ABSTRACT
Currently, there is a worldwide trend toward the food consumption without the use of preservatives. For this reason, biocontrols have emerged as a natural option to replace preservatives. We identify and select native wine yeasts with antimicrobial activity (AA) that work against pathogenic bacteria of food importance. We evaluated the antimicrobial capacity of 103 yeast against Salmonella typhimurium, Listeria monocytogenes, and Escherichia coli, by measuring the growth inhibition. AA was qualitatively determined by measuring the inhibition zone diameter accompanied by death zone of target cells. Results revealed that nine yeast strains showed AA against the three pathogens, being mainly of the genera Pichia, Candida, and Saccharomyces. To determine the type of AA, the viability was assessed by the method of SYTOX Green®. The results suggest that yeast exhibit AA bactericidal type. Thus, the use of yeasts arises as a natural and safe for the biocontrol of bacterial growth alternative.
RESUMEN
Actualmente existe una tendencia mundial por el consumo de alimentos sin el uso de preservantes. De esta forma, biocontroladores han emergido como una opción natural para reemplazar preservantes. En este estudio se identificaron y seleccionaron levaduras vínicas con actividad antimicrobiana (AA) frente a bacterias patógenas. Evaluamos la capacidad antimicrobiana de 103 levaduras contra Salmonella Typhimurium, Listeria monocytogenes y Escherichia coli, determinando la inhibición del crecimiento. La AA se determinó cualitativamente midiendo el diámetro de la zona de inhibición acompañado de la zona de muerte de las células diana. Los resultados mostraron que 9 cepas de levadura mostraron AA frente a los tres patógenos, siendo principalmente de los géneros Pichia, Candida, Saccharomyces y Metschnikowia. Para determinar el tipo de AA, se evaluó la viabilidad usando SYTOX Green®. Los resultados sugieren que las levaduras presentan un tipo de AA bactericida. Así, el uso de levaduras surge como una alternativa natural y segura para el control biológico del crecimiento bacteriano.
KEYWORDS:
Introduction
Food-borne illness (FBI) is caused by the ingestion of foods and/or beverages that have contaminants capable of compromising quality. FBI may also compromise the health of the consumer when is present in sufficient quantities, (Barreto, Sadrés, Rodríguez, & Guevara, Citation2010). Close to 250 agents that cause FBI and present different symptomologies have been described. These agents include bacteria, viruses, fungi, and metals, among others. However, out of these, bacteria stand out as the primary food pathogen (Olea, Díaz, Vquero, Fuentes, & García, Citation2012).
For these reasons, microbiological control is an important consideration that must be assured in the food industry, which is achieved by means of quality control and food safety systems. In this context, various drugs have been developed against disease-causing bacteria. However, their constant application meant that these microorganisms developed mechanisms of resistance to them (Panda, Patra, & Kar, Citation2012). Currently, the food industry incorporates preservatives to guarantee the safety. However, these compounds do not fulfill the consumer demand, where the new trends in the conservation of food is focused on the reduction of preservatives levels and /or the replacement of preservatives by antimicrobial use ‘naturally occurring’ (Hayouni et al., Citation2008) in order to obtain freshest products, as similar as to its original form and safe (Rodríguez, Citation2011).
These results have led to concern among consumers that has resulted in a rejection of foods formulated with chemical preservatives, as exhibited by the trend toward consumption of fresher, healthier products that are most similar to their natural form and which do not have negative side effects (Cleveland, Montville, Nes, & Chikindas, Citation2001; Rodríguez, Citation2011).
For these reasons, it has become necessary to search for new natural antimicrobial agents that originate from plant, animal, and microbial sources (Rodríguez, Citation2011) as alternatives to chemical preservatives, with microorganisms being the primary source of new antimicrobial agents (Leiva, Yáñez, Zaror, Rodríguez, & García-Quintana, Citation2004). In this context, those microorganisms that are capable of controlling the growth of different pathogens or decay microorganisms are called antagonistic microorganisms (Rodov, Citation2007; Sundh & Melin, Citation2011). From the industrial point of view, yeasts are the most important of these microorganisms. They are present in the ecosystem, regularly participate in fermentation processes, and are mostly innocuous to human beings. Among those applications, some genera have been described that have a biocontrolling effect on the growth of other yeast and filamentous fungi. One example is the potential use of antagonistic yeast such as Pichia anomala and Candida spp. for postharvest biological control of putrefying fungi during the storage of fresh fruits (Hernández-Lauzardo, Bautista-Baños, Velázquez-Del Valle, & Hernández-Rodríguez, Citation2007). Various mechanisms have been proposed for this antagonistic activity, including parasitism of the pathogen, the induction of host resistance, and the formation of biofilms. More recently, the participation of reactive oxygen species as a defensive response (Liu, Sui, Wisniewski, Droby, & Liu, Citation2013), competition for nutrients and space, changes in pH, and the production of organic acids (Buyuksirit and Kuleasan, Citation2014; Sigler & Höfer, Citation1991) have been studied. Other studies have focused on the secretion of antimicrobial compounds such as killer toxins, which currently constitute an area of research into natural biocontrollers of industrial interest (Hatoum, Labrie, & Fliss, Citation2012).
