ABSTRACT
Antioxidant polypeptides of Zanthoxylum bungeanum Maxim seeds kernel (ZBMSK) were investigated based on molecular weight distribution, amino acid composition, and functional properties. The polypeptide had a molecular weight distribution of 1026 ~ 2249 Da and 4926 ~ 6398 Da, and was rich in glutamic acid, arginine, aspartic acid, and leucine, but poor in cysteine and tryptophan. Total amino acids were 56.42 g/100 g, and lysine was the first limiting amino acid. The polypeptide showed excellent solubility, viscosity, emulsifying properties, and foam expansion compared with its original protein. In addition, the polypeptide exhibited favorable reduction power and scavenging capacities for DPPH radicals with an IC50 value of 8.86 mg/mL. These results suggest that the polypeptide derived from ZBMSK has potential future application among functional foods, and may be a promising source of natural antioxidants.
RESUMEN
Se investigaron los polipéptidos antioxidantes de semillas de Zanthoxylum bungeanum (ZBMSK) en base a la distribución del peso molecular, la composición de aminoácidos y las propiedades funcionales. Los polipéptidos presentaban un peso molecular de 1026~2249 Da y 4926~6398 Da, además eran ricos en ácido glutámico, arginina, ácido aspártico y leucina, aunque pobres en cisteína y triptófano. El total de aminoácidos fue de 56,42 g/100 g y la lisina fue el primer aminoácido de limitación. Los polipéptidos mostraron una excelente solubilidad, viscosidad, propiedades emulsionantes y expansión de espuma en comparación con su proteína original. Además, los polipéptidos exhibieron un poder reductor favorable y capacidades de barrido de radicales DPPH con un valor IC50 de 8,86 mg/mL. Estos resultados sugieren que los polipéptidos derivaros de ZBMSK tienen una futura aplicación potencial entre los alimentos funcionales, además podrían ser una prometedora fuente de antioxidantes naturales.
Introduction
Zanthoxylum L. is a species-rich genus in the Rutaceae family comprising more than 200 trees and shrubs distributed worldwide. Zanthoxylum bungeanum Maxim (ZBM) belongs to the genus Zanthoxylum and is native to Western China in Shanxi, Gansu, Sichuan, Yunnan, Guizhou, and Chongqing Provinces (Li et al., Citation2015; Tian et al., Citation2016; Zhu, Huang, Ji, Su, & Zhou, Citation2016). It is a well-known edible and medicinal plant. ZBM fruits are commonly used in cooking and traditional Chinese medications. ZBM seeds are a major by-product of ZBM fruit processing that is widely underutilized. It has been estimated that annual potential ZBM seed production amounts to 1 million metric tons in China with end usage as fertilizer, fuel, or muck (Zhang, Wu, Yang, & Zhang, Citation2015). Previous studies indicate that ZBM seeds are rich in oil, protein, and essential mineral substances (Liu, Yao, Yin, Zheng, & Shen, Citation2016). Although defatted ZBM seeds possess 60.34% protein (Yang, Tian, & Quan, Citation2008), they remain underutilized as a protein source compared with soybeans. This may be because protein products prepared from ZBM seeds exhibit poorer functionality than soybean protein products. Further research is well-merited to exploit ZBM seed protein bioactivity to improve its utilization.
Functional protein properties can be enhanced by utilizing enzymatic hydrolysis under certain conditions. Hydrolysis influences surface hydrophobicity, molecular weight distribution, and hydrolysate polar groups (Bučko, Katona, Popović, Petrović, & Milinković, Citation2016; Quaglia & Orban, Citation1990). Hydrolysate characteristics further influence functional properties of protein resource such as solubility, interfacial, and emulsifying and foaming properties (Kristinsson & Rasco, Citation2000).
Protein hydrolysates have been found to possess antioxidant activity apart from their functionalities. In recent years, investigations focused on protein hydrolysates as natural antioxidants have reported the antioxidant activities from animal sources such as bovine hair (Zeng, Zhang, He, & Shi, Citation2015), porcine cerebral (Zou et al., Citation2016), whey protein (Jost, Monti, & Pahud, Citation1977), fish (Jang, Liceaga, & Yoon, Citation2016; Wang et al., Citation2014), and egg white protein (Chen, Chi, & Xu, Citation2011), as well as plant sources, including pearl millet (Agrawal, Joshi, & Gupta, Citation2016), soy (Park, Lee, Baek, & Lee, Citation2010), rapeseed (Pan, Jiang, & Pan, Citation2009), corn (Jin, Liu, Zheng, Wang, & He, Citation2016), and peanuts (Chen, Zhao, Zhao, Cong, & Bao, Citation2007).
Enzymatic hydrolysis of ZBM protein to obtain bioactive peptides has been widely reported in studies mainly focused on antioxidant peptides preparation (Wang, Tian, Ma, & Guo, Citation2009; Zhang, Song, Jiang, Wu, & Liu, Citation2014), antibacterial peptides (Jiang, Wu, Wang, & Zhang, Citation2014), as well as antihypertensive peptides (Wu, Jiang, Yang, & Zhang, Citation2014). There is limited information on the nutritional value and functional properties of polypeptide from the protein hydrolysate, however. It is essential to further investigate nutritional value and properties of the Zanthoxylum bungeanum Maxim seeds kernel (ZBMSK) polypeptide.
