ABSTRACT
Super-atmospheric O2 has been shown to affect respiration rate (RR), but no model describing its effect on RR for pomegranate arils has been reported. This study investigated the effects of four different gas compositions (5 kPa O2, 10 kPa CO2 and 85 kPa N2; 10 kPa O2, 5 kPa CO2 and 85 kPa N2; 70 kPa O2, 10 kPa CO2 and 20 kPa N2; and air) on RR of pomegranate arils (cv. Wonderful) stored at 5°C. Michaelis–Menten enzyme kinetics models were used to investigate the effect of CO2 inhibition on O2 consumption rate. Respiratory quotient was used to determine fermentation threshold. The O2 consumption rate increased from 0.87 to 2.81 mL/kg h, with increase in O2 concentration from 5 kPa to 70 kPa. All enzyme kinetics model parameters adequately described the influence of gas concentration on aril RR with correlation coefficient (R2adj = 81–91%).
RESUMEN
Se ha demostrado que el O2 súper-atmosférico incide en la frecuencia respiratoria (FR); sin embargo, no existe ningún modelo que describa su efecto en la FR de los arilos de granada. El presente estudio analizó los efectos que cuatro composiciones diferentes de gas (5 kPa O2, 10 kPa CO2 y 85 kPa N2; 10 kPa O2, 5 kPa CO2 y 85 kPa N2; 70 kPa O2, 10 kPa CO2 y 20 kPa N2; y aire) tuvieron en la FR de arilos de granada (cv. Wonderful) almacenados a 5°C. Así, para investigar el efecto de inhibición que el CO2 produce en la tasa de consumo de O2 se utilizaron modelos de cinética enzimática de Michaelis–Menten (MM), mientras que para determinar el límite de fermentación se empleó el cociente respiratorio (CR). Se constató que el aumento de la concentración de O2 de 5 kPa a 70 kPa produjo un incremento de la tasa de consumo de O2, que se elevó de 0.87 a 2.81 mL/kg h. Todos los parámetros del modelado de cinética enzimática describen adecuadamente la influencia de las concentraciones de gas en la FR de arilos de granada, con el coeficiente de correlación (R2adj = 81–91%).
Introduction
After harvest, fresh produce are susceptible to increased physiological stress and deterioration in quality due to accelerated respiration rate (RR) (Aindongo, Caleb, Mahajan, Manley, & Opara, Citation2014; Caleb, Mahajan, Opara, & Witthuhn, Citation2012a). This process consumes O2 and produces CO2 in a series of enzymatic reaction (Iqbal, Rodrigues, Mahajan, & Kerry, Citation2009). Thus, higher RR could indicate a faster rate of physiological aging, senescence and deterioration of fresh produce (Cliffe-Byenes & O`Beirne, Citation2007). Improved postharvest handling methods which apply modified atmosphere (MA) around the produce can be used to reduce RR and slow down metabolic processes, thereby maintaining quality and extending shelf life (Belay, Caleb, & Opara, Citation2016; Caleb et al., Citation2012a; Jing-Jun et al., Citation2012).
Studies have reported different approaches to determine suitable gas concentration for reducing the RR of pomegranate arils. Ersan, Gunes, and Zor (Citation2010) investigated the effect of O2 (2, 10 and 21 kPa) and CO2 (0, 10 and 20 kPa) concentration on the RR of pomegranate arils (cv. Hicaznar) at 5°C. Similarly, Banda, Caleb, Jacobs, and Opara (Citation2015) studied the effect of O2 (5, 21 and 30 kPa) and CO2 (0, 10 and 40 kPa) on the RR of ‘Wonderful’ pomegranate arils. These studies showed that low O2 atmosphere significantly reduced the RR. In addition, 2–4 kPa O2 was recommended for maintaining qualities of ‘Mollar de Elche’ pomegranate arils by López-Rubira, Conesa, Allende, and Artés (Citation2005). Overall, the results reported in these studies demonstrated that the application of MA systems was effective in maintaining quality and extended shelf life of pomegranate arils. However, low O2 concentration can cause the build-up of anaerobic atmospheres which then leads to fermentation. Recent studies have introduced the benefits of super-atmospheric O2 (>70 kPa) to overcome the limitation of low O2 atmosphere on postharvest physiology and quality maintenance of fresh produce (Belay, Caleb, & Opara, Citation2017; Maghoumi et al., Citation2013; Molinu et al., Citation2016).
In addition, predicting respiratory kinetics of different fruits and vegetables has been based on consideration of the RR as a function of gas composition using enzyme kinetics model (Belay et al., Citation2016; Iqbal et al., Citation2009; Torrieri, Perone, Cavella, & Masi, Citation2010). For pomegranate fruit, Caleb, Opara, and Witthuhn (Citation2012b) investigated the effect storage temperature (5, 10 and 15°C) and time on RR of pomegranate arils (cvs. Acco and Herskawitz) by using Arrhenius-type model. Similarly, Aindongo et al. (Citation2014) studied the effect of temperature (5, 10, 15 and 22°C) on RR of different pomegranate fractions cv. ‘Bhagwa’ by using Arrhenius equation. These studies reported that temperature had significant effect on RR of pomegranate aril and Arrhenius-type model adequately predicted this effect regarding different temperatures and cultivars used.