Yeasts are promising candidates for a wide range of applications in the food industry (Sánchez et al., Citation2007). In addition, yeasts have a positive image among consumers as a safe source of ingredients and additives for the production of foods (Fleet, Citation2006) and are an interesting model for the study of natural inhibitors of the growth of pathogenic or decay microorganisms. So, considering the yeast potential in food industry, the objective of the current study was to identify native wine yeast with AA against pathogenic bacteria with potential importance in the food industry, and evaluate them quantitatively and qualitatively.
Materials and methods
Microorganisms
shows the 103 selected yeasts with killer activity reported in the literature. All the strains correspond to native wine yeast obtained from the strain collection of the Laboratories of Biotechnology and Applied Microbiology of the University of Santiago de Chile (Santiago, Chile). In addition, five control strains with two different origin were studied: Escherichia coli ATCC25922, Listeria monocytogenes ATCC13932, and S. typhimurium ATCC14028 (strains from the collection); and Salmonella spp 144 and Listeria monocytogenes 145, isolated from a sample of whole chicken from the poultry slaughterhouse in Santiago and from turkey ham from the cured meats factory in Santiago, respectively (Laboratorio de Salud Pública, Ambiental y Laboral. SEREMI de Salud Región Metropolitana, Santiago, Chile).
Table 1. Strains of wine yeasts used in this study.
Tabla 1. Cepas de levaduras vínicas usadas en este estudio.
Culture conditions for the pre-inoculum
The bacterial strains were grown in Luria-Bertani (LB) medium (0.5% yeast extract, 1% tryptone, and 1% NaCl) overnight at 37 ± 1ºC with constant agitation. Then, they were maintained in LB agar (0.5% yeast extract, 1% tryptone, 1% NaCl, and 2% agar) at 4 ± 1°C until use. The analyzed yeasts were grown in yeast extract–peptone–dextrose (YPD) medium (0.5% peptone, 0.5% yeast extract, and 2% glucose) at 28 ± 1ºC with agitation (120 rpm) for 1–3 days, according to the strain. Posteriorly, they were maintained in YPD agar (0.5% peptone, 0.5% yeast extract, 2% glucose, and 4% agar) at 4 ± 1°C until use.
Detection of antimicrobial activity
By means of a classic qualitative method, the ability of each yeast strain to inhibit growth of the three bacterial cultures from the collection was tested. The bacterial cultures were grown at 37 ± 1°C in 2.55% peptone water medium (Merck KGA Darmstadt, Germany) to obtain 1 × 104 cells/mL. The cellular concentration was standardized using a calibration curve by relating known cell concentrations to a given optical density measured at 600 nm in a spectrophotometer (Shimadzu UV-1700, Kyoto, Japan).
The yeasts were grown for 48 h at 28 ± 1ºC with agitation (120 rpm) in glucose yeast-extract broth (GYEB) liquid medium (2% glucose and 0.5% yeast extract) with the pH adjusted to 4.5 using a 0.9 M phosphate-citrate buffer, until a concentration of 1 × 108 cells/mL was obtained. In this case, the pH was modified according to a procedure in the literature (Golubev, Citation2006). The cellular concentration was determined by total cell count in a Neubauer chamber according to the specifications of the manufacturer (Neubauer Improved camera; Precicolor, HBG, Germany).
First, it was necessary to generate a culture medium that would permit the growth of these two types of microorganisms. To this end, different culture media were tested utilizing the agar plate technique. The following solid medium was selected for this work: YGSA (0.5% yeast extract, 2% glucose, 1% tryptone, and 1.5% agar) with 1% NaCl added to the medium with the aim of improving the detection of AA, and 0.003% methylene blue (MB) following the methodology in the literature (Lopes & Sangorrín, Citation2010). The pH was adjusted to 5.0 with a 0.9 M phosphate-citrate buffer. Seeding was carried out by the pour plate method, mixing 1 mL of bacterial culture with 15–20 mL of agar. The tests were performed using the spots technique (Lopes & Sangorrín, Citation2010), whereby 10 μL spot of the yeast culture suspensions were inoculated onto the surface once the agar had gelled. The plates were incubated at 28 ± 1ºC for 72 h.
The lawn of target bacteria was designated as sensitive when a clear zone of growth inhibition surrounding the yeast spot was observed.
Characterization of microbial activity and stability
Of the tested yeast strains, those with inhibitory activity simultaneously against the three bacteria from the ATCC collection under study were selected. To determine whether there was AA in the supernatant of the yeast cultures in the presence of the yeast cells, the yeast cultures at a concentration of 1 × 108 cells/mL were centrifuged at 15,142 × g for 10 min at 4 ± 1°C (Hettich Universal 32R centrifuge) (Mehlomakulu, Setati, & Divol, Citation2014). The supernatant was separated, and the precipitate obtained for each culture was washed and resuspended in 500 μL of sterile distilled water. As previously mentioned the bacterial lawn was inoculated by the pour plate technique and with spot of the supernatant (S), precipitate (P), and total yeast culture (TC).