The main objective of the present study is to investigate the biochemical and functional properties of the antioxidant peptides in ZBMSK, which include molecular weight distribution, amino acid composition, solubility, viscosity, emulsifying and foaming properties, as well as antioxidant capacity.
Materials and methods
Materials
The ZBM (Zanthoxylum L.) used in this work is of the Dahongpao variety, and was collected from Hancheng (Shaanxi, China). The Alcalase 2.4 L (2.4 AU/g) was purchased from Novozymes (Denmark) Co. All other reagents were of analytical grade.
Preparation of ZBMSK hydrolysates
The ZBMSK was first shelled and the oil was squeezed from them, then they were sieved through a 40-mmmesh screen and dried at 35°C for 3 days. Experimental preliminary protein extraction results using different methods (acid extraction, salt extraction) proved salt extraction to be the optimal approach to extract ZBMSK protein. The ZBMSK (0.5 g) was mixed with NaCl solution (0.84 mol/L) at a ratio of 1:17 (w/v), and extracted at 39°C for 60 min, then the pH of the resulting mixture was adjusted to 9.0. After being centrifuged under 3000 r/min for 15 min, the supernatant was dialyzed in a dialysis bag for 24 h and collected and lyophilized using a freeze dryer.
The ZBMSK protein hydrolysate was prepared according to the enzymolysis method (Zhang et al., Citation2014) with slight modification. Our previous work regarding antioxidant experiments using different commercial proteases (Alcalase 2.4 L, Papain, Protamex, Neutrase 0.5 L, and Flavourzyme 500 mg) revealed that hydrolysates antioxidant activity was highest for those obtained using Alcalase 2.4L (Wang et al., Citation2009). The results of our preliminary study showed the optimum ZBMSK protein hydrolyzation conditions were Alcalase 2.4 L at pH 10.5 with a hydrolysis temperature of 55°C for 3.5 h and enzyme/substrate ratio of 8% (w/w). We utilized the same conditions in the present study. The mixture was heated in water bath at 95°C for 15 min to inactivate the enzyme, and then centrifuged at 4000 r/min for 20 min to isolate the hydrolysate. The supernatant was collected, lyophilized, and stored at −40°C for further use.
Determination of peptide content
The peptide content of ZBMSK protein hydrolysate fractions was determined using the Folin–Ciocalteu method (Wang et al., Citation2009). An appropriately diluted sample (1.0 mL) and 1.0 mL alkaline copper solution was poured into a test tube, followed by 4.0 mL of Folin–Ciocalteu reagent, mixed immediately, and incubated for 5 min at 55°C in a water bath and then allowed the mixture to stand in cold water for 10 min. The absorbance was determined at 650 nm. Bovine serum albumin was used as a standard for quantification of peptide content.
Purification of antioxidant peptide
Ultrafiltration
ZBMSK protein hydrolysate was filtered using an ultrafiltration system through two ultrafiltration membranes with molecular weight cut off (MWCO) of 10 and 30 kDa, respectively. The fraction was divided into three parts designated as <10 kDa, 10 ~ 30 kDa, and >30 kDa, and the ultrafiltration pressure was 0.25 MPa. The content and antioxidant activity of each fraction were then evaluated. The fraction with the highest antioxidant activity was lyophilized and stored for further purification.
Gel filtration chromatography
The fraction exhibiting the highest antioxidant activity after ultrafiltration separation was used for further purification on a Sephadex G-25 column (1.6 cm × 50 cm). The column was eluted with distilled water at a flow rate of 0.35 mL/min, volume injection and detection sensitivity were 2 mL and 0.2 A, respectively. The elution was monitored at 280 nm. Blue Sephadex-2000 (Citation2000 kDa), bovine serum albumin (67,000 Da), vitamin B12 (1350 Da), glutathione (307.32 Da), L-tyrosine (181.19 Da), and potassium chromate were used as molecular weight standards.
Antioxidant activity determination
Reducing power assay
Total antioxidant capacity (TAC) was measured according to the ferric-reducing antioxidant power (FRAP) method (Benzie & Strain, Citation1996) with slight modification. A freshly prepared 1.8 mL FRAP solution (25 mL 0.3 mol/L acetate buffered solution, 2.5 mL 10 mmol/L TPTZ solution, 2.5 mL 20 mmol/L FeCl3 solution) was preheated to 37°C, and 0.1 mL ZBMSK protein hydrolysates were added. After vigorously shaking, the mixture was placed at room temperature for 10 min, and solution absorbance was measured at 593 nm. FeSO4 (1.0 mmol/L) was used as the standard, and the antioxidant activity of the hydrolysates was expressed as the required number (mmol) of FeSO4, which achieved the same absorbance.
DPPH radical scavenging activity
The effect of protein hydrolysate on the DPPH radical was determined using the method previously described by Wang et al. (Citation2009). Briefly, DPPH (25 mg) was mixed with methanol to 500 mL and the mother liquor (0.5 mg/mL) was obtained, and diluted with methanol to 0.005, 0.015, 0.025, 0.035, 0.045 mg/mL. Solutions absorbance was measured at 515 nm with a spectrophotometer, and the calibration curve was constructed accordingly.
The sample (0.2 mL) was mixed with 7.8 mL 0.025 mg/mL DPPH-methanol solution and absorbance was measured at 515 nm. After incubation in the dark for 30 min, the absorbance of the resulting solution was measured once again. The radical scavenging activity was calculated as following Equation (1).
