ABSTRACT
A simple, sensitive, and specific capillary electrophoretic method based on 1-phenyl-3-methyl-5-pyrazolon (PMP) derivatization has been developed for simultaneous separation and determination of 11 aldoses (maltose, xylose, arabinose, ribose, glucose, rhamnose, fucose, galactose, mannose, glucuronic acid, and galacturonic acid). The separation of PMP-labeled maltose and monosaccharides was carried out under the selected optimum conditions with pH 11.0, 200 mM borate buffer containing 4% methanol at voltage 20 kV, and capillary temperature 25°C, and the 11 PMP aldoses could be perfectly separated from each other within 41 min. Our experiment results demonstrated that the molar ratios of carbohydrates in 10 kinds of honey were greatly different, and the carbohydrates’ constitutions varied greatly between commodity honey and natural honey. This proposed zone electrophoresis method provides an accurate and economic alternative for monosaccharide and maltose analysis to identify honeys, and can also be applied to routine detection of reducing carbohydrates in real-life samples.
RESUMEN
El presente artículo da cuenta del desarrollo de un método de electroforesis capilar sencillo, sensible y específico, basado en la derivatización de 1-fenilo-3-metilo-5-pirazolona (PMP), para llevar a cabo, de manera simultánea, la separación y la determinación de 11 aldosas (maltosa, xilosa, arabinosa, ribosa, glucosa, ramnosa, fucosa, galactosa, manosa, ácido glucurónico, ácido galacturónico). Bajo condiciones seleccionadas óptimas, con pH 11,0, 200 mM de solución amortiguadora de borato, con metanol al 4%, voltaje de 20 kV y una temperatura capilar de 25°C, se efectuó la separación de la maltosa y los monosacáridos, etiquetados PMP. Las 11 aldosas PMP pudieron ser separadas perfectamente unas de otras en menos de 41 minutos. Los resultados de nuestros experimentos mostraron que las relaciones molares de carbohidratos presentes en 10 variedades de miel son muy diferentes, y que la composición de carbohidratos de la miel comercial varía significativamente de la encontrada en la miel natural. El método de electroforesis por zona propuesto constituye una alternativa precisa y económica para efectuar el análisis de monosacáridos y maltosa que posibilite identificar la variedad de miel. Además, puede ser utilizado para la detección rutinaria de carbohidratos reductores en muestras reales.
1. Introduction
Honey is the sweet substance produced in honeycomb by honeybees from nectars of blossoms or other active secretions of plants (Megherbi, Herbreteau, Faure, & Salvador, Citation2009). It is not only a remarkably complex natural nutritious tonic which contains at least 181 substances but also a type of supersaturated solution which contains small amounts of proteins, minerals, inorganic salts as well as a variety of enzymes which have the capability of nourishing, moistening, and detoxifying, such as catalase, invertase, and diastase (Alvarez-Suarez, Tulipani, Romandini, Bertoli, & Battino, Citation2010; Baroni et al., Citation2009; Daglia, Ferrari, Collina, & Curti, Citation2013; Noori & Al-Waili, Citation2004). However, carbohydrate is the main substance (95–99%) containing fructose, glucose, maltose, and other complex carbohydrates. Because of different floral source and external factors, the composition of carbohydrates in honey is different (Arráez-Román, Gómez-Caravaca, Gómez-Romero, Segura-Carretero, & Fernández-Gutiérrez, Citation2006; Blasa et al., Citation2006; LaGrange & Sanders, Citation1988; Shin & Ustunol, Citation2005).