On the other hand, the effects of gas composition (2, 10, 21 kPa O2 and 0, 10, 20 kPa CO2) on the RR of pomegranate arils (cv. Hicaz) was studied by Ersan et al. (Citation2010) using Michaelis–Menten (MM) model. The study showed that MAs affected the RR of pomegranate arils and this relationship was best expressed using the MM competitive inhibition model. However, their study did not report on the application of super-atmospheric O2 condition and its effects on the physiological response of pomegranate arils cv. ‘Wonderful’. In addition, pomegranate fruit undergo a long-term shipping from southern hemisphere (producers) to northern hemisphere (market) and vice versa, prior to processing into arils (Belay et al., Citation2017). There is limited report on the impacts of this long supply chain on the physiology of the fruit. Therefore, the aim of this study was to investigate the effects of low and super-atmospheric O2 combined with low and high CO2 concentrations on the RR of pomegranate arils (cv. Wonderful) after long-term storage. The goal of this study is to provide valuable information on the respiratory kinetics of pomegranate arils cv. ‘Wonderful’, which would guide the role players along the value chain to better design and optimize the postharvest handling and packaging of arils.
Materials and methods
Plant materials and sample preparation
Pomegranate fruits (cv. Wonderful) were obtained at commercially ripened stage with characteristic deep-red skin and aril with mature kernel (Mphahlele, Caleb, Fawole, & Opara, Citation2016), from Sonlia Packhouse, Wellington, Western Cape (33° 38′ 23″ S, 19° 00′ 40″ E), South Africa. Fruits were transported in an air-conditioned and ventilated vehicle to the Postharvest Research Laboratory at Stellenbosch University and stored in a cold room (about 5°C and 95% relative humidity (RH)) for 4 months until the experiment started. The storage was done to simulate pre-shipping storage plus long-term shipping duration from southern hemisphere production region to the northern hemisphere market and vice versa. Damaged fruits were removed and the outer skin of selected healthy fruits was surface disinfected using 70% ethanol solution (Aindongo et al., Citation2014). Arils were extracted manually by carefully removing the husk. Extracted arils were collected in a tray and mixed together to create a homogenous batch of all the fruits. Arils (350 g) were transferred to 3000 mL airtight glass jars, which were designed to achieve a completely hermetic seal. The glass jars were prepared in triplicate for each gas mixture and stored at 5°C. Measurement was performed using four gas mixtures as shown in .
Table 1. Experimental gas combinations investigated.
Tabla 1. Combinaciones experimentales de gas investigadas.
Experimental set-up
Rate of O2 consumption () and CO2 evolution (
) of the arils were measured using a closed system as described by Iqbal et al. (Citation2009), Mahajan and Goswami (Citation2001) and Techavuthiporn and Boonyaritthongchai (Citation2016). Airtight jars of size 3000 mL made up of glass fitted with lid were used. Each jar lid had three valves (inlet, outlet and gas sampling ports). A rubber ring was fixed between the bottle and the lid seal from air leakage. A plastic tube was attached to the inlet valve, which was inserted down to the bottom of the jar to ensure uniform flushing of gas mixture. Before sealing, jars were flushed with humidified air and the selected gas composition, until equilibrium archived. Three jars were flushed for each of the four MAs, resulting in 12 jars. The headspace gas (about 100 µL) was sampled at hourly intervals for the duration of 5 h at 5°C through the silicon septum provided in the jar lid. The headspace gas was analysed for O2 and CO2 concentrations using O2/CO2 gas analyser (Checkmate 3, PBI Dansensor, Ringstead, Denmark). This measurement cycle was repeated over a period of 12 days, based on experimental design reported by Ayhan and Esturk (Citation2009). The
and
were calculated using Equations (1) and (2):
where and
are, respectively, O2 concentration (kPa) at the initial time
(hours, h) and at time
(h) and
and
are, respectively, CO2 concentration (kPa) at the initial time
(h) and at time
(h).
and
are
in mL/kg h,
is temperature (Kelvin),
is universal gas constant (0.008314 kJ/K mol),
is the total weight of the product (kg) and
is the free volume (
), where
is the volume of the jar (m3),
is the weight of the minimally processed arils (kg) and ρ the apparent density of the arils (0.98 g/cm). The ratio of CO2 produced to O2 consumption is further expressed by the respiration quotient as follows:
Development of mathematical model of aril RR
RR was described by enzyme kinetics approach as a function of one limiting enzymatic reaction in which the substrate is O2 by using a simple MM model as described in Equation (4).
In order to account for the possible inhibitory effect of CO2, was described by an MM-type model for change in gas composition under MA storage (Guillard, Guillaume, & Destercke, Citation2012; Paul & Clarke, Citation2002). MM competitive inhibition (Equation (5)), where both the inhibitor (CO2) and the substrate (O2) compete for the same active site; uncompetitive (Equation (6)), where CO2 reacts with the substrate–enzyme complex; and non-competitive (Equation (7)), when CO2 reacts both with the enzyme and within the enzyme–substrate complex, models were fitted to the data obtained experimentally to account for the possible effect of
on O2 consumption rate (Gomes, Beaudry, Almeida, & Malcata, Citation2010). According to Fonseca, Oliveira, and Brecht (Citation2002), during competitive inhibition, the maximum RR is lower in high CO2 concentration, whereas, for uncompetitive inhibition, the maximum RR is not much influenced by high CO2; on the other hand, for non-competitive, the maximum RR lies between competitive and uncompetitive inhibitions. The models variables were analysed and compared to select the best fit of the experimental data (Equations (5)–(7)):
where Vmax is the maximal value of RR for O2 consumption (mL/kg h),
and
are the concentrations of O2 and CO2, respectively, inside the glass jar in kPa, Km is MM constant for O2 consumption and
is inhibition constant for CO2 evolution. Estimation of model MM parameters and constants, based on the experimental data, was performed by non-linear regression based on the Levenverg–Marguardt method using STATISTICA software (vr. 13 StatSoft Inc., Tulsa, USA). The model estimates obtained at each MA conditions were used to describe the dependency of the model parameters on gas composition. The accuracy of the models’ estimation was given by standard error (SE) value.