With the goal of evaluating the nature of the AA, the fresh yeast cultures were subject to thermal denaturation in a thermoregulated bath at 95 ± 1ºC for 10 min. After cooling at room temperature, they were cultured as 10 μL on YGSA + agar plates, pH 5.0, and 0.003% of MB previously inoculated with bacteria at 1 × 104 cells/mL and gelled. They were allowed to dry at room temperature and then incubated at 28 ± 1ºC for 72 h.
In addition, the supernatant from the cultures was filtered (0.22 μm, EdLab, USA) and was concentrated to 10× and 33× by lyophilization (IlShin Bondiro Freeze Dryer, Gyeonggi-do, Korea). The samples were reconstituted in sterile distilled water.
The diameter of the inhibition halo around the spot was used as a measure of inhibition activity; this measurement was recorded in millimeters (mm). The result is expressed as the total diameter of the growth inhibition zone (halo plus inoculum).
Antibacterial activity was also evaluated according to the concept of specific productivity (SP) described by Lopes and Sangorrín (Citation2010). This is defined as the ratio between the size of the inhibition zone and the number of yeast cells tested (aliquots of known concentration).
Another parameter used to evaluate the inhibitory capacity of yeast was the initial inoculum bacteria.
To determine the initial contamination, 1 mL of bacterial suspension at increasing concentrations (1 × 104, 1 × 106, and 1 × 108 cells/mL) was inoculated using the pour plate technique and was left to gel at room temperature. The precipitates of the yeast cultures were inoculated as 10 μL spot on each of the plates contaminated with bacteria. They were dried at room temperature and incubated at 28 ± 1ºC for 72 h. The tests were carried out in triplicate, and the growth inhibition halo was measured in mm.
Quantification of antibacterial activity
A culture with suspensions of 1 × 104 cells/mL of bacteria and 2 × 106 cells/mL of yeast was prepared in tubes with a total of 5 mL of YGSA + NaCl liquid medium at pH 5.0, adjusted with a 0.9 M phosphate-citrate buffer. As a control, 100 µL of bacteria and yeast were cultured separately in the same medium. All the tubes were incubated at 28ºC for 48 h. After this time, serial decimal dilutions in 0.1% peptone water were carried out, which were cultured in 100 µL aliquots on LB plates supplemented with 0.1 mg/mL cycloheximide (viable bacterial count). The controls were inoculated onto LB agar plates with cycloheximide or YPD agar supplemented with 0.1 mg/mL ampicillin.
All tests were performed in duplicate, and the results are reported in colony-forming units (CFU/mL).
Evaluation of cell viability of bacteria
Fresh cultures of yeast and bacteria were utilized to inoculate 96-well microplates as follows: 190 μL at a concentration of 1 × 104 cells/mL of each pathogen with 10 μL at a concentration of 2 × 106 cells/mL of each yeast, including the following controls: (i) 200 μL of water as a standard, (ii) 200 μL of bacterial culture at a concentration of 1 × 104 cells/mL, (iii) 200 μL of yeast culture at a concentration of 1 × 108 cells/mL, (iv) 198 μL of bacterial culture at a concentration of 1 × 104 cells/mL with 2 μL of ampicillin at a concentration of 100 mg/mL, and (v) 60 μL of bacterial culture at a concentration of 1 × 104 cells/mL with 140 μL of 99.8% ethanol. Incubation without agitation was carried out at 28 ± 1ºC for 48 h. Each experiment was performed in triplicate.
After incubation, SYTOX® Green Dead Cell Stain (Invitrogen, USA) was used (Roth, Poot, Yue, & Millard, Citation1997) at a concentration of 50 nm to evaluate the integrity of the bacterial plasma membranes. Imaging of the samples was performed by fluorescence microscopy at an excitation wavelength of 488 nm and emission at 530 nm (Motic BA410 microscope, Moticam Pro Camera).
Statistical analysis
The data were analyzed using the Statgraphics Centurion XVI.I program (Statpoint Technologies, Warrenton, USA) by means of Student’s t-test or analysis of variance as indicated.
Results
Characterization of antimicrobial activity and stability
After evaluating the ability of each yeast strain to inhibit the growth of cultures of the three collections of bacteria (E. coli ATCC25922, L. monocytogenes ATCC13932 and S. typhimurium ATCC14028), nine strains of different species on the bacterial lawn were selected based on the generation of inhibition halos ().
Table 2. Selected wine yeasts with antimicrobial activity.
Tabla 2. Levaduras vínicas con actividad antimicrobiana seleccionadas.
In assessing whether the active antimicrobial agent was secreted into the medium (extracellular), inhibition zone (halo) was not observed when supernatant (S) was inoculated directly over target bacteria, as well as when these supernatants were concentrated 10× and 33× by lyophilization (data not shown). These results suggest that the presence of yeast cells (P) is necessary to observe an antimicrobial effect on the target bacteria and that this yeast must be metabolically active, given that upon heating the total yeast culture (CT), there was no halo formation ().
