ABSTRACT
In this study, the food-grade fungal strain DCY-1 was isolated from Chinese traditional fermented soymilk residues (okara) and identified as Actinomucor elegans. The novel strain was used in the solid-state fermentation (SSF) of okara to improve their functional properties. Sodium dodecyl sulfate–polyacrylamide gel electrophoresis (SDS-PAGE) and reverse-phase high-performance liquid chromatography (RP-HPLC) analyses showed that okara proteins were degraded into peptides during fermentation. SSF okara showed high antioxidant activities, which included reducing power, ferrous ion-chelating activity, and scavenging effect of DPPH, ABTS, and hydroxyl radicals. Compared with the control, the antioxidant activities mentioned above increased by 4.29, 2.32, 3.45, 1.55, and 1.63-fold, respectively. SSF okara also showed a significantly higher ACE-inhibitory activity (61.17% ± 0.94%) at the end of fermentation. Therefore, SSF can be employed as a valuable process to obtain bioactive ingredients from okara, which would encourage their utilization in the formulation of value-added functional foods.
RESUMEN
En este estudio, la cepa fúngica de calidad alimentaria DCY-1 se aisló de los residuos de leche de soja tradicional china fermentada (okara) y se identificó como Actinomucor elegans. La nueva cepa se utilizó para la fermentación de okara en estado sólido (SSF) para mejorar sus propiedades funcionales. Los análisis SDS-PAGE y RP-HPLC mostraron que las proteínas de la okara fueron degradadas a péptidos durante la fermentación. La okara SSF mostró un alto nivel de actividades antioxidantes, las cuales incluían: el poder reductor, la actividad quelante del ión ferroso, además del efecto de barrido de radicales DPPH, ABTS y hidroxilo. En comparación con la muestra control, las actividades antioxidantes mencionadas anteriormente aumentaron a 4,29; 2,32; 3,45; 1,55 y 1,63 veces, respectivamente. La okara SSF también mostró una actividad inhibitoria ACE significativamente alta (61,17% ± 0,94%) al final de la fermentación. De este modo, SSF puede ser utilizado como un proceso valioso para obtener ingredientes bioactivos de la okara, los cuales podrían favorecer su utilización en la formulación de alimentos funcionales con valor añadido.
1. Introduction
Soybean is one of the most important legumes and oil crops in Asia. The popularity of consumption of soy-derived foods, such as soymilk and tofu, has led to the production of soymilk residues. Soymilk residues, also named okara in Japanese, are a group of insoluble byproducts resulting from the production of soybean curd (tofu), soymilk, and other soybean products. Except for a small part used as animal feed, the rest is discarded as industrial garbage (Mizumoto, Hirai, & Shoda, Citation2006). As this residue is abundant in nutrients and consists of large amount of proteins, carbohydrates, and bioactive compounds such as isoflavones, polysaccharides, phytosterols, saponins, and phytates, a growing interest to utilize and to further explore these byproducts as a source of bio-functional compound now exists (Li, Qiao, & Lu, Citation2012).
Solid-state fermentation (SSF) is a conventionally biotechnological method of enhancing the nutritional value and functional properties of cereal gain (Xiao, Zhang et al., Citation2015). The biological activities of legumes can be improved by SSF as microbial proteases promote protein degradation and generate bioactive peptides during fermentation. Researchers demonstrated that SSF can improve the antioxidant properties of soy protein by increasing the concentrations of bioactive components, for instance, free amino acids and small-size peptides (Sanjukta, Rai, Muhammed, Jeyaram, & Talukdar, Citation2015; Teng et al., Citation2012). Furthermore, SSF was also employed to produce legume-based food with angiotensin I-converting enzyme (ACE)-inhibitory activity targeting the prevention and treatment of hypertension (Torino et al., Citation2013).
Several studies have investigated the use of the soymilk residues, including hydrolyzing soymilk residues to increase their digestibility (Kasai, Murata, Inui, Sakamoto, & Kahn, Citation2004), which produces some bioactive compounds by SSF (Mizumoto et al., Citation2006; Rashad, Mahmoud, & Abdou et al., Citation2011; Zhu, Fan, Cheng, & Li, Citation2008). These studies have well expanded our knowledge bases for the application of soymilk residues and developing them into novel functional foods. In addition, fermentation was observed to be an efficient approach in producing some bioactive compounds. However, the information on the influences of fermentation on the antioxidant activity and ACE-inhibitory activity of soymilk residues is limited.
In this study, Actinomucor elegans DCY-1 (A. elegans DCY-1) has been isolated from Chinese traditional fermented okara, and the impacts of SSF on antioxidant and ACE-inhibitory activities of okara were investigated. In addition, reverse-phase high-performance liquid chromatography (RP-HPLC) analysis and sodium dodecyl sulfate–polyacrylamide gel electrophoresis (SDS-PAGE) were carried out to investigate the proteolytic effect of A. elegans DCY-1 on soymilk residues.
2. Materials and methods
2.1. Chemicals
The compounds 2, 2-diphenyl-1-picrylhydrazyl (DPPH), 2, 2-azinobis (3-ethylbenzothiazoline-6-sulfonic acid) diammonium salt (ABTS), ferrozine, ethylenediaminetetraacetic acid disodium salt (EDTA-2Na), ascorbic acid AA (VC), ACE), hippuryl-l-histidyl-L-leucine (HHL), sodium dodecyl sulfate (SDS), and O-Phthaldialdehyde (OPA) were purchased from Sigma Chemical Co. (St. Louis, MO, U.S.A.). HPLC acetonitrile and trifluoroacetic acid were purchased from Merck (Darmstadt, Germany). All other reagents used were of analytical grade.
2.2. Isolation and identification of fungal strain, DYC-1
The fungal strain DYC-1 used in this study was previously isolated from Meidouza, a traditional Chinese fermented food made from soymilk residues. To study its morphological identification, DYC-1 was identified using established procedures which employ microscopic and macroscopic characteristics.
