Kumar, R., & Sangwan, R.B. (2009). Antioxidant activity of Cheddar cheeses at different stages of ripening [original research]. International Journal of Dairy Technology, 62(3), 339–347. doi:10.1111/j.1471-0307.2009.00509.x
- Gupta, A., Mann, B., Kumar, R., & Sangwan, R.B. (2013). ACE-inhibitory activity of cheddar cheeses made with adjunct cultures at different stages of ripening. Advances in Dairy Research, 1(1). doi:10.4172/2329-888X.1000102
- Hajirostamloo, B. (2010). Bioactive component in milk and dairy product [review]. World Acad Sci Eng Technol, 72, 162–166. doi:no
- Hayaloglu, A.A., & Brechany, E.Y. (2007). Influence of milk pasteurization and scalding temperature on the volatile compounds of Malatya, a farmhouse Halloumi-type cheese. Lait, 87, 39–57. doi:10.1051/lait:2006025
- Hernández Galán, L., Cardador Martínez, A., Picque, D., Spinnler, H.E., López Del Castillo Lozano, M., & Martín Del Campo Barba, S.T. (2016). Angiotensin converting enzyme inhibitors and antioxidant peptides release during ripening of Mexican Cotija hard cheese. Journal of Food Research, 5(3). doi:10.5539/jfr.v5n3p85
- Hernández-Ledesma, B., Amigo, L., Ramos, M., & Recio, I. (2004). Angiotensin converting enzyme inhibitory activity in commercial fermented products. Formation of peptides under simulated gastrointestinal digestion. Journal of Agricultural and Food Chemistry, 52(6), 1504–1510. doi:10.1021/jf034997b
- Hernández-Ledesma, B., Del Mar Contreras, M., & Recio, I. (2011). Antihypertensive peptides: Production, bioavailability and incorporation into foods. Advances in Colloid and Interface Science, 165(1), 23–35. doi:10.1016/j.cis.2010.11.001
- Jäkälä, P., & Vapaatalo, H. (2010). Antihypertensive peptides from milk proteins [review]. Pharmaceuticals, 3, 251–272. doi:10.3390/ph3010251
- Kırmacı, H.A., Hayaloğlu, A.A., Özer, H.B., Atasoy, A.F., & Türkoğlu, H. (2014). Evolution of proteolysis in Urfa cheese made from ewe’s milkby wild type starter culture systems [research]. Small Ruminant Research, 119, 120–129. doi:10.1016/j.smallrumres.2014.01.006
- Kitts, D.D., & Weiler, K. (2003). Bioactive proteins and peptides from food sources. Applications of bioprocesses used in isolation and recovery [review]. Current Pharmaceutical Design, 9(16), 1309–1323. doi:10.2174/1381612033454883
- Korhonen, H., & Pihlanto, A. (2003). Food-derived bioactive peptides – Opportunities for designing future foods. Current Pharmaceutical Design, 9, 1297–1308. doi:10.1016/j.idairyj.2005.10.012
- Korhonen, H., & Pihlanto, A. (2006). Bioactive peptides: Production and functionality [review]. International Dairy Journal, 16, 945–960. doi:10.1016/j.idairyj.2005.10.012
- Lacroix, C., Verret, P., & Paquin, P. (1996). Regional and seasonal variations of nitrogen fractions in commingled milk. International Dairy Journal, 6, 947–961. doi:10.1016/S0958-6946(96)00022-2
- McSweeney, P.L.H., Fox, P.F., Lucey, J.A., Jordan, K.N., & Cogan, T.M. (1993). Contribution of the indigenous microflora to the maturation of cheddar cheese. International Dairy Journal, 3, 613–634. doi:10.1016/0958-6946(93)90104-8
- Meira, S.M.M., Daroit, D.J., Helfer, V.E., Corrêa, A.P.F., Segalin, J., Carro, S., & Brandelli, A. (2012). Bioactive peptides in water-soluble extracts of ovine cheeses from Southern Brazil and Uruguay. Food Research International, 48(1), 322–329. doi:10.1016/j.foodres.2012.05.009
- Meisel, H. (2004). Multifunctional peptides encrypted in milk proteins. BioFactors, 21, 55–61. doi:10.1002/biof.552210111
- Mullally, M.M., Mehra, R., & Fitzgerald, R.J. (1998). Thermal effects onthe conformation and susceptibility of beta-lactoglobulin to hydrolysis by gastric and pancreatic endoproteinases. Irish Journal of Agricultural and Food Research, 37(1), 51–60.
