ABSTRACT
Characterization, antioxidant activities and hepatoprotective effects of polysaccharides from pre-pressing separation apple (Fuji) peel pomace were investigated in this study. Gel permeation chromatography indicated that apple peel polysaccharides (APPs) were a kind of heteropolysaccharides composed of three fractions with homogenous polarity. Both GC–MS and HPLC analysis showed that arabinose, galactose and galacturonic acid were the main monosaccharides in APP. In vitro antioxidant activities of APP were characterized. Furthermore, administration of APP (450 mg/kg bw) in mice significantly decreased the CCl4-induced elevations of serum alanine aminotransferase, asparate aminotransferase and lactic dehydrogenase activities, and hepatic malondialdehyde level and inhibited the decreases in superoxide dismutase and glutathione peroxidase activities caused by CCl4. Histopathological observation further confirmed that APP could protect the liver tissues from CCl4-induced histological alternation. These findings indicate that APP has the antioxidant and hepatoprotective potentials and provide a scientific basis for comprehensive utilization of pre-pressing separation apple peel pomace.
RESUMEN
En este estudio se investigaron la caracterización, la actividad antioxidante y los efectos hepatoprotectores de los polisacáridos de la separación del orujo de pela de manzana (Fuji) previamente prensado. La cromatografía de permeación en gel indicó que los polisacáridos de la pela de manzana (APP) eran un tipo de heteropolisacáridos compuestos de tres fracciones con polaridad homogénea. Los dos análisis GC-MS y HPLC mostraron que la arabinosa, la galactosa y el ácido galacturónico fueron los principales monosacáridos en APP. Se caracterizó la actividad antioxidante in vitro de APP. Además, la administración de APP (450 mg/kg bw) en ratones disminuyó significativamente las elevaciones inducidas con CCl4 de la alanina aminotransferasa sérica, el aspartato aminotransferasa, la actividad lactato deshidrogenasa y el nivel hepático de malondialdehído, además inhibió la disminución de la actividad de superóxido dismutasa y glutatión peroxidasa causada por CCl4. Posteriormente la observación histopatológica confirmó que APP podría proteger los tejidos del hígado de la alternación histológica inducida con Cl4. Estos hallazgos indican que APP tiene potencial antioxidante y hepatoprotector, además proporciona una base científica para una utilización integral de la separación de orujo de pela de manzana previamente prensado.
Introduction
Apple is the pomaceous fruit of apple tree and known to be an important source of bioactive nutrition in human’s diets (Sun, Guo, Fu, Li, & Li, Citation2013). It has been revealed that apple extracts, such as polyphenols and polysaccharides, are beneficial to human health and have a potential of preventing cardiovascular diseases (Chai et al., Citation2012). Apple juice is one of the most popular soft drinks in the world made by maceration and pressing of apple fruits. According to the manufacturers and end products, apple fruits may be processed in different ways before pressing. Commonly, apples are directly pressed for juice after broken, so some parts of the fruits (peel, stalk and seed) are mixed and pressed together with flesh, which may cause adverse effects on juice quality, such as pesticide residues, colour burn and off-flavour. Besides, after the traditional pressing process, all the waste residues, including peel, flesh, seed and stalk pomace, are mixed together, which decreases the recycling values of pomace as fodders, food additives and research materials. In order to solve these issues and improve juice quality, ‘pre-pressing separation technology’ is being introduced to juice industry with the design of ‘pre-pressing separator’. This separator is designed to scrape apples to remove peel, seed and stalk on the base of the differences in toughness of different fruit parts, followed by breaking and pulping flesh. Under the interception of screen mesh, the flesh pulp can be separated from other parts before pressing process (), and the juice is only from flesh part. Therefore, through this separation technique, the potential issues mentioned above can be avoided as much as possible. After the complete juicing process, apple juice with lighter colour than the traditional juice can be obtained (), and the flesh pomace and the mixtures of peel, seed and stalk pomace can be obtained separately. The peel pomace with very little attached flesh can be sequentially isolated by centrifugation after adding water (). Thereby, the ‘pure apple peel’ can be used to study its comprehensive utilization values.
Figure 1. Pre-pressing separation technology. Flesh pulp (a), apple juices obtained by traditional pressing method and pre-pressing separation technology, respectively (b), and apple peel pomace (c).
Figura 1. Tecnología de separación con previo prensado. Pulpa (a), zumos de manzana obtenidos con el método tradicional de prensado y la tecnología de separación con previo prensado, respectivamente (b) y orujo de piel de manzana (c).
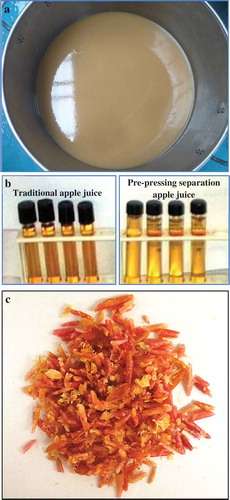
In recent years, various plant polysaccharides have been shown to play an important role in reducing the risk of chronic diseases through their potential antioxidant, anticancer, antibacterial activities (Nada, Omara, Abdel-Salam, & Zahran, Citation2010; Ren, Perera, & Hemar, Citation2012). It is well known that the liver is a vital organ of animals in terms of biotransformation and detoxification. There are increasing reports suggesting in vivo free radicals or reactive oxygen species (ROS), such as superoxide anion radical (O2•−) and hydroxyl radical (HO•), are one of the factors inducing chronic liver diseases (Hsiao et al., Citation2003; Yang, Dong, & Ren, Citation2011). Also, carbon tetrachloride (CCl4), a widely applied hepatotoxin, has been used in establishing a liver-injured model to screen hepatoprotective medicines (Nada et al., Citation2010). However, there are limited numbers of hepatoprotective medicines in the commercial market, and some of them have been reported to have adverse effects, especially for chronic or subchronic administration (Tian, Zhao, Guo, & Yang, Citation2011). Therefore, natural antioxidant phytochemicals have recently been shown a substantial increase as alternative therapies of liver diseases because of better effectiveness and fewer side effects (Jiang et al., Citation2013). Recently, antioxidant extracts from Pink Lady apples were found to possess hepatoprotective effects against CCl4-induced liver injury in mice (Yang, Li, Wang, & Wu, Citation2010; Yang, Yang, Guo, Jiao, & Zhao, Citation2013). Fuji apple is one of the most popular apple cultivars in the world (Murata, Tsurutani, Tomita, Homma, & Kaneko, Citation1995). Actually, apple peel pomace is a potential polysaccharides source because there are large amount of peel pomace produced as juice industry wastes every year, especially in China. Furthermore, it becomes more easy to obtain apple peel pomace than before by use of pre-pressing separation technology in juicing factories. As there is very little flesh attached on peel, the properties of polysaccharides from peel pomace can be studied without the influence of polysaccharides from flesh pomace. To our knowledge, there are few literatures available to address the compositional features and hepatoprotective activity of Fuji apple peel polysaccharides (APPs), especially for the apple peel obtained from pre-pressing separation.
Therefore, the objectives of this study were to identify the monosaccharide composition and primary molecular structure of polysaccharides from Fuji apple peel. Then, in vitro antioxidant activities and in vivo hepatoprotective effects against CCl4-induced hepatic damage in mice were further investigated. Besides, we considered this study addressing an interdisciplinary between food engineering and food nutrition, as well as a comprehensive application of juicing manufacturing wastes.