Honey has been widely used in food and pharmaceutical with its high nutritional and medicinal values (Hamdy, Ismail, AL-Ahwal, & Gomaa, Citation2009; Mendes et al., Citation1998). Nevertheless, more and more honeys are intentionally adulterated with cheap sugar in order to increase its economic benefits (Megherbi et al., Citation2009; Paradkar & Irudayaraj, Citation2001). Even though many methods have been successfully applied to evaluate the quality of honey, such as stable carbon isotope ratio analysis (Cotte et al., Citation2007; Elflein & Raezke, Citation2008; Padovan, Jong, Rodrigues, & Marchini, Citation2003), high-performance liquid chromatography (HPLC) (Cavazza, Corradini, Musci, & Salvadeo, Citation2013; Ouchemoukh, Schweitzer, Bey, Djoudad-Kadji, & Louailech, Citation2010), gas chromatography coupled to a flame-ionization detector (Cotte, Casabianca, Chardon, Lheritier, & Grenier-Loustalot, Citation2003; Low & South, Citation1995), and mass spectrometry (Horvath & Molnar-Perl, Citation1997; Sanz, Gonzalez, De, Lorenzo, Sanz, & Martinez-Castro, Citation2004), establishing a series of accurate and rapid methods to detect the adulterated honey is extremely urgent (Megherbi et al., Citation2009). Recently, capillary zone electrophoresis (CZE) has become a rapid and high-resolution method including separation, characterization, and quantitative chromatographic separation to analyze trace amounts of samples, and thus, the CZE method has gained widespread interest as a favorable technique for the determination of pharmacologically interesting compounds in biological matrices, including honey (Martos et al., Citation2000; Morales, Citation2002). Several papers have applied capillary electrophoresis previously for the identification of organic acids and phenolic compounds in honey (Mato, Huidobro, Simal-Lozano, & Sancho, Citation2006; Navarrete et al., Citation2005), but seldom research is involved in the analysis for monosaccharide composition. In this paper, we first demonstrated the application of CZE with 1-phenyl-3-methyl-5-pyrazolone (PMP)-tagged UV detection method to detect compositional carbohydrates in honey.
The purpose of the present study was to develop a rapid and simple CZE analytical method for the simultaneous, efficient separation and determination of 11 reducing carbohydrates possibly found in honey. Considering the complex carbohydrate composition of honey, the developed CZE method was effectively applied to quantitative analysis of the carbohydrate composition in the honey samples. In addition, this method was successfully used in quantitative analysis of the monosaccharides which were extracted and degraded of polysaccharides in honey sample. Furthermore, the developed CZE method proves to be precise and practical for quality control of reducing carbohydrates in honey.
2. Materials and methods
2.1. Materials and chemicals
The proposed assay was applied to 10 honey samples, including multifloral honey, Acacia sp. honey, and so on. Among them, four honey samples, such as multifloral honey (Multifloral), Acacia sp. honey (Acacia sp.), Ziziphus jujuba flower honey (Ziziphus jujuba), and Fagopyrum esculentum honey (Fagopyrum esculentum) were from the apiary, which were not processed. Rongshiwang Mountain Chrysanthemum morifolium honey (Chrysanthemum morifolium), Rongshiwang Mountain Wuxing Eriobotrya japonica honey (Eriobotrya japonica), Rongshiwang Mountain Salum Sophora japonica honey (Salum Sophora japonica) were collected from Shaanxi Old Beekeepers Biological Technology Co., China. Astragalus spp.honey (Astragalus spp.) was from Shanghai Guanshengyuan Bee Products Co., China. Bai Hua Acacia sp. honey (Bai Hua Acacia sp.) was from Beijing Baihua Honey Co., China, and the Organic Sophora japonica honey (Organic Sophora japonica) came from Chengdu Fengs Apiculture Co., China. The samples were stored in darkness at room temperature until analysis. D-Mannose, D-ribose, D-glucose, L-rhamnose, D-glucuronic acid, D-galacturonic acid, D-xylose, D-galactose, L-arabinose, maltose, and D-fucose were all obtained from Sigma (St. Louis, U.S.A.). 1-Phenyl-3-methyl-5-pyrazolone (PMP) and trifluoroacetic acid (TFA) were the products of Beijing Reagent Plant (Beijing, China). HPLC-grade methanol was purchased from Honeywell (U.S.A.). Doubly deionized water was obtained with a Milli-Q water purification system (Millipore, Bedford, MA), and all of the other chemicals were of analytical grade.
2.2. Extraction of the honey polysaccharides
The honey polysaccharides were isolated by hot-water extraction and ethanol precipitation with slight modification. Briefly, 50 g honey was extracted with 400 mL distilled water at 80°C for twice, 2 h each time. The mixture was concentrated to 150 mL under a diminished pressure and centrifuged at 3000g for 15 min. After the supernatant was collected, the residue was redissolved by adding 500 mL of 95% ethanol slowly by stirring and kept at 4°C overnight. Finally, the polysaccharide pellets were obtained by centrifugation at 3000g for 10 min and repeatedly washed sequentially with ethanol, acetone, and ether, respectively. The refined polysaccharide pellets were completely dissolved in appropriate volume of distilled water and intensively dialyzed for 2 days (water change regularly) against distilled water (cutoff Mw 8000 Da), and then the remaining portion was concentrated, deproteinated by a freeze–thaw process (FD-1, Henan Yuhua Instrument Co., China) repeated 10 times followed by filtration. Finally, the filtrate was lyophilized to obtain the crude water-soluble honey polysaccharides.
