ABSTRACT
Operational conditions such as temperature and pH are well known to influence the production of biosurfactants, bacteriocins, and lactic acid by lactic acid bacteria. The bacterial strain Lactococcus lactis subsp. lactis CECT-4434 was used to investigate the effects of temperature (30°C, 37°C and 40°C) and pH control on the production of these biomolecules, in order to establish whether they are able to preferentially address its metabolism towards one product or another. It was observed that the pH control within the range 5.0–5.3 favored the production of lactic acid, which was 38% higher than that obtained without control. The bacteriocin production was higher in the temperature range between 30°C and 37°C, evidenced by the formation of inhibition zones against Lactobacillus sakei and Staphylococcus aureus, 5–22% and 4–14% higher, respectively, compared with those achieved at 40°C. Finally, the best results for biosurfactant synthesis occurred at 37°C without pH control, with a surface tension reduction between 22.5 and 24.7 mN/m after 6–24 h of culture, respectively.
RESUMEN
Es bien conocido que determinadas condiciones operacionales, tales como la temperatura y el pH, influyen en la producción de biosurfactantes, bacteriocinas y ácido láctico por parte de las bacterias ácido lácticas. En este trabajo se empleó la cepa bacteriana Lactococcus lactis subsp. lactis CECT-4434 para investigar los efectos de la temperatura (30, 37 y 40°C) y el control del pH en la producción de estas biomoléculas, con el fin de determinar como influyen en su metabolismo. Se observó que a pH controlado, dentro del rango 5,0–5,3, se favorecía la producción de ácido láctico, que resultó 38% superior a la obtenida sin control. La producción de bacteriocina fue mayor dentro del rango de temperatura 30–37ºC, evidenciada por la formación de zonas de inhibición frente a las cepas indicadoras Lactobacillus sakei y Staphylococcus aureus, 5–22% y 4–14% respectivamente superiores que aquellos valores alcanzados a 40°C. Finalmente, se observaron los mejores resultados para la síntesis de biosurfactantes a 37ºC sin control de pH, con una reducción de la tensión superficial entre 22,5 y 24,7 mN/m tras 6–24 h de cultivo respectivamente.
1. Introduction
Lactic acid bacteria (LAB) are Gram-positive cocci or rods, which are Generally Recognized as Safe by the FDA (US Food and Drug Administration) and produce lactic acid as the major or sole fermentation product (Von Wright & Axelsson, Citation2012). They comprise a large number of species involved in the production of fermented milk products that belong mainly to the genera Lactobacillus, Lactococcus, Leuconostoc, Pediococcus, and Streptococcus (Mayo et al., Citation2010).
It is important to note that although lactic acid is the major metabolite produced by LAB, other biomolecules can simultaneously be produced depending on the species such as peptides called bacteriocins (Arauz, Jozala, Mazzola, & Penna, Citation2009). Bacteriocins produced by LAB are proteins or protein complexes with antimicrobial activity and therapeutic potential, even against the cancer (Moreno, Lerayer, Baldini, & Leitão, Citation2000; Panesar, Kennedy, Gandhi, & Bunko, Citation2007; Parada, Caron, Medeiros, & Soccol, Citation2007). The economic importance of bacteriocins in food preservation has increased their exploitation as food preservatives (Rosa & Franco, Citation2002).
Currently, the most widely studied and industrially exploited bacteriocin is nisin, which is produced by certain strains of the probiotic bacterium Lactococcus lactis, is classified as a lantibiotic, contains 34 amino acids and has a molecular weight of 3.5 kDa (Arauz et al., Citation2009; Oladunjoye, Singh, & Ijabadeniyi, Citation2016). Nisin is able to inhibit pathogens and contaminants and is the only bacteriocin internationally legalized for use as a natural biopreservative of foods, since its intake does not exert toxic effects to the human body, does not alter the ecology of the gastrointestinal tract, and, then, does not present the same risks related to the use of antibiotics (Yoneyama & Katsumata, Citation2006).