Figure 1. Characterization of the antimicrobial activity against E. coli. P: precipitate, S: supernatant and CT: culture treatment.
Figura 1. Caracterización de la actividad antimicrobiana contra E. coli. P: precipitado, S: sobrenadante y CT: cultivo tratado.
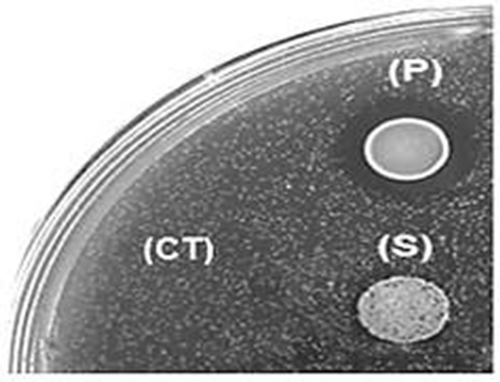
The diameter of the inhibition halo around the spot in mm () was used as a measure of inhibition activity. As control, the diameter corresponding to a droplet of yeast strain without AA was measured.
Figure 2. Diameter of growth inhibition (halo) bacteria by precipitates yeast strains with antibacterial activity. Arabic numerals indicate the strain number according to . The experiments were performed in triplicate and results are the average. Different letters indicate significant at 95% confidence differences.
Figura 2. Diámetro del halo de inhibición del crecimiento de las bacterias por parte de los precipitados de las cepas de levaduras con actividad antibacteriana. Los números arábigos indican el número cepa particular de acuerdo con la . Los experimentos fueron realizados en triplicado y los resultados corresponden al promedio. Letras diferentes indican diferencias significativas al nivel de confianza del 95%.
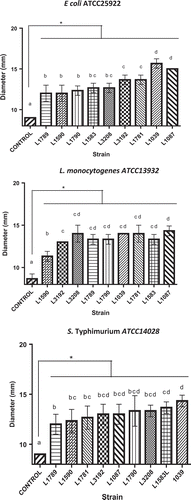
It was observed that all yeasts tested generated inhibition diameters greater control (spot diameter), with values of 10 mm (). For L. monocytogenes ATCC13932 and S. typhimurium ATCC14028, all yeasts tested showed similar inhibition halos and no statistically significant differences were observed. For E. coli ATCC25922, only yeast strains S. cerevisiae L1039 and P. carsonii L1087 generated an inhibition halo statistically superior to all tested yeasts, with values close to 15 mm.
Another parameter evaluated was the SP of AA. The halo of inhibition resulting for each strain was related to the cell concentration of the yeast ().
Table 3. Specific productivity of yeast against bacterial pathogen.
Tabla 3. Productividad Específica de levaduras contra bacterias patógenas.
The results in suggest that SP of target microorganisms seem to respond to yeast strain-specific phenomena considering the statistically significant differences that were observed for the value of SP For example, P. carsoni L1087 and S. cerevisiae L1039 have the highest SP for the case of E. coli ATCC25922, without statistically significant differences between them.
However, the M. pulcherrima L1590 and M. pulcherrima L1781 strains show significant differences in SP values when E. coli ATCC25922 was used suggesting the existence of a strain-dependent antimicrobial effect by the yeast.
Quantification of antibacterial activity
This test was performed by counting viable bacteria after incubation with yeast in YGSA + NaCl liquid medium, pH 5 ().
Figure 3. Bacterial population after 48 h of coculture with yeasts as biocontrol. Arabic numerals indicate the strain number according to . The experiments were performed in triplicate and results are the average. Different letters indicate significant at 95% confidence differences.
Figura 3. Población bacteriana luego de 48 horas de cocultivo con levaduras como biocontrol. Los números arábigos indican el número cepa particular de acuerdo con la . Los experimentos fueron realizados en triplicado y los resultados corresponden al promedio. Letras diferentes indican diferencias significativas al nivel de confianza del 95%.
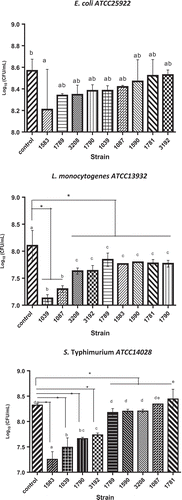
In the case of L. monocytogenes ATCC13932, it was observed that its growth rate is slower than the control (bacterial cultures), when it was grew with the S. cerevisiae L1039 strain, generating a reduction of 1.04 log units compared to the bacterial growth control. In relation to S. typhimurium ATCC14028 culture, C. intermedia L1583 strain decreased 1.14 log units compared to the control. Finally, E. coli ATCC25922 did no statistically significant reduction in growth was observed with the antagonistic strains tested.
Evaluation of cell viability of bacteria cocultured with yeast with antimicrobial activity
Bacterial viability was determined in L. monocytogenes ATCC13932 and S. typhimurium ATCC14028, because these bacteria showed a reduction in growth when cocultured with yeasts tested. The results are shown in .