Molecular identification of DYC-1 was performed by sequencing the fungal internal transcribed spacer (ITS) region. Extraction of fungal genomic DNA was conducted with Fast DNA Kit (Bio 101, Carlsbad, U.S.A.) according to the manufacturer’s instructions. The ITS region primers (ITS 1, 5ʹ-TCCGTAGGTGAACCTGCGG- 3ʹ; ITS 4, 5ʹ-TCCTCCGCTTATTGATATG-3ʹ) utilized the conserved regions of the 18S (ITS 1) and the 28S (ITS 4) rRNA genes to amplify the intervening 5.8S gene and the ITS 1 and ITS 2 noncoding regions. The polymerase chain reaction (PCR) amplification was conducted using a previously described method (Khan et al., Citation2008), and the DNA samples were sequenced using 3730XL automated sequencer in Shanghai Sangon Biotechnology Company, China.
2.3. SSF of soymilk residues (okara)
The fresh soymilk residues used for this study were purchased from the local market. Then, the fresh okara (85% moisture) was dried to 60% moisture content and autoclaved at 121°C for 15 min. After cooling down to room temperature, 1% (v/w) A. elegans DYC-1 spore suspension (108–109 spores/ml) was added into the autoclaved okara, paved in polyethylene bags with a toothpick hole every 2 cm and incubated for 60 h at 25°C in an incubator with relative humidity at around 95% (LTI-601 SD, Tokyo Rikakikai Co., Ltd., Tokyo, Japan). The samples were withdrawn at 0, 24, 36, 48, and 60 h to lyophilize and ground separately. The flours were passed through a 0.2 mm sieve and then stored at 4°C for further analysis.
2.4. Evaluation of antioxidant activities
2.4.1. Preparation of SSF okara extracts
The extraction of okara was conducted based on a previously described method with some modifications (Zhu et al., Citation2008). The different fermentation time samples were extracted with 25 ml of deionized water at 50°C for 4 h. The extracts were then centrifuged at 12,000 g for 15 min at 4°C, and the precipitates were discarded. The turbid supernatants were then filtered using a 0.45 µm membrane filter, and the filtrates were used as the water extract of soymilk residues for further analysis of the antioxidant properties.
2.4.2. Assay of fermentation extract for the analysis of reducing power
The reducing power was determined using a previously described method with some modifications (Sanjukta et al., Citation2015). An aliquot of 0.2 ml extract was mixed with 1 ml of phosphate buffer (0.2 M, pH 6.6) and 1 ml of 1% potassium ferric cyanide, and the mixture was incubated at 50°C for 20 min. After the mixture was cooled to room temperature, 1 ml of 10% TCA was added to terminate the reaction. Then, the mixture was centrifuged at 420 g for 10 min. Afterward, 1 ml of the upper layer was mixed with 0.2 ml 0.1% FeCl3. After 10 min of mixing, the absorbance was measured at 700 nm. Increased absorbance of the reaction mixture corresponded to higher reducing power. VC was used as calibration standard, and results were expressed as µg VC equivalents (VCE) per gram of dry weight sample (µg VCE/g dw).
2.4.3. Assay of scavenging DPPH radicals
The DPPH radical scavenging property was determined using a previously described method with the addition of slight modifications (Bhanja, Kumari, & Banerjee, Citation2009). An aliquot (0.5 ml) of the DPPH radical solution (0.4 mM, in methanol) and 0.5 ml of the sample solution were added to the centrifuge tube and the mixture was then kept in the dark for 30 min at room temperature. After incubation, the absorbance was measured at 517 nm. Final results were expressed as µg VCE/g of dry weight sample (µg VCE/g dw).
2.4.4. Assay of scavenging ABTS radical cation
ABTS radical cation (ABTS·+) scavenging activity of the sample was analyzed using previously described method with inclusion of some modifications (Sasipriya & Siddhuraju, Citation2012). ABTS·+ was generated by reacting 10 ml of 7 mmol/l ABTS solution with 20 ml of 2.45 mM potassium persulfate in the dark at room temperature for 12–16 h before use. To study the food extracts, the freshly prepared ABTS·+ solution was diluted with ethanol to obtain an absorbance of 0.70 ± 0.02 at 734 nm and equilibrated at 30°C. Then, 1 ml of the sample solution was mixed with 4 ml of ABTS radical solution, and the absorbance was measured at 734 nm after 6 min. Similar to DPPH radical scavenging property, the ABTS radical scavenging activity was calculated as VCE capacity expressed as µg VCE/g dry weight sample (µg VCE/g dw).
2.4.5. Assay of scavenging hydroxyl radicals (OH)
The hydroxyl radical scavenging activity was conducted using a previously described method with minor modifications (Liu, Jia, Kan, & Jin, Citation2013). Briefly, the hydroxyl radical was generated by incubating 1 ml of 9 mM FeSO4, 1 ml of 9 mM salicylic acid–ethanol solution, and 1 ml of 8.8 mM H2O2. After addition of 1 ml sample solution, the mixture was incubated at 37°C for 30 min, and the absorbance of the reaction mixture was then determined at 510 nm. Results were expressed as µg VCE/g dry weight sample (µg VCE/g dw).
2.4.6. Assay of ferrous ion with chelating ability
The ferrous ion-chelating ability was measured using a previously described method with minor modifications (Sun et al., Citation2015). Briefly, 0.5 ml of the extracts was mixed with 0.75 ml of deionized water and 0.05 ml of ferrous chloride (2 mM). Then, 0.1 ml of 5.0 mM ferrozine was added and reacted for 20 min at room temperature. The absorbance was then read at 562 nm. The chelating ability was quantified by external calibration using EDTA-2Na as standard, and results were expressed as µg EDTA-2Na/g dry weight sample (µg EDTA-2Na/g dw).