Materials and methods
Materials and reagents
The apples (Fuji) were harvested in October 2015 in Liquan, Shaanxi province of China. Monosaccharides, including d-mannose, l-rhamnose, d-glucuronic acid, d-galacturonic acid, d-glucose, d-xylose, d-galactose, l-arabinose, d-fucose, mannitol and ascorbic acid (Vc), were obtained from Sigma Co. (St. Louis, US). Biphenydicarboxylate bill (BP) was obtained from Zhejiang Wanbang Pharmaceutical Co., Ltd. (Wenling, China). Haematoxylin and eosin (H & E) were the products of Shanghai Lanji Technological Development Co., Ltd. (Shanghai, China). CCl4 was purchased from Tianjin Tianli Chemical Reagent Co. (Tianjin, China). Test kits for alanine aminotransferase (ALT), asparate aminotransferase (AST), lactic dehydrogenase (LDH), superoxide dismutase (SOD), glutathione peroxidase (GSH-Px) and malonaldehyde (MDA) were purchased from Nanjing Jiancheng Bioengineering Institute (Nanjing, China). Trifluoroacetic acid, triethylamine and 1-phenyl-3-methyl-5-pyrazolone (PMP) were obtained from Merck Co. (Darmstadt, Germany). HPLC-grade acetonitrile, methanol, potassium ferricyanide [K3Fe(CN)6] and trichloroacetic acid were purchased from Sigma Co. (Steinheim, Germany). 1,1-Diphenyl-2-picryl-hydrazyl (DPPH•), nitroblue tetrazolium, nicotinamide adenine dinucleotide and phenazine methosulphate were products of Applichem Co. (Darmstadt, Germany). All other reagents were of analytical grade.
Isolation of APP
The fresh apples picked up in the field were transferred to Dinghe machinery manufacturing Co., Ltd. (Xi’an, China). Then, the washed apples were uploaded into the pre-pressing separator according to the operating instruction. As mentioned above, the flesh pulp was separated from the mixtures of peel, seed and stalk pomace. Then, the mixtures were collected for further separation of peel pomace. To do this, water (1:1, v/w) was added to a small amount of mixtures, followed by centrifugation at 4000 × g for 15 min. The peel pomace was easily obtained from the upper layer of the centrifuge tube because of the lower density for peel pomace than other parts (stalk and seed). Finally, the peel pomace was oven-dried for further study.
The obtained dry peel was grated and bleached to suppress the activities of polyphenol oxidases for lighter colour of polysaccharides. Then, the tissue homogenate was lyophilized. The polysaccharides of apple peel were extracted by the reported method with some modifications (Lv et al., Citation2009). Briefly, the pomace was extracted with 95% ethanol (1:10, w/v) refluxing at 80°C for 4 h to remove the phenolic and lipophilic components. Next, the pre-processed pomace was dried and then extracted with hot water (1:30, w/v) at 80°C for 2 h, followed by filtration. The residues were extracted again for two more cycles, and the combined extraction solution was condensed to approximately quarter of the original volume by use of a rotary vacuum evaporator under reduced pressure, followed by centrifugation at 4000 × g for 15 min. Then, the supernatant was collected and precipitated with triple volumes of 95% (v/v) ethanol solution at 4°C. The polysaccharides were obtained by centrifuging at 4000 × g for 15 min, followed by dissolved in distilled water and dialyzed intensively (cut-off Mw 8 kDa) for 3 days against distilled water to remove impurities, such as soluble monosaccharides, inorganic salts and polyphenols. Subsequently, the residual portion in the dialysis bag was deproteinized by the process of freeze–thaw, repeated 10 times, followed by filtration. Finally, the supernatant was condensed and lyophilized to obtain total APPs.
Characterization of APP
Determination of contents of carbohydrates, polyphenols, proteins and nucleic acid
The content of total carbohydrates was determined using a phenol–sulphuric acid colorimetric method with glucose as a standard (Dubois, Gilles, Hamilton, Rebers, & Smith, Citation1956). The content of total polyphenols was determined using spectrophotometry as reported before with gallic acid as a standard (Singleton, Orthofer, & Lamuela-Raventos, Citation1999). UV spectrum was recorded in the range of 190–400 nm to measure the potential presences of proteins and nucleic acid as the maximum absorption wavelengths for the two compounds were 280 and 260 nm, respectively (Jia et al., Citation2015).
Determination of monosaccharide composition by HPLC
The monosaccharide composition of APP was analysed using HPLC method established by Yang et al. (Citation2013) with some modifications. Specifically, 20 mg APP was hydrolysed in a 5-mL sealed ampoule by the addition of 2.0 mL of 3 M trifluoroacetic acid (TFA) at 95°C for 8 h to fully release the constitutive monosaccharides. The hydrolysate mixture was centrifuged at 4000 × g for 5 min at room temperature, and the supernatant was evaporated to dry under reduced pressure followed by dissolved with 1.0 mL distilled water. Subsequently, 100 µL monosaccharides solution obtained was mixed with 200 µL of 0.5 M methanol solution of PMP and 300 µL of 0.3 M NaOH solution for derivatization to obtain a strengthen UV absorption. The mixture was then incubated at 70°C for 1 h before neutralization with 300 µL of 0.3 M HCl. The resulting solution was extracted with chloroform and repeated two more cycles. Finally, the aqueous layer was filtered through a 0.45-µm membrane for HPLC analysis.
The analysis of PMP-derived monosaccharides was performed on a Shimadzu® LC-2010A HPLC system. The analytical column was a Venusil® RP C18 column (4.6 mm × 250 mm, I.D., 5 µm). The mobile phases consisted of acetonitrile (A) and 3.3 mM KH2PO4/3.9 mM TEA buffer containing 10% acetonitrile (B) using a gradient elution: 0–4 min, 94% B; 4–9 min, 94–88% B; 9–30 min, 88% B at a flow rate of 1.0 mL/min. The column was maintained at 37°C.
Determination of monosaccharide composition by GC–MS
Monosaccharide composition of APP was also analysed using GC–MS method. Specifically, APP (~10 mg) was hydrolysed in 1 mL of 2 M TFA at 120°C for 2 h. Then, the solution was dried under a stream of nitrogen gas, followed by a derivatization procedure according to one previously reported method (Sassaki et al., Citation2008). Briefly, 1 mL of 0.5 M NH4OH was added and mixed thoroughly for 10 min. Then, 1 mg of NaBH4 was added and stirred for 5 h. The solution was dried under nitrogen gas. The residue was dissolved in 1 mL of 0.5 M HCl–MeOH and heated at 100°C for 15 min and then dried under nitrogen gas. 1 mL of pyridine–acetic anhydride was added and heated at 95°C for 30 min. The solution was then dried under nitrogen gas and dissolved in 1 mL MeOH. The procedure of GC–MS was conducted on an Agilent® GC system (7890A, California, USA) coupled with an Agilent® MS system (5975C, California, USA). The separation was performed according to one previously reported method (Cheong et al., Citation2016) on a DB-5 column (30 m × 0.25 mm×0.25 µm). The column temperature was held at 165°C for 7 min, programmed at 5°C/min to 185°C and held for 5 min, then at 4°C/min to 200°C, finally at 20°C/min to 280°C and held for 2 min. The injection volume was 2 µL and the split ratio was 1:50. A flow rate of 1.0 mL/min helium gas was used as the carrier gas. The mass spectrometer was operated in electron-impact mode (70 eV) at 2.89 s per scan from the range of 10–550 amu. The temperatures of the interface and ionization source were 250 and 280°C, respectively. Monosaccharide standards mix including rhamnose, fucose, arabinose, xylose, galacturonic acid, glucuronic acid, inositol (internal standard), mannose, glucose and galactose was prepared and analysed in the same way.
Fourier transform infrared analysis
The infrared spectrum of APP was performed using a Bruker® Fourier transform infrared (FT-IR) spectrophotometer (EQUINX 55, US). A volume of 4 mg APP was mixed with KBr powder and ground together, followed by pressed into a 1-mm pellet for measurement. The FT-IR of APP was recorded in the range of 4000–500 cm−1 (You et al., Citation2013).
Separation of APP by gel permeation chromatography
To characterize the homogeneity of APP, gel permeation columns were applied to separate it according to the molecular size and polarity. The purified APP was separated sequentially by Cellulose DEAE-52 and Sephadex G-150 chromatography columns based on the reported method with some modifications (Jia et al., Citation2015; Ye, Wang, Zhou, Liu, & Zeng, Citation2008). Briefly, 0.5 g purified polysaccharides were dissolved in 10 mL distilled water and then centrifuged at 4000 × g for 15 min. The supernatant was subjected onto a Cellulose DEAE-52 column (2.5 cm×60 cm). Subsequently, the column was eluted gradiently with distilled water and NaCl solution (0.1–0.5 M) at a flow rate of 0.8 mL/min. The elutions were collected automatically tube by tube, and the carbohydrate contents were determined using a phenol–sulphuric acid method (Dubois et al., Citation1956). Then, the separated fractions were collected, condensed, dialyzed before further purified on a Sephadex G-150 column (1.6 cm×60 cm) eluted with distilled water at a flow rate of 0.5 mL/min. The elutions through the Sephadex G-150 column were collected automatically tube by tube, and the carbohydrate contents were determined using the phenol–sulphuric acid method above. Finally, the tubes with high contents of respective fractions were collected, condensed, dialyzed and lyophilized.