The 50% scavenging activity of ZBMSK polypeptide (IC50) was then as the peptide concentration required to scavenge calculated.
Nutritional evaluation
Amino acid composition analysis
ZBMSK polypeptide (50 mg) was hydrolyzed to estimate amino acid composition with 6 mol/L HCl for 22 h at 100°C and analyzed by automatic amino acid analyzer.
Determination of amino acid score
The amino acid score (AAS) of ZBMSK polypeptide was determined as the description by Bhaskar, Benila, Radha, and Lalitha (Citation2008) with minor modifications. The essential amino acid (EAA) ratio contents in the samples were evaluated based on the requirement patterns for adults (Noack, Citation1974). The AAS was calculated using the following Equation (2).
Determination of functional properties
Solubility
Solubility was performed according to the procedures reported by Sila et al. (Citation2015) with slight modifications. The sample (1 g) was dissolved with distilled water to 20 mL and stirred with a magnetic stirrer, then, the solution was adjusted to the desired pH. The mixture was stirred continuously at room temperature for 25 min to extract the substrate/liquid ratio of 1:20 (w/v) and further centrifuged at 3000 r/min for 10 min. The nitrogen content in the supernatants was then determined via Kjeldahl method (AOAC, Citation2000). The solubility of ZBMSK polypeptide, defined as the amount of soluble nitrogen from the total nitrogen, was calculated using the following Equation (3).
Viscosity
The ZBMSK protein and polypeptide were prepared as 1.0%, 2.5%, 5.0%, 7.5%, 10%, 20%, 30%, 40%, and 50% of the solution. Apparent viscosity was then determined by NDJ-1 rotational viscometer.
Emulsifying properties
Emulsifying capacity index (ECI) and emulsion stability index (ESI) were determined according to Shahidi, Han, and Synowiecki (Citation1995) with minor modifications. Rapeseed oil (25 mL) and 25 mL 0.8% ZBMSK protein/polypeptide solution were mixed and the pH was adjusted to 2, 4, 6, 8, 10, and 12, respectively. The mixture was homogenized using a homogenizer at a speed of 1000 r/min for 2 min. The 5 mL emulsion was transferred into 5 mL of centrifugal tube and centrifuged at 2000 r/min for 5 min, then emulsified layer height was recorded. ESI of the samples was determined by heating the mixture for 30 min at 80°C in a water bath, and the height of emulsified layer was recorded. The ECI and ESI were calculated according to the following Equations (4) and (5).
where h0 is emulsified layer height before heating, h1 is emulsified layer height after heating.
Foaming properties
The foam expansion (FE) and foam stability (FS) of ZBMSK protein and polypeptide (different concentrations from 1–5%) were measured using a slightly modified version of the method described by Pedroche et al. (Citation2004). A small amount of ZBMSK protein/polypeptide was dissolved in 100 mL distilled water, the solutions were stirred at 30°C for 60 min using a magnetic stirrer, and the pH was adjusted to 7.0. The solution was poured into a 250 mL cylinder and the total volume was recorded. This was followed by homogenization for 30 s, then the whipped sample was immediately transferred into a 250 mL cylinder and volume was recorded. The whipped sample stood at room temperature for 30 min and the volume was again recorded. The FE and FS were calculated as following Equations (6) and (7).
where VT is volume after whipping, V0 is volume before whipping, and Vt is volume after standing.
Statistical analysis
Data were analyzed using SPSS 13.0 software (SPSS for Windows, Version 13.0, USA) and expressed as mean ± SD. One-way analysis of variance was used to determine the least significant differences between variables (p < 0.05).
Results and discussion
Purification of the antioxidant peptide
The degree of molecular weight distribution of the three fractions (<10 kDa, 10 ~ 30 kDa, and >30 kDa) was obtained by ultrafiltration (). The ZBMSK protein hydrolysate with molecular weight ˂10 kDa accounted for 55.47% of the whole hydrolysate, and the fraction contents with molecular weight between 10 ~ 30 kDa and >30 kDa were 20.98% and 23.54%, respectively. The result indicated that the hydrolysate contained mostly low-molecular-weight peptides. The antioxidant activities of different molecular weight fractions and the original ZBMSK protein hydrolysate were then compared (). Among all fractions, the fraction with molecular weight ˂10 kDa exhibited the strongest antioxidant activity. Thus, the peptide with low molecular weight possessed greater antioxidant activity than the peptides with high molecular weight. This might be because low-molecular-weight peptides easily react with and eliminate free radicals. Accordingly, the fraction with molecular weight ˂10 kDa was selected for further purification.
Table 1. Degree of molecular weight distribution for the ZBMSK protein hydrolysate.
Tabla 1. Grado de distribución del peso molecular de hidrolizado proteínico ZBMSK.
Table 2. Antioxidant ability of original ZBMSK protein hydrolysate and fraction obtained by ultrafiltration.
Tabla 2. Habilidad antioxidante de hidrolizado proteínico ZBMSK original y fracción obtenida mediante ultrafiltración.