Figure 4. Cell viability assay of L. monocyotgenes ATCC 13932 and S. typhimurium ATCC 14028 in the presence of previously selected yeast (a) representative micrograph growth of strain L. monocyotgenes ATCC 13932 treated with yeast. 1) L1039, 2) L3192, 3) L3208 4); Control ethanol 70% and 5) untreated control. (b) Field observed by epifluorescence (excitation, 490 nm), (excitation, 490 nm), (c) representative micrograph of growth of the strain S. typhimurium ATCC 14028 treated with yeasts 6) L1039, 7) L1583, 8) L1790, 9) Control ethanol 70%, and 10) untreated control. (d) Field observed by epifluorescence (excitation, 490 nm). 552 The images were adjusted and compared with filter (Photoshop Cs4 Ultra).
Figura 4. Tinción de viabilidad por fluorescencia con SYTOX Green. (a) micrografía representante de crecimiento de la cepa L. monocyotgenes ATCC 13932 tratada con las levaduras. 1) L1039, 2) L3192, 3) L3208 4) control etanol 70% y 5) control sin tratamiento. (b) el mismo campo observado por epifluorescencia (excitación, 490 nm), (c) micrografía representante de crecimiento de la cepa S. typhimurium ATCC 14028 tratada con las levaduras 6) L1039, 7) L1583, 8) L1790, 9) etanol 70% y 10) control sin tratamiento. (d) el mismo campo observado por epi fluorescencia (excitación, 490 nm).
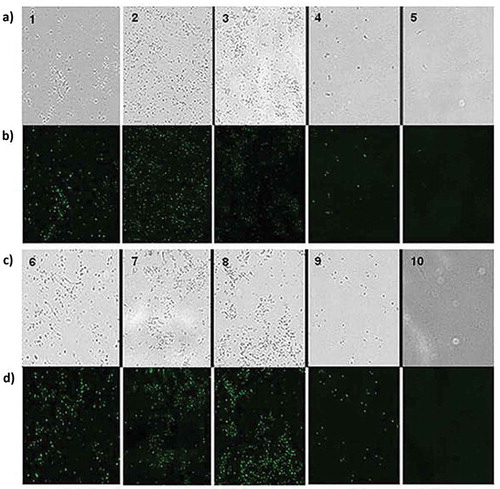
The results indicate that fluorescence of this strains were much higher in comparison with the control conditions (no treatment) (b5,d10), respectively). Comparison of the micrograph with and without a filter revealed that the cells grown in control conditions (untreated) does not fluoresce, suggesting that have no damage to the plasma membrane and thus remained viable during incubation. In b) (1, 2, and 3), it is possible to observe a large percentage of fluorescent cells, suggesting that the AA of the yeast causes cell death by damaging the plasma membrane of bacterial cells. This also occurs upon the incubation of the cells with 70% ethanol (b4,d9)), used as a positive control in the test.
Discussion
Antagonism of yeast with microorganisms mainly occurs due to competition for nutrients, pH changes, and the production of organic acids (Buyuksirit and Kuleasan, Citation2014; Sigler & Höfer, Citation1991), in addition to mechanisms based on the secretion of antimicrobial compounds such as killer toxins. All these methods are an important area of research into natural biocontrollers of industrial interest (Hatoum et al., Citation2012).
In this study, upon concentrating the selected yeast culture supernatants to 33× by lyophilization, no AA was found (data not shown). These results suggest that there is no activity present in the supernatant or that the amount of the antimicrobial compound in the supernatant initially was insufficient for the activity to be detectable. Furthermore, if this compound is of a different nature, such as a volatile organic acid, for example, the possibility exists that the compound was damaged by the procedure, it has been described for alcohols, aldehydes, and sulfur compounds, for which between 20% and 70% can be lost (Her, Kim, Kim, & Lee, Citation2015).
Waema, Maneesri, and Masniyom (Citation2009) studied the antimicrobial effect of Candida krusei on E. coli, Staphylococcus aureus, Bacillus cereus, and S. typhimurium. They observed that the diameters of the inhibition halo varied between 6 and 11 mm for E. coli and S. typhimurium, respectively. Similar results were reported by Roostita, Fleet, Wendry, Apon, and Gemilang (Citation2011), who observed inhibition halos over a range of 35, 8, and 10 mm against Pseudomonas aeruginosa, S. aureus, and E. coli, respectively, using Candida parapsilosis as the biocontrolling agent. The results observed in this study () suggest that the antimicrobial effect is strain dependent and that with increased susceptibility, a greater zone of inhibition is produced, thus differentiating the intensity of the AA.
Conversely, the strains isolated from food, Salmonella spp 144 and L. monocytogenes 145, were more resistant than the bacterial strains from the collection to the inhibitory activity of the nine strains of selected yeast. The formation of a small halo (<2 mm) of sample bacterial cells was observed (data not shown).