2.3. Hydrolysis of the honey polysaccharides
The honey polysaccharides were hydrolyzed extensively by TFA as our previously described by Lv et al. (Citation2009). In brief, a suitable amount of honey polysaccharide (Multifloral/21.4 mg, Acacia sp./20.4 mg, Ziziphus jujuba/18.6 mg, Fagopyrum esculentum/22.9 mg, Chrysanthemum morifolium/19.7 mg, Eriobotrya japonica/16.1 mg, Salum Sophora japonica/19.3 mg, Astragalus spp./17.6 mg, Bai Hua Acacia sp./20.1 mg, Organic Sophora japonica/17.7 mg) were hydrolyzed with 2 mL of 2 M TFA in ampoules (10 mL), respectively. The ampoules were sealed under a nitrogen atmosphere. After hydrolysis at 110°C for 8 h with an oil bath, the mixture was cooled to room temperature and centrifuged at 1000g for 5 min. The supernatant was collected and dried with nitrogen gas to remove the excess TFA and redissolved in 1.0 mL distilled water, which was ready for derivatization.
2.4. Derivatization procedure
The PMP derivatization of monosaccharides and maltose was carried out as described previously with proper modification (Honda et al., Citation1989; Honda, Suzuki, & Taga, Citation2003). Briefly, 10 kinds of honey samples (0.3 g for each) were hydrolyzed with 10 mL distilled water in ampoules. 20 μL hydrolyzed solution of monosaccharides (aqueous) or the diluted samples of honey was dissolved in 400 μL of 0.3 M aqueous NaOH and 400 μL of 0.5 M PMP–methanol solution. After shaking for 10 s, the solution was allowed to stand for 30 min at 70°C in a water bath, and then cooled to room temperature and neutralized with 400 μL 0.3 M HCl. The resulting solution was extracted with 1.0 mL chloroform, and after vigorous shaking and centrifuging, the organic phase was carefully discarded, and the extraction process was repeated for three times. Finally, the aqueous layer was filtered through a 0.22-μm membrane for CZE analysis. The reagent solution was freshly prepared before derivatization, and the samples were dried by nitrogen and diluted with 0.5 ml of distilled water.
2.5. Preparation of buffer and standard solution
As Hu, Wang, Yang, and Zhao (Citation2014) reported that the concentration of 200 mM of working buffer solution was obtained by adding 500 mL of deionized water to the 250 mL of stocked buffer solution (400 mM), and neutralized to pH 11.0 with saturated sodium hydroxide. The stock standard monosaccharide solutions (10 mM) were prepared by dissolving each standard monosaccharide in ultrapure water. The sample solutions were filtered through a 0.22-μm syringe filter and degassed using an ultrasonic bath for 2 min prior to use. All the solutions prepared were stored in the dark at 4°C until being used.
2.6. Apparatus and electrophoretic procedures
The PMP-labeled monosaccharides and maltose were analyzed by a Beckman P/ACE MDQ capillary electrophoresis instrument equipped with a UV detector, an automatic injector, and an uncoated fused silica capillary (Beckman-Coulter, Fullerton, CA, U.S.A.). Data acquisition and processing were carried out with Beckman System Gold software. Fused silica capillaries had an internal diameter of 50 μm and were 58.5 cm in total length (effective length 48.5 cm). At the start of each working day, the capillary was preconditioned by flushing with the following reagents for 5 min: water, 0.1 M NaOH, water, 0.1 M HCl, water, and then running buffer. Between injections, the capillary was rinsed sequentially with water, 0.1 M NaOH and water, both for 5 min, and then equilibrated with borate buffer at a pressure of 20 psi for 3 min. After all analysis of the day, the capillary was rinsed with water for 5 min and stored overnight with water inside. Samples were introduced by pressure injection at 0.5 psi for 5 s into the capillary. The UV absorbance was measured at 245 nm.