2.5. Determination of the ACE inhibition activity
Samples at different fermentation periods (40 mg) were extracted using 1 ml of borate buffer (0.1 M, containing 0.3 M sodium chloride, pH 8.3) at room temperature for 1 h, respectively. The mixtures were centrifuged at 12,000 g for 15 min, and the supernatants were then used to determine ACE inhibition activity according to a previously described method with minor modifications (Rui et al., Citation2015). Briefly, 10 µl of sample solution was added to 50 µl of 2.17 mM HHL and 10 µl of ACE (100 mU/ml), and incubated for 30 min at 37°C. After terminating the reaction by adding 85 µl of 1 M HCl, 20 µl of the solution was injected into the HPLC system (4.60 × 250 mm, 5 µm particle size, Agilent) to measure the HA peak (mobile phase: 50% methanol in 0.1% TFA, 50% water in 0.1% TFA, detection wavelength: 228 nm; flow rate: 0.8 ml/min). The reactions on the standard solution were carried out by replacing the sample extracts with distilled water, and the blank solutions were prepared without the addition of ACE and sample extracts. ACE-inhibitory activity was calculated according to the following equation:
ACE-inhibitory activity (%) = (Astandard−Asample)/(Astandard−Ablank) × 100,
where Astandard, Ablank, Asample is the absorbance of the standard, blank, and sample solution, respectively.
2.6. Determination of proteolytic activity
One gram of each sample taken at different fermentation periods was suspended in 20 ml of deionized water for 1 h at room temperature with occasional shaking and centrifuged at 12,000 g for 10 min. The supernatants were determined using a previously described protease assay with some modifications (Elkhalifa & Bernhardt, Citation2010). Briefly, 1 ml supernatant was mixed with 1 ml of 1% casein and incubated for 10 min at 40°C in a shaking water bath. Then, 2 ml of 10% TCA was added to the enzyme mixture to completely precipitate the protein which was subsequently filtered through a filter. Tyrosine liberated from the filtrate during casein hydrolysis was measured and was determined using a previously described method (Lowry, Rosebrough, Farr, & Randall, Citation1951). One unit (U) of protease activity was defined as the amount of enzyme that is released per 1 µg of tyrosine per minute under the assay conditions (using tyrosine as the reference). Results were expressed as U/g of dry weight sample.
2.7. Determination of peptide content
The O-Phthaldialdehyde (OPA) assay was carried out to measure the peptide content of soymilk residues in different fermentation periods. The sample solution obtained in 2.4.1 and the OPA solution were prepared using a previously described method (Muhialdin, Hassan, Bakar, & Saari, Citation2016). About 50 ml of the sample solution was mixed with 2 ml of reagent, and the absorbance at 340 nm was measured after 20 min of incubation at room temperature. The peptide content was quantified using l-glutathione (reduced form) as standard, and final results were expressed as mg l-glutathione/g dry weight sample.
2.8. Electrophoresis
The hydrolysis of soymilk residues protein during fermentation was monitored by SDS-PAGE using 4% stacking gel and 12% separating gels according to previously described methods (Wu et al., Citation2015). The extracted soymilk residues were mixed with the sample treatment buffer (1:1, v/v) and boiled for 5 min prior to the electrophoresis run. Electrophoresis was carried out at 60 V in the stacking gel, followed by 120 V in the separating gel. The gels were stained with 0.1% (w/v) Coomassie brilliant blue R-250 at room temperature, followed by destaining with 10% (v/v) acetic acid. MW of protein fractions were estimated by Bio-Rad Laboratories (Hercules, CA, U.S.A.). The protein-stained bands were scanned with Image Scanner III (GE Healthcare Biosciences, Uppsala, Sweden) and then analyzed using Quantity One software, version 4.6.2 (Bio-Rad Laboratories, Inc., Hercules, CA, U.S.A.).
2.9. Analysis of peptide by RP-HPLC
RP-HPLC was widely utilized to generate a peptide map using a ZORBAX Eclipse PLUS C18 reversed-phase analytical column (4.60 mm × 250 mm, 5 µm particle size, Agilent). Briefly, 20 µl samples were filtrated using a 0.45 µm membrane and injected into the HPLC system for analysis. The mobile phase consisted of deionized water in 0.1% TCA (solvent A) and acetonitrile in 0.1% TCA (solvent B). The solvent flow rate was 0.8 ml/min, and the elution included the following eluent B concentrations: 0–7 min, 5–10%; 7–10 min, 10–15%; 10–20 min, 15–20%; 20–30 min, 20–30%; 30–35 min, 30–100%; and 35–45 min, 100–5%. The UV absorbance of the eluents was monitored at 280 nm using a DAD detector (G1315 B).
2.10. Statistical analysis
All experiments were performed in triplicates and expressed as mean ± standard deviation. Analysis of variance (ANOVA) and Duncan’s multiple comparison tests were used to determine the significant differences between means (p < 0.05) using IBM SPSS Statistics version 17.0. Correlations among variables were examined with the coefficient of determination (R2) calculated using MS Excel.
3. Results and discussion
3.1. Identification of strain DCY-1 from Chinese fermented soymilk residues
Our results showed that strain DCY-1 grew rapidly on PDA at 25°C, filling the entire 90 mm Petri dish within 3 days. The colonies were initially white, loosely floccose, and formed abundant aerial mycelium until they reached the lid of the Petri dish. After 3 days of incubation, the colonies turned yellowish brown and aerial growth became more interwoven or mat-like. Lactophenol cotton blue stain revealed distinct microscopic features of DCY-1 (,). In the figures, the small arrow points to the aseptate aerial hyphae and branched sporangiophores terminating at sporangium of various lengths, and the double-headed arrow shows the intact sporangium with globose to subglobose structure. Crystalline granular materials, which were spherical and smooth sporangiospores, were released from the sporangium as indicated by the large arrow. The neighbor-joining phylogenetic tree obtained from the ITS region sequences using the sequence from Mucor hiemalis as an out-group is shown in ). The isolated DCY-1 formed a separate lineage that branched off before the branch of the common ancestor of A. elegans and A. elegans var. meitauzae strains.