Several studies have reported LAB as producers not only of bacteriocins but also of biosurfactants and stressed their potential application in food processing and public health (Gudiña, Teixeira, & Rodrigues, Citation2011; Portilla-Rivera, Moldes, Torrado, & Domínguez, Citation2008; Rodrigues, Teixeira, & Oliveira, Citation2006b; Rodrigues, Teixeira, Oliveira, & Van Der Mei, Citation2006a; Rodríguez, Salgado, Costés, & Domínguez, Citation2010; Rodríguez-Pazo, Salgado, Cotéz-Diéguez, & Domínguez, Citation2013; Sabo, Converti, Todorov, Domínguez, & Oliveira, Citation2015). Recent studies describe the ability of different probiotic LAB to produce biosurfactants extracellularly, intracellularly, or even adsorbed on their plasma membrane (Ceresa et al., Citation2015; Galabova, Sotirova, Karpenko, & Karpenko, Citation2014; Rodrigues, Teixeira, Van De Mei, & Oliveira, Citation2006c; Sharma, Saharan, Chauhan, Bansal, & Procha, Citation2014), among which the best known is just L. Lactis (Machado, Mohideen, Saravanakumari, & Prabhavathi, Citation2013).
The commercial interest and industrial use of biosurfactants are increasing due to their advantages over chemically synthesized surfactants, including high biodegradability, low toxicity, and effectiveness even at extreme temperatures and pH (Bustos, De La Torre, Moldes, Cruz, & Domínguez, Citation2007; Souza, Penna, & Oliveira, Citation2014). Furthermore, biosurfactants also find a wider range of applications in the pharmaceutical, cosmetic, food and oil industries, as dispersant, foaming, emulsifying, micelle-forming agents, and for their anti-adhesive action against pathogens (Banat et al., Citation2010; Cortés-Sánchez, Hernández-Sánchez, & Jaramillo-Flores, Citation2013; Jhaa, Sanket, Joshia, & Geetha, Citation2016).
Since this bacterium is able to simultaneously produce bacteriocins and biosurfactants (Rodríguez et al., Citation2010), the need was seen in this study to investigate factors that may address to the production of one metabolite or the other depending on desired product.
Cell growth and metabolite accumulation are highly influenced by the culture medium composition and the operating conditions such as pH, temperature, dissolved oxygen concentration, and degree of aeration. Therefore, the influence of different parameters makes it difficult to optimize biotechnological processes (Chen, Juang, & Wei, Citation2015; Desai & Banat, Citation1997; Li, Bai, Cai, & Ouyang, Citation2002). In some cases, biosurfactant production can be mainly regulated by pH and growth temperature (Mulligan, Sharma, Mudhoo, & Makhijani, Citation2014).
LAB have optimum growth temperature between 20°C and 45°C and survive at pH 5 or less, hence demonstrating high acid tolerance, depending on the selected microorganism (Hofvendahl, Niel, & Hahn-Hägerdal, Citation1999). The importance of pH control in the simultaneous biosurfactant and bacteriocin production by L. lactis was studied by Rodríguez et al. (Citation2010), who observed that at 30°C under pH control at 6.8 only biosurfactant was produced, while both metabolites were produced without pH control. However, these authors did not assess the effect of temperature, which can exert great influence on the production of these biomolecules, favoring the production of one or the other.
In this context, the objective of this study was to identify the effects of temperature (30°C, 37°C and 40°C) and pH control on the production of biosurfactant, bacteriocins, and lactic acid by L. lactis subsp. lactis CECT-4434, which has the ability to simultaneously synthesize these metabolites, thus contributing to the profitability of the biotechnological process under investigation.
2. Materials and methods
2.1. Microorganisms and culture conditions
Lactococcus lactis subsp. lactis CECT-4434 and the indicator strain Staphylococcus aureus CECT-239 were obtained from the Spanish Type Culture Collection (Valencia, Spain), while the other indicator strain Lactobacillus sakei ATCC 15521 from the American Type Culture Collection (Manassas, VA, USA). All strains were maintained under cryopreservation at −70°C in culture media specific for each microorganism, supplemented with 20% glycerol (v/v).