3. Results and discussion
3.1. Establishment of standard analysis method
For quantification of reducing saccharides by selective, sensitive, and robust CZE methods, pre-column derivatization is among the most popular approaches. In the present case, reductive 1-phenyl-3-methyl-5-pyrazolone (PMP) as the strong UV-active tag was chosen and an excess of PMP label was used to guarantee optimal conversion into the labeled saccharide derivatives, which could work in a simplified aqueous methanol system, and could afford stable PMP derivative as previously described. Separation of the PMP-labeled derivatives of standard aldoses was performed by CZE, and detection was carried out at 245 nm and identified by spiking samples with standards. To guarantee the ionization of the analytes, we made a detailed study on some important parameters, including the pH of running borate buffer, the borate concentrations, and the proportions of methanol. The best resolution versus the lowest migration time was obtained at the optimized conditions of pH 11.0, 175 mM borate buffer containing 4% methanol at 25°C and 15 kV, at which value the PMP-carbohydrate derivatives were negatively charged. An optimum chromatographic profile of the standard PMP-labeled carbohydrates is shown in . The result indicated that 11 kinds of reducing sugars with PMP label, including maltose and 10 monosaccharides (xylose, arabinose, ribose, glucose, rhamnose, fucose, galactose, mannose, glucuronic acid, and galacturonic acid as internal standard), were simultaneously separated under the optimized conditions.
Figure 1. Typical electropherograms of standard PMP-labeled reducing carbohydrates (maltose and 10 monosaccharides). Analytical conditions: fused capillary 58.5 cm (48.5 cm to the detector) ×50 µm, i.e. 200 mM borate buffer, pH = 11.0, applied voltage 15 kV, capillary temperature 25°C, UV detection at 245 nm; Peaks: 1. maltose, 2. xylose, 3. arabinose, 4. glucose, 5. ribose, 6. rhamnose, 7. fucose, 8. galactose, 9. mannose, 10. glucuronic acid, 11. galacturonic acid (internal standard).
Figura 1. Electroferograma típico de carbohidratos reductores (maltosa y 10 monosacáridos) estándar, etiquetados PMP. Condiciones analíticas: Amortiguador de borato de capilar fundido 58,5 cm (48,5 cm para el detector) ×50 µm i.d., 200 mM, pH = 11,0, voltaje aplicado 15 kV, temperatura capilar 25 °C, detección de UV a 245 nm; Picos: 1. maltosa, 2. xilosa, 3. arabinosa, 4. glucosa, 5. ribosa, 6. ramnosa, 7. fucosa, 8. galactosa, 9. manosa, 10. Ácido glucurónico, 11. Ácido galacturónico (estándar interno).
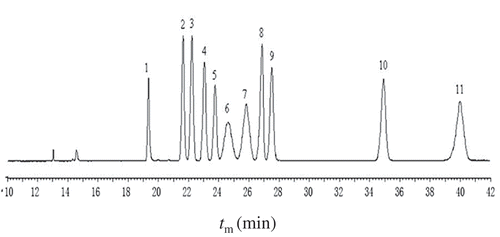
In this study, we have tried to analyze the reducing carbohydrate composition of honey samples and honey polysaccharides using the established CZE method. For the 10 kinds of diluted honeys, they were directly derivatized with PMP and subsequently analyzed by the developed CZE method. For the 10 kinds of isolated honey polysaccharides, they were hydrolyzed with TFA, and then the acid hydrolysates were neutralized and derivatized with PMP. Finally, the released monosaccharide as PMP derivatives was analyzed by the described CZE method. As shown in , all of the peaks were identified within 41 min by comparing their tm values with those of the standards spiked in the samples. Nevertheless, the results showed that all the samples did not contain galacturonic acid (GalUA), and thus, GalUA is used as internal standard for its good separation from other standards. As can be seen, the sequence of peaks is maltose, xylose, arabinose, glucose, ribose, rhamnose, fucose, galactose, mannose, glucuronic acid, and galacturonic acid. Therefore, the final optimized experimental conditions in this work were 200 mM borate buffer at pH 11.0, running voltage 15 kV, and capillary temperature 25°C.
