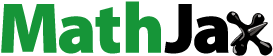
ABSTRACT
Maize silks are used worldwide as a natural-based treatment for various illnesses. The biological activity of maize silks is mainly attributed to their content of polysaccharides and polyphenols. Therefore, it is worthwhile the identification of maize varieties or races that accumulate large amounts of these compounds in their staminate flowers. In the present study, the compositional and antioxidant properties of silks from three maize races (Cónico, Gordo and Cristalino) natives to Mexico were investigated. In general, the content of phenolic compounds including flavonoids and anthocyanins as well as the antioxidant capacity of maize silks from the landrace Cónico were higher than values reported in silks of hybrid maize varieties. The presence of the flavonoid maysin was confirmed in the silks of the landrace Cónico but not in the other two races. Whereas, the largest amount of polysaccharides was observed in silks from the landrace Cristalino.
RESUMEN
Las sedas del maíz (estigmas de maíz) se utilizan en todo el mundo como un tratamiento natural para diversas enfermedades. La actividad biológica de las sedas del maíz se atribuye principalmente a su contenido de polisacáridos y polifenoles. Por lo tanto, es importante identificar variedades o razas de maíz que acumulen una gran cantidad de estos compuestos en sus estigmas. En el presente estudio se investigaron las propiedades de composición y antioxidantes de las sedas de tres razas de maíces nativos de México (Cónico, Gordo y Cristalino). El contenido de polifenoles como flavonoides y antocianinas así como la capacidad antioxidante fue mayor en las sedas de la raza Cónico que lo reportado previamente para las sedas de maíces híbridos. La presencia del flavonoide maysina fue confirmado en las sedas de la raza Cónico, pero no en las otras dos razas. Mientras que la mayor cantidad de polisacáridos se observó en las sedas de de la raza Cristalino.
1. Introduction
The domestication of maize occurred in southern Mexico about 9000 years ago and gave rise to a group of ancestral landraces adapted to diverse climatic and geographic conditions. Over time, maize spread throughout the continent and all over the world, as well as the utilization of maize silks for medicinal purposes (Kato-Yamakake, Mapes-Sánchez, Mera-Ovando, Serratos-Hernández, & Bye-Boettler, Citation2009; Vielle-Calzada & Padilla, Citation2009). Nowadays, maize silks are employed worldwide as an alternative natural-based treatment for various illnesses, standing out their use in Latin American and Asiatic countries (Hasanudin, Hashim, & Mustafa, Citation2012; Žilić, Janković, Basić, Vančetović, & Maksimović, Citation2016).
Maize silks are the female inflorescences of maize which arise from the fusion of two carpels that surrounds a single ovule. After a successful fertilization, this ovule becomes a kernel in the maize cob. During pollination, the tassel release copious amounts of pollen that are captured by the silks. Then, a tube grows from a pollen grain captured by a hair silk. This pollen tube penetrates into the hair silk delivering two sperm cells for a double fertilization. The silks will detach from the (immature) kernel just 2–3 days after the ovule has been fertilized, and an ear with few detached silks indicates that few ovules are fertilized (Bedinger & Fowler, Citation2009; Scanlon & Takacs, Citation2009). Therefore, pollen, kernels and silks share some components like sugars and phenolic compounds.
Traditionally, maize silks are used as a therapeutic remedy for urinary infections, kidney stones, cystitis, prostate disorders, obesity and diabetes (Hasanudin et al., Citation2012). Experimental studies in rats with kidney failure demonstrated that maize silks improve kidney function (Sukandar, Sigit, & Adiwibowo, Citation2013) and produce a renal protective action by decreasing lipid peroxidation (Karami et al., Citation2013). The hypoglycemic (Guo, Liu, Han, Liu, & Gou, Citation2009), hypotensive (George & Idu, Citation2015) hypocholesterolemic (Cha et al., Citation2016), neuroprotective (Doo Jin, Sun-Lim, Ji Won, & Yong, Citation2014) and anti-inflammatory effect of maize silks have been also confirmed. Likewise, it has been proved the capacity of maize silks to inhibit the expression of genes involved in adipocyte differentiation, fat accumulation and fat synthesis, reducing body fat accumulation in mice (E. Lee et al., Citation2016).