Determination of average molecular distributions of three APP fractions
The molecular distributions of three APP fractions were measured with high performance size exclusion chromatography by use of a Shimadzu® LC-2010A HPLC system equipped with a Shodex® size exclusion chromatography column (SB-804 HQ). A volume of 20 µL of 2 mg/mL respective APP fraction was injected into the column and separated using distilled water at a flow rate of 0.8 mL/min. The calibration curve was made with the Dextran standards with known molecular weights (Shao, Chen, & Sun, Citation2014).
In vitro antioxidant activities of APP
The antioxidant activities of APP were demonstrated by reducing powder (Yang et al., Citation2013) and three radicals scavenging methods reported previously, including DPPH•, O2•−and HO• (Chun, Kim, & Lee, Citation2003; Ye et al., Citation2008).
Animal and treatment
Kunming male mice (weight 18–22 g, around 1 month of age) were allowed free access to distilled water and rodent chow (40% corn flour, 26% wheat flour, 10% bran, 10% fish meal, 10% bean cake, 2% mineral, 1% coarse meal and 1% vitamin complex). All the mice were housed together in a plastic cage (20 × 40 cm) in a specific pathogen-free animal room with a 12-h light/dark cycle at a temperature of 22 ± 2°C and a humidity of 60 ± 5%. The mice were allowed to acclimatize to the environment for 3 days before the experiments, and all the related studies were conducted according to the Regulation of Experimental Animal Administration issued by the State Committee of Science and Technology of People’s Republic of China.
After environment adaption for 3 days, mice were divided into 6 groups with 10 mice selected at random in each group. For the normal and CCl4-intoxicated groups, mice were provided 0.5 mL of physiological saline once a day. For the BP (positive) group, mice were given 0.5 mL of 450 mg/kg bw BP once a day. For the polysaccharide-treated group, mice were received 0.5 mL of respective 150, 300 and 450 mg/kg bw APP once a day. All the administrations were conducted for seven consecutive days. After that, all the mice except the normal group were given a dose of 0.3 mL of 0.8% CCl4/peanut oil mixture, while the normal group was given 0.3 mL peanut oil alone. Then, all the mice were fasted with only water provided for 48 h. The mice were weighted and blood samples were collected from the orbit. After the animals were decapitated and exsanguinated, the livers were immediately taken out and weighted after rinsing by physiological saline (ice-cold) and then stored at −80°C before use. The blood samples were centrifuged and the serum was stored at 4°C before use. Hepatosomatic index (HI) was calculated as the ratio of body weight to the corresponding liver weight.
Assay for biochemical index in serum and liver
Determination of the activities of ALT, AST and LDH in serum
The serum was separated after clot by centrifugation at 4°C. The activities of ALT, AST and LDH were measured according to the method of Reitman–Frankel (1957) by commercially available assay kits. All the experiments were conducted in triplicates, and the average counts were obtained from each individual sample.
Determination of hepatic MDA, GSH-Px and SOD levels
A volume of 1.0 g each liver sample was mixed with 9 mL cold physiological saline and then homogenized, followed by centrifugation at 1400 × g for 10 min. The content of MDA in the supernatant was measured by the thiobarbituric acid reaction method, and the activities of GSH-Px and SOD were assayed as described by the manufacturer’s instruction of commercially available test kits. Total protein contents were determined by Coomassie Brilliant Blue with bovine serum albumin as a standard. All the experiments were conducted in triplicates, and the average counts were obtained from each individual sample.
Histopathological observation
Some parts of liver tissues were mixed with a 4% paraformaldehyde solution at 4°C for 24 h and then embedded in paraffin wax (~5 µm) and stained with H & E solution. The slides were observed under an Olympus® light microscope and photographed.
Statistical analysis
The data were presented as the mean ± standard deviations of triplicate measurements and evaluated by one-way analysis of variance using SPSS 18.0 Statistics (SPSS Inc., Chicago, US). The mean values were compared using Dunnett’s t-test at 95% or 99% significant level (p < 0.05 or p < 0.01).
Results and discussion
Chemical composition of APP
The crude APP was obtained from pre-pressing separation apple peel using traditional hot water extraction and ethanol precipitation. After deproteinization and dialysis, the yield of APP was 9.53% (w/w) based on the weight of dried peel pomace. The content of total carbohydrate in APP was determined as 83.75% by use of the phenol–H2SO4 method. Reaction was hardly observed between Folin–Ciocalteu reagent and APP, indicating that there existed no phenolic components. Besides, little absorbance at 280 and 260 nm in the UV spectrum indicated the absence of proteins and nucleic acid in APP, whereas the absorbance at ~190 nm suggested that the main component in APP was polysaccharides (You et al., Citation2011). In addition, no starch was detected in APP because no reaction was observed after the addition of I2/KI solution.
Monosaccharide composition of APP
Monosaccharide composition of APP by HPLC
The monosaccharide composition and contents in APP were determined using HPLC method and are shown in . Nine kinds of standard monosaccharides derived with PMP could be separated well through the column within 25 min. As shown, APP was composed of mannose, rhamnose, glucuronic acid, galacturonic acid, glucose, xylose, galactose and arabinose, and their corresponding mole percentages were 10.5%, 9.2%, 5.3%, 18.5%, 6.5%, 7.2%, 20.3% and 21.5% of all the quantitative monosaccharides, respectively, which was similar to the results obtained by Yang et al. (Citation2013), even though the polysaccharides tested were from two different varieties (Fuji and Pink Lady, respectively). Both kinds of polysaccharides contained some parts of pectin. The HPLC method established in our study could simultaneously separate a number of monosaccharides both for neutral and uronic sugars with a short analytical time.
Figure 2. The HPLC chromatograms of PMP derivatives of 9 standard monosaccharides (a) and component monosaccharides released from APP (b). Peaks: 1 mannose, 2 rhamnose, 3 glucuronic acid, 4 galacturonic acid, 5 glucose, 6 xylose, 7 galactose, 8 arabinose, 9 fucose (internal standard).
Figura 2. Cromatogramas HPLC de los derivados de PMP de 9 monosacáridos estándar (a) y componentes de monosacáridos liberados de APP (b). Picos: 1 manosa, 2 ramnosa, 3 ácido glucurónico, 4 ácido galacturónico, 5 glucosa, 6 xilosa, 7 galactosa, 8 arabinosa, 9 fucosa (estándar interno).
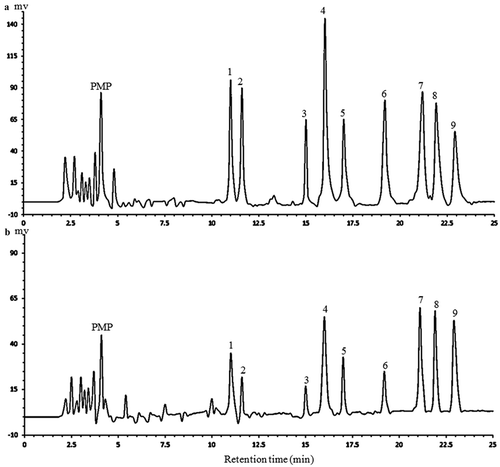
Monosaccharide composition of APP by GC–MS
To encourage the monosaccharide composition of APP, GC–MS was performed and the chromatography is presented in . As shown, the monosaccharide composition of APP determined by GC–MS was consistent with that determined by HPLC (), with the mole percentages of mannose, rhamnose, glucuronic acid, galacturonic acid, glucose, xylose, galactose and arabinose in total monosaccharides of 12.4%, 8.1%, 2.0%, 13.2%, 8.2%, 10.1%, 21.0% and 24.3%, respectively. Obviously, the order of individual mole percentages of monosaccharides in APP by GC–MS method was essentially same as that by HPLC, in which galactose and arabinose accounted for the higher mole percentages than other monosaccharides. This indicates that the analysis of monosaccharide composition in APP by GC–MS and HPLC methods was shown comparable results; therefore, the two methods may be combined together in determining the mole percentages of monosaccharides in some certain plant polysaccharides. Furthermore, the contents (mass percentage) of mannose, rhamnose, glucuronic acid, galacturonic acid, glucose, xylose, galactose and arabinose in APP were determined to be 11.0%, 6.5%, 1.9%, 12.5%, 7.2%, 7.5%, 18.5% and 17.8%, respectively, by GC–MS. It has been reported that the bioactivity of polysaccharides is highly related to the monosaccharide composition and contents (Yang et al., Citation2013). Besides, polysaccharides with more uronic acids have higher bioactivities due to the modified properties of polysaccharides, such as solubility (Tian, Zhao, Guo, & Yang, Citation2011). The total content of uronic acids (including both galacturonic acid and glucuronic acid) in APP was 14.4%, suggesting that APP may have a potential to be developed as one important plant extracts with biological responses.