3.2. Rationality and linearity
Method precision expressed as coefficient of variation (CV, %) was obtained by measuring the repeatability for both intraday variability and inter-day variability of retention time (tR) and corrected peak areas (A/t), which was estimated by making six repetitive injections of a standard mixture solution (10 µg/mL for each analyte) under the same CZE condition. The results showed that the CV values in intraday were less than 1.8% for tR and 2.7% for A/t, and the inter-day CV values were less than 5% for all variables. The validated results indicated that this method was rapid, accurate, and reliable.
Under the optimized conditions, five series of concentrations in the range of 1.2–140.8 μM of the standard sugars (maltose, xylose, arabinose, glucose, ribose, rhamnose, fucose, galactose, mannose, glucuronic acid, and galacturonic acid) were tested to determine the linearity by CZE, each point of the calibration plot was repeated three times in an independent solution, and the regression equation and correlation coefficient were listed in . As a consequence, a good linearity with the correlation coefficients (r) in the range of 0.9908–0.9967 was obtained between Y (peak area ratio of the analytes with internal standard) and X (concentration of the standards).
Table 1. Experimental results of linearity relationship and precision of analysis of reducing monosaccharides and disaccharide (maltose).
Tabla 1. Resultados experimentales de la relación de linealidad y precisión del análisis de monosacáridos y disacáridos (maltosa) reductores.
3.3. Aldoses analysis of honeys and its isolated polysaccharides
In this study, the developed CZE analytical method was further applied to the quantification of reducing carbohydrate composition of natural honey and the component monosaccharides in the honey polysaccharides. The analytical results were shown in and , respectively. As depicted in , reducing glucose and maltose were dominant components in all tested honeys, and all the 10 different kinds of honeys contained maltose, xylose, glucose, and galactose with different mole ratios (2.8:1.2:8.8:1 for Multifloral, 0.9:1.4:5.2:1 for Acacia sp., 2.6:1.7:5.6:1 for Ziziphus jujuba, 3.7:2.3:11.9:1 for Fagopyrum esculentum, 1.9:1.8:9.4:1 for Chrysanthemum morifolium, 2.7:1.0:9.4:1 for Eriobotrya japonica, 4.1:2.1:7.6:1 for Salum Sophora japonica, 4.5:0.6:8.2:1 for Astragalus spp., 2.7:0.5:13.9:1 for Bai Hua Acacia sp., 1.9:0.4:9.7:1 for Organic Sophora japonica). Besides, multifloral honey and Ziziphus jujuba flower honey contained glucuronic acid, and only Astragalus spp. honey did not contain arabinose. In addition, only multifloral honey contained free mannose and only Acacia sp. honey contained free fucose.
Table 2. Determination of free reducing monosaccharides and maltose in the samples of the tested honeys.
Tabla 2. Determinación de monosacáridos reductores libres y de maltosa en muestras de mieles sometidas a prueba.
Table 3. Determination of the component monosaccharides of the isolated polysaccharides derived from 10 kinds of honeys.
Tabla 3. Determinación de los monosacáridos componentes de los polisacáridos aislados derivados de 10 variedades de miel.
As can be seen in , the compositional monosaccharides released from all the purified 10 kinds of honey polysaccharides consisted of xylose and glucose, and their corresponding mole ratios in different samples were 1:8.1; 1:2.7; 1:0.7; 1:1.1; 1:1.4; 1:2.4; 1:1.2; 1:4.2; 1:3.9; 1:3.9, respectively. It was clear that all the honey polysaccharides did not contain arabinose except for Ziziphus jujuba flower honey and Fagopyrum esculentum honey, while only two kinds of polysaccharides from honeys covered rhamnose. Glucose was the main part of the monosaccharide composition in honey polysaccharides.
The results of CZE assay indicated that all the tested natural honeys contained xylose, glucose, and galactose, while all the commercial honey did not contain rhamnose, mannose, fucose, and galactose, and the main free aldose in honeys was glucose and maltose, suggesting that the proportion of compositional reducing disaccharide and monosaccharides altered with different nectars. It was found that all the honey polysaccharides consisted of xylose and glucose. Except for Rongshiwang Mountain Wuxing Eriobotrya japonica honey, all the commercial honey polysaccharides did not contain galactose. Multifloral honey and Ziziphus jujuba flower honey and their polysaccharides contained rhamnose and glucuronic acid. Besides, Acacia sp. honey and its polysaccharide were composed of fucose, whereas commercial Acacia sp. honey and their polysaccharides did not contain it.