Molecular weight distribution
The fraction with a molecular weight ˂10 kDa was further separated into two peaks () using a Sephadex G-25 gel filtration column. The corresponding molecular weights ranged from about 4926 ~ 6398 Da and 1026 ~ 2249 Da. These results are consistent with previous antioxidant peptide study from soy protein (Park et al., Citation2010) or sandfish (Jang et al., Citation2016), in which the most potent antioxidant peptides were observed in the peptides with molecular weight ˂3000 Da. Higher antioxidant properties by low-molecular-weight peptides are thought to be because of their low molecular weight as they can easily react with free radicals and thereby eliminate free radicals (Jang et al., Citation2016). Further purification needs to be done to confirm the molecular weight by RP-HPLC or ion-exchange chromatography.
Amino acid composition and amino acid score
The fraction with molecular weight ˂10 kDa was next analyzed for amino acid composition (). This polypeptide was composed of 18 amino acids with the total amount of 56.42 g per 100 g ZBMSK protein hydrolysate and 8 EAAs (16.66 g per 100 g). It was rich in glutamic acid (13.06%), arginine (7.294%), aspartic acid (5.027%), and leucine (4.706%), but low in cysteine and tryptophan. Total hydrophobic amino acid content was 14.90 g per 100 g of the sample. Several studies have demonstrated that hydrophobic amino acids in protein hydrolysate act as antioxidants by enhancing lipid solubility, thus facilitating better interaction with free radicals (Rajapakse, Mendis, Jung, Je, & Kim, Citation2005; Saiga, Tanabe, & Nishimura, Citation2003). Rapeseed protein hydrolysates also displayed similar amino acid composition (Pan et al., Citation2009). Thus, these amino acids may contribute to the antioxidative activity of polypeptide.
Table 3. Amino acid composition and amino acid score (AAS) of ZBMSK polypeptide.
Tabla 3. Composición de los aminoácidos y puntuación de los aminoácidos (AAS) de polipéptidos ZBMSK.
AAS was used here to evaluate protein nutritive value by comparing the amount of EAAs with standard proteins. revealed that leucine (75.90) and tryptophan (67.23) have higher AAS in the ZBMSK polypeptide. Lysine (35.76) was the most limiting amino acid, thus ZBMSK protein hydrolysate might be a proper protein source to meet human requirements with lysine as the rate-limiting amino acid.
Functional properties of ZBMSK polypeptide
Solubility
Solubility is one of the most important characteristics of protein and their products. (a) shows the solubility of protein and polypeptide from ZBMSK. Polypeptide solubility was significantly greater compared with the original protein, especially in the vicinity of the protein’s isoelectric point. All polypeptides were soluble over a wide pH range (2.0 ~ 12.0) with more than 95% solubility. The solubility profile of the protein exhibited a U-shaped carve at pH 2 ~ 7, but solubility was stable in alkaline conditions (pH 7 ~ 12). This further supports the findings of Sila et al. (Citation2015) and Chobert, Bertrand-Harb, and Nicolas (Citation1988) who reported that the protein hydrolysate has better solubility than the original protein. The high solubility of hydrolysate might be attributed to its low molecular weight and higher ratio of hydrophilic groups.
Figure 2. Functional properties of ZBMSK polypeptide: (a). Effect of pH value on the solubility of ZBMSK protein and polypeptide; (b). Effect of ZBMSK protein and polypeptide concentration on viscosity; (c). Effect of pH on emulsifying properties of ZBMSK protein and polypeptide; (d). Effect of pH on emulsion stability of ZBMSK protein and polypeptide; (e). Effect of ZBMSK protein and polypeptide concentration on foaming ability; (f). Effect of ZBMSK protein and polypeptide concentration on foam stability.
Figura 2. Propiedades funcionales de polipéptidos ZBMSK: (a). Efecto del valor pH en la solubilidad de proteína y polipéptidos ZBMSK; (b). Efecto de la concentración de proteína y polipéptidos ZBMSK en la viscosidad; (c). Efecto del pH en las propiedades emulsionantes de proteína y polipéptidos ZBMSK; (d). Efecto del pH en la estabilidad de emulsión de proteína y polipéptidos ZBMSK; (e). Efecto de la concentración de proteína y polipéptidos ZBMSK en la habilidad de espumosidad; (f). Efecto de la concentración de proteína y polipéptidos ZBMSK en la estabilidad de la espuma.
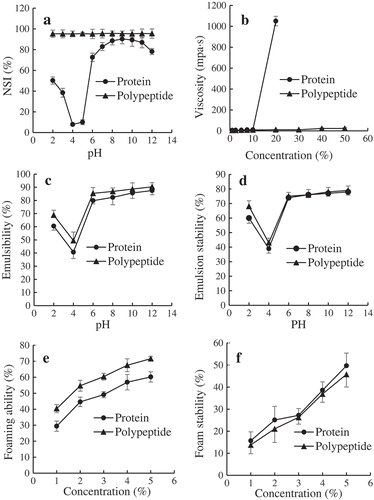
Enzymatic hydrolysis potentially increases polar and ionizable protein hydrolysate groups which can form stronger hydrogen bonds with water. Consequently, the hydrolysate is more soluble post-hydrolysis (Chen et al., Citation2011; Gbogouri, Linder, Fanni, & Parmentier, Citation2004; Mutilangi, Panyam, & Kilara, Citation1996). The fact that pH showed little effect on ZBMSK polypeptide solubility can be attributed to the amino acid composition (especially the content of acidic amino acids) (Yuan et al., Citation2009). Zhang and Xu also found that soybean protein hydrolysate has a stable solubility across a wide pH range (Citation2003). In general, our protein hydrolysates have excellent solubility over a wide pH range, suggesting that ZBMSK polypeptide has a similarly wide application range on the attractive appearance and a smooth mouthfeel of the food.