Figure 1. Lactophenol cotton blue stain revealed distinct microscopic features of DCY-1 (magnification, ×40) (a and b), and parsimonious tree was obtained from ITS sequences data, showing the relationships of strain DCY-1 compared with relevant collection strains or ITS sequences (c).
Figura 1. La mancha azul algodón de Lactophenol reveló distintas características microscopicas de DCY-1 (magnificación, ×40) (a y b) y el árbol parsimonioso se obtuvo de las secuencias de datos ITS, mostrando las relaciones de la cepa DCY-1 en comparación con las cepas recogidas o secuencias ITS (c).
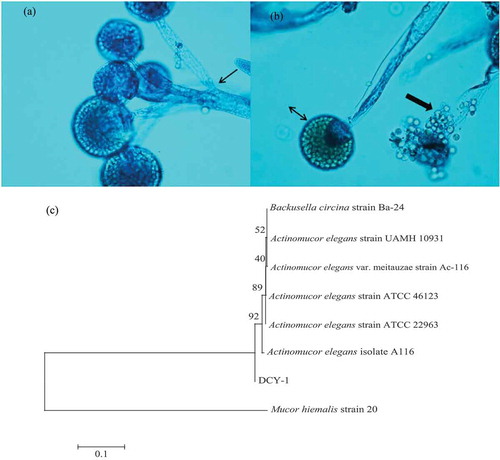
3.2. Effect of SSF on antioxidant activities of soymilk residues (okara)
Due to the varied radicals and oxidizing agents which cause different irreversible cellular and tissue damages, antioxidant activity is influenced by many factors (Shahidi & Zhong, Citation2015). Thus, a single antioxidant activity measurement hardly reflected the antioxidant properties of samples. In our study, five antioxidant activities of fermented okara were measured.
As shown in , the enhancement of the reducing power of soymilk residues was notable during the fermentation process. Fermented okara exhibited the highest reducing power that ranged from 132.70 ± 6.34 to 575.83 ± 23.78 µg VCE/g dw. Given the direct correlation between reducing power and antioxidant activity, results of reducing power analysis can serve as a significant indicator of potential antioxidant activity. The increased reducing power of fermented okara observed in the present study is consistent with previous reports showing that the fermented samples have more effective reducing power than the non-fermented ones (Singh, Singh, Singh, & Nautiyal, Citation2010; Xiao, Xing et al., Citation2015). During fermentation, the enhanced reducing power observed was associated with the presence of reductones in the sample which directly reacted with free radicals to terminate the radical chain reactions (Sanjukta et al., Citation2015). In addition, the peptides of the starter organism, intracellular antioxidants, and hydrogen-donating ability also possibly contributed to the high reducing power observed (I.–H. Lee, Hung, & Chou, Citation2008). Furthermore, free amino acids, such as leucine, methionine, tyrosine, histidine, and tryptophan have also been associated with strong reducing power (Ajibola, Fashakin, Fagbemi, & Aluko, Citation2011).
Table 1. Antioxidant activities of the water extract of soymilk residues at different periods.
Tabla 1. Actividades antioxidantes del extracto de agua de los residuos de leche de soja en diferentes periodos.
Ability to mitigate radicals is very important because free radicals poison living systems under oxidative stress. Results suggest that fermented soymilk residues by A. elegans DCY-1 obviously enhanced the DPPH, ABTS·+, and ·OH radical scavenging activities. The highest scavenging abilities for DPPH, ABTS·+, and OH were 550.54 ± 3.61, 4298.66 ± 93.94, and 27,570.51 ± 182.59 µg VCE/g dw, respectively. The scavenging activities compared with that of the unfermented okara was enhanced by 3.45, 1.55, and 1.63-fold, respectively. Researchers have shown that numerous active peptides and amino acids produced via microbial fermentation can scavenge the free radicals which thereby improve the antioxidant activity (Sanjukta et al., Citation2015; Sun et al., Citation2015; Teng et al., Citation2012). A previous study reported a similar result showing that the higher DPPH, and OH radical scavenging activities of cottonseed meal fermented by Bacillus subtilis BJ-1 was probably due to the higher proteolytic properties of B. subtilis during the fermentation process (Sun et al., Citation2015). The hydroxyl radical can be directly scavenged or chelated by ferrous ions which are needed to generate hydroxyl radicals in the assay system. Therefore, the increase in hydroxyl radical scavenging activity by okara fermentation process can be attributed to the chelating activity of ferrous ions, which retarded the generation of hydroxyl radicals via Fenton reaction.
Provided that ferrous ions are the most effective prooxidants in food systems (Yang, Mau, Ko, & Huang, Citation2000) and ferrous ions are commonly found in food systems, their higher chelating effects are beneficial. In the initial period of fermentation (0–48 h), the ferrous ion-chelating activity of the extract increased with values ranging from 567.77 ± 10.53 to 1309.42 ± 15.66 µg VCE/g dw which then steadily rose until the end of fermentation. A previous study reported a similar showing result that SSF has a positive influence on the Fe2+-chelating ability of soybean seed and cottonseed meal (Singh et al., Citation2010; Sun et al., Citation2015). Previous thoughts claimed that many active peptides play an important role in the chelation of metal ions because of the carboxyl and amino groups in their side chains which can capture the prooxidative free metal ions from the hydroxyl radical system (Zhang et al., Citation2011). In addition, researchers indicated the importance of aromatic and hydrophobic amino acids in the Fe2+-chelating ability of compounds (Pownall, Udenigwe, & Aluko, Citation2010). Therefore, the ferrous ion-chelating activity observed in the study depended on peptide composition and specific amino acid sequence. The fermented okara possibly inhibited the formation of radicals by stabilizing the transition metals and consequently protecting the organism against oxidative damage. Therefore, fermented okara caused by the chelating activity of ferrous ion can be used as an effective antioxidant agent.