For L. lactis inoculum, 1.0 mL of stock culture was incubated at 37°C and 100 rpm in 250 mL-Erlenmeyer flasks containing Man-Rogosa-Sharpe broth (MRS, Difco, NJ, USA) composed of (g/L): 10.0 peptone, 10.0 meat extract, 5.0 yeast extract, 20.0 dextrose, 1.0 polysorbate, 2.0 ammonium citrate, 5.0 sodium acetate, 0.1 magnesium sulfate, 0.05 manganese sulfate, and 2.0 dibasic potassium phosphate.
After reaching an optical density (O.D.) of 0.8–0.9 at 600 nm, 12 mL of this cell suspension were inoculated into 500 mL Erlenmeyer flasks containing 120 mL of MRS broth. The flasks were incubated under stirring at 100 rpm for 24 h at 30°C, 37°C or 40°C, with or without pH control at 5.0–5.3 using 1.0% CaCO3, and samples of culture medium were collected at fixed times (6, 16, and 24 h). The experiment was performed in triplicate.
The pre-inoculum of L. sakei and S. aureus was carried out in MRS broth (Difco) and Tryptic Soy Broth (TSB, Difco), respectively, under the same conditions described above for L. lactis, up to the achievement of O.D. of 0.3–0.4 at 600 nm.
2.2. Bacteriocin extraction
After centrifugation (15.000 g for 15 min at 4°C) of fermented broth samples, the supernatant with the pH adjusted to 6.0–6.5, was filtered with 0.45 µm-pore diameter membrane filter (Millipore, Billerica, MA, USA), transferred to Eppendorf tubes, heating for 3 min at 80°C to inhibit proteases, and stored at 4°C for analysis.
The antimicrobial activity of produced bacteriocin was analyzed by the diffusion technique in wells. 150 μL of each indicator strain, L. sakei ATCC 15521 or S. aureus CECT-239, were inoculated in MRS and TSB, respectively, both containing 1% agar. Wells of 7 mm in diameter were cut in the gelled medium with the aid of a sterile metal cylinder and filled with 50 μL of each extract obtained as previously described. After the plates had been incubated for 24 h at 37°C, the inhibition zones evidenced by bright areas around the wells (halos) were measured with the aid of a caliper.
2.3. Extraction and analysis of biosurfactant
After centrifugation at 4470 g for 10 min at 10°C, the supernatant was recovered for measurement of extracellular biosurfactant. To extract intracellular biosurfactant and/or the adhered one, cells were washed with deionized water, resuspended in phosphate saline buffer PBS (10 mM KH2PO4/K2HPO4 and 150 mM NaCl, pH 7.0) at a ratio of 1.0 mL of PBS per 6.0 mL of the initial sample, and subjected to stirring for 2 h at 25°C. After that time, samples were centrifuged at 4470 g for 10 min and 25°C, and the supernatants surface tension (ST) was measured.
ST either of cell-free culture medium or extract in PBS was measured by the ring method, using a tensiometer, model Krüss K9 (Krüss, Hamburg, Germany), equipped with platinum de Noüy ring with 1.9 cm diameter. ST values represent the average of three independent measurements at 25°C.
2.4. Determinations of glucose and lactic acid
After filtration of samples through membrane filters (Millipore) with 0.45 µM-pore diameter, glucose and lactic acid concentrations were determined at 30°C by a high performance liquid chromatography (HPLC), model LC-20A Prominence (Shimadzu, Kyoto, Japan), equipped with a separation column, model Supelcogel C-610H (Supelco, Bellefonte, PA, USA) (H 30 cm × 7.8 mm, 9 µM particle), using as eluent an aqueous solution of 1% H3PO4 at a flow rate of 0.5 mL/min. Glucose was quantified using standard curves obtained beforehand with high purity standard solutions for use in HPLC.
3. Results and discussion
Various external parameters can interfere with the production and effective throughput of both biosurfactants and bacteriocins, including culture medium composition, temperature, pH, and incubation time (Cortés-Sánchez et al., Citation2013).