Figure 3. Typical chromatograms of monosaccharides in monosaccharide standards mix (a) and APP (b) analysed by GC–MS. Peaks are 1 rhamnose, 2 fucose, 3 arabinose, 4 xylose, 5 galacturonic acid, 6 glucuronic acid, 7 inositol (internal standard), 8 mannose, 9 glucose, 10 galactose.
Figura 3. Cromatogramas típicos de monosacáridos en mezcla estándar de monosacáridos (a) y APP (b) analizados mediante GC-MS. Los picos son 1 ramnosa, 2 fucosa, 3 arabinosa, 4 xilosa, 5 ácido galacturónico, 6 ácido glucurónico, 7 inositol (estándar interno), 8 manosa, 9 glucosa, 10 galactosa.
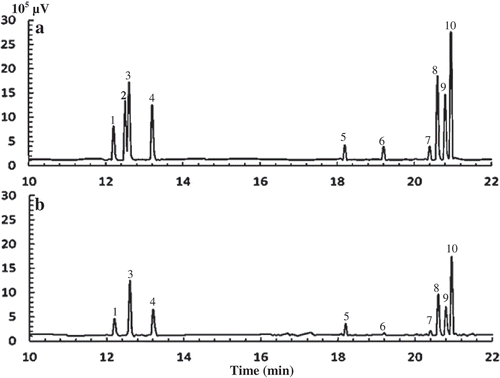
FT-IR spectrum of APP
To characterize APP composition more precisely, the characteristic FT-IR spectrum was performed in the wavelength range of 4000–500 cm−1 (). Specifically, the intense broad peak at 3432.12 cm−1 was characteristic stretching vibration of hydroxyl groups (Zhao, Yang, Yang, Jiang, & Zhang, Citation2007), and the weak absorption peak at 2917.55 cm−1 was caused by the stretching vibration of the C–H bonds (You et al., Citation2013). As reported, the characteristic absorption peak at 1342.42 cm−1 was ascribed to the bending vibration of C–H (Ma et al., Citation2013; Zheng et al., Citation2014). In addition, the stretching absorption peak at 1613.42 cm−1 along with the peak at 1419.59 cm−1 indicated the presence of COO− (Lee et al., Citation2013; Tian, Zhao, Guo, & Yang, Citation2011), which conforms to the presence of uronic acids detected by HPLC method. Each particular polysaccharide has specific absorptions in the region of 1200–1000 cm−1; therefore, this is often considered as the fingerprints of polysaccharides because it allows the characterization of the position and intensity of special bonds in each polysaccharide molecules (Zhang et al., Citation2010). The characteristic peaks at 1038.34 and 1079.94 cm−1 were conformed to the stretching vibration of C–OH side groups and C–O–C glycosidic bond, indicating that there existed pyranose unit in APP (Zhao et al., Citation2014).
Separation of APP by gel permeation chromatography
The purified APP was initially separated through a Cellulose DEAE-52 anion-exchange column. As a result, three main elution peaks were obtained with distilled water, 0.1 and 0.2 M NaCl solutions, respectively (), indicating that APP was a kind of heteropolysaccharides. The three eluted fractions were collected and concentrated for further purification by gel-filtration chromatography using a Sephadex G-150 column. The column was eluted with distilled water and the resulting elutions were collected. It is shown in that each eluted fraction obtained through DEAE-52 column generated one single elution peak through Sephadex G-150 column, indicating that three polysaccharides components with homogeneous polarity were obtained through the two columns. The respective molecular weights for the three fractions (APP-1, APP-2 and APP-3) were 201, 393 and 301 kDa (), using a regression equation lg Mw = 9.0356 − 0.5729tR (R2 = 0.9968).
Figure 5. Gel permeation chromatograms and molecular distributions of three APP fractions. The gradient elution curve of purified APP on a Cellulose DEAE-52 column with 0–0.5 M NaCl solution (a); the elution curves of APP-1 (b), APP-2 (c) and APP-3 (d) on a Sephadex G-150 column with distilled water; the average molecular distributions of APP-1 (e), APP-2 (f) and APP-3 (g).
Figura 5. Cromatogramas de permeación en gel y distribuciones moleculares de tres fracciones de APP. La curva de elución en gradiente de APP en la columna Celulosa DEAE-52 con solución 0–0,5 M de NaCl (a); Las curvas de elución de APP-1 (b), APP-2 (c) y APP-3 (d) en la columna Sephadex G-150 con agua destilada; Las distribuciones moleculares medias de APP-1 (e), APP-2 (f) y APP-3 (g).
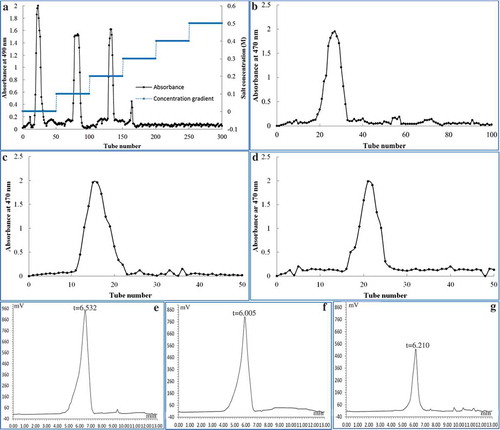
In vitro antioxidant activity of APP
The antioxidant activities of plant extracts are highly related to their reducing powers (Chen, Ma, Liu, Liao, & Zhao, Citation2012). It is shown in that the reducing power of APP increased with the concentration increasing, indicating that the reducing effect was concentration dependant in the range of 0.25–2.0 mg/mL. Even though, the reducing power of APP was weaker than that of Vc, a well-recognized reducing agent. DPPH• is a stable free-radical compound that is widely applied to evaluate the ability of an antioxidant to scavenge-free radicals. The DPPH• scavenging rate of APP is presented in . APP showed a weaker scavenging effect than Vc, which was also correlated positively with the concentration in the range of 0.25–5.0 mg/mL. Hydroxyl radical (HO•) is one of the most ROS and mainly responsible for the oxidative injury of biomolecules such as lipids, proteins and DNA (Spencer et al., Citation1994). The HO• scavenging activity of APP is shown in . Although the scavenging activity was around 50% at 0.5 mg/mL, it remained lower than that of Vc in the concentration range measured. Hence, APP seemed to be a moderate HO• scavenger. Furthermore, superoxide anion radical (O2•−) is less reactive but has a longer half-life than some other radicals. Numerous biological and photochemical reactions can generate this free radical that may subsequently form secondary radicals including hydrogen peroxide and hydroxyl radical through dismutation reaction and lead to tissue damages and even various cancers (Liu, Wang, Pang, Yao, & Gao, Citation2010). Therefore, the determination of scavenging effect on O2•− is a necessary way to elucidate the mechanism of antioxidant activity (Wade, Jackson, Highton, & Vanrij, Citation1987). As shown in , the O2•− scavenging activity of APP was concentration dependant as well, and the activity may not be developed as potentially as Vc. Generally, the weaker antioxidant activity of APP than both Vc () and apple peel polyphenols (reported by Wolfe, Wu, and Liu, Citation2003) indicated that APP might provide hydrogen protons more hardly than both the compounds when contacting with free radicals (Schulz, Citation1991).