3.3. Effect of SSF on ACE-inhibitory activity of soymilk residues (okara)
ACE-inhibitory activities of the fermented soymilk residues are presented in . Results indicate that fermented okara showed a strong ACE-inhibitory activity, which was also markedly enhanced as the fermentation time progressed. Non-fermented okara showed a low ACE-inhibitory activity (27.23% ± 1.10%). After 24 h of fermentation process, a steady rise in the ACE-inhibitory activity was observed until the end of fermentation. The highest inhibition ratio (61.17% ± 0.94%) was obtained at the end of the fermentation process, which was possibly caused by the accumulation of bioactive peptides in the soymilk residues. Studies reported (B.-H. Lee, Lai, & Wu, Citation2015; Torino et al., Citation2013) that fermented cereals showed higher ACE-inhibitory activity than non-fermented cereals, suggesting that A. elegans DCY-1 in the present study possibly produced ACE-inhibitory substances during fermentation. Small peptides are generally accepted as the main substance that induces potent ACE-inhibitory activity (Lee et al., Citation2015; Rui et al., Citation2015). Our results also correspond to the generation of small peptides caused by the strong proteolytic capacity of A. elegans DCY-1, leading to observation of significant ACE-inhibitory activity in the fermented soymilk residues.
Figure 2. In vitro ACE-inhibitory activity of fermented okara at different fermentation periods. Means with different lower case letters (a, b, c, d, and e) indicate significant difference (p < 0.05) among the different fermentation periods of okara.
Figura 2. Actividad inhibitoria ACE in vitro de okara fermentada en diferentes periodos de fermentación. Los promedios con distintas letras minúsculas (a, b, c, d y e) indican diferencias significativas (p < 0,05) entre los diferentes periodos de fermentación de la okara.
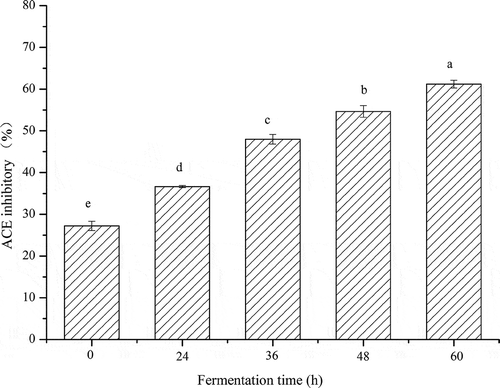
3.4. Effect of SSF on protease activity and peptide content of soymilk residues (okara)
Results for the protease activity of the fermented okara are presented in . Protease activity of the fermented okara increased by 1319.33 ± 66.22 U/g during fermentation. Previous studies reported similar findings (Chutmanop, Chuichulcherm, Chisti, & Srinophakun, Citation2008) for the protease production by microbial during fermentation. The protease produced during A. elegans DCY-1 fermentation led to the breakdown of complex stored proteins into simpler and more soluble substances (Xiao, Xing et al., Citation2015). As a result, some active substances such as small-size peptides were released by the action of the proteases produced during fermentation.
Figure 3. Changes in protease activity and peptide content of okara at different fermentation periods. Means with different lower case letters (a, b, c, and d) indicate significant difference (p < 0.05) among the different fermentation periods of okara.
Figura 3. Cambios en la actividad de las proteasas y el contenido de péptidos de la okara en los diferentes periodos de fermentación. Los promedios con distintas letras minúsculas (a, b, c y d) indican diferencias significativas (p < 0,05) entre los diferentes periodos de fermentación de la okara.
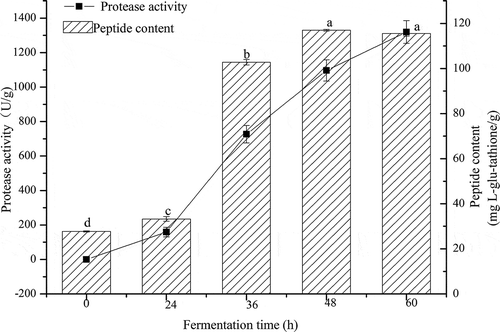
Peptides are the well-known products of protein hydrolysis, and small molecular weight peptides have been reported to possess high nutritional value and biological activity. Thus, solutions filtered with 10 kDa exclusion membranes were used to measure the peptide content of the sample at different fermentation periods (). The initial peptide content of non-fermented water-soluble extracts was 27.72 ± 0.28 mg l-glutathione/g dw, indicating that water is an efficient solvent for extracting peptide compounds. After 24 h of fermentation, the peptide content reached 33.26 ± 1.00 mg l-glutathione/g dw and then quickly increased to 102.82 ± 1.22 mg l-glutathione/g dw at 36 h. The highest peptide concentrations reached 117.00 ± 0.36 mg l-glutathione/g dw after 48 h of fermentation and then statistically remained at a constant level until the end of the fermentation. Such results are in partial agreement with a previous study which reported that SSF with Bacillus subtilis or Aspergillus oryzae enhanced the peptide content of soybean meal (Teng et al., Citation2012). The increase in the peptide contents can be ascribed to a specific microbial metabolism process that occurs during fermentation. Although the growth of A. elegans DCY-1 was not monitored during fermentation process, all the SSF processes were sterile. Increase in peptide content can be attributed to the proteolytic activity of A. elegans DCY-1, not of other microorganisms. Overall, we can conclude that the increase in peptide content was responsible for the remarkable action of proteolysis of A. elegans DCY-1, and fermented soymilk residues have great potential as source of bioactive peptides.