- Ng-Kwai-Hang, K.F., Hayes, J.F., Moxley, J.E., & Monardes, H.G. (1984). Variability of test-day milk production and composition and relation of somatic cell counts with yield and compositional changes of bovine milk. Journal of Dairy Science, 67, 361–366. doi:10.3168/jds.S0022-0302(84)81309-0
- Olvera-García, V., Cardador-Martínez, A., & Martín Del Campo, S.T. (2015). Effect of thermal process and filtration on the antioxidant activity and physicochemical parametes of agave atrovirens extracts. Journal of Food Research, 4, 155–167. doi:10.5539/jfr.v4n1p155
- Ong, L., Henriksson, A., & Shah, N.P. (2007). Angiotensin converting enzyme-inhibitory activity in Cheddar cheeses made with the addition of probiotic Lactobacillus casei sp [original article]. Lait, 87, 149–165. doi:10.1051/lait:2007004
- Paul, M., & Van Hekken, D.L. (2011). Assessing antihypertensive activity in native and model Queso Fresco cheeses. Journal of Dairy Science, 94, 2280–2284. doi:10.3168/jds.2010-3852
- Pihlanto, A. (2006). Antioxidative peptides derived from milk proteins. International Dairy Journal, 16, 1306–1314. doi:10.1016/j.idairyj.2006.06.005
- Rinaldi, L., Gauthier, S.F., Britten, M., & Turgeon, S.L. (2014). In vitro gastrointestinal digestion of liquid and semi-liquid dairy matrixes. LWT - Food Science and Technology, 57(1), 99–105. doi:10.1016/j.lwt.2014.01.026
- Sánchez-Macías, D., Morales-delaNuez, A., Moreno-Indias, I., Hernández-Castellano, L.E., Mendoza-Grimón, V., Castro, N., & Argüello, A. (2011). Lipolysis and proteolysis profiles of fresh artisanal goat cheese made with raw milk with 3 different fat contents. Journal of Dairy Science, 94, 5786–5793. doi:10.3168/jds.2011-4423
- Scholz, W. (1997). Elaboración de quesos de oveja y de cabra (pp. 145). Zaragoza, Spain: Acribia.
- Silva, S., Pihlanto, A., & Malcata, F. (2006). Bioactive peptides in ovine and caprine cheeselike systems prepared with proteases from cynara cardunculus [experimental]. Journal of Dairy Science, 89(9), 3336–3344. doi:10.3168/jds.S0022-0302(06)72370-0
- Théolier, J., Hammami, R., Fliss, I., & Jean, J. (2014). Antibacterial and antifungal activity of water-soluble extracts from Mozzarella, Gouda, Swiss, and Cheddar commercial cheeses produced in Canada. Dairy Science & Technology, 94, 427–438. doi:10.1007/s13594-014-0170-9
- Timón, M.L., Parra, V., Otte, J., Broncano, J.M., & Petrón, M.J. (2014). Identification of radical scavenging peptides (<3 kDa) from Burgos-type cheese. LWT - Food Science and Technology, 57(1), 359–365. doi:10.1016/j.lwt.2014.01.020
- Torres-Llanez, M., González-Córdova, A., Hernandez-Mendoza, A., Garcia, H., & Vallejo-Cordoba, B. (2011). Angiotensin-converting enzyme inhibitory activity in Mexican Fresco cheese [experimental]. Journal of Dairy Science, 94(8), 3794–3800. doi:10.3168/jds.2011-4237
- Turgeon, S.L., & Rioux, L. (2011). Food matrix impact on macronutrients nutritional properties. Food Hydrocolloids, 25, 1915–1924. doi:10.1016/j.foodhyd.2011.02.026
- Wang, W., Wang, N., Zhang, Y., Cai, Z., Chen, Q., & He, G. (2013). A convenient RP-HPLC method for assay bioactivities of Angiotensin I-converting enzyme inhibitory peptides. ISRN Biotechnology, 2013, 1–5. doi:10.5402/2013/453910