Effects of APP on body weight and HI
It has been reported that in vivo CCl4-induced liver damage is the mechanism of lipid peroxidation (Altas, Kizil, Kizil, Ketani, & Haris, Citation2011; Hsiao et al., Citation2003). In this study, the hepatoprotective effects of APP were further measured in mice model. The effects of APP on the body weight, liver weight and for experimental mice were summarized in . For the CCl4-intoxicated mice group, significant increases in liver weight and HI were observed compared to the normal mice (p < 0.05 and p < 0.01, respectively). Although there was no significant difference in the changes of body weights, the administration of APP at the high doses of 300 and 450 mg/kg bw resulted in significant decreases in liver weights and HIs, relative to the CCl4 group, respectively (p < 0.05 and p < 0.01, respectively). Pre-treatment with positive 450 mg/kg bw of BP showed similar decreasing effects on both the indexes (), indicating that the administration of APP to mice may generate a comparable preventive effect against CCl4-induced damage as BP. Our results presented consist with the reported observations (Wang et al., Citation2012; Yang et al., Citation2013).
Table 1. Effects of APP on body weight, liver weight and hepatosomatic index (HI) of mice after treatment with CCl4.
Tabla 1. Efectos de APP en el peso corporal, peso del hígado e índice hepatosomático (HI) de ratones después del tratamiento con CCl4.
Effects of APP on ALT, AST and LDH activities in serum
The activities of enzymes in serum, such as ALT, AST and LDH, have been known as the sensitive indicators of liver injury (Zhang, Zheng, Pan, & Zheng, Citation2004) because the damage to hepatocytes alters the transport function and membrane permeability, resulting in the leakages of these enzymes from the injured cells (Jayakumar, Ramesh, & Geraldine, Citation2006). The activity levels of ALT, AST and LDH for the normal group were determined as 35.64 ± 5.69, 25.16 ± 3.87 and 160.56 ± 11.66 U/L, respectively. As shown in , the acute hepatotoxicity induced by CCl4 in the experimental mice was observed as the levels of ALT, AST and LDH in serum increased significantly (p < 0.01), relative to the normal group. As expected, daily administration of APP for seven consecutive days prior to the single intoxication of CCl4 effectively lowered the CCl4-induced elevations of serum enzymes (ALT, AST and LDH) in a dosage-dependent manner. Specifically, at a dosage of 300 mg/kg bw of APP, the activities of ALT, AST and LDH decreased to 58.45 ± 5.12, 50.24 ± 6.05 and 190.69 ± 25.36 U/L, and at 450 mg/kg bw, the corresponding data were 45.21 ± 3.52, 37.89 ± 2.55 and 172.45 ± 5.69 U/L, respectively, which were close to that of the positive control BP at 450 mg/kg bw, for which the respective values were determined as 42.56 ± 4.33, 35.58 ± 4.17 and 165.35 ± 20.59 U/L. However, at a low dosage of 150 mg/kg bw of APP, there was no significant decrease in the levels of ALT, AST and LDH activities.
Figure 6. In vitro antioxidant activities of APP determined using ferric reducing powder assay (a), DPPH• scavenging assay (b), HO• scavenging assay (c) and O2•− scavenging assay (d). Vc was used as positive control.
Figura 6. Actividad antioxidante in vitro de APP determinada utilizando el ensayo de poder reductor férrico (a), el ensayo de barrido DPPH• (b), el ensayo de barrido HO• (c) y el ensayo de barrido O2•− (d). Vc se utilizó como control positivo.
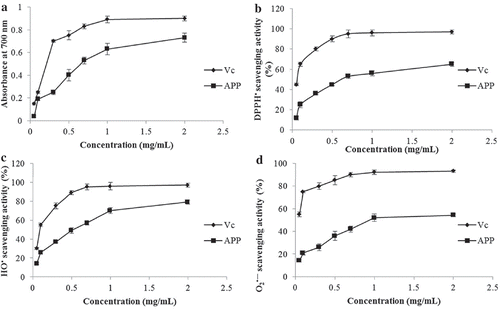
Figure 7. Effects of APP on the enzymic activities of ALT (a), AST (b) and LDH (c) in serum and on the level of hepatic MDA (d) after treatment with CCl4 in mice. All the values are expressed as mean ± SD (n = 10). Mice were treated intragastrically with APP (150, 300 and 450 mg/kg bw) and BP (450 mg/kg bw) once a day for 7 consecutive days before a single administration of 0.8% CCl4. ##p < 0.01, significantly different compared to the normal group. *p < 0.05, **p < 0.01, significantly different compared to the CCl4-intoxicated group.
Figura 7. Efecto de APP en la actividad enzimática de ALT (a), AST (b) y LDH (c) en suero y en el nivel de MDA hepático (d) después del tratamiento con CCl4 en ratones. Todos los valores se expresan como promedio ± SD (n = 10). Los ratones fueron tratados intragástricamente con APP (150, 300 y 450 mg/kg bw) y BP (450 mg/kg bw) una vez al día durante 7 días consecutivos antes de una única administración de 0,8% de CCl4. ##p < 0,01, significativamente diferente en comparación con el grupo normal. *p < 0,05 **p < 0,01, significativamente diferente en comparación con el grupo intoxicado con CCl4.
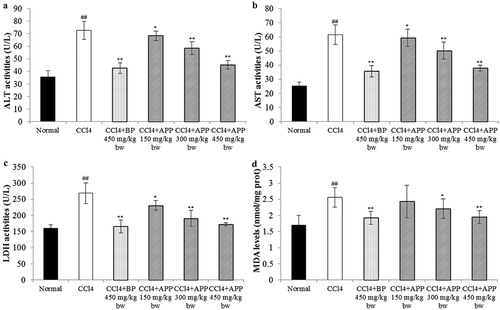
It is well known that CCl4-induced hepatic injury mouse is a widely applied model in screening hepatoprotective medicines or plant active extracts (Banskota, Tezuka, & Kadota, Citation2001; Gilani, Janbaz, & Shah, Citation1998). Liver functions can be well evaluated by determining the activity levels of ALT, AST and LDH (Wang et al., Citation2008; Yang et al., Citation2011). After treatment with CCl4, under the reductive dehalogenation by cytochrome P450, a highly reactive free radical •CCl3 can be formed, which can further promote the generation of reactive peroxy radical CCl3OO• (Mccay, Lai, Poyer, Dubose, & Janzen, Citation1984). The peroxy radical may cause lipid peroxidation of membranes and result in liver damage, leading to the leakages of ALT, AST and LDH from the hepatic cells. In our study, APP was shown to scavenge-free radicals in vitro (); hence the mechanism of hepatoprotective effects of APP on CCl4-induced liver injury may partially lie in its radical scavenging activity in vivo. In this study, treatment with CCl4 along resulted in significant increases in the levels of ALT, AST and LDH in the serum of mice, and these elevations could be effectively inhibited by pre-administration with APP. A similar hepatoprotective observation has been reported for polysaccharides from Pink Lady apple peel (Yang et al., Citation2013). The polysaccharides in this study were from Fuji apple peel obtained by pre-pressing separation technology (one kind of separation technique, through which peel pomace can be separated with little flesh attached on it). Therefore, the present results from in vivo mice experiments indicate that in spite of the differences in monosaccharide compositions and contents, APPs from both the apple cuhivars can develop comparable hepatoprotective effect. Notably, both the APPs contain relatively high mole percentage of uronic acids (23.8% and 17.7% for Fuji and Pink Lady, respectively) that have been shown to play an important role in polysaccharides bioactivities (Chen, Zhang, & Xie, Citation2004; Yang et al., Citation2013).
Effects of APP on the levels of MDA, GSH-Px and SOD in hepatic tissue
MDA is a well-known index of free radical chain reaction of lipid peroxidation (Yang et al., Citation2010). As shown in , treatment with CCl4 caused a significant increase in liver MDA level from 1.70 ± 0.30 to 2.56 ± 0.35 nmol/mg prot, compared to the normal group (p < 0.01). However, the elevated MDA level by CCl4 was found to be reduced by the administration of APP in a dosage-dependent manner. Especially, at 450 mg/kg bw, the MDA level in hepatic tissue was significantly reduced to 1.95 ± 0.21 nmol/mg prot in comparison with the CCl4-intoxicated group (p < 0.01).