3.5. Effect of SSF on SDS-PAGE profile of soymilk residues (okara)
As fermentation progressed, the main protein composition of soymilk residues was altered as observed through the SDS-PAGE (). The non-fermented soymilk residues (Lane 1) exhibited almost all the major protein subunits, including the α′subunit (83 kDa), α subunit (70 kDa), β subunit (55 kDa) of β-conglycinin (7S fraction), A3 protein (43 kDa), other acidic subunits (37 kDa), and the basic subunits (20 kDa) of glycinin (11S fraction). A complete absence of the α′ subunit, α subunit, and A3 protein was observed after 24 h of fermentation, and the other subunits were also degraded to some extent. Notably, after 48 h of fermentation (Lane 4), more β-conglycinin and acidic subunits proteins were hydrolyzed into low molecular weight peptides, resulting in the enhanced concentrations of small peptides and generation of newly formed peptides with molecular weights of 20–35 kDa; however, they were not well separated in the gel.
Figure 4. Sodium dodecyl sulfate–polyacrylamide gel electrophoresis (SDS-PAGE) profiles of fermented okara at different fermentation periods. Lanes 1–5 represent the samples fermented at 0, 24, 36, 48, and 60 h, respectively. Lane 6 represents the molecular weight marker.
Figura 4. Perfiles electroforéticos del gel de poliacrilamida dodecil sulfato de sodio (SDS-PAGE) de okara fermentada en diferentes periodos de fermentación. Las barras 1–5 representan las muestras fermentadas a 0, 24, 36, 48 y 60 h, respectivamente. La barra 6 representa el marcador de peso molecular.
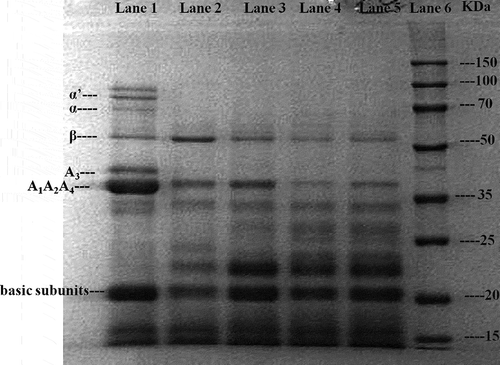
Our findings corroborate well with earlier report showing that soy proteins decreased during fermentation with Monascus, whereas newly generated peptide bands of 22–31 kDa were not clearly separated in the gel (Lim et al., Citation2010). Our results differ substantially from a previous report for chickpeas (Xiao, Xing et al., Citation2015), in which the proteins were almost completely hydrolyzed after SSF with Cordyceps militaris strains. The discrepancy in the extent of protein degradation is determined by the material, fermentation conditions, and strains used during fermentation, which influenced the specificity of the protease secreted. Due to the structure and complexity of soybean protein, the 11S fraction, particularly polypeptide B, is more difficult to hydrolyze than the 7S fraction (Ortiz & An, Citation2000). Therefore, A.elegans DCY-1 was possibly unable to hydrolyze some protein subunits completely.
3.6. Effect of SSF peptide profile of soymilk residues
RP-HPLC was conducted to analyze the peptide profiles of soymilk residues and to prove the occurrence of protein degradation that led to the production of lower molecular weight peptides during the fermentation process (). Samples taken at different fermentation periods were filtered with 10 kDa exclusion membranes to determine the peptides that resulted from proteolysis and which were not detected by SDS-PAGE. The 10 major peaks which demonstrated similar areas in the chromatograms were chosen for detailed comparison and calculation. Slight reductions of 2.9% ± 0.52% and 53.8% ± 0.78% (calculated based on area) were observed for peaks 5, 6, 7, 8, 9, and 10 in the RP-HPLC profiles at the beginning of fermentation. Then, peaks of 1, 2, 3, and 4 showed an increase ranging from 85.5% ± 0.86%, 234.8% ± 1.32%, 144.0% ± 0.96%, and 76.3% ± 0.68%, respectively, whereas further hydrolysis of the other peaks indicated that soymilk residues protein degradation occurred during the 36 h of incubation with A. elegans DCY-1.
Figure 5. Reverse phase high-performance liquid chromatography (RP-HPLC) profiles of fermented okara at different periods.
Figura 5. Perfiles cromatográficos líquidos de alta resolución de fase inversa (RP-HPLC) de okara fermentada en diferentes periodos.
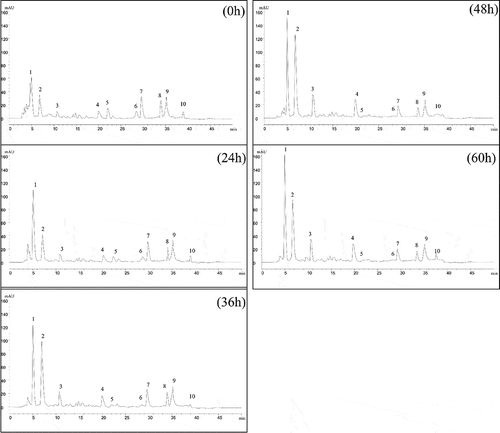
A previous study reported that under the condition of gradient elution, peptide spectra with longer retention time represented components with higher hydrophobicity and larger molecular weights (Wu et al., Citation2015). Therefore, alternation of the HPLC chromatogram indicated the changes of larger/more hydrophobic peptide into smaller/more hydrophilic peptides during fermentation with A. elegans DCY-1. Moreover, complete hydrolysis at peaks 5 and 6 was observed at the late stages of fermentation (48 and 60 h). Previous results (Rui et al., Citation2015; Wu et al., Citation2015) which reported the reduction in the size of several peaks and formation of new peaks during the fermentation process supported our present result, showing that fermentation can induce the hydrolysis of protein fractions due to a developed proteolytic and peptidase system of the microorganism. Therefore, in our study, we assumed that because of the strong proteolytic activity and peptidase system of A. elegans DCY-1 during fermentation, the protein in soymilk residues was hydrolyzed into smaller peptides or free amino acids.