3.4. Recovery experiments
In order to verify the accuracy and stability of the detection method, the recovery experiments were carried out for the main compositional aldoses. The standard solutions of glucose and maltose were accurately added into sample diluents, and the analysis was performed with the method described in 3.1. Ultimately, the analytical data in recovery experiments were given in . In this study, the average recoveries were calculated based on the difference between the amount determined in the spiked samples and the amount observed in the non-spiked samples. All the analysis was carried out in triplicate. The results showed that the recoveries of glucose in all the honey samples ranged from 96.2% to 103.8%, and the relative standard deviation (RSD) values were not more than 3.9%. The recovery of maltose was between 94.5% and 102.5%, and the RSD values were not more than 4.1%. These results showed that the developed method was accurate and practical for the component analysis of monosaccharides and oligosaccharides in honey samples.
Table 4. Results of the recovery test of glucose and maltose (n = 3).
Tabla 4. Resultados de la prueba de recuperación de glucosa y maltosa (n = 3).
It is widely recognized that the separation and identification of a large number of carbohydrates are a complex and challenging area for research. One of the major problems is the risk of coelution of the different sugars. Another reason is that honey as natural sweeteners mainly contains carbohydrates, in which fructose is the most followed by glucose and other complicated sugars (Cavia et al., Citation2002; Kaškonienė, Venskutonis, & Čeksterytė, Citation2010). However, fructose as ketose can not be derivatized for the absence of aldehyde group. Although there is some published information in this area, (Honda et al., Citation2003; Sjöberg, Adorjan, Rosenau, & Kosma, Citation2004), no previous studies have attempted to quantify all of these carbohydrates. Therefore, an efficient method has been developed to control the quality of honey by determining the components of reducing sugars, and the different ratio of each component was established in this study. To our knowledge, this is the first report showing a new CZE method for separating 11 kinds of reducing monosaccharides and oligosaccharides from honeys within 41 min, which provides a rapid and effective method to control quality of honeys and detect the adulteration of honeys.
4. Conclusions
An efficient simultaneous CZE separation of 10 monosaccharides and disaccharide (maltose) as common reducing carbohydrate components found in honeys was detailedly examined and optimized. The newly established CZE method has been successfully applied to the qualitative and quantitative determination of reducing monosaccharides and disaccharide components in four natural and six commercial honeys with high separation efficiency, precision, and simple analysis process. This study has also allowed the conclusive identification of 10 monosaccharides released from isolated polysaccharides in the 10 kinds of honeys. The different proportion of reducing carbohydrate components between natural and commercial honeys were found by the CZE analysis. This proposed CZE method provides an accurate and economic alternative for monosaccharide and oligosaccharides analysis to identify honeys, and can also be applied to routine detection of monosaccharide and oligosaccharides in real-life samples.
Acknowledgments
The authors acknowledge financial supports from the National Natural Science Foundation of China (C31171678), and the Development program for Innovative Research Team of Shaanxi Normal University, China (GK201501006), and Science and Technology Innovation as a Whole Plan Projects of Shaanxi Province, China (2015KTCQ02-01). as well as the Construction Program of Chongqing Engineering Research Center (167001).
Disclosure statement
No potential conflict of interest was reported by the authors.