It is well known that SOD and GSH-Px are two important and active antioxidants in liver and that the increased lipid peroxidation is usually attributed to the reduction of free radical scavenging antioxidant system. Therefore, the levels of hepatic SOD and GSH-Px in mice liver need further determination. As shown in , under the administration of CCl4, the activities of GSH-Px and SOD were significantly decreased to 814.7 ± 65.6 prot and 77.4 ± 6.59 U/mg prot from 950.7 ± 40.3 and 215.3 ± 14.6 U/mg prot in comparison with the untreated normal group, respectively. Interestingly, the reduced GSH-Px and SOD levels by CCl4 were observed to be recovered by the administration of APP in a dosage-dependent manner. Specifically, for the group pretreated with 450 mg/kg bw APP, the GSH-Px activity was significantly elevated to 907.4 ± 40.2 U/mg prot (p < 0.01), very close to that of positive 450 mg/kg bw BP group (914.8 ± 20.1 U/mg prot). In the case of SOD activity, this dosage treatment resulted in a similar increase to 186.7 ± 9.3 U/mg prot, relative to the inhibited enzymatic activity caused by CCl4 intoxication (p < 0.01).
Figure 8. Effects of APP on the enzymic activities of GSH-Px (a) and SOD (b) in mice liver tissues after treatment with CCl4. All the values are expressed as mean ± SD (n = 10). Mice were treated intragastrically with APP (150, 300 and 450 mg/kg bw) and BP (450 mg/kg bw) once a day for 7 consecutive days before a single administration of 0.8% CCl4. ##p < 0.01, significantly different compared to the normal group. *p < 0.05, **p < 0.01, significantly different compared to the CCl4-intoxicated group.
Figura 8. Efectos de APP en la actividad enzimática de GSH-Px (a) y SOD (b) en los tejidos de hígado de ratón después del tratamiento con CCl4. Todos los valores se expresan como promedio ± SD (n = 10). Los ratones fueron tratados intragástricamente con APP (150, 300 y 450 mg/kg bw) y BP (450 mg/kg bw) una vez al día durante 7 días consecutivos antes de una única administración de 0,8% de CCl4. ##p < 0,01, significativamente diferente en comparación con el grupo normal. *p < 0,05 **p < 0,01 significativamente diferente en comparación con el grupo intoxicado con CCl4.
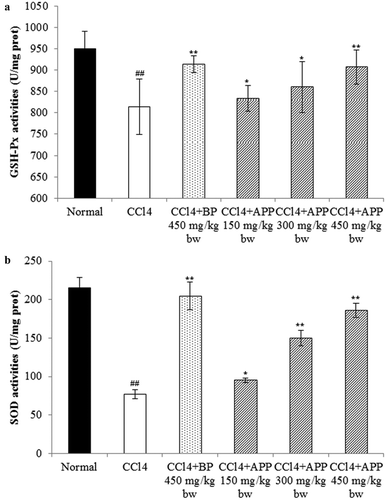
In the aerobic metabolism, in vivo oxidative stress is essentially an imbalance between the production of free radicals and the ability of the body to counteract or detoxify their harmful effects through neutralization by antioxidants. The balance of intracellular oxidative stress depends on both the cellular oxidant species and the antioxidant defence system in cells (Jia et al., Citation2011). Therefore, antioxidant supplementation to suppress the free radical-induced damage has become an alternative and promising therapeutic strategy for reducing the risk of liver diseases (Tian, Zhao, Guo, & Yang, Citation2011). In our study, APP was shown to have hepatoprotective effects against CCl4-induced liver damage, through significantly decreasing the level of MDA and elevating the enzymatic activities of GSH-Px and SOD in liver tissues, especially at the dosage of 450 mg/kg bw.
Histopathological observation of mice liver tissues
To support the hepatoprotective effects of medicines and plant extracts on CCl4-induced liver damage, histopathological observation is commonly provided additionally (Cui, Yang, Lu, Chen, & Zhao, Citation2014). As shown in , for the normal group, typical hepatic cells with complete cytoplasm, prominent nucleus and visible veins could be observed (). However, the CCl4 treatment could cause visible liver tissue damage, parenchymal disarrangement, ballooning degeneration and loss of cellular boundaries (). As demonstrated in , the pre-administration with BP or APP brought about a marked improvement in the histopathology of liver tissues against CCl4-induced histological degradation. Besides, the recovering effect of APP on histological alteration was dosage dependent, indicating that administration of high dosage of APP might access to almost full recovery of histological alternation of liver tissues.
Figure 9. Effect of APP on liver histopathology of mice using H & E staining (original magnification of 400 times). Liver tissues of normal mice (a), Vehicle + CCl4 group (b), BP (450 mg/kg bw) + CCl4 group (c), APP (150 mg/kg bw) + CCl4 group (d), APP (300 mg/kg bw) + CCl4 group (e), APP (450 mg/kg bw) + CCl4 group (f).
Figura 9. Efecto de APP en la histopatología de hígado de ratón utilizando coloración H y E (ampliación original de 400 veces). Los tejidos del hígado de ratones normales (a), Vehículo + grupo CCl4 (b), BP (450 mg/kg bw) + grupo CCl4 (c), APP (150 mg/kg bw) + grupo CCl4 (d), APP (300 mg/kg bw) + grupo CCl4 (e), APP (450 mg/kg bw) + grupo CCl4 (f).
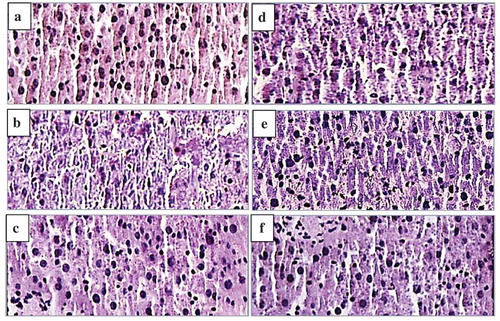
It has been widely considered that one of the principal causes of liver diseases is the occurrence of lipid peroxides induced by free radicals or corresponding derivatives that are thought to cause intracellular oxidative stress (Shim et al., Citation2010; Zhang et al., Citation2004). Therefore, it may be hypothesized that the supplement of dietary antioxidants can inhibit the oxidative stress and thus reduce the risk of liver diseases. Besides apple polyphenols reported before, apple polysaccharides are also shown to be a source of antioxidants in this study. It has been widely recognized that acidic polysaccharides from plants or fruits have antioxidant, antitumor and hepatoprotective activities (Ma et al., Citation2013; Xin et al., Citation2012; Yang et al., Citation2013). The present study clearly implied that the polysaccharides from Fuji apple peel pomace were also one kind of acidic saccharides (including some parts of pectin). The hepatoprotective effects of polysaccharides from Pink Lady apple peel have been reported by Yang et al. (Citation2013). Although there are some structural differences in the two cultivar polysaccharides, the similar molar percentage of uronic acids partially explained the comparable antioxidant and hepatoprotective effects. Apple peel is usually considered as a waste of juicing industry. Besides, through the pre-pressing separation technology, large amount of apple peel pomace with little flesh attached on it can be more easily obtained, compared to peeling apples in the lab level. It has been previously found that galacturonic acid, arabinose and galactose are the main component monosaccharides of apple polysaccharides (Mehrlander, Dietrich, Sembries, Dongowski, & Will, Citation2002; Yang et al., Citation2013), and our quantitative results for the structural composition of APPs are in agreement with the previous founding, but in different amounts and ratios. This may be caused by the differences in extraction process and apple cultivars. Herein, we showed for the first time that the polysaccharides from pre-pressing separation apple (Fuji) peel pomace have antioxidant and hepatoprotective activities, suggesting that apple peel pomace, as the by-product of apple juice industry, has the potential as a source of hepatoprotective polysaccharides.