It has been reported that wild strains exhibit greater antimicrobial resistance than strains of the collection because the former are exposed to various environmental factors that force them to create efficient mechanisms to adapt to the environment, making them more resistant than a strain from the collection (Davidson & Harrison, Citation2002; Sousa, Machado, Nicolau, & Pereira, Citation2013). For that reason, wild strains were not used for subsequent experiments.
It is noteworthy that the inhibition generated depends not only on the antimicrobial compound or the production thereof by the yeast but also on the target organism. These results suggest the existence of a specific strain-dependent antimicrobial effect by the yeast (Budak, Sahl, & Brandis, Citation1982; Goerges, Aigner, Silakowski, & Scherer, Citation2006). Based on the above results, it was observed that, with the same species of yeast, such as M. pulcherrima L1590 and L1781, significant differences for E coli ATCC25922 were obtained using the same biomass ().
In addition, it was observed that the inhibitory ability of yeast decreased with the increasing of the initial bacterial contamination, which is a determinant factor for testing sensitivity. Similar results indicate that when the lawn of the target strain is too dense and the inoculum of the active strain is small, the inhibition zone size can either be reduced and quickly covered or not developed at all (Golubev, Citation2006).
Quantification of antibacterial activity
Microbial growth rates are a determinant factor for the results growth bacterial in coculture with yeasts as biocontrol, shown in . These are measured in terms of generation time or doubling time, which refers to the time that a microorganism takes to double its mass. Typical doubling times for bacteria vary between 45 min and 1 h; for yeast, these times vary between 1.5 and 2 h (Scott Fogler, Citation1999; Watson, Citation2008), in addition to different adaptation times that required both microorganisms to be changed to a coculture test medium.
Similar results for viable bacterial counts after incubation with yeast in liquid medium () have been reported by Goerges et al. (Citation2006). They evaluated the yeast potential to inhibit growth in L. monocytogenes. They also identified a strain of C. intermedia with the ability to reduce the cell count by 4 log units. And three other strains of C. intermedia and Kluyveromyces marxianus capable of reducing the cell count by 3 log units.
Additionally, a difference in the morphological characteristics of colonies of the three bacteria from the collection was observed. This change was a decrease in size in the colonies of bacteria exposed to antagonistic yeast. This phenomenon might be associated with a macroscopic manifestation of biological strategies that microorganisms adopt to cope with stress conditions, such as substrate shortages, depletion of oxygen, and/or exposure to antimicrobial compounds (Sousa et al., Citation2013) (data not shown).
Evaluation of cell viability of bacteria
SYTOX® Green Dead Cell Stain is impermeable to live cells, but it easily penetrates cells that have damaged plasma membranes, allowing the differentiation of cells and the determination of cell viability by the emission of a fluorescence signal due to binding with double-stranded DNA (Roth et al., Citation1997).
The results of cell viability assay are shown in , in which the fluorescence emission of bacteria with damaged membranes was 10 times higher than the staining of intact organisms. In addition to not fluorescing in aqueous media, the compound was applied directly on a sample of the culture medium.
Several studies show that yeast strains are capable of inhibiting the growth of unrelated microorganisms such as bacteria. The mechanism associated with this inhibition is quite controversial. Some authors have reported that the killer phenomenon, which had previously been limited to yeast–yeast interaction, might occur between yeast and bacteria, noting that killer strains are capable of inhibiting the growth of bacteria such as E. coli, S. aureus, L. monocytogenes, and Klebsiella oxytoca (Bajaj, Raina, & Singh, Citation2013; Leverentz et al., Citation2006; Meneghin, Reis, & Ceccato-Antonini, Citation2010; Polonelli & Morace, Citation1986). However, it has been suggested that S. cerevisiae produces substances with AA against malolactic bacteria and that one of these substances has lysozyme characteristics (Dick, Molan, & Eschenbruch, Citation1992). In addition, compounds produced by Candida spp. and Geotrichum candidum capable of inhibiting the growth of L. monocytogenes have been characterized. These were identified as organic acids that cause changes in the structure and rigidity of the cell wall of L. monocytogenes, causing its disintegration (Dieuleveux, Lemarinier, & Gueguen, Citation1998; Narayanan & Rao, Citation1974, Citation1976).
Conclusion
It can be concluded that AA is not restricted to a single species of yeast. However, this activity was only present in some strains of a species, which accounted for 8.7% of the tested strains, showing some degree of growth inhibition against Gram-positive and Gram-negative bacteria when in contact with viable cells. These exploratory results are not sufficient to attribute AA to a particular compound or mechanism. However, this study set directions on species-specific antimicrobial inhibition that can be used in further testing, in order to clarify how these yeasts are capable of inhibiting the growth of the bacteria, to strengthen and enhance their effect.
Acknowledgments
This work was supported by grant FONDECYT 11140194, grant Millennium Nucleus for Fungal Integrative and Synthetic Biology (MN-FISB) 120043, CONICYT Postdoctorado/FONDECYT 3140083 and USA1555-USACH.
Disclosure statement
No potential conflict of interest was reported by the authors.