Most of the therapeutic effects of maize silks are attributed to the presence of polysaccharides or polyphenols. For instance, the reduction of cholesterol and fat deposition has been linked to the content of flavonoids (like maysin) in the silks (Cha et al., Citation2016; Kan, Orhan, Coksari, & Sener, Citation2012; E. Lee et al., Citation2016). Furthermore, the hypoglycemic capacity of maize silks is associated with its polysaccharide content (Zhao, Yin, Yu, Liu, & Chen, Citation2012). Experiments on diabetic rats revealed that consumption of 100–500 mg/kg body weight of maize-silk polysaccharides (from white-dented maize) reduces the blood glucose, serum lipid, triglyceride and cholesterol levels significantly (Zhao et al., Citation2012). It therefore seems worthwhile to identify maize varieties or races that accumulate large amounts of polysaccharides or polyphenols in their staminate flowers. The aim of this work was to characterize the gross and minor components of maize silks from three Mexican landraces of maize, as well as their free radical scavenging properties.
2. Materials and methods
2.1 Plant material
The female inflorescences from three maize races natives to Mexico were used in this study (). The landraces used were Gordo with white kernels (RG-w), Cónico with red kernels (RC-r), dark red kernels (RC-dr) and white-blue kernels (RC-wb) as well as the race Cristalino with yellow kernels (RCr-Y). The identification of the maize landraces was done with help of the local farmers, the geographical location of collection and their phenotypic traits as well. These landraces were selected to obtain silks with different colors and probably different content of polysaccharides and polyphenols. The maize cobs from each race of maize were harvested during the fall of 2015 in three different regions of Mexico (). The silks were carefully pulled apart from each maize cob, weighted (fresh weights) and then dried for 24 h at 45°C and 15 mm Hg (Shel Lab, model 19, Cornelius, Ore). The dried silks were milled (Mini-mill, Thomas Wiley Fisher, model 3383-L19, USA) reaching an approximate particle size of 250 μm (60-mesh sieve). The milled silks were then stored at −20°C in hermetically sealed glass bottles until their analysis.
Table 1. Maize landraces used in this study and some traits of their female inflorescences (maize silks) in fresh.
Tabla 1. Razas nativas de maíz usadas en éste estudio así como algunas características de sus inflorescencias femeninas (sedas de maíz) en fresco.
2.2 Proximate analysis
The water content of the fresh and dry silks was determined using the AOAC method 934.01 (AOAC International, Citation1998d), whereas the ash content was estimated by the AOAC method 942.05 (AOAC International, Citation1998a). The micro-Kjeldahl method (AOAC method 960.52) with a nitrogen conversion factor of 6.25 was used to calculate the content of protein (AOAC International, Citation1998c). The crude fat content was measured using a Soxhlet-type extractor (E-812 SOX, Büchi, Switzerland) and petroleum ether as solvent (AOAC International, Citation1998b). The total soluble and insoluble fiber was evaluated by the enzymatic–gravimetric method according to the AOAC method 991.43 (AOAC International, Citation1998e).
2.3 Analysis of minor constituents
The dried and milled silks from each landrace were subject to maceration (1:10 w/v) with an ethanol–water solution (80:20 v/v) for 24 h at 25°C. These crude ethanolic extracts were concentrated by evaporation (CCE) and used to measure the content of some minor constituents on the silks. The total content of sugars was determined by the phenol-sulfuric method (Chow & Landhäusser, Citation2004) and the reducing sugars were measured by the 3,5-dinitrosalicylic assay (Krivorotova & Sereikaite, Citation2014). The Folin–Ciocalteu method was used to calculate the total phenolic compounds (Blainski, Lopes, & de Mello, Citation2013), whereas flavonoid content was estimated by the colorimetric aluminum chloride method (Ebrahimzadeh, Pourmorad, & Hafezı, Citation2008). The quantification of anthocyanins was done according to the pH differential method as described by Lee (Citation2005). Further analysis of phenolic compounds by thin layer chromatography (TLC) was performed using silica gel plates (60F254) and chloroform–ethyl acetate (60:40) as mobile phase (Choi et al., Citation2002). After running, plates were air dried and viewed under UV light (Black ray lamp, San Gabriel, CA).