Statistical analysis
The results were presented as mean and standard deviation of three measurements. The data were analysed using STATISTICA software (vr. 13 StatSoft Inc., Tulsa, USA). Two-way ANOVA was used to investigate the effects of atmosphere modification and storage duration on RR of pomegranate arils. Fishers’ least significant difference test was used to determine significant differences among the means of response variables (p < 0.05).
Results and discussion
Change in head space gas composition
Concentrations of O2 and CO2 changed significantly with respect to the different initial gas concentrations and storage duration as shown in . The decrease in O2 concentration showed similar trend. Initial O2 concentration of 5 kPa decreased to a concentration of 2.04 kPa for low O2 (MA-1) and from 10 kPa for MA-2 atmospheres at day 12. Similarly, O2 concentration decreased from 21 kPa to 14.06 kPa for air storage and from 70.05 kPa of O2 decreased to 37.83 kPa under super-atmospheric O2. Meanwhile, the headspace CO2 concentration increased in all MA storage from the first day of storage and it reached the recommended range of <10–20 kPa CO2 for pomegranate arils except under MA-3 storage treatment where the CO2 concentration was >20 kPa on day 6. Furthermore, CO2 increased from 9.3 kPa to 15.7 kPa for low O2 atmosphere and from 10.15 kPa to 28.56 kPa under super-atmospheric O2 condition at day 12. However, the increase in CO2 was highest (17.08 kPa) for arils stored under MA-4 at day 12 compared with the initial concentration (0.03 kPa).
Figure 1. Effect of initial gas atmosphere on the change in headspace gas concentration of pomegranate arils stored at 5°C for 12 days. Error bar represents standard deviation of mean values (n = 3) at 95% confident interval. (a) O2 consumption and (b) CO2 production. MA-1: (5 kPa O2, 10 kPa, CO2 and 85 kPa N2); MA-2: (10 kPa O2, 5 kPa CO2 and 85 kPa N2), MA-3: (70 kPa O2, 10 kPa CO2 and 20 kPa N2); and MA-4 (air).
Figura 1. Efecto de la atmósfera de gas inicial en el cambio de concentración de gases en el espacio de cabeza de arilos de granada almacenados a 5°C durante 12 días. La barra de error representa la desviación estándar de los valores medios (n = 3) con un intervalo de confianza de 95%. (A) Consumo de O2 y (B) Producción de CO2. MA-1: (5 kPa O2, 10 kPa, CO2 y 85 kPa N2); MA-2: (10 kPa O2, 5 kPa CO2 y 85 kPa N2), MA-3: (70 kPa O2, 10 kPa CO2 y 20 kPa N2); y MA-4 (aire).
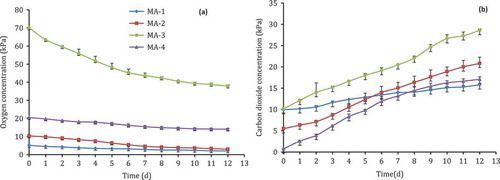
Increase in O2 consumption and CO2 production corresponding to different initial gas concentrations were observed. This phenomenon was reflected in the gradual linear reduction of slope for O2 concentration while coefficients of determination () for fitted linear models ranged from 0.98 to 0.99 for all MA conditions. The linearity of the model could be attributed to the effect of increasing production of CO2 and decreasing O2 concentration over time. The linear changes in O2 concentration in this study were in agreement with the pattern of a linear decline under aerobic conditions whereas non-linearity can be indicative of anaerobic RR or suppression of respiratory activity due to limited O2 concentration (Guevara, Yahia, Beaudry, & Cedeño, Citation2006). The result showed significant (p < 0.05) effects of storage MA condition and duration on headspace O2 consumption and CO2 evolution for arils. Similarly, Ayhan and Esturk (Citation2009) reported high CO2 concentration (35 kPa) for O2 enriched atmosphere at 18 days of storage. Accumulation of CO2 concentration within high O2 atmosphere was reported by Maghoumi et al. (Citation2014). This study further reported that the presence of high O2 concentration and accumulation of CO2 resulted in enhancement of the production of reactive O2 species that could cause some respiratory stress, increase in RR, induced more CO2 and depletion of antioxidants. Therefore, the increase in RR at MA-3 in the current study could be associated with the reason of highest accumulation of CO2.
Influence of initial gas composition on RR
The potential effect of initial gas concentration on RR of pomegranate arils stored at different MAs was analysed. The results indicated that gas concentration had significant (p < 0.05) effect on RR of pomegranate arils during cold storage. However, the interaction of time and MA types did not significantly affect the RR of pomegranate arils for all MA conditions applied. Based on the calculated RR values obtained from O2 consumption and CO2 production, it was observed that RR decreased over storage duration across all MA conditions. Comparing the RR of pomegranate arils at different atmospheres; for low O2 atmospheres, RO2 decreased from 3.14 mL/kg h to 0.87 mL/kg h at low O2 atmosphere for MA-1 and RO2 decreased from 4.11 mL/kg h to 0.94 mL/kg h for MA-2 and from 7.25 mL/kg h to 2.81 at super-atmospheric O2 atmosphere. Whereas for arils at ambient atmosphere, RO2 decreased from 5.16 mL/kg h to 1.95 mL/kg h (). Generally, the RR appeared to slow down as time progressed for all MA storage conditions. Furthermore, the effect of O2 concentration on RR of pomegranate arils clearly showed when the RR decreases with the decrease in O2 concentration. Similarly, pomegranate arils increased from 0.875 mL/kg h to 2.81 ± 0.37 mL/kg h by increasing the O2 concentration from 5 to 70 kPa at day 12. Lowest CO2 production rate (
) was 1.15 mL/kg h under MA-1 atmosphere compared to MA-2, MA-3 and MA-4. For the other MA conditions (MA-2 and MA-4), which had 10 and 21 kPa initial O2 concentration, respectively,
of arils ranging from 0.94 mL/kg h for MA-2 and 1.95 mL/kg h for MA-4 was observed at the end of storage time (day 12).