Additional information
Funding
References
- Alvarez-Suarez, J.M., Tulipani, S., Romandini, S., Bertoli, E., & Battino, M. (2010). Contribution of honey in nutrition and human health: A review. Mediterranean. Nutrition & Metabolism, 3, 15–23. doi:10.1007/s12349-009-0051-6
- Arráez-Román, D., Gómez-Caravaca, A.M., Gómez-Romero, M., Segura-Carretero, A., & Fernández-Gutiérrez, A. (2006). Identification of phenolic compounds in rosemary honey using solid-phase extraction by capillary electrophoresis-electrospray ionization-mass spectrometry. Journal of Pharmaceutical and Biomedical Analysis, 41, 1648–1656. doi:10.1016/j.jpba.2006.02.035
- Baroni, M.V., Arrua, C., Nores, M.L., Fayé, P., Díaz, M.P., Chiabrando, G.A., & Wunderlin, D.A. (2009). Composition of honey from Córdoba (Argentina): Assessment of North/South provenance by chemometrics. Food Chemistry, 114, 727–733. doi:10.1016/j.foodchem.2008.10.018
- Blasa, M., Candiracci, M., Accorsi, A., Piacentini, M.P., Albertini, M.C., & Piatti, E. (2006). Raw Millefiori honey is packed full of antioxidants. Food Chemistry, 97, 2217–2222. doi:10.1016/j.foodchem.2005.03.039
- Cavazza, A., Corradini, C., Musci, M., & Salvadeo, P. (2013). High-performance liquid chromatographic phenolic compound fingerprint for authenticity assessment of honey. Journal of the Science of Food and Agriculture, 93, 1169–1175. doi:10.1002/jsfa.5869
- Cavia, M.M., Fernandez-Muino, M.M., Gomez-Alonso, E., Montes-Pérez, M.J., Huidobro, J.F., & Sancho, M.T. (2002). Evolution of fructose and glucose in honey over one year: Influence of induced granulation. Food Chemistry, 78, 157–161. doi:10.1016/S0308-8146(01)00393-4
- Cotte, J.F., Casabianca, H., Chardon, S., Lheritier, J., & Grenier-Loustalot, M.F. (2003). Application of carbohydrate analysis to verify honey authenticity. Journal of Chromatography A, 1021, 145–155. doi:10.1016/j.chroma.2003.09.005
- Cotte, J.F., Casabianca, H., Lheritier, J., Perrucchietti, C., Sanglar, C., Waton, H., & Grenier-Loustalot, M.F. (2007). Study and validity of 13C stable carbon isotopic ratio analysis by mass spectrometry and 2H site-specific natural isotopic fractionation by nuclear magnetic resonance isotopic measurements to characterize and control the authenticity of honey. Analytica Chimica Acta, 582, 125–136. doi:10.1016/j.aca.2006.08.039
- Daglia, M., Ferrari, D., Collina, S., & Curti, S. (2013). Influence of in vitro simulated gastroduodenal digestion on methylglyoxal concentration of manuka (Lectospermum scoparium) honey. Journal of Agricultural and Food Chemistry, 61, 2140–2145. doi:10.1021/jf304299d
- Elflein, L., & Raezke, K.P. (2008). Improved detection of honey adulteration by measuring differences between 13C/12C stable carbon isotope ratios of protein and sugar compounds with a combination of elemental analyser-isotope ratio mass spectrometry and liquid chromatography-isotope ratio mass spectrometry. Apidologie, 39, 574–587. doi:10.1051/apido:2008042
- Hamdy, A.A., Ismail, H.M., AL-Ahwal, A.-M., & Gomaa, N.F. (2009). Determination of flavonoid and phenolic acid contents of clover, cotton and citrus floral honeys. The Journal of the Egyptian Public Health Association, 84, 245–259.
- Honda, S., Akao, E., Suzuki, S., Ckuda, M., Kakehi, K., & Wakamura, J. (1989). High-performance liquid chromatography of reducing carbohydrates as strongly ultraviolet-absorbing and electrochemically sensitive 1-phenyl-3-methyl-5-phrazolone derivatives. Analytical Biochemistry, 180, 351–357. doi:10.1016/0003-2697(89)90444-2
- Honda, S., Suzuki, S., & Taga, A. (2003). Analysis of carbohydrates as 1-phenyl-3-methyl-5-pyrazolone derivatives by capillary/microchip electrophoresis and capillary electrochromatography. Journal of Pharmaceutical and Biomedical Analysis, 30, 1689–1714. doi:10.1016/S0731-7085(02)00512-5
- Horvath, K., & Molnar-Perl, I. (1997). Simultaneous quantitation of mono-, di- and trisaccharides by GC-MS of their TMS ether oxime derivates: II. In honey. Chromatographia, 45, 328–335. doi:10.1007/BF02505579
- Hu, Y.Y., Wang, T., Yang, X.B., & Zhao, Y. (2014). Analysis of compositional monosaccharides in fungus polysaccharidesby capillary zone electrophoresis. Carbohydrate Polymers, 102, 481–488. doi:10.1016/j.carbpol.2013.11.054
- Kaškonienė, V., Venskutonis, P.R., & Čeksterytė, V. (2010). Carbohydrate composition and electrical conductivity of different origin honeys from Lithuania. LWT-Food Science and Technology, 43, 801–807. doi:10.1016/j.lwt.2010.01.007
- LaGrange, V., & Sanders, S.W. (1988). Honey in cereal-based new food products. Cereal Foods World, 33, 833–838.