2.4 DPPH scavenging activity
The antioxidant capacity of silk extracts was measured using the DPPH (2,2-diphenyl-1-picrylhydrazyl) free radical scavenging activity assay (Sánchez-Moreno, Larrauri, & Saura-Calixto, Citation1998; Sarepoua, Tangwongchai, Suriharn, & Lertrat, Citation2015). Before the analysis, the total solids in the CCEs were determined gravimetrically after being dried on a stove and expressed as μm mL−1. Then, the CCEs were combined with different amounts of methanol for obtaining a set of diluted solutions of maize-silk extracts ranging from 2 to 10,000 mg mL−1. These concentrations were based on the total content of solids in the extracts was calculated with base of the total. An aliquot of 0.1 mL from these diluted extracts was added to 3.9 mL of DPPH solution (0.025 mol L−1 prepared daily). The mixture was incubated at 25°C in darkness for 4 h (time necessary to reach the steady state) and then read at 517 nm (Perkin Elmer, UV-Vis lambda 25, Norwalk, CT). The blank was prepared similarly but mixing 0.1 mL of methanol with 3.9 mL of DPPH solution. Ascorbic acid standards at 0.25, 0.625, 1.25, 2.5, 3.75 and 5 μm mL−1 were measured as described previously. The percentage of DPPH scavenging activity was calculated as follows:
The percentages of inhibition obtained at various concentrations of the maize-silk extracts were plotted and fitted to the following hyperbolic (single rectangular, two parameters) function:
The concentration of maize-silk extract required to decrease 50% of the initial DPPH free radical concentration (EC50) was obtained from each hyperbola equation using the SigmaPlot software (version 12, Jandel Scientific, San Rafael, CA).
2.5 Statistical analysis
Data obtained from the amount of silks (g) per single maize cob was fitted to normal distributions and subject to one-way analysis of variance (ANOVA) and multiple mean comparisons using the Tukey–Kramer method. Similarly, differences among maize races on their proximate and minor constituent composition were determined by one-way ANOVA and Tukey–Kramer test. Additionally, a principal component analysis (PCA) was calculated using a covariance matrix of non-standardized data obtained from the DPPH radical scavenging assays, as well as the proximate and minor constituent analysis. All the statistical tests were carried out with the software Minitab version 16 (Minitab Inc., Sate College, PA).
3. Results and discussion
3.1 General characteristics of the fresh maize silks
The most evident difference among fresh silks obtained from distinct maize landraces was their color (). Red silks were recovered from maize with red kernels (RC-r, RC-dr), whereas brown and light brown silks were recovered from white-dented maize (RG-W, RC-wb). The maize cobs with yellow kernels (RCr-Y) had translucent silks with a green-yellow color. The major component (>80%) of silks just after being pulled apart from the maize cob was water, irrespectively of the maize landrace (). The high moisture content in the silks is crucial for the accomplishment transportation of the pollen tube (that contains the male genetic material) through the silks. Only the staminate (female) flowers that emerge from the maize husk to capture the pollen become dry by the weather conditions (Vollbrecht & Schmidt, Citation2009). Regardless of the silks from all the maize landraces that had similar water content (82–88%), obvious discrepancies were observed among the mean weights of silks recovered per maize cob. Three out five maize landraces produced on average 5 g of silks from single maize cob, whereas the samples from RC-r, RC-dr yielded 12 g (). Once all the maize cobs were harvested at their maturity stage, it was ruled out that differences in the weights of silks recovered per maize cob were due to discrepancies on the maturity stages of the corn plants. However, the thickness of silks from RC-r and RC-dr was twice bigger than silks from white or yellow maize, which explains the higher weight per maize cob.
3.2 Main constituents of dried maize silks
Regardless of the maize landrace, the most abundant component in the dried maize silks was carbohydrates (65–70%), particularly fiber (39–53%). From this fiber content, the insoluble fiber was predominant (36–52%) and soluble fiber only represented a small fraction (>3%). Silks from some landraces had more insoluble fiber than others, like silks from white-dented maize (RG-w) (). Large proportions of insoluble fiber in the silks are undesired for the recovering of bioactive compounds, as long as the cell wall material may act as a barrier during a liquid–solid extraction. Besides, cellulose, hemicellulose and lignin may interact with polyphenols in reversible (hydrogen and hydrophobic bonds, van der Waals forces) or irreversible (covalent bonds) ways avoiding its release into the solvent of extraction (Le Bourvellec & Renard, Citation2012).