Figure 2. Effect of gas concentration on the calculated respiration rate (RR) oxygen (a) and carbon dioxide (b) of minimally processed pomegranate arils stored at 5°C for 12 days. Error bar represents standard deviation of mean values (n = 3) at 95% confident interval. MA-1: (5 kPa O2, 10 kPa, CO2 and 85 kPa N2); MA-2: (10 kPa O2, 5 kPa CO2 and 85 kPa N2), MA-3: (70 kPa O2, 10 kPa CO2 and 20 kPa N2); and MA-4 (air).
Figura 2. Efecto de la concentración de gas en la frecuencia de respiración (FR) calculada, oxígeno (A) y dióxido de carbono (B) de arilos de granada mínimamente procesados y almacenados a 5°C durante 12 días. La barra de error representa la desviación estándar de los valores medios (n = 3) con un intervalo de confianza de 95%. MA-1: (5 kPa O2, 10 kPa, CO2 y 85 kPa N2); MA-2: (10 kPa O2, 5 kPa CO2 y 85 kPa N2), MA-3: (70 kPa O2, 10 kPa CO2 y 20 kPa N2); y MA-4 (aire).
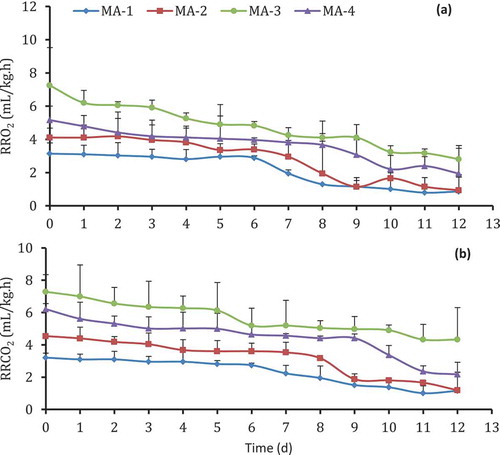
In addition, reducing the O2 concentration from 70 to 5 kPa decreased the at the same magnitude as
. This finding is supported by the fact that RR decreases with decreasing availability of O2 through the reduction of overall metabolic activity (Oms-Oliu, Soliva-Fortuny, & Martín-Belloso, Citation2008). The reduction in RR response due to low O2 could be a result of decrease in activity of oxidative enzymes such polyhenoloxidase, ascorbic acid oxidase and glycolic acid oxidase (Conesa, Verlinden, Artés-Hernández, Nicolaï, & Artés, Citation2007). MA-2 enhanced CO2 production rate after 5 days of storage in comparison to MA-1 and MA-4 atmospheres. Furthermore, the rapid depletion of O2 and accumulation of CO2 can be related to the low barrier properties of the glass jars used for the experiment. Similarly, low RR of pomegranate arils (cv. Hicaz) stored at 4°C under a combination of low O2 (2 kPa) and high CO2 (10 kPa) was reported by Ersan et al. (Citation2010). The authors reported that the minimum
of 1.5 mL/kg h and
of 0.52 mL/kg h of pomegranate arils was obtained at low O2 (2 kPa). Aindongo et al. (Citation2014) reported
and
of ‘Bhagwa’ pomegranate arils with ranges 1.9–18.6 mL/kg h and 3.2–28.9 mL/kg h, respectively, stored at 5–22°C, while Caleb, Mahajan, Opara, and Witthuhn (Citation2012c) observed
of 2.5–7.6 mL/kg h and
ranges from 2.7 to 9.0 mL/kg h for pomegranate arils (cvs. Acco and Herskawitz) stored at 5–15°C. In addition, Banda and others (Citation2015) investigated the effects of different combination of atmospheres (5 kPa O2 + 10 kPa CO2 + 85 kPa N2; 30 kPa O2 + 10 kPa CO2 + 60 kPa N2; 100 kPa N2; and air) on post-storage RR of ‘Wonderful’ pomegranate arils. The authors reported that
significantly changed over time and the highest RR was observed for pomegranate arils stored under passive MA in high barrier films, while arils packed under 100 kPa N2 maintained the lowest RR (0.58 ± 0.12 kg−1 h−1) at day 12.
Increase in RR (from 1.77 to 2.30 mL/kg h) of pomegranate arils (cv. Malese-Saveh) under 90 kPa O2 condition has been reported (Maghoumi et al., Citation2013). Furthermore, increase in RR under super-atmospheric O2 concentration was reported by Jacxsens, Devlieghere, and Debevere (Citation2002) for mushroom, grated celeriac and shredded chicory endives. Similar observation was reported by Allende, Luo, Mc-Evoy, Artés, and Wang (Citation2004) for baby spinach leaves under super-atmospheric O2 (>70 kPa). The authors observed highest and
under super-atmospheric O2 MA condition. The increase in RR was associated with the production of reactive O2 species and respiratory stress due to the presence of high O2 concentration (Jacxsens et al., Citation2002). However, the effect of super-atmospheric O2 depends on the commodity, maturity and ripeness stage, O2 and CO2 concentration, time and storage temperature (Kader & Ben-Yehoshua, Citation2000). Overall, the data suggested that low O2 atmosphere would be important to lower the RR of pomegranate arils at 5°C compared to super-atmospheric O2 and passive atmosphere.