3.7. Correlations of peptide contents with antioxidant and ACE-inhibitory activities
Previous studies have reported that during microbial fermentation, large amounts of proteases are produced, and some of the active peptides liberated by these enzymes exhibit a wide range of physiological properties (Sanjukta et al., Citation2015; Sun et al., Citation2015; Teng et al., Citation2012). In order to establish the contribution of the protease activity of A. elegans DCY-1 and peptide contents to the antioxidant and ACE-inhibitory activities, a fit linear analysis was carried out ( and ). Positive linear relationships were found between the peptide values and protease activities. From the R2 values, we concluded that proteases (R2 = 0.9078) played a major role in the small-size peptide release of A. elegans DCY-1. As shown in , the antioxidant properties, ACE-inhibitory properties, and peptide contents showed significant correlations (p < 0.05). The peptide contents were highly associated with the reducing power (R2 = 0.8578), scavenging ability against DPPH radical (R2 = 0.9863), ABTS·+ radical (R2 = 0.9603), and OH radical (R2 = 0.9399), Fe2+-chelating ability (R2 = 0.8814), and ACE-inhibitory activity (R2 = 0.8574). These results provide strong evidence that the predominant source of antioxidant activity and ACE- inhibitory activity are the peptide compounds in soymilk residues.
Table 2. Relationship of peptide content with antioxidant and ACE-inhibitory activities.
Tabla 2. Relación del contenido de péptidos con las actividades antioxidantes y inhibitorias ACE.
4. Conclusions
The present investigation showed that the okara fermented with A.elegans DCY-1 caused degradation of okara proteins and release of some newly produced small-sized peptides. The antioxidant and ACE-inhibition activity of okara was significantly increased by SSF with A. elegans DCY-1, suggesting that fermented okara can be used as a good source of antioxidants and can be further utilized as a health-promoting food. The enhanced antioxidant effects, as well as ACE inhibitory activity, were probably due to the increased level of peptides during the SSF process. The findings would help obtaining value-added fermented soymilk residues and be advantageous toward the treatment of the waste as well. We aim to further identify the bioactive peptides from the fermented okara in our future studies.
Acknowledgments
This study was supported by the Project Funded by the Jiangsu Collaborative Innovation Center of Meat Production and Processing, Quality and Safety Control.
Disclosure statement
No potential conflict of interest was reported by the authors.
Additional information
Funding
References
- Ajibola, C.F., Fashakin, J.B., Fagbemi, T.N., & Aluko, R.E. (2011). Effect of peptide size on antioxidant properties of African yam bean seed (Sphenostylis stenocarpa) protein hydrolysate fractions. International Journal of Molecular Sciences, 12(10), 6685–6702. doi:10.3390/ijms12106685
- Bhanja, T., Kumari, A., & Banerjee, R. (2009). Enrichment of phenolics and free radical scavenging property of wheat koji prepared with two filamentous fungi. Bioresource Technology, 100(11), 2861–2866. doi:10.1016/j.biortech.2008.12.055
- Chutmanop, J., Chuichulcherm, S., Chisti, Y., & Srinophakun, P. (2008). Protease production by Aspergillus oryzae in solid‐state fermentation using agroindustrial substrates. Journal of Chemical Technology & Biotechnology, 83(7), 1012–1018. doi:10.1002/(ISSN)1097-4660
- Elkhalifa, A.E.O., & Bernhardt, R. (2010). Influence of grain germination on functional properties of sorghum flour. Food Chemistry, 121(2), 387–392. doi:10.1016/j.foodchem.2009.12.041
- Kasai, N., Murata, A., Inui, H., Sakamoto, T., & Kahn, R. (2004). Enzymatic high digestion of soybean milk residue (okara). Journal of Agricultural and Food Chemistry, 52, 5709–5716. doi:10.1021/jf035067v
- Khan, Z.U., Ahmad, S., Mokaddas, E., Chandy, R., Cano, J., & Guarro, J. (2008). Actinomucor elegans var. kuwaitiensis isolated from the wound of a diabetic patient. Antonie van Leeuwenhoek, 94(3), 343–352. doi:10.1007/s10482-008-9251-1
- Lee, B.-H., Lai, Y.-S., & Wu, S.-C. (2015). Antioxidation, angiotensin converting enzyme inhibition activity, nattokinase, and antihypertension of Bacillus subtilis (natto)-fermented pigeon pea. Journal of Food and Drug Analysis, 23(4), 750–757. doi:10.1016/j.jfda.2015.06.008
- Lee, I.-H., Hung, Y.-H., & Chou, -C.-C. (2008). Solid-state fermentation with fungi to enhance the antioxidative activity, total phenolic and anthocyanin contents of black bean. International Journal of Food Microbiology, 121(2), 150–156. doi:10.1016/j.ijfoodmicro.2007.09.008
- Li, B., Qiao, M., & Lu, F. (2012). Composition, nutrition, and utilization of okara (soybean residue). Food Reviews International, 28(3), 231–252. doi:10.1080/87559129.2011.595023
- Lim, J.-Y., Kim, J.J., Lee, D.S., Kim, G.H., Shim, J.-Y., Lee, I., & Imm, J.-Y. (2010). Physicochemical characteristics and production of whole soymilk from Monascus fermented soybeans. Food Chemistry, 120(1), 255–260. doi:10.1016/j.foodchem.2009.10.017
- Liu, J., Jia, L., Kan, J., & Jin, C.-h. (2013). In vitro and in vivo antioxidant activity of ethanolic extract of white button mushroom (Agaricus bisporus). Food and Chemical Toxicology, 51, 310–316. doi:10.1016/j.fct.2012.10.014
- Lowry, O.H., Rosebrough, N.J., Farr, A.L., & Randall, R.J. (1951). Protein measurement with the Folin phenol reagent. Journal of Biological Chemistry, 193(1), 265–275.