- Low, N.H., & South, W. (1995). Determination of honey authenticity by capillary gas chromatography. Journal of Aoac International, 78, 1210–1218.
- Lv, Y., Yang, X.B., Zhan, Y., Ruan, Y., Yang, Y., & Wang, Z.Z. (2009). Separation and quantification of component monosaccharides of the tea polysaccharides from Gynostemma pentaphyllum by HPLC with indirect UV detection. Food Chemistry, 112, 742–746. doi:10.1016/j.foodchem.2008.06.042
- Martos, I., Ferreres, F., Yao, L., D’Arcy, B., Caffin, N., & Tomas-Barberan, F.A. (2000). Flavonoids in monospecific eucalyptus honeys from Australia. Journal of Agricultural and Food Chemistry, 48, 4744–4748. doi:10.1021/jf000277i
- Mato, I., Huidobro, J.F., Simal-Lozano, J., & Sancho, M.T. (2006). Rapid determination of nonaromatic organic acids in honey by capillary zone electrophoresis with direct ultraviolet detection. Journal of Agricultural and Food Chemistry, 54, 1541–1550. doi:10.1021/jf051757i
- Megherbi, M., Herbreteau, B., Faure, R., & Salvador, A. (2009). Polysaccharides as a marker for detection of corn sugar syrup addition in honey. Journal of Agricultural and Food Chemistry, 57, 2105–2111. doi:10.1021/jf803384q
- Mendes, E., Brojo, Proenc, E., Ferreira, I., Mplvo, I., & Ferreira, M.A. (1998). Quality evaluation of Portuguese honey. Carbohydrate Polymers, 37, 219–223. doi:10.1016/S0144-8617(98)00063-0
- Morales, F.J. (2002). Application of capillary zone electrophoresis to the study of food and food-model melanoidins. Food Chemistry, 76, 363–369. doi:10.1016/S0308-8146(01)00295-3
- Navarrete, M., Casado, S., Minelli, M., Segura, A., Bonetti, A., Dinelli, G., & Fernández, A. (2005). Direct determination of aliphatic acids in honey by coelectroosmotic capillary zone electrophoresis. Journal of Apicultural Research, 44, 65–70. doi:10.1080/00218839.2005.11101151
- Noori, S., & Al-Waili. (2004). Natural honey lowers plasma glucose, C-Reactive protein. Homocysteine, and blood lipids in healthy. Diabetic, and hyperlipidemic subjects: Comparison with dextrose and sucrose. Journal of Medicinal Food, 7, 100–107. doi:10.1089/109662004322984789
- Ouchemoukh, S., Schweitzer, P., Bey, M.B., Djoudad-Kadji, H., & Louailech, H. (2010). HPLC sugar profiles of Algerian honeys. Food Chemistry, 121, 561–568. doi:10.1016/j.foodchem.2009.12.047
- Padovan, G.J., Jong, D.D., Rodrigues, L.P., & Marchini, J.S. (2003). Detection of adulteration of commercial honey samples by the 13C/12C isotopic ratio. Food Chemistry, 82, 633–636. doi:10.1016/S0308-8146(02)00504-6
- Paradkar, M.M., & Irudayaraj, J. (2001). Discrimination and classification of beet and cane inverts in honey by FT-Raman spectroscopy. Food Chemistry, 76, 231–239. doi:10.1016/S0308-8146(01)00292-8
- Sanz, M.L., Gonzalez, M., De, Lorenzo, C., Sanz, J., & Martinez-Castro, L. (2004). Carbohydrate composition and physicochemical properties of artisanal honeys from Madrid (Spain): Occurrence of Echium sp honey. Journal of the Science of Food and Agriculture, 84, 1577–1584. doi:10.1002/jsta.1823
- Shin, H.S., & Ustunol, Z. (2005). Carbohydrate composition of honey from different floral sources and their influence on growth of selected intestinal bacteria: An in vitro comparison. Food Research International, 38, 721–728. doi:10.1016/j.foodres.2005.01.007
- Sjöberg, J., Adorjan, I., Rosenau, T., & Kosma, P. (2004). An optimized CZE method for analysis of mono- and oligomeric aldose mixtures. Carbohydrate Research, 339, 2037–2043. doi:10.1016/j.carres.2004.06.00