Additional information
Funding
References
- Bajaj, B.K., Raina, S., & Singh, S. (2013). Killer toxin from a novel killer yeast Pichia kudriavzevii RY55 with idiosyncratic antibacterial activity. Journal of Basic Microbiology, 53(8), 645–656. doi:10.1002/jobm.201200187
- Barreto, G., Sadrés, M., Rodríguez, H., & Guevara, G. (2010). Agentes bacterianos asociados a brotes de Enfermedades Trasmitidas por Alimentos (ETA) en Camagüey, Cuba, durante el período 2000-2008 - Bacterial agents associated to foodborne outbreaks in Camagüey, Cuba, in the period 2000-. REDVET. Revista electrónica de Veterinaria 1695-7504. Volumen 11 Número 02.
- Budak, A., Sahl, H., & Brandis, H. (1982). Antibacterial activities of Candida yeasts. Partial purification and characterization of the active substance of Candida guilliermondii. Zentralblatt Fur Bakteriologie, Mikrobiologie Und Hygiene. 1. Abt. Originale. A, Medizinische Mikrobiologie, Infektionskrankheiten Un Parasitologie, 253(3), 390–396. doi:10.1016/S0174-3031(82)80075-9
- Buyuksirit, T., & Kuleasan, H. (2014). Antimicrobial agents produced by yeasts. International Journal of Biological, Biomolecular, Agricultural, Food and Biotechnological Engineering, 8(10), 1013–1016.
- Cleveland, J., Montville, T.J., Nes, I.F., & Chikindas, M.L. (2001). Bacteriocins: Safe, natural antimicrobials for food preservation. International Journal of Food Microbiology, 71(1), 1–20. doi:10.1016/S0168-1605(01)00560-8
- Davidson, P.M., & Harrison, M.A. (2002). Resistance and adaptation to food antimicrobials, sanitizers, and other process controls. Food Technology, 56(11), 69–78.
- Dick, K., Molan, P., & Eschenbruch, R. (1992). The isolation from Saccharomyces cerevisiae of two antibacterial cationic proteins that inhibit malolactic bacteria. VITIS - Journal of Grapevine Research, 31, 105–116.
- Dieuleveux, V., Lemarinier, S., & Gueguen, M. (1998). Antimicrobial spectrum and target site of D −3-phenyllactic acid. International Journal of Food Microbiology, 40, 177–183.
- Fleet, G. (2006). The commercial and community significance of yeasts in food and beverange production. In A. Querol & G.H. Fleet (Eds.), Yeasts in food and beveranges (pp. 1–12). Berlin: Springer-Verlag Berlin Heidelberg. doi:10.1007/978-3-540-28398-0
- Goerges, S., Aigner, U., Silakowski, B., & Scherer, S. (2006). Inhibition of Listeria monocytogenes by food-borne yeasts. Applied and Environmental Microbiology, 72(1), 313–318. doi:10.1128/AEM.72.1.313-318.2006
- Golubev, W. I. (2006). Antagonistic interactions among yeasts. In G. Péter & C. Rosa (Eds.), Biodiversity and ecophysiology of yeasts (pp. 197–219). Berlin: Springer.
- Hatoum, R., Labrie, S., & Fliss, I. (2012). Antimicrobial and probiotic properties of yeasts: From fundamental to novel applications. Frontiers in Microbiology, 3(DEC), 1–12. doi:10.3389/fmicb.2012.00421
- Hayouni, E.A., Chraief, I., Abedrabba, M., Bouix, M., Leveau, J.-Y., Mohammed, H., & Hamdi, M. (2008). Tunisian Salvia officinalis L. and Schinus molle L. essential oils: Their chemical compositions and their preservative effects against Salmonella inoculated in minced beef meat. International Journal of Food Microbiology, 125(3), 242–251. doi:10.1016/j.ijfoodmicro.2008.04.005
- Her, J.-Y., Kim, M.S., Kim, M.K., & Lee, K.-G. (2015). Development of a spray freeze-drying method for preparation of volatile shiitake mushroom (Lentinus edodes) powder. International Journal of Food Science & Technology, 50(10), 2222–2228. doi:10.1111/ijfs.12888
- Hernández-Lauzardo, A.N., Bautista-Baños, S., Velázquez-Del Valle, M.G., & Hernández-Rodríguez, A. (2007). Uso de Microorganismos Antagonistas en el Control de Enfermedades Postcosecha en Frutos. Revista Mexicana de Fitopatología, 25(1), 66–74.
- Leiva, S., Yáñez, M., Zaror, L., Rodríguez, H., & García-Quintana, H. (2004). Antimicrobial activity of actinomycetes isolated from aquatic environments in Southern Chile. Revista Médica de Chile, 132, 151–159.