In this context, a series of batch fermentations was performed in MRS broth in order to identify the effects of temperature (30°C, 37°C, and 40°C) and pH control on the productions of biosurfactant, bacteriocin, and lactic acid by L. lactis CECT-4434, to determine the best conditions for producing each of these metabolites.
3.1. Lactic acid production
shows the pH values obtained as a function of time in runs carried out with or without pH control in the range 5.0–5.3 at different temperatures. It can be seen that, in the absence of control, the pH values at 30°C were close to those obtained at 37°C, while at 40°C there was a smaller reduction in pH over time, probably due to the scarce of lactic acid production (under 15 g/L in all cases), as it can be seen in , resulting from a metabolism slowdown at this relatively high temperature. On the other hand, the pH control favored the uptake of glucose as carbon source, allowing an increase of lactic acid concentration of 38.8% at 30°C, 40.3% at 37°C, and 47.4% at 40°C compared with runs performed without control, with a maximum value (23.4 g/L) after 16 h of cultivation at 40°C. As expected by the homofermentative metabolism of L. lactis, lactic acid production was proportional to glucose consumption.
Table 1. pH changes as a function of time during Lc. lactis cultures carried out at 30°C, 37°C, and 40°C, with or without pH control.
Tabla 1. Variación del pH a lo largo del tiempo durante el cultivo de Lc. Lactis llevado a cabo a 30, 37 y 40ºC, con y sin control de pH.
Figure 1. Glucose consumption (•), surface tension reduction (∎), lactic acid production (♦). Runs without pH control at: (a) 30°C, (b) 37°C, and (c) 40°C. Runs with pH control at: (d) 30°C, (e) 37°C, and (f) 40°C.
Figura 1. Consumo de glucosa (•), reducción de tensión superficial (∎), producción de ácido láctico (♦). Ensayos sin control del pH: (a) 30°C, (b) 37°C and (c) 40°C. Ensayos con control del pH: (d) 30°C, (e) 37°C y (f) 40°C.
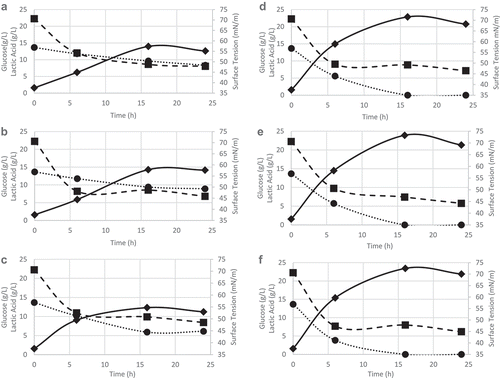
The same effect was observed by Rodríguez et al. (Citation2010) for the same strain, which produced, in bioreactor under pH control (6.8), 23 g/L of lactic acid in 6 h at 30°C, with complete glucose consumption, while the absence of control reduced the consumption and delayed lactic acid production (7.8 g/L in 30 h).
3.2. Bacteriocin production
Penna, Jozala, Gentille, Pessoa, and Cholewa (Citation2006) observed a strong influence of pH of the culture medium on nisin release by L. lactis ATCC 11454, that is, at pH <6, more than 80% of it was released extracellularly, while at pH >6, it was mainly linked to cell membrane or intracellularly trapped. According to these results, nisin production by L. lactis subsp. lactis IT-4 achieved a maximum at pH 5 and decreased at higher pH (Sadiq et al., Citation2014), which confirms the validity of investigating, in the present study, the effect of pH control in the range 5.0–5.3.
shows that there was no statistically significant difference between the values of extracellular activity of bacteriocin produced by L. lactis CECT-4434 in cultured performed at 30°C and 37°C, with or without pH control, expressed as inhibition diameter of growth of Lactobacillus sakei ATCC 15521 (9.0–10.6 mm) and Staphylococcus aureus CECT-239 (12.5–14.0 mm). The lowest activity was observed at 40°C, especially without pH control, resulting in inhibition halos of only 7.5 mm against L. sakei and 11.0 mm against S. aureus after 24 h of cultivation. It is also noteworthy that the largest inhibition diameters were obtained with broth samples mainly taken after 6 h, that is, during the exponential growth phase (), while the narrowest one with stationary phase samples, suggesting possible bacteriocin degradation by the microorganism under conditions of substrate starvation.