Viscosity
(b) shows the viscosity variations of ZBMSK protein and its polypeptide at different concentrations. Hydrolysate viscosity was lower compared with the original protein. As the concentration increased from 0–10%, the viscosity resulted in insignificant change in the protein and polypeptide. When the concentration was >10%, protein viscosity rose steeply and liquidity rapidly decreased. The polypeptide’s viscosity change remained relatively small, even at the concentration of 50%, however, it was also rich in mobility. This is in line with the work of Wang et al. who reported that increasing protein/polypeptide concentrations affects viscosity (Wang, He, Shi, Bian, & Sui, Citation2007). Previous studies on soybean protein have also shown that hydrolysate viscosity declines sharply compared with the maternal protein (Zhang & Xu, Citation2003). Viscosity variations may be related to protein polymer degradation and increase in ionic groups (–NH2 and –COOH) (Guo & Zhang, Citation1999). A lower viscosity is of great importance to the food industry because of its necessary functionality, specifically in improving qualities like texture and mouthfeel.
Emulsifying properties
Emulsifying capacity (ECI) and emulsifying stability (ESI) are two indexes generally used to determine the ability of protein hydrolysates to form and stabilize emulsions. (c,d) displayed the ECI and ESI of ZBMSK protein and polypeptide at different pH values. The change tendencies of protein and polypeptide ECI and ESI were consistent with pH. The lowest ECI and ESI were found at pH 4.0, and then remarkably increased with pH until becoming stable. A recent study regarding antibacterial peptide from ZBMSK reached the same conclusion concerning emulsifying properties and pH effects (Jiang et al., Citation2015). Moreover, the ECI and ESI of the protein were slightly lower than that of the polypeptide at similar pH values, which may be ascribed to the differences in molecular size and lipophilic–hydrophilic arrangement.
Higher concentrations of larger-molecular-weight peptides or more hydrophobic peptides contribute to emulsion stability (Mutilangi et al., Citation1996). The hydrolysate is surface active, which promotes oil-in-water emulsion due to the presence of hydrophilic and hydrophobic groups with their associated charges (Gbogouri et al., Citation2004). Jost et al. (Citation1977) reported a direct relationship between surface activity and peptide length, further implying that enzymatic hydrolysis can improve protein emulsifying properties. This finding may have a potential application in functional foods.
In addition, ECI and ESI trends similarly influenced pH trends regarding ZBMSK protein solubility, Yuan et al. observed a similar trend in a study on soy glycinin (Citation2009). These results confirm that protein solubility contributes significantly to protein emulsifying properties. Higher ECI and EAI values of hydrolysates accompanied higher solubility (Mutilangi et al., Citation1996). Proteins/polypeptides with high solubility show enhanced emulsifying efficiency by rapid diffusion and absorption at the interface. In our experiment, peptides could not move rapidly to the interface when the lowest solubility occurred at pH 4. The peptide’s net charge was minimized at pH 4, thus the ECI and EAI of protein/polypeptide decreased.
Foaming properties
Foaming properties are also in food applications. FE and FS of the ZBMSK protein/polypeptide as a function of concentration (pH = 7) are shown in (e,f). The polypeptide exhibited a stronger FE and lower FS than the protein. The FE and FS both increased with substance concentration increment for both protein and polypeptide. Both FE and FS appeared to be affected by size, surface charges, surface hydrophobicity, and viscosity (Kinsella, Citation1976). This may be because after hydrolyzation, the protein hydrolysates were extensively unfolded at the air–water interface, causing the hydrolysate viscosity to decrease and thus facilitating foam formation while improving FC (Townsend & Nakai, Citation1983). Lower hydrolysate viscosity led to less robust adsorbed surface film. The liquid membrane strength was also weakened, and FS was lower than in the protein (Guo & Zhang, Citation1999; Phillips, Whitehead, & Kinsella, Citation1994). Shahidi et al. (Citation1995) found that excessive hydrolysis can decrease FS because microscopic peptides do not have the strength necessary to maintain stable foam. Foams with higher protein concentrations are generally denser and more stable due to an increase in interfacial film thickness. Further, a polypeptide with good foaming ability has poor FS, and which often has the poor foam ability and vice versa. This conclusion was also reached by Jiang et al. (Citation2015) while studying the effects of pH on the FC and FS of ZBMSK antibacterial peptides.
Antioxidant properties
The antioxidant activities of ZBMSK polypeptide were measured using two test systems, including FRAP and DPPH radical scavenging capacity. TAC increased as concentration increased in the range of 10 ~ 30 mg/mL, as shown in (a). However, it did not increase past this polypeptide concentration. This increase can be explained by the fact that compounds with reducing power, as electron donors, cause the oxidized intermediates of the lipid peroxidation processes to decrease. The presence of these antioxidant reducers converts Fe3+ complex to a ferrous form (Fe2+) (Agrawal et al., Citation2016). The ZBMSK polypeptide was observed possessing strong DPPH radical scavenge ability, as shown in (b). At a concentration of 4 ~ 20 mg/mL, the scavenging activity range on DPPH radicals reached 24.56–78.45% with the low IC50 values of 8.86 mg/mL, which increased as polypeptide concentration increased. A higher polypeptide concentration leads to higher DPPH radical scavenging activity. This was also found for corn protein hydrolysate reported by Jin et al. (Citation2016) and for egg white protein hydrolysate reported by Chen et al. (Citation2011). As this tendency was in line with the index curve, the DPPH radical scavenging activity can be estimated using the formula: y = 33.552 Ln(x)−23.186. Polypeptide concentration possessed a good positive correlation with DPPH scavenging ability (R2 = 0.9963). In addition, some scholars have confirmed that ZBMSK polypeptides also have a certain capacity to inhibit the lipid peroxidation according to the ferric thioeyanate method (Wang et al., Citation2009). In the present study, the two assays of polypeptide antioxidant activities strongly depended on peptide concentration, and both of TAC and DPPH free radical scavenging activity increased as ZBMSK concentration increased.