Respiration quotient (RQ)
The average ratio of CO2 produced and O2 consumed (RQ) value of pomegranate arils stored at all MA conditions was within the range of acceptable limit (0.7–1.3) for aerobic respiration of fruits and vegetables (Kader, Zagory, Kerbel, & Wang, Citation1989). In contrast, arils under super-atmospheric O2 (MA-3) condition after 10 days of storage had the highest RQ of 1.54. The lowest RQ was observed for pomegranate arils stored under MA-4 treatment. The results further showed that the RQ for all MA conditions increased slightly at the end of 7 d storage. Based on the assumption that the value of RQ for fresh produce is equal to 1 when the metabolic substrates oxidized during respiration are carbohydrates (Castro-Giraldez, Fito, Ortola, & Balaguer, Citation2013; Fonseca et al., Citation2002), it can be suggested that the fermentation threshold for stored pomegranate arils was not reached across all MA conditions. On the other hand, a slight increase in RQ > 1 suggests that the oxidized substrates were organic acidic (Fonseca et al., Citation2002). Furthermore, Conesa et al. (Citation2007) associated the higher value of RQ with the production of high CO2 for fresh-cut bell peppers. Similar to the current study, RQ values ranges from 1.14 to 1.26 for minimally processed pomegranate arils ‘Acco’ and ‘Herskawitz’ were reported by Caleb et al. (Citation2012b) and RQ values of 0.9–1.24 for citric acid treated and untreated pomegranate arils (cv. Wonderful) by Banda, Caleb, and Opara (Citation2014) at passive atmosphere.
The results showed that gas concentration had no influence on the RQ of pomegranate arils (as shown in ), except a slight increase (38%) from 0.95 to 1.33 when the O2 concentration dropped below 2.81 kPa for MA-1 at day 12. Since the lower O2 limit can be determined by the immediate increase in RQ (Beaudry, Citation1999), and if the lowest O2 limit is considered to be the O2 concentration that causes a 50% increase in the RQ as stated by Hong and Kim (Citation2001), then the lowest O2 limit of ‘Wonderful’ pomegranate arils can be estimated to be less than 2.8 kPa. This further showed the influence of gas concentration on RQ and the slight but non-significant change in the respiratory process.
Table 2. Effects of modified atmosphere on respiratory quotient (RQ) of pomegranate arils stored at 5°C for 12 d.
Tabla 2. Efectos de la atmósfera modificada en el cociente respiratorio (CR) de arilos de granada almacenados a 5°C durante 12 días.
Modelling the influence of gas composition on RR
MM model with different types of inhibition were tested using the experimental data. The predictions from the MM models as well as the inhibition models (competitive, un-competitive and non-competitive) were compared by using the model parameter estimates as presented in . The MM model parameters for each gas concentration ( and
) and the CO2 inhibition constant (
were estimated individually from the experimental data obtained at each gas concentration and their MA dependence subsequently studied using Equations (5), (6) and (7). All the four types of MM enzyme kinetics models were capable of describing the O2 consumption rate as function of MA (MA-2, MA-3 and MA-4 treatments) with observed percentage variance (R2adj = 81–91%). Whereas, lower percentage variance of R2adj > 70% were found for (MA-1) which had the lowest initial O2 concentration (2 kPa), except for competitive inhibition model. This could be due to the presence of very low O2, which can influence effectiveness of MM model, since the model is effective when the reaction is in MM type (Hertog, Peppelenbos, Evelo, & Tijskens, Citation1998).
Table 3. Model parameter estimates obtained from non-linear regression analysis for enzyme kinetics model for O2 consumption rate in closed system under various initial O2 and CO2 concentrations.
Tabla 3. Estimaciones de los parámetros del modelo obtenidas del análisis de regresión no lineal para el modelo de cinética enzimática de la tasa de consumo de O2 en un sistema cerrado bajo varias concentraciones iniciales de O2 y CO2.
Both the maximum O2 consumption rate and the MM model constant ( and
) varied between the different MAs. Highest
was observed in MA-3 and lowest was for MA-1. This can be inferred that both parameters were influenced by gas composition. The increase in
with increase in O2 concentrations suggested that
was dependent on gas composition. The gas concentration dependency of
increased substantially when O2 concentration increased from low to super-atmospheric O2 (). In addition, the highest value of
which represent the O2 concentration at which half the maximum RR is reached (assuming no inhibition by CO2), was observed under super-atmospheric O2 (MA-3). Given that all the arils were harvested at commercial maturity and processed under the same condition, the observed variation in the
values might be attributed to the differences in the tissue resistance to diffusion commonly associated with storage MA (Gomes et al., Citation2010). Furthermore, this observation could suggest that the internal O2 concentration in the arils increased proportional to the external O2 concentration applied (Hertog et al., Citation1998).
Figure 3. Correlation of head space O2 concentration (kPa) on the O2 consumption rate (mL/kg h) of minimally processed pomegranate arils stored at 5°C for 12 days in a closed system. MA-1: (5 kPa O2, 10 kPa, CO2 and 85 kPa N2); MA-2: (10 kPa O2, 5 kPa CO2 and 85 kPa N2), MA-3: (70 kPa O2, 10 kPa CO2 and 20 kPa N2); and MA-4 (air).
Figura 3. Correlación de espacio de cabeza, concentración de O2 (kPa) en la tasa de consumo de O2 (mL/kg h) de arilos de granada mínimamente procesados y almacenados a 5°C durante 12 días en un sistema cerrado. MA-1: (5 kPa O2, 10 kPa, CO2 y 85 kPa N2); MA-2: (10 kPa O2, 5 kPa CO2 y 85 kPa N2), MA-3: (70 kPa O2, 10 kPa CO2 y 20 kPa N2); y MA-4 (aire).