Table 2. Inhibition zones (mm) of Lactobacillus sakei ATCC 15521 and Staphylococcus aureus CECT-239 growth induced by Lactococcus lactis CECT-4434 bacteriocin produced at different temperatures, with and without pH control.
Tabla 2. Zonas de inhibición de crecimiento (mm) de las cepas Lactobacillus sakei ATCC 15521 y Staphylococcus aureus CECT-239 por la bacteriocina producida por Lactococcus lactis CECT-4434 a diferentes temperaturas, con y sin control de pH.
Table 3. Lactococcus lactis CECT-4434 biomass concentration (g/L) and reduction of surface tension (STred) as a result of biosurfactant production at different temperatures, with and without pH control.
Tabla 3. Concentración de biomasa de Lactococcus lactis CECT-4434 (g L) y reducción de la tensión superficial (STred) como resultado de la producción de biosurfactante a diferentes temperaturas, con y sin control del pH.
These results agree qualitatively with those of Sadiq et al. (Citation2014), who observed at 25–30°C maximum antimicrobial activity of L. lactis PI-2 (8.0–10.0 mm) nisin against L. lactis IT-4 and a decrease to approximately 6.0 mm at 33–37°C. Comparison with such a thermal profile suggests a certain thermostability of bacteriocin produced in this study.
3.3. Biosurfactant production
In addition to bacteriocins, LAB may produce biosurfactants extracellularly, intracellularly, or adsorbed on their plasma membrane. Although there are many studies describing the ability of different LAB to produce biosurfactants, one of the most important biosurfactant producer is L. Lactis (Rodrigues et al., Citation2006a)
In particular, Rodrigues et al. (Citation2006a) reported the production of extracellular biosurfactant by L. lactis 53, while Rodríguez et al. (Citation2010) reported no extracellular biosurfactant production by L. lactis CECT-4434, even though observed the production of biosurfactant adsorbed onto the cells after extraction with PBS (pH 7).
Based on these results, we investigated in this study the biosurfactant production by the latter strain measuring the capacity of reducing the culture medium surface tension (STred), either with or without pH control, but the ST variations (not shown) were not statistically significant, thus confirming the observations of Rodríguez et al. (Citation2010).
On the other hand, runs performed either with or without pH control showed interesting results in terms of production of intracellular biosurfactant and/or biosurfactant adsorbed onto the cell membrane after extraction with PBS (pH 7.0) ().
Biosurfactant production was associated to cell growth in the present study. Despite of the slow growth of L. lactis CECT-4434 at 40°C (0.1–4.6 g/L), biosurfactant production was satisfactory in the culture performed with pH control (), being STred after 6 h of cultivation (23.3 mN/m) appreciably higher than without control (19.1 mN/m). Indeed, it is worth remembering that a substance can be considered a biosurfactant if is able to reduce the ST by no less than 8 mN/m (Van Der Vegt, Van Der Mei, Noordmans, & Busscher, Citation1991).
The pH control allowed for better cell growth at all tested temperatures (21.5% higher as an average), and the highest cell concentration (7.1 ± 0.1 g/L) was obtained at 37°C after 16 h. Possibly, without any pH control, the acidity of the medium may have inhibited the growth of microorganism (Parada et al., Citation2007).
The culture at 30°C with pH control showed higher STred after only 6 h (21.1 mN/m) compared with the one without pH control, which took more than twice as long to achieve the same reduction at the same temperature. On the other hand, cultivations at 37°C showed the opposite trend. In particular, with pH control STred after 6 h of culture (20.0 mN/m) was 11% lower and cell growth (6.0 g/L) 20% higher than without pH control.