Figure 3. Antioxidant capacity of ZBMSK polypeptide: (a). Reducing power assay of ZBMSK polypeptide; (b). DPPH radical scavenging activity.
Figura 3. Capacidad antioxidante de polipéptidos ZBMSK: (a). Ensayo de poder reductor de polipéptidos ZBMSK; (b). Actividad de barrido de radicales DPPH.
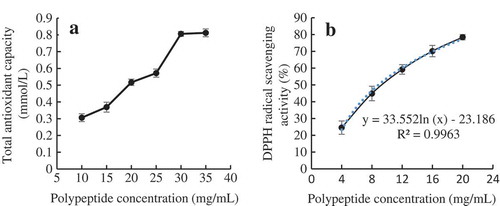
The results imply that ZBMSK polypeptides possess noticeable antioxidant capacity (). The DPPH radical scavenging rate of ZBMSK polypeptides is close to those of protein hydrolysates from egg white (Chen et al., Citation2011) and peanuts (Chen et al., Citation2007; Hwang, Shyu, Wang, & Hsu, Citation2010), and higher than that of protein hydrolysates from spotless smooth-hound muscle (Wang et al., Citation2014). ZBMSK polypeptide antioxidant activity might be attributed to its specific amino acid composition and sequence.
Table 4. Comparison of ZBMSK polypeptide antioxidant capacity with other polypeptides from different sources.
Tabla 4. Comparación de la capacidad antioxidante de polipéptidos ZBMSK con otros polipéptidos de diferentes fuentes.
Conclusions
In summary, our results indicate that ZBMSK polypeptide is a high-quality plant protein resource rich in amino acid composition. ZBMSK polypeptides possess satisfactory functional properties, including solubility, viscosity, and emulsifying and foaming properties, which suggest that ZBMSK polypeptides might have good applications in functional foods. Furthermore, ZBMSK polypeptides exhibited strong antioxidant activities when evaluated by FRAP and DPPH radical scavenging ability, and may be used to produce antioxidant-rich foods.
Disclosure statement
No potential conflict of interest was reported by the authors.
References
- Agrawal, H., Joshi, R., & Gupta, M. (2016). Isolation, purification and characterization of antioxidative peptide of pearl millet (Pennisetum glaucum) protein hydrolysate. Food Chemistry, 204, 365–372. doi:10.1016/j.foodchem.2016.02.127
- AOAC. (2000). Official methods of analysis of AOAC international (17th ed.). Gaithersburg: Association of Official Analytical Chemists.
- Benzie, I.F.F., & Strain, J.J. (1996). The ferric reducing ability of plasma as a measure of “antioxidant power”: The FRA Passay. Analytical Biochemistry, 239, 70‒76. doi:10.1006/abio.1996.0292
- Bhaskar, N., Benila, T., Radha, C., & Lalitha, R.G. (2008). Optimization of enzymatic hydrolysis of visceral waste proteins of Catla (Catla catla) for preparing protein hydrolysate using a commercial protease. Bioresource Technology, 99(2), 335–343. doi:10.1016/j.biortech.2006.12.015
- Bučko, S., Katona, J., Popović, L., Petrović, L., & Milinković, J. (2016). Influence of enzymatic hydrolysis on solubility, interfacial and emulsifying properties of pumpkin (Cucurbita pepo) seed protein isolate. Food Hydrocolloids, 60, 271–278. doi:10.1016/j.foodhyd.2016.04.005
- Chen, C., Chi, Y.-J., & Xu, W. (2011). Comparisons on the functional properties and antioxidant activity of spray-dried and freeze-dried egg white protein hydrolysate. Food and Bioprocess Technology, 5(6), 2342–2352. doi:10.1007/s11947-011-0606-7
- Chen, G.-T., Zhao, L., Zhao, L.-Y., Cong, T., & Bao, S.-F. (2007). In vitro study on antioxidant activities of peanut protein hydrolysate. Journal of the Science of Food and Agriculture, 87, 357–362. doi:10.1002/jsfa.2744
- Chobert, J.-M., Bertrand-Harb, C., & Nicolas, M.-G. (1988). Solubility and emulsifying properties of caseins and whey proteins modified enzymatically by trypsm. Journal of Agricultural and Food Chemistry, 36, 883‒892. doi:10.1021/jf00083a002
- Gbogouri, G.A., Linder, M., Fanni, J., & Parmentier, M. (2004). Influence of hydrolysis degree on the functional properties of salmon byproducts hydrolysates. Journal of Food Science, 69, 615–622. doi:10.1111/j.1365-2621.2004.tb09909.x