Conclusion
Pre-pressing separation technology is being introduced to apple juicing industry for enhancing the quality of apple juice. Through this technology, large amount of apple peel pomace with very little flesh attached can be easily obtained after juicing process. It is valuable and necessary to utilize this juicing waste; therefore, APPs were isolated from the pre-pressing separation Fuji peel pomace in this study. APP was found to be a heteropolysaccharides with arabinose, galactose and galacturonic acid as the main monosaccharides. The potential carboxylic group of uronic acids was identified by FT-IR, and the total contents of uronic acid, including both galacturonic and galacturonic acid, were determined to be 14.4% by GC–MS. APP was shown to have in vitro antioxidant activity and in vivo hepatoprotective effect, decreasing the CCl4-induced elevations of enzymic activities (serum ALT, AST and LDH) and hepatic malondialdehyde level, as well as inhibiting the decreases in SOD and GSH-Px activities. Our findings here provide not only the potential for utilization of juicing industry peel wastes, but also a base for further exploitation of APPs as a novel hepatoprotective pharmaceutical ingredient.
Disclosure statement
No potential conflict of interest was reported by the authors.
Additional information
Funding
References
- Altas, S., Kizil, G., Kizil, M., Ketani, A., & Haris, P.I. (2011). Protective effect of Diyarbakır watermelon juice on carbon tetrachloride-induced toxicity in rats. Food and Chemical Toxicology, 49, 2433–2438. doi:10.1016/j.fct.2011.06.064
- Banskota, A.H., Tezuka, Y., & Kadota, S. (2001). Recent progress in pharmacological research of propolis. Phytotherapy Research, 15, 561–571. doi:10.1002/ptr.1029
- Chai, S.C., Hooshmand, S., Saadat, R.L., Payton, M.E., Brummel-Smith, K., & Arjmandi, B.H. (2012). Daily apple versus dried plum: Impact on cardiovascular disease risk factors in postmenopausal women. Journal of the Academy of Nutrition and Dietetics, 112, 1158–1168. doi:10.1016/j.jand.2012.05.005
- Chen, G.T., Ma, X.M., Liu, S.T., Liao, Y.L., & Zhao, G.Q. (2012). Isolation, purification and antioxidant activities of polysaccharides from Grifola frondosa. Carbohydrate Polymers, 89, 61–66. doi:10.1016/j.carbpol.2012.02.045
- Chen, H.X., Zhang, M., & Xie, B.J. (2004). Quantification of uronic acids in tea polysaccharide conjugates and their antioxidant properties. Journal of Agricultural and Food Chemistry, 52, 3333–3336. doi:10.1021/jf0349679
- Cheong, K.L., Wu, D.T., Deng, Y., Leong, Y., Zhao, J., Zhang, W.J., & Li, S.-P. (2016). Qualitation and quantification of specific polysaccharides from Panax species using GC-MS, saccharide mapping and HPSEC-RID-MALLS. Carbohydrate Polymers, 153, 47–54. doi:10.1016/j.carbpol.2016.07.077
- Chun, O.K., Kim, D.-O., & Lee, C.Y. (2003). Superoxide radical scavenging activity of the major polyphenols in fresh plums. Journal of Agricultural and Food Chemistry, 51, 8067–8072. doi:10.1021/jf034740d
- Cui, Y.M., Yang, X.B., Lu, X.S., Chen, J.W., & Zhao, Y. (2014). Protective effects of polyphenols-enriched extract from Huangshan Maofeng green tea against CCl4-induced liver injury in mice. Chemico-Biological Interactions, 220, 75–83. doi:10.1016/j.cbi.2014.06.018
- Dubois, M., Gilles, K.A., Hamilton, J.K., Rebers, P.A., & Smith, F. (1956). Colorimetric method for determination of sugars and related substances. Analytical Chemistry, 28, 350–356. doi:10.1016/j.cbi.2014.06.018
- Gilani, A.H., Janbaz, K.H., & Shah, B.H. (1998). Esculetin prevents liver damage induced by paracetamol and CCl4. Pharmacological Research, 37, 31–35. doi:10.1006/phrs.1997.0262
- Hsiao, G., Shen, M.Y., Lin, K.H., Lan, M.H., Wu, L.Y., Chou, D.S. … Sheu, J.-R. (2003). Antioxidative and hepatoprotective effects of Antrodia camphorata extract. Journal of Agricultural and Food Chemistry, 51, 3302–3308. doi:10.1021/jf021159t
- Jayakumar, T., Ramesh, E., & Geraldine, P. (2006). Antioxidant activity of the oyster mushroom, Pleurotus ostreatus, on CCl4-induced liver injury in rats. Food and Chemical Toxicology, 44, 1989–1996. doi:10.1016/j.fct.2006.06.025
- Jia, X.J., Zhang, C., Qiu, J.F., Wang, L.L., Bao, J.L., Wang, K. … He, C. (2015). Purification, structural characterization and anticancer activity of the novel polysaccharides from Rhynchosia minima root. Carbohydrate Polymers, 132, 67–71. doi:10.1016/j.carbpol.2015.05.059
- Jia, X.Y., Zhang, Q.A., Zhang, Z.Q., Wang, Y., Yuan, J.F., Wang, H.Y., & Zhao, D. (2011). Hepatoprotective effects of almond oil against carbon tetrachloride induced liver injury in rats. Food Chemistry, 125, 673–678. doi:10.1016/j.foodchem.2010.09.062
- Jiang, C.X., Xiong, Q.P., Gan, D., Jiao, Y.P., Liu, J., Ma, L.P., & Zeng, X. (2013). Antioxidant activity and potential hepatoprotective effect of polysaccharides from Cyclina sinensis. Carbohydrate Polymers, 91, 262–268. doi:10.1016/j.carbpol.2012.08.029
- Lee, J.S., Synytsya, A., Kim, H.B., Choi, D.J., Lee, S., Lee, J. … Park, Y.I. (2013). Purification, characterization and immunomodulating activity of a pectic polysaccharide isolated from Korean mulberry fruit Oddi (Morus alba L.). International Immunopharmacology, 17, 858–866. doi:10.1016/j.intimp.2013.09.019
- Liu, W., Wang, H.Y., Pang, X.B., Yao, W.B., & Gao, X.D. (2010). Characterization and antioxidant activity of two low-molecular-weight polysaccharides purified from the fruiting bodies of Ganoderma lucidum. International Journal of Biological Macromolecules, 46, 451–457. doi:10.1016/j.ijbiomac.2010.02.006
- Lv, Y., Yang, X.B., Zhao, Y., Ruan, Y., Yang, Y., & Wang, Z.Z. (2009). Separation and quantification of component monosaccharides of the tea polysaccharides from Gynostemma pentaphyllum by HPLC with indirect UV detection. Food Chemistry, 112, 742–746. doi:10.1016/j.foodchem.2008.06.042
- Ma, L.P., Qin, C.L., Wang, M.C., Gan, D., Cao, L., Ye, H., & Zeng, X. (2013). Preparation, preliminary characterization and inhibitory effect on human colon cancer HT-29 cells of an acidic polysaccharide fraction from Stachys floridana Schuttl. ex Benth. Food and Chemical Toxicology, 60, 269–276. doi:10.1016/j.fct.2013.07.060
- Mccay, P.B., Lai, E.K., Poyer, J.L., Dubose, C.M., & Janzen, E.G. (1984). Oxygen-centered and carbon-centered rree-radical formation during carbon-tetrachloride metabolism - observation of lipid radicals in vivo and in vitro. Journal of Biological Chemistry, 259, 2135–2143.