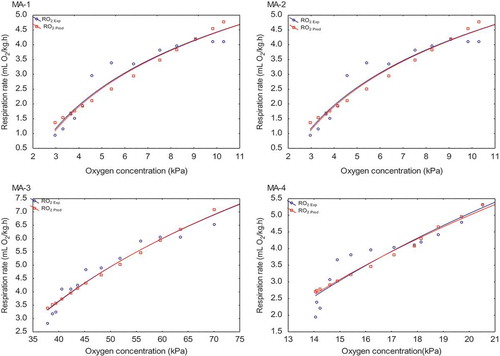
Comparing simple and inhibition models for each atmosphere, there was no clear difference on effect of CO2 on O2 consumption rate for all MAs. However, according to the , which showed the extent to which RR can be inhibited by CO2 in , the simultaneous existence of competitive, un-competitive and non-competitive inhibition were observed depending on the initial gas concentration. Comparing all models, relatively higher
value was observed in MA-1 during competitive inhibition model, which implies inhibition by CO2 has not occurred, whereas, a slight un-competitive and non-competitive inhibition was observed. This indicated that a model which combined un-competitive and non- competitive inhibition could better explain the CO2 effect on the RR (Guillard et al., Citation2012). On the contrary, the RR of pomegranate arils for the other atmospheres was competitive inhibition, with the lowest
. However, the influence of CO2 on the metabolic rate could be due to its effect on changing the pH than its direct influence on enzymatic reaction (Torrieri et al., Citation2010).
The values obtained from the current experiment were similar to those reported by Ersan et al. (Citation2010) for ‘Hicaz’ pomegranate arils at 5°C. At low O2,
of 3.6 mL kg/h was comparable to 3.1 mL kg/h reported by Ersan et al. (Citation2010). In contrast, the
and
values were different. The authors found relatively higher
ranging from 3.8 to 5.1 for ‘Hicaz’ pomegranate arils, whereas the values in the current experiment were within the range of 0.29–2.67 for ‘Wonderful’ under-low O2 atmosphere. This variation in
could be associated with the different model-fitting methods used. Ersan et al. (Citation2010) linearized the data before fitting, while for the current study a non-linear regression model has been used, since linearization is equivalent to changing the weight given to the data in the estimation procedure and it should be avoided (Fonseca et al., Citation2002).
Comparing the accuracy of the parameter estimates by using the estimated SEs for all models, small SE values of all and
were observed. Low SE values (lower value than the sample mean) for the parameter estimates can be considered as well defied (Hertog, Lammertyn, Scheerlinck, & Nicolaï, Citation2007). Thus, it can be concluded that the models accurately predicted the influence of MA on the RR of ‘Wonderful’ pomegranate arils. On the other hand, the accuracy for the competitive and non-competitive inhibition model for MA-1 and for non-competitive inhibition for MA-3 could be less defined by the increase in SE for
. However, the higher SE could also explain the coexistence of both competitive and uncompetitive inhibition of RR by CO2 (Hertog et al., Citation2007). Furthermore, the model parameters were statistically significant (p < 0.05) and the correlation coefficient of determination was R2 = 0.85–0.97% for all atmospheres. This showed a good fit of the model to the experimental data. Therefore, these results demonstrated that the MM enzyme kinetics models can be used to describe the dependency of RR of pomegranate arils on the modified storage atmospheres. Since the results for MA-2, MA-3 and MA-4 showed a competitive inhibition RR and a slight combination of competitive and un-competitive inhibition for MA-1, a non-linear regression was applied to the whole set of experimental data using competitive model. The model adequately predicted the O2 consumption rate (R2 = 0.94%) as shown in , with a normal distribution of the residual values. Based on this result, it can be suggested that competitive inhibition can be able to describe the effect of CO2 to O2 consumption rate at low and super-atmospheric O2 condition.
Figure 4. Correlation between experimental data and predicted (mL/kg h) using Michaelis–Menten competitive inhibition model. Small plot inserted shows the frequency distribution of the residuals.
Figura 4. Correlación entre los datos experimentales y el (mL/kg h) previsto, utilizando el modelo de inhibición competitiva Michaelis-Menten. El pequeño trazado insertado muestra la distribución de frecuencia de los residuales.
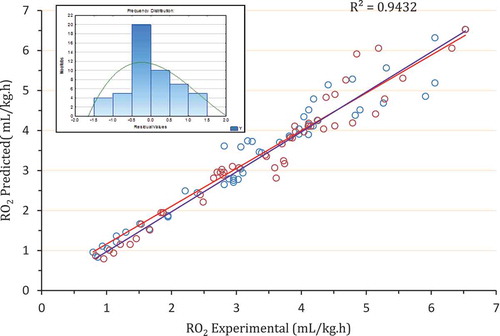
Conclusion
This study showed that RR of pomegranate arils (cv. Wonderful) was significantly affected by initial gas concentrations during storage. Low O2 MA conditions reduced the RR of the arils. In contrast, the use of super-atmospheric O2 concentration (70 kPa) induced physiological stress, and consequently slightly increased the RR of arils. Based on the respiratory kinetics of pomegranate arils, this study showed that super-atmospheric O2 is of limited or no benefit in slowing down the metabolic process of pomegranate arils. The MM enzyme kinetics model (using competitive-inhibition-type equation) adequately described the effect of CO2 on O2 consumption rate at low O2 (5 kPa), super-atmospheric O2 (70 kPa) and enriched CO2 concentrations. The parameter estimates obtained provide a guiding step for the management of MA systems to avoid unfavourable conditions for pomegranate arils (cv. Wonderful).
Disclosure statement
No potential conflict of interest was reported by the authors.