In agreement with these results, Rodrigues et al. (Citation2006a) observed that biosurfactant production by L. lactis 53 was associated with growth. However, Rodríguez et al. (Citation2010) reported that, although ST decreased since the beginning of fermentation as a result of metabolism and growth, it achieved its maximum value (31.1 mN/m) at pH 6.8 in the early stationary phase, but then decreased to only 17.5 mN/m due to possible consumption of biosurfactant as a carbon source under conditions of substrate starvation. No similar decrease was observed in this study during the stationary phase of growth.
4. Conclusions
In this work, we studied the behavior of L. lactis in cultures performed at different temperatures with or without pH control. Under both conditions, the temperature range 30–37°C allowed for the largest bacteriocin production, as evidenced by the formation of inhibition zones against Lactobacillus sakei and Staphylococcus aureus 5–22% and 4–14% higher, respectively, compared with 40°C. At temperature of 37°C, without pH control, would be the best choice for mainly favoring biosurfactant production, as evidenced by a reduction in ST between 22.5 and 24.7 mN/m after 6–24 h of culture. In addition, this temperature would allow producing bacteriocin with satisfactory antimicrobial activity (mean inhibition zone of 9.3 and 12.9 mm against L. sakei and S. aureus, respectively) and lactic acid at concentration of 14.28 g/L, thus contributing to the economy of the process.
Acknowledgments
The authors would like to thank FAPESP (São Paulo Research Foundation) for the financial support by the grant number 2013/15065-0 and to CAPES (Coordination of High-Level Personnel Training, Brazil) for Prof. J. M. Domínguez fellowship as special visiting researcher (Science without Borders Program, project number 88881.062223/2014-01).
Disclosure statement
No potential conflict of interest was reported by the authors.
Additional information
Funding
References
- Arauz, L.J., Jozala, A.F., Mazzola, P.G., & Penna, T.C.V. (2009). Nisin biotechnological production and application: A review. Trends in Food Science & Technology, 20, 146–154. doi:10.1016/j.tifs.2009.01.056
- Banat, I., Franzetti, A., Gandolfi, I., Bestetti, G., Martinotti, M., Fracchia, L., … Marchant, R. (2010). Microbial biosurfactants production, applications and future potential. Applied Microbiology and Biotechnology, 87, 427–444. doi:10.1007/s00253-010-2589-0
- Bustos, G., De La Torre, N., Moldes, A.B., Cruz, J.M., & Domínguez, J.M. (2007). Revalorization of hemicellulosic trimming vine shoots hydrolyzates trough continuous production of lactic acid and biosurfactants by L. pentosus. Journal of Food Engineering, 78, 405–412. doi:10.1016/j.jfoodeng.2005.10.008
- Ceresa, C., Tessarolo, F., Caola, I., Nollo, G., Cavallo, M., Rinaldi, M., & Fracchia, L. (2015). Inhibition of Candida albicans adhesion on medical-grade silicone by a Lactobacillus-derived biosurfactant. Journal of Applied Microbiology, 118, 1116–1125. doi:10.1111/jam.12760
- Chen, W.C., Juang, R.S., & Wei, Y.H. (2015). Applications of a lipopeptide biosurfactant, surfactin, produced by microorganisms. Biochemical Engineering Journal, 103, 158–169. doi:10.1016/j.bej.2015.07.009
- Cortés-Sánchez, A., Hernández-Sánchez, H., & Jaramillo-Flores, M.E. (2013). Biological activity of glycolipids produced by microorganisms: New trends and possible therapeutic alternatives. Microbiological Research, 168, 22–32. doi:10.1016/j.micres.2012.07.002
- Desai, J.D., & Banat, I.M. (1997). Microbial production of surfactant and their commercial potential. Microbiology and Molecular Biology Reviews, 61, 47–64.
- Galabova, D., Sotirova, A., Karpenko, E., & Karpenko, O. (2014). Role of microbial surface-active compounds in environmental protection. In M. Fanun (ed.), The role of colloidal systems in environmental protection (pp. 41–83). Amsterdam: Elsevier.