- Leverentz, B., Conway, W.S., Janisiewicz, W., Abadias, M., Kurtzman, C.P., & Camp, M.J. (2006). Biocontrol of the food-borne pathogens Listeria monocytogenes and Salmonella enterica serovar poona on fresh-cut apples with naturally occurring bacterial and yeast antagonists. Applied and Environmental Microbiology, 72(2), 1135–1140. doi:10.1128/AEM.72.2.1135-1140.2006
- Liu, J., Sui, Y., Wisniewski, M., Droby, S., & Liu, Y. (2013). Review: Utilization of antagonistic yeasts to manage postharvest fungal diseases of fruit. International Journal of Food Microbiology, 167, 153–160. Elsevier B.V. doi:10.1016/j.ijfoodmicro.2013.09.004
- Lopes, C., & Sangorrín, M. (2010). Optimization of killer assays for yeast selection protocols. Revista Argentina de Microbiología (2010), 306, 298–306.
- Mehlomakulu, N.N., Setati, M.E., & Divol, B. (2014). Characterization of novel killer toxins secreted by wine-related non-Saccharomyces yeasts and their action on Brettanomyces spp. International Journal of Food Microbiology, 188, 83–91. doi:10.1016/j.ijfoodmicro.2014.07.015
- Meneghin, M.C., Reis, V.R., & Ceccato-Antonini, S.R. (2010). Inhibition of bacteria contaminating alcoholic fermentations by killer yeasts. Brazilian Archives of Biology and Technology, 53(5), 1043–1050. doi:10.1590/S1516-89132010000500006
- Narayanan, T.K., & Rao, G.R. (1974). Production of 2-phenethyl alcohol and 2-phenyllactic acid in Candida species. Biochemical and Biophysical Research Communications, 58(3), 728–735. doi:10.1016/S0006-291X(74)80478-X
- Narayanan, T.K., & Rao, G.R. (1976). Beta-indoleethanol and beta-indolelactic acid production by Candida species: Their antibacterial and autoantibiotic action. Antimicrobial Agents and Chemotherapy, 9(3), 375–380. doi:10.1128/AAC.9.3.375
- Olea, A., Díaz, J., Vquero, A., Fuentes, R., & García, M. (2012). Vigilancia de brotes de enfermedades transmitidas por alimentos en Chile. Revista Chilena Infectología, 29(5), 504–510.
- Panda, S.K., Patra, A.K., & Kar, R.N. (2012). Monitoring of multiple drug-resistant pathogens in a selected stretch of Bay of Bengal, India. Environmental Monitoring and Assessment, 184, 193–200. doi:10.1007/s10661-011-1958-1
- Polonelli, L., & Morace, G. (1986). Reevaluation of the yeast killer phenomenon. Journal of Clinical Microbiology, 24(5), 866–869.
- Rodov, V. (2007). Biotechnological approaches to improving quality and safety of fresh-cut fruit and vegetable products. Acta Horticulturae, (746), 181–194. doi:10.17660/ActaHortic.2007.746.20
- Rodríguez, E. (2011). Uso de agentes antimicrobianos naturales en la conservación de frutas y hortalizas. Ra Ximhai, 7(1), 153–170.
- Roostita, L.B., Fleet, G.H., Wendry, S.P., Apon, Z.M., & Gemilang, L.U. (2011). Determination of yeasts antimicrobial activity in milk and meat products. Advance Journal of Food Science and Technology, 3(6), 442–445.
- Roth, B., Poot, M., Yue, S., & Millard, P. (1997). Bacterial viability and antibiotic susceptibility testing with SYTOX green nucleic acid stain. Applied And Environmental Microbiology, 63(6), 2421–2431.
- Sánchez, M.I., Santos, A., Dustet, J.C., Guerra, G., León, T., Ramos-Leal, M., … Casado, G. (2007). Estudio fisiológico de una cepa de levadura con potencialidades para el enriquecimiento proteico del bagazo de caña de azúcar. Revista CENIC Ciencias Biológicas, 38(1), 39–43.
- Scott Fogler, H. (1999). Elementos de ingeniería de las reacciones químicas [Elements of chemical reaction engineering] (3rd ed.). Upper Saddle River, NJ: Prentice Hall PTR; London: Prentice-Hall.
- Sigler, K., & Höfer, M. (1991). Mechanisms of acid extrusion in yeast. Biochimica Et Biophysica Acta, 1071(4), 375–391. doi:10.1016/0304-4157(91)90003-F
- Sousa, A.M., Machado, I., Nicolau, A., & Pereira, M.O. (2013). Improvements on colony morphology identification towards bacterial profiling. Journal of Microbiological Methods, 95(3), 327–335. doi:10.1016/j.mimet.2013.09.020
- Sundh, I., & Melin, P. (2011). Safety and regulation of yeasts used for biocontrol or biopreservation in the food or feed chain. Antonie Van Leeuwenhoek, International Journal of General and Molecular Microbiology, 99(1), 113–119. doi:10.1007/s10482-010-9528-z
- Waema, S., Maneesri, J., & Masniyom, P. (2009). Isolation and identification of killer yeast from fermented vegetables. Asian Journal of Food and Agro-Industry, 2(4), 126–134.
- Watson, J. (2008). Biología molecular del gen [Molecular Biology of the Gene]. San Francisco, CA: Pearson/Benjamin Cummings.