- Mizumoto, S., Hirai, M., & Shoda, M. (2006). Production of lipopeptide antibiotic iturin A using soybean curd residue cultivated with Bacillus subtilis in solid-state fermentation. Applied Microbiology and Biotechnology, 72(5), 869–875. doi:10.1007/s00253-006-0389-3
- Muhialdin, B.J., Hassan, Z., Bakar, F.A., & Saari, N. (2016). Identification of Antifungal Peptides Produced by Lactobacillus plantarum IS10 grown in the MRS broth. Food Control, 59, 27–30. doi:10.1016/j.foodcont.2015.05.022
- Ortiz, S.E.M., & An, M.C. (2000). Analysis of products, mechanisms of reaction, and some functional properties of soy protein hydrolysates. Journal of the American Oil Chemists’ Society, 77(12), 1293–1301. doi:10.1007/s11746-000-0204-4
- Pownall, T.L., Udenigwe, C.C., & Aluko, R.E. (2010). Amino acid composition and antioxidant properties of pea seed (Pisum sativum L.) enzymatic protein hydrolysate fractions. Journal of Agricultural and Food Chemistry, 58(8), 4712–4718. doi:10.1021/jf904456r
- Rashad, M.M., Mahmoud, A.E., Abdou, H.M., & Nooman, M.U. (2011). Improvement of nutritional quality and antioxidant activities of yeast fermented soybean curd residue. African Journal of Biotechnology, 10(28), 5504–5513.
- Rui, X., Wen, D., Li, W., Chen, X., Jiang, M., & Dong, M. (2015). Enrichment of ACE inhibitory peptides in navy bean (Phaseolus vulgaris) using lactic acid bacteria. Food & Function, 6(2), 622–629. doi:10.1039/C4FO00730A
- Sanjukta, S., Rai, A.K., Muhammed, A., Jeyaram, K., & Talukdar, N.C. (2015). Enhancement of antioxidant properties of two soybean varieties of Sikkim Himalayan region by proteolytic Bacillus subtilis fermentation. Journal of Functional Foods, 14, 650–658. doi:10.1016/j.jff.2015.02.033
- Sasipriya, G., & Siddhuraju, P. (2012). Effect of different processing methods on antioxidant activity of underutilized legumes, Entada scandens seed kernel and Canavalia gladiata seeds. Food and Chemical Toxicology, 50(8), 2864–2872. doi:10.1016/j.fct.2012.05.048
- Shahidi, F., & Zhong, Y. (2015). Measurement of antioxidant activity. Journal of Functional Foods, 18, 757–781. doi:10.1016/j.jff.2015.01.047
- Singh, H.B., Singh, B.N., Singh, S.P., & Nautiyal, C.S. (2010). Solid-state cultivation of Trichoderma harzianum NBRI-1055 for modulating natural antioxidants in soybean seed matrix. Bioresource Technology, 101(16), 6444–6453. doi:10.1016/j.biortech.2010.03.057
- Sun, H., Yao, X., Wang, X., Wu, Y., Liu, Y., Tang, J., & Feng, J. (2015). Chemical composition and in vitro antioxidant property of peptides produced from cottonseed meal by solid-state fermentation. CyTA-Journal of Food, 13(2), 264–272. doi:10.1080/19476337.2014.948072
- Teng, D., Gao, M., Yang, Y., Liu, B., Tian, Z., & Wang, J. (2012). Bio-modification of soybean meal with Bacillus subtilis or Aspergillus oryzae. Biocatalysis and Agricultural Biotechnology, 1(1), 32–38. doi:10.1016/j.bcab.2011.08.005
- Torino, M.I., Limón, R.I., Martínez-Villaluenga, C., Mäkinen, S., Pihlanto, A., Vidal-Valverde, C., & Frias, J. (2013). Antioxidant and antihypertensive properties of liquid and solid state fermented lentils. Food Chemistry, 136(2), 1030–1037. doi:10.1016/j.foodchem.2012.09.015
- Wu, H., Rui, X., Li, W., Chen, X., Jiang, M., & Dong, M. (2015). Mung bean (Vigna radiata) as probiotic food through fermentation with Lactobacillus plantarum B1-6. LWT-Food Science and Technology, 63(1), 445–451. doi:10.1016/j.lwt.2015.03.011
- Xiao, Y., Xing, G., Rui, X., Li, W., Chen, X., Jiang, M., & Dong, M. (2015). Effect of solid-state fermentation with Cordyceps militaris SN-18 on physicochemical and functional properties of chickpea (Cicer arietinum L.) flour. LWT-Food Science and Technology, 63(2), 1317–1324. doi:10.1016/j.lwt.2015.04.046
- Xiao, Y., Zhang, Q., Miao, J., Rui, X., Li, T., & Dong, M. (2015). Antioxidant activity and DNA damage protection of mung beans processed by solid state fermentation with Cordyceps militaris SN-18. Innovative Food Science & Emerging Technologies, 31, 216–225. doi:10.1016/j.ifset.2015.06.006
- Yang, J.-H., Mau, J.-L., Ko, P.-T., & Huang, L.-C. (2000). Antioxidant properties of fermented soybean broth. Food Chemistry, 71(2), 249–254. doi:10.1016/S0308-8146(00)00165-5
- Zhang, Y., Zhang, H., Wang, L., Guo, X., Qi, X., & Qian, H. (2011). Influence of the degree of hydrolysis (DH) on antioxidant properties and radical-scavenging activities of peanut peptides prepared from fermented peanut meal. European Food Research and Technology, 232(6), 941–950. doi:10.1007/s00217-011-1466-0
- Zhu, Y.P., Fan, J.F., Cheng, Y.Q., & Li, L.T. (2008). Improvement of the antioxidant activity of Chinese traditional fermented okara (Meitauza) using Bacillus subtilis B2. Food Control, 19(7), 654–661. doi:10.1016/j.foodcont.2007.07.009