- Gudiña, E.J., Teixeira, J.A., & Rodrigues, L.R. (2011). Biosurfactant-producing lactobacilli: Screening, production profiles, and effect of medium composition. Applied and Environmental Soil Science, 1, 1–9. doi:10.1155/2011/201254
- Hofvendahl, K., Niel, E.W.J., & Hahn-Hägerdal, B. (1999). Effect of temperature and pH on growth and product formation of Lactococcus lactis ssp. lactis ATCC 19435 growing on maltose. Applied Microbiology and Biotechnology, 51, 669–672. doi:10.1007/s002530051449
- Jhaa, S.S., Sanket, J., Joshia, S.J., & Geetha, S.J. (2016). Lipopeptide production by Bacillus subtilis R1 and its possible applications. Brazilian Journal of Microbiology, 47, 955–964. doi:10.1016/j.bjm.2016.07.006
- Li, C., Bai, J., Cai, Z., & Ouyang, F. (2002). Optimization of a cultural medium for bacteriocin production by Lactococcus lactis using a response surface methodology. Journal of Biotechnology, 93, 27–34. doi:10.1016/S0168-1656(01)00377-7
- Machado, T.R., Mohideen, R.A.H., Saravanakumari, M., & Prabhavathi, P. (2013). Anti adhesive, antimicrobial and biodegradability assay of a lipopeptide biosurfactant from Lactococcus lactis. International Journal of Science Innovations and Discoveries, 3, 478–483.
- Mayo, B., Aleksandrzak-Piekarczyk, T., Fernández, M., Kowalczyk, M., Álvarez- Martín, P., & Bardowski, J. (2010). Updates in the metabolism of lactic acid bacteria. In F. Mozzi, R.R. Raya, & G.M. Vignolo (Eds.), Biotechnology of lactic acid bacteria: Novel applications (pp. 3–33). Iowa, USA: Wiley-Blackwell.
- Moreno, I., Lerayer, A.S.L., Baldini, V.L.S., & Leitão, M.F.F. (2000). Characterization of bacteriocins produced by Lactococcus lactis strains. Brazilian Journal of Microbiology, 31, 184–192. doi:10.1590/S1517-83822000000300007
- Mulligan, C.N., Sharma, S.K., Mudhoo, A., & Makhijani, K. (2014). Green chemistry and biossurfactant research. In C.N. Mulligan, S.K. Sharma, & A. Mudhoo (Eds), Biosurfactants: Research trends and applications (pp. 1–30). Boca Raton: CRC Press.
- Oladunjoye, A.O., Singh, S., & Ijabadeniyi, O.A. (2016). Inactivation of Listeria monocytogenes ATCC 7644 on fresh-cut tomato using nisin in combinations with organic salts. Brazilian Journal of Microbiology, 47, 757–763. doi:10.1016/j.bjm.2016.04.027
- Panesar, P.S., Kennedy, J.F., Gandhi, D.N., & Bunko, K. (2007). Bioutilisation of whey for lactic acid production. Food Chemistry, 105, 1–14. doi:10.1016/j.foodchem.2007.03.035
- Parada, J.L., Caron, C.R., Medeiros, A.B.P., & Soccol, C.R. (2007). Bacteriocins from lactic acid bacteria: Purification, properties and use as biopreservatives. Brazilian Archives of Biology and Technology, 50, 521–542. doi:10.1590/S1516-89132007000300018
- Penna, T.C.V., Jozala, A.F., Gentille, T.R., Pessoa, J.A., & Cholewa, O. (2006). Detection of nisin expression by lactococcus lactis using two susceptible bacteria to associate the effects of nisin with EDTA. Applied Biochemistry and Biotechnology, 121, 334–346. doi:10.1007/978-1-59745-268-7_27
- Portilla-Rivera, A.O.M., Moldes, A.B., Torrado, A.M., & Domínguez, J.M. (2008). Stability and emulsifying capacity of biosurfactants obtained from lignocellulosic sources using Lactobacillus pentosus. Journal of Agricultural and Food Chemistry, 56, 8074–8080. doi:10.1021/jf801428x