Additional information
Funding
References
- Aindongo, W.V., Caleb, O.J., Mahajan, P.V., Manley, M., & Opara, U.L. (2014). Modelling the effects of storage temperature on the respiration rate of different pomegranate fractions. South African Journal of Plant and Soil, 31(4), 227–231. doi:10.1080/02571862.2014.944230
- Allende, A., Luo, Y., Mc-Evoy, J.L., Artés, F., & Wang, C.Y. (2004). Microbial and quality changes in minimally processed baby spinach leaves stored under super-atmospheric oxygen and modified atmosphere conditions. Postharvest Biology and Technology, 33(1), 51–59. doi:10.1016/j.postharvbio.2004.03.003
- Ayhan, Z., & Esturk, O. (2009). Overall quality and shelf life of minimally processed and modified atmosphere packaging ready to eat pomegranate arils. Journal of Food Science, 74(5), 399–405. doi:10.1111/j.1750-3841.2009.01184.x
- Banda, K., Caleb, O.J., Jacobs, K., & Opara, U.L. (2015). Effect of active-modified atmosphere packaging on the respiration rate and quality of pomegranate arils (cv. Wonderful). Postharvest Biology and Technology, 109, 97–105. doi:10.1016/j.postharvbio.2015.06.002
- Banda, K., Caleb, O.J., & Opara, U.L. (2014). Effect of citric acid and storage conditions on the respiration rate of ‘Wonderful’ pomegranate arils. V International Conference of Postharvest Unlimited, 1079, 481–486. doi:10.17660/ActaHortic.2015.1079.63
- Beaudry, R.M. (1999). Effect of O2 and CO2 partial pressure on selected phenomena affecting fruit and vegetable quality. Postharvest Biology and Technology, 15(3), 293–303. doi:10.1016/S0925-5214(98)00092-1
- Belay, Z.A., Caleb, O.J., & Opara, U.L. (2016). Modelling approaches for designing and evaluating the performance of modified atmosphere packaging (MAP) systems for fresh produce: A review. Food Packaging and Shelf Life, 10(1), 1–15. doi:10.1016/j.fpsl.2016.08.001
- Belay, Z.A., Caleb, O.J., & Opara, U.L. (2017). Impacts of low and super-atmospheric oxygen concentrations on quality attributes, phytonutrient content and volatile compounds of minimally processed pomegranate arils (cv. Wonderful). Postharvest Biology and Technology, 124, 5119–5127. doi:10.1016/j.postharvbio.2016.10.007
- Caleb, O.J., Mahajan, P.V., Opara, U.L., & Witthuhn, C.R. (2012a). Modelling the respiration rates of pomegranate fruit and arils. Postharvest Biology and Technology, 64(1), 49–54. doi:10.1016/j.postharvbio.2011.09.013
- Caleb, O.J., Mahajan, P.V., Opara, U.L., & Witthuhn, C.R. (2012c). Modelling the effect of time and temperature on respiration rate of pomegranate arils (cv. Acco and Herskawitz). Journal of Food Science, 77(4), 80–87. doi:10.1111/j.1750-3841.2012.02623.x
- Caleb, O.J., Opara, U.L., & Witthuhn, C.R. (2012b). Modified atmosphere packaging of pomegranate fruit and arils: A review. Food Bioprocess Technology, 5, 15–30. doi:10.1007/s11947-011-0525-7
- Castro-Giraldez, M., Fito, P.J., Ortola, M.D., & Balaguer, N. (2013). Study of pomegranate ripening by dielectric spectroscopy. Postharvest Biology and Technology, 86, 346–353. doi:10.1016/j.postharvbio.2013.07.024
- Cliffe-Byenes, V., & O`Beirne, D. (2007). Effect of gas atmosphere and temperature on the respiration rates of whole and sliced mushrooms (Agaricus bisporus) – implications for film permeability in modified atmosphere packages. Journal of Food Science, 74(4), 197–204. doi:10.1111/j.1750-3841.2007.00321
- Conesa, A., Verlinden, B.E., Artés-Hernández, F., Nicolaï, B., & Artés, F. (2007). Respiration rates of fresh-cut bell peppers under super atmospheric and low oxygen with or without high carbon dio xide. Postharvest Biology and Technology, 45(1), 81–88. doi:10.1016/j.postharvbio.2007.01.011
- Ersan, S., Gunes, G., & Zor, A.O. (2010). Respiration rate of pomegranate arils as affected by O2 and CO2, and design of modified atmosphere packaging. In X International Control and Modified Atmosphere Research Conference, 876, 189–196. doi:10.17660/ActaHortic.2010.876.24
- Fonseca, S.C., Oliveira, F.A.R., & Brecht, J.K. (2002). Modelling respiration rate of fresh fruits and vegetables for modified atmosphere packages: A review. Journal of Food Engineering, 52, 99–119. doi:10.1016/S0260-8774(01)00106-6
- Gomes, M.H., Beaudry, R.M., Almeida, D.P., & Malcata, F.X. (2010). Modelling respiration of packaged fresh-cut ‘Rocha’ pear as affected by oxygen concentration and temperature. Journal of Food Engineering, 96(1), 74–79. doi:10.1016/j.jfoodeng.2009.06.043
- Guevara, J.C., Yahia, E.M., Beaudry, R.M., & Cedeño, L. (2006). Modelling the influence of temperature and relative humidity on respiration rate of prickly pear cactus cladodes. Postharvest Biology and Technology, 41, 260–265. doi:10.1016/j.postharvbio.2006.04.012
- Guillard, V., Guillaume, C., & Destercke, S. (2012). Parameter uncertainties and error propagation in modified atmosphere packaging modelling. Postharvest Biology and Technology, 67, 154–166. doi:10.1016/j.postharvbio.2011.12.014