- Mehrlander, K., Dietrich, H., Sembries, S., Dongowski, G., & Will, F. (2002). Structural characterization of oligosaccharides and polysaccharides from apple juices produced by enzymatic pomace liquefaction. Journal of Agricultural and Food Chemistry, 50, 1230–1236. doi:10.1021/jf011007i
- Murata, M., Tsurutani, M., Tomita, M., Homma, S., & Kaneko, K. (1995). Relationship between apple ripening and browning: Changes in polyphenol content and polyphenol oxidase. Journal of Agricultural and Food Chemistry, 43, 1115–1121. doi:10.1021/jf00053a001
- Nada, S.A., Omara, E.A., Abdel-Salam, O.M.E., & Zahran, H.G. (2010). Mushroom insoluble polysaccharides prevent carbon tetrachloride-induced hepatotoxicity in rat. Food and Chemical Toxicology, 48, 3184–3188. doi:10.1016/j.fct.2010.08.019
- Reitman, S., & Frankel, S. (1957). A colorimetric method for the determination of serum glutamic oxalacetic and glutamic pyruvic transaminases. American Journal of Clinical Pathology, 28, 56–63. doi:10.1093/ajcp/28.1.56
- Ren, L., Perera, C., & Hemar, Y. (2012). Antitumor activity of mushroom polysaccharides: A review. Food & Function, 3, 1118–1130. doi:10.1039/c2fo10279j
- Sassaki, G.L., Souza, L.M., Serrato, R.V., Cipriani, T.R., Gorin, P.A., & Lacomini, M. (2008). Application of acetate derivatives for gas charomtography-mass spectrometry: Novel approaches on carbohydrates, lipids and amino acids analysis. Journal of Chromatography A, 1208, 215–222. doi:10.1016/j.chroma.2008.08.083
- Schulz, H. (1991). Beta-oxidation of fatty-acids. Biochimica Et Biophysica Acta, 1081, 109–120. doi:10.1016/0005-2760(91)90015-A
- Shao, P., Chen, X.X., & Sun, P.L. (2014). Chemical characterization, antioxidant and antitumor activity of sulfated polysaccharide from Sargassum horneri. Carbohydrate Polymers, 105, 260–269. doi:10.1016/j.carbpol.2014.01.073
- Shim, J.Y., Kim, M.H., Kim, H.D., Ahn, J.Y., Yun, Y.S., & Song, J.Y. (2010). Protective action of the immunomodulator ginsan against carbon tetrachloride-induced liver injury via control of oxidative stress and the inflammatory response. Toxicology and Applied Pharmacology, 242, 318–325. doi:10.1016/j.taap.2009.11.005
- Singleton, V.L., Orthofer, R., & Lamuela-Raventos, R.M. (1999). Analysis of total phenols and other oxidation substrates and antioxidants by means of Folin-Ciocalteu reagent. Oxidants and Antioxidants, Pt A, 299, 152–178. doi:10.1016/S0076-6879(99)99017-1
- Spencer, J.P.E., Jenner, A., Aruoma, O.I., Evans, P.J., Kaur, H., Dexter, D.T. … Halliwell, B. (1994). Intense oxidative DNA-damage promoted by L-dopa and its metabolites implications for neurodegenerative disease. Febs Letters, 353(3), 246–250. doi:10.1016/0014-5793(94)01056-0
- Sun, L.J., Guo, Y.R., Fu, C.C., Li, J.J., & Li, Z. (2013). Simultaneous separation and purification of total polyphenols, chlorogenic acid and phlorizin from thinned young apples. Food Chemistry, 136, 1022–1029. doi:10.1016/j.foodchem.2012.09.036
- Tian, L.M., Zhao, Y., Guo, C., & Yang, X.B. (2011). A comparative study on the antioxidant activities of an acidic polysaccharide and various solvent extracts derived from herbal Houttuynia cordata. Carbohydrate Polymers, 83, 537–544. doi:10.1016/j.carbpol.2010.08.023
- Wade, C.R., Jackson, P.G., Highton, J., & Vanrij, A.M. (1987). Lipid-peroxidation and malondialdehyde in the synovial-fluid and plasma of patients with rheumatoid-arthritis. Clinica Chimica Acta, 164, 245–250. doi:10.1016/0009-8981(87)90298-1
- Wang, D.Y., Zhao, Y., Jiao, Y.D., Yu, L.H., Yang, S., & Yang, X.B. (2012). Antioxidative and hepatoprotective effects of the polysaccharides from Zizyphus jujube cv. Shaanbeitanzao. Carbohydrate Polymers, 88, 1453–1459. doi:10.1016/j.carbpol.2012.02.046
- Wang, T., Sun, N.L., Zhang, W.D., Li, H.L., Lu, G.C., Yuan, B.J. … Zhang, C. (2008). Protective effects of dehydrocavidine on carbon tetrachloride-induced acute hepatotoxicity in rats. Journal of Ethnopharmacology, 117, 300–308. doi:10.1016/j.jep.2008.02.010
- Wolfe, K., Wu, X.Z., & Liu, R.H. (2003). Antioxidant activity of apple peels. Journal of Agricultural and Food Chemistry, 51, 609–614. doi:10.1021/jf020782a
- Xin, T., Zhang, F.B., Jiang, Q.Y., Chen, C.H., Huang, D.Y., Lv, Y.J. … Jin, Y. (2012). Purification and antitumor activity of two acidic polysaccharides from the roots of Polygala tenuifolia. Carbohydrate Polymers, 90, 1671–1676. doi:10.1016/j.carbpol.2012.07.048
- Yang, J.Y., Li, Y., Wang, F., & Wu, C.F. (2010). Hepatoprotective effects of apple polyphenols on CCl4-induced acute liver damage in mice. Journal of Agricultural and Food Chemistry, 58, 6525–6531. doi:10.1021/jf903070a
- Yang, X.B., Yang, S., Guo, Y.R., Jiao, Y.D., & Zhao, Y. (2013). Compositional characterisation of soluble apple polysaccharides, and their antioxidant and hepatoprotective effects on acute CCl4-caused liver damage in mice. Food Chemistry, 138, 1256–1264. doi:10.1016/j.foodchem.2012.10.030
- Yang, X.S., Dong, C.A., & Ren, G.X. (2011). Effect of soyasaponins-rich extract from soybean on acute alcohol-induced hepatotoxicity in mice. Journal of Agricultural and Food Chemistry, 59, 1138–1144. doi:10.1021/jf103749r
- Ye, H., Wang, K.Q., Zhou, C.H., Liu, J., & Zeng, X.X. (2008). Purification, antitumor and antioxidant activities in vitro of polysaccharides from the brown seaweed Sargassum pallidum. Food Chemistry, 111, 428–432. doi:10.1016/j.foodchem.2008.04.012
- You, L.J., Gao, Q., Feng, M.Y., Yang, B., Ren, J.Y., Gu, L.J. … Zhao, M. (2013). Structural characterisation of polysaccharides from Tricholoma matsutake and their antioxidant and antitumour activities. Food Chemistry, 138, 2242–2249. doi:10.1016/j.foodchem.2012.11.140
- You, R.X., Wang, K.P., Liu, J.Y., Liu, M.C., Luo, L., & Zhang, Y. (2011). A comparison study between different molecular weight polysaccharides derived from Lentinus edodes and their antioxidant activities in vivo. Pharmaceutical Biology, 49, 1298–1305. doi:10.3109/13880209.2011.621960
- Zhang, S., He, B., Ge, J.B., Li, H.B., Luo, X.Y., Zhang, H. … Fei, X. (2010). Extraction, chemical analysis of Angelica sinensis polysaccharides and antioxidant activity of the polysaccharides in ischemia-reperfusion rats. International Journal of Biological Macromolecules, 47, 546–550. doi:10.1016/j.ijbiomac.2010.07.012
- Zhang, Y.T., Zheng, Q.S., Pan, J., & Zheng, R.L. (2004). Oxidative damage of biomolecules in mouse liver induced by morphine and protected by antioxidants. Basic & Clinical Pharmacology & Toxicology, 95, 53–58. doi:10.1111/j.1742-7843.2004.950202.x
- Zhao, M.M., Yang, N., Yang, B., Jiang, Y.M., & Zhang, G.H. (2007). Structural characterization of water-soluble polysaccharides from Opuntia monacantha cladodes in relation to their anti-glycated activities. Food Chemistry, 105, 1480–1486. doi:10.1016/j.foodchem.2007.05.031
- Zhao, T., Mao, G.H., Feng, W.W., Mao, R.W., Gu, X.Y., Li, T. … Wu, X. (2014). Isolation, characterization and antioxidant activity of polysaccharide from Schisandra sphenanthera. Carbohydrate Polymers, 105, 26–33. doi:10.1016/j.carbpol.2014.01.059
- Zheng, W., Zhao, T., Feng, W.W., Wang, W., Zou, Y., Zheng, D.H. … Wu, X. (2014). Purification, characterization and immunomodulating activity of a polysaccharide from flowers of Abelmoschus esculentus. Carbohydrate Polymers, 106, 335–342. doi:10.1016/j.carbpol.2014.02.079