- Rodrigues, L., Teixeira, J., Oliveira, R., & Van Der Mei, H.C. (2006a). Response surface optimization of the medium components for the production of biosurfactants by probiotic bacteria. Process Biochemistry, 41, 1–10. doi:10.1016/j.procbio.2005.01.030
- Rodrigues, L., Teixeira, J.A., & Oliveira, R. (2006b). Low-cost fermentative medium for biosurfactant production by probiotic bacteria. Biochemical Engineering Journal, 32, 135–142. doi:10.1016/j.bej.2006.09.012
- Rodrigues, L.R., Teixeira, J.A., Van De Mei, H.C., & Oliveira, R. (2006c). Physicochemical and functional characterization of a biosurfactant produced by Lactococcus lactis 53. Colloids and Surfaces B: Biointerfaces, 49, 78–85. doi:10.1016/j.colsurfb.2006.03.003
- Rodríguez, N., Salgado, J.M., Costés, S., & Domínguez, J.M. (2010). Alternatives for biosurfactants and bacteriocins extraction from Lactococcus lactis cultures producer under different pH conditions. Letters in Applied Microbiology, 51, 226–233. doi:10.1111/j.1472-765X.2010.02882.x
- Rodríguez-Pazo, N., Salgado, J.M., Cotéz-Diéguez, S., & Domínguez, J.M. (2013). Biotechnological production of phenyllactic acid and biosurfactants from trimming vine shoot hydrolyzates by microbial coculture fermentation. Applied Biochemistry and Biotechnology, 169, 2175–2188. doi:10.1007/s12010-013-0126-1
- Rosa, C.M., & Franco, B.D.G.M. (2002). Bacteriocinas de bactérias lácticas. Conscientiae Saúde, 1, 9–15. doi:10.5585/conssaude.v1i0.156
- Sabo, S.S., Converti, A., Todorov, S.D., Domínguez, J.M., & Oliveira, R.P.S. (2015). Effect of inulin on growth and bacteriocin production by Lactobacillus plantarum in stationary and shaken cultures. International Journal of Food Science & Technology, 50, 864–870. doi:10.1111/ijfs.12711
- Sadiq, S., Imran, M., Hassan, M.N., Iqbal, M., Zafar, Y., & Hafeez, F.Y. (2014). Potential of bacteriocinogenic Lactococcus lactis subsp. lactis inhabiting low pH vegetables to produce nisin variants. LWT- Food Sciences Technological, 59, 204–210. doi:10.1016/j.lwt.2014.05.018
- Sharma, D., Saharan, B.S., Chauhan, N., Bansal, A., & Procha, S. (2014). Production and structural characterization of Lactobacillus helveticus derived biosurfactant. The Scientific World Journal, 1, 1–9. doi:10.1155/2014/493548
- Souza, E.C., Penna, T.C.V., & Oliveira, R.P.S. (2014). Biosurfactant-enhanced hydrocarbon bioremediation: An overview. International Biodeterioration & Biodegradation, 89, 88–94. doi:10.1016/j.ibiod.2014.01.007
- Van Der Vegt, W., Van Der Mei, H.C., Noordmans, J., & Busscher, H.J. (1991). Assessment of bacterial biosurfactant production through axisymmetric drop shape analysis by profile. Applied Microbiology and Biotechnology, 35, 766–770. doi:10.1007/BF00169892
- Von Wright, A., & Axelsson, L. (2012). Lactic acid bacteria: An introduction. In S. Lahtinem, A.C. Ouwehand, S. Salminen, & A. Von Wright (Eds), Lactic acid bacteria: Microbiological and functional aspects (pp. 1–16). Boca Raton, FL, USA: CRC Press.
- Yoneyama, H., & Katsumata, R. (2006). Antibiotic resistance in bacteria and its future for novel antibiotic development. Bioscience, Biotechnology, and Biochemistry, 70, 1060–1075. doi:10.1271/bbb.70.1060