- Hertog, M.L., Lammertyn, J., Scheerlinck, N., & Nicolaï, B.M. (2007). The impact of biological variation on postharvest behaviour: The case of dynamic temperature conditions. Postharvest Biology and Technology, 43(2), 183–192. doi:10.1016/j.postharvbio.2006.09.014
- Hertog, M.L.A.T.M., Peppelenbos, H.W., Evelo, R.G., & Tijskens, L.M.M. (1998). A dynamic and generic model of gas exchange of respiring produce: The effects of oxygen, carbon dioxide and temperature. Postharvest Biology and Technology, 14(3), 335–349. doi:10.1016/S0925-5214(98)00058-1
- Hong, S.I., & Kim, D.M. (2001). Influence of oxygen concentration and temperature on respiratory characteristics of fresh‐cut green onion. International Journal of Food Science and Technology, 36(3), 283–289. doi:10.1046/j.1365-2621.2001.00456.x
- Iqbal, T., Rodrigues, F.A., Mahajan, P.V., & Kerry, J.P. (2009). Mathematical modelling of the influence of temperature and gas composition on the respiration rate of shredded carrots. Journal of Food Engineering, 91(2), 325–332. doi:10.1016/j.jfoodeng.2008.09.012
- Jacxsens, L., Devlieghere, F., & Debevere, J. (2002). Predictive modelling for packaging design: Equilibrium modified atmosphere packages of fresh-cut vegetables subjected to a simulated distribution chain. International Journal of Food Microbiology, 73(2), 331–341. doi:10.1016/S0168-1605(01)00669-9
- Jing-Jun, Y., Jain-Rong, L.I., Xiao-Xiang, H., Lei, Z., Tian-Jia, J., & Miao, X. (2012). Effect of active modified atmosphere packaging on postharvest quality of shiitake mushrooms (Lentinula edodes) stored at cold storage. Journal of Integrated Agriculture, 11(3), 474–482. doi:10.1016/S2095-3119(12)60033-1
- Kader, A.A., & Ben-Yehoshua, S. (2000). Effects of super atmospheric oxygen levels on postharvest physiology and quality of fresh fruits and vegetables. Postharvest Biology and Technology, 20(1), 1–13. doi:10.1016/S0925-5214(00)00122-8
- Kader, A.A., Zagory, D., Kerbel, E.L., & Wang, C.Y. (1989). Modified atmosphere packaging of fruits and vegetables. Critical Review in Food Science and Nutrition, 28(1), 1–30. doi:10.1080/10408398909527490
- López-Rubira, V., Conesa, A., Allende, A., & Artés, F. (2005). Shelf life and overall quality of minimally processed pomegranate arils modified atmosphere packaged and treated with UV-C. Postharvest Biology and Technology, 37(2), 174–185. doi:10.1016/j.postharvbio.2005.04.003
- Maghoumi, M., Gómez, P.A., Artés‐Hernández, F., Mostofi, Y., Zamani, Z., & Artés, F. (2013). Hot water, UV‐C and super atmospheric oxygen packaging as hurdle techniques for maintaining overall quality of fresh‐cut pomegranate arils. Journal of the Science of Food and Agriculture, 93(5), 1162–1168. doi:10.1002/jsfa.5868
- Maghoumi, M., Mostofi, Y., Zamani, Z., Talaie, A., Boojar, M., & Gómez, P.A. (2014). Influence of hot‐air treatment, super atmospheric O2 and elevated CO2 on bioactive compounds and storage properties of fresh‐cut pomegranate arils. International Journal of Food Science and Technology, 49(1), 153–159. doi:10.1111/ijfs.12290
- Mahajan, P.V., & Goswami, T.K. (2001). Enzyme kinetics based modelling of respiration rate for apple. Journal of Agricultural Engineering Research, 79(4), 399–406. doi:10.1006/jaer.2001.0718
- Molinu, M.G., Dore, A., Palma, A., D’Aquino, S., Azara, E., Rodov, V., & D’hallewin, G. (2016). Effect of super-atmospheric oxygen storage on the content of phytonutrients in ‘Sanguinello Comune’ blood orange. Postharvest Biology and Technology, 112, 24–30. doi:10.1016/j.postharvbio.2015.09.037
- Mphahlele, R.R., Caleb, O.J., Fawole, O.A., & Opara, U.L. (2016). Effect of different maturity stages and growing locations on changes in chemical, biochemical and aroma volatile composition of ‘Wonderful’ pomegranate juice. Journal of Science of Food and Agriculture, 96(3), 1002–1009. doi:10.1002/jsfa.7186
- Oms-Oliu, G., Soliva-Fortuny, R., & Martín-Belloso, O. (2008). Physiological and microbiological changes in fresh-cut pears stored in high oxygen active packages compared with low oxygen active and passive modified atmosphere packaging. Postharvest Biology and Technology, 48(2), 295–301. doi:10.1016/j.postharvbio.2007.10.002
- Paul, D.R., & Clarke, R. (2002). Modelling of modified atmosphere packaging based on designs with a membrane and perforations. Journal of Membrane Science, 208(1), 269–283. doi:10.1016/S0376-7388(02)00303-4
- Techavuthiporn, C., & Boonyaritthongchai, P. (2016). Effect of prestorage short-term Anoxia treatment and modified atmosphere packaging on the physical and chemical changes of green asparagus. Postharvest Biology and Technology, 117, 64–70. doi:10.1016/j.postharvbio.2016.01.016
- Torrieri, E., Perone, N., Cavella, S., & Masi, P. (2010). Modelling the respiration rate of minimally processed broccoli (Brassica rapa var. sylvestris) for modified atmosphere package design. International Journal of Food Science & Technology, 45(10), 2186–2193. doi:10.1111/j.1365-2621.2010.02387.x