- Guo, Q.Q., & Zhang, L.W. (1999). The research of active peptide in food. Food & Machinery, 12‒14. doi:10.13652/j.issn.1003-5788.1999.06.003
- Hwang, J.-Y., Shyu, Y.-S., Wang, Y.-T., & Hsu, C.-K. (2010). Antioxidative properties of protein hydrolysate from defatted peanut kernels treated with esperase. LWT-Food Science and Technology, 43, 285–290. doi:10.1016/j.lwt.2009.08.020
- Jang, H.L., Liceaga, A.M., & Yoon, K.Y. (2016). Purification, characterisation and stability of an antioxidant peptide derived from sandfish (Arctoscopus japonicus) protein hydrolysates. Journal of Functional Foods, 20, 433–442. doi:10.1016/j.jff.2015.11.020
- Jiang, T.L., Wu, H.Y., Dong, X.H., Zhang, M.M., Shen, G.H., & Zhang, Z.Q. (2015). The foaming and emulsifying properties of the antibacterial peptides from the Zanthoxylum bungeanum seed. Journal of Nuclear Agricultural Sciences, 29, 2320‒2326. doi:10.11869/j.issn.100-8551.2015.12.2320
- Jiang, T.L., Wu, H.Y., Wang, H., & Zhang, Z.Q. (2014). Optimization of antibacterial peptides preparation using pepsin from prickly ash seed protein by response surface methodology. Science and Technology of Food Industry, 35, 226‒23. doi:10.13386/j.issn1002-0306.2014.20.041
- Jin, D.-X., Liu, X.-L., Zheng, X.-Q., Wang, X.-J., & He, J.-F. (2016). Preparation of antioxidative corn protein hydrolysates, purification and evaluation of three novel corn antioxidant peptides. Food Chemistry, 204, 427‒436. doi:10.1016/j.foodchem.2016.02.119
- Jost, R., Monti, J.C., & Pahud, J.J. (1977). Partial enzymatic hydrolysis of whey protein by trypsin. Journal of Dairy Science, 60, 1387‒1393. doi:10.3168/jds.S0022-0302(77)84041-1
- Kinsella, J.E. (1976). Functional properties of proteins in foods: A survey. Critical Reviews in Food Science & Nutrition, 7, 219–280. doi:10.1080/10408397609527208
- Kristinsson, H.G., & Rasco, B.A. (2000). Fish protein hydrolysates: Production, biochemical, and functional properties. Critical Reviews in Food Science and Nutrition, 40, 43–81. doi:10.1080/10408690091189266
- Li, J.K., Hui, T., Wang, F.L., Li, S., Cui, B.W., Cui, Y.Q., & Peng, Z.Q. (2015). Chinese red pepper (Zanthoxylum bungeanum Maxim.) leaf extract as natural antioxidants in salted silver carp (Hypophthalmichthys molitrix) in dorsal and ventral muscles during processing. Food Control, 56, 9‒17. doi:10.1016/j.foodcont.2015.03.001
- Liu, T., Yao, S.-Y., Yin, Z.-Y., Zheng, X.-X., & Shen, Y. (2016). Study on preparation method of Zanthoxylum bungeanum seeds kernel oil with zero trans-fatty acids. Environmental Science and Pollution Research, 23(8), 7132–7137. doi:10.1007/s11356-015-5052-z
- Mutilangi, W.A.M., Panyam, D., & Kilara, A. (1996). Functional properties of hydrolysates from proteolysis of heat-denatured whey protein isolate. Journal of Food Science, 61, 270–275. doi:10.1111/j.1365-2621.1996.tb14174.x
- Noack, R. (1974). Energy and protein requirements. Report of a Joint FAO/WHO Ad Hoc Expert Committee. WHO Technical Report Series No. 522, 118 S., Genf 1973. Nahrung, 18, 329–332. doi:10.1002/food.19740180314
- Pan, M., Jiang, T.S., & Pan, J.L. (2009). Antioxidant activities of rapeseed protein hydrolysates. Food and Bioprocess Technology, 4(7), 1144–1152. doi:10.1007/s11947-009-0206-y
- Park, S.Y., Lee, J.-S., Baek, H.-H., & Lee, H.G. (2010). Purification and characterization of antioxidant peptides from soy protein hydrolysate. Journal of Food Biochemistry, 34, 120–132. doi:10.1111/j.1745-4514.2009.00313.x
- Pedroche, J., Yust, M.M., Lqari, H., Girón-Calle, J., Alaiz, M., Vioque, J., & Millán, F. (2004). Brassica carinata protein isolates: Chemical composition, protein characterization and improvement of functional properties by protein hydrolysis. Food Chemistry, 88(3), 337–346. doi:10.1016/j.foodchem.2004.01.045
- Phillips, L.G., Whitehead, D.M., & Kinsella, J.E. (1994). Structure-function of food proteins: Protein stabilized foams. New York, NY: Academic Press.
- Quaglia, G.B., & Orban, E. (1990). Influence of enzymatic hydrolysis on structure and emulsifying properties of sardine (Sardina pilchardus) protein hydrolysates. Journal of Food Science, 55, 1571–1573, 1619. doi:10.1111/jfds.1990.55.issue-6
- Rajapakse, N., Mendis, E., Jung, W.-K., Je, J.-Y., & Kim, S.-K. (2005). Purification of a radical scavenging peptide from fermented mussel sauce and its antioxidant properties. Food Research International, 38(2), 175–182. doi:10.1016/j.foodres.2004.10.002
- Saiga, A., Tanabe, S., & Nishimura, T. (2003). Antioxidant activity of peptides obtained from porcine myofibrillar proteins by protease treatment. Journal of Agricultural and Food Chemistry, 51, 3661–3667. doi:10.1021/jf021156g
- Shahidi, F., Han, X.-Q., & Synowiecki, J. (1995). Production and characteristics of protein hydrolysates from capelin (Mallotus villosus). Food Chemistry, 53, 285–293. doi:10.1016/0308-8146(95)93934-J
- Sila, A., Martinez-Alvarez, O., Haddar, A., Gómez-Guillén, M.C., Nasri, M., Montero, M.P., & Bougatef, A. (2015). Recovery, viscoelastic and functional properties of Barbel skin gelatine: Investigation of anti-DPP-IV and anti-prolyl endopeptidase activities of generated gelatine polypeptides. Food Chemistry, 168, 478–486. doi:10.1016/j.foodchem.2014.07.086
- Tian, J.-M., Wang, Y., Xu, Y.-Z., Yu, Z.-C., Wei, A.-Z., Zhang, W.-M., & Gao, J.-M. (2016). Characterization of isobutylhydroxyamides with NGF-potentiating activity from Zanthoxylum bungeanum. Bioorganic & Medicinal Chemistry Letters, 26(2), 338–342. doi:10.1016/j.bmcl.2015.12.015
- Townsend, A.-A., & Nakai, S. (1983). Relationships between hydrophobicity and foaming characteristics of food proteins. Journal of Food Science, 48, 588–594. doi:10.1111/j.1365-2621.1983.tb10796.x
- Wang, B., Gong, Y.-D., Li, Z.-R., Yu, D., Chi, C.-F., & Ma, J.-Y. (2014). Isolation and characterisation of five novel antioxidant peptides from ethanol-soluble proteins hydrolysate of spotless smoothhound (Mustelus griseus) muscle. Journal of Functional Foods, 6, 176–185. doi:10.1016/j.jff.2013.10.004
- Wang, H., Tian, C.R., Ma, S.L., & Guo, J.Y. (2009). Enzyme screening for the preparing of antioxidant peptide from Zanthoxylum bungeanum seed kernel. Food and Fermentation Industries, 35, 119‒123. doi:10.13995/j.cnki.11-1802/ts.2009.03.019
- Wang, J., He, H., Shi, Y.L., Bian, X.L., & Sui, Y.J. (2007). Study on ACE inhibitory peptide and its functional properties from corn and soybean. Journal of the Chinese Cereals and Oils Association, 22, 38–42.
- Wu, H.Y., Jiang, T.L., Yang, C.Z., & Zhang, Z.Q. (2014). Separation and purification of antihypertensive peptides from Zanthoxylum bungeanum seed protein. Journal of Nuclear Agricultural Sciences, 28, 2058‒2064. doi:10.11869/j.issn.100-8551.2014.11.2058
- Yang, L.Y., Tian, C.R., & Quan, M.Y. (2008). Classification of Zanthoxylum bungeanum Maxim seeds kernel protein. China Oils and Fat, 33, 16–18.
- Yuan, D.-B., Yang, X.-Q., Tang, C.-H., Zheng, Z.-X., Min, W., Ahmad, I., & Yin, S.-W. (2009). Physicochemical and functional properties of acidic and basic polypeptides of soy glycinin. Food Research International, 42(5–6), 700‒706. doi:10.1016/j.foodres.2009.02.005
- Zeng, W.-C., Zhang, W.-H., He, Q., & Shi, B. (2015). Purification and characterization of a novel antioxidant peptide from bovine hair hydrolysates. Process Biochemistry, 50(6), 948‒954. doi:10.1016/j.procbio.2015.03.007
- Zhang, L., Wu, H.-T., Yang, F.-X., & Zhang, J.-H. (2015). Evaluation of Soxhlet extractor for one-step biodiesel production from Zanthoxylum bungeanum seeds. Fuel Processing Technology, 131, 452‒457. doi:10.1016/j.fuproc.2014.12.025
- Zhang, Y.L., & Xu, Z. (2003). Studies on function properties of soy protein hydrolysate. Food and Fermentation Industries, 29, 27‒30. doi:10.13995/j.cnki.11-1802/ts.2003.09.007
- Zhang, Z.Q., Song, Y., Jiang, T.L., Wu, H.Y., & Liu, X. (2014). Optimization of prickly ash seed protein hydrolyzing by Alcalase proteases to prepare antioxidative peptides. Science and Technology of Food Industry, 35, 179‒183, 187. doi:10.13386/j.issn1002‒0306.2014.11.031
- Zhu, H., Huang, Y.-J., Ji, X.-P., Su, T., & Zhou, Z.-K. (2016). Continuous existence of Zanthoxylum (Rutaceae) in Southwest China since the Miocene. Quaternary International, 392, 224‒232. doi:10.1016/j.quaint.2015.05.020
- Zou, Y., Wang, W., Lia, Q., Chen, Y., Zheng, D.H., Zou, Y.M., … Yang, L.Q. (2016). Physicochemical, functional properties and antioxidant activities of porcine cerebral hydrolysate peptides produced by ultrasound processing. Process Biochemistry, 51(3), 431‒443. doi:10.1016/j.procbio.2015.12.011