Table 2. Proximate analysis of the dried and milled maize silks obtained from different maize landraces.
Tabla 2. Análisis proximal de las sedas de maíz secas y molidas obtenidas de diferentes razas nativas de maíz.
The content of soluble fiber in the maize silks was small (), but it is noteworthy due to its claimed beneficial properties. The water-soluble fiber from plants consists of mucilages, gums, pectins, few hemicelluloses and polysaccharides. Some components from the water-soluble fiber of a wide variety of plants have been linked to beneficial effects on human health (Glore, Van Treeck, Knehans, & Guild, Citation1994), including the polysaccharides of maize silks (Hasanudin et al., Citation2012). Polysaccharides from maize silks are composed of various reducing sugars like glucose, galactose and mannose with some residues of xylose, arabinose and rhamnose. Although, the composition and content of polysaccharides in the silks have been reported to differ among maize varieties (Chen et al., Citation2014). Likewise, in this work, it was observed that silks from some maize landraces accumulate larger amounts of polysaccharides than others. For instance, the silks from white-dented maize (RG-w) had a proportion near to one (0.93) between reducing and total sugars (), which means that most of their sugars were as free sugars. Therefore, it can be assumed that the content of polysaccharides in these silks was small. In contrast, silks from the red- and yellow-dented maize (RC-r and RCr-Y) showed the lowest ratio between reducing and total sugars (>0.20) and thereby the largest presence of polysaccharides (), making these silks ideal for the extraction of polysaccharide. The discrepancies among silks in their content of polysaccharides might arise from its ability to capture a large amount of pollen. Mature pollen contains starch granules (besides sperm) that become hydrolyzed during germination of the pollen tube just after landing on a silk hair (Bedinger & Fowler, Citation2009). Then, the sperm and some hydrolyzed starch (polysaccharides) are delivered into the silks through the pollen tube. However, silks with larger size, higher number of multicellular hairs and high content of flavonoids may capture more pollen than others (Bedinger & Fowler, Citation2009), leading to a possible increase in polysaccharides.
Table 3. Content of phenolic compounds and saccharides on maize silks from maize races natives to Mexico.
Tabla 3. Contenido de compuestos fenólicos y sacáridos en las sedas de maíz obtenidas de maíces nativos de México.
3.3 Content of phenolic compounds
Silks from all the variants of the landrace Cónico (RC-r, RC-dr and RC-wb) had about 10-fold more phenolic compounds than silks from white-dented (RG-w) or yellow-dented maize (RCr-Y) (). A study conducted in Thailand reported that silks from purple maize had twice polyphenol content than those from yellow maize and five times more than silks from white maize (Sarepoua et al., Citation2015). Similarly, Žilić et al. (Citation2016) reported that red and pink silks from hybrid maize cultivated in Serbia had more polyphenols and flavonoids than green and yellow silks. The content of polyphenols in silks from maize races natives to Mexico has not been previously described but there is information about the polyphenol content in blue grains of the landrace Cónico. These blue grains were reported with a polyphenol content of 12.8 g GAE kg−1 (Urias-Lugo, Heredia, Serna-Saldivar, Muy-Rangel, & Valdez-Torres, Citation2015) which was somewhat lower than we observed in the red silks of the same race (). According to other authors, the silks accumulate more polyphenols than other parts of the corn plant like the husks, rachis or even the grains of the maize cob (Dong et al., Citation2014; Shahzadi, Sheikh, Hameed, Jamil, & Ur-Rehman, Citation2015).
White maize is the most cultivated race (or hybrid varieties) in Mexico (Kato-Yamakake et al., Citation2009), therefore, their silks are most used as herbal medicine. The silks from white maize had a phenolic content of 2.42 g GAE kg−1 (), which was in agreement with reported for silks of white hybrid maize (3.99 g GA kg−1) (Dong et al., Citation2014). The silks from white and yellow maize (RG-w and RCr-Y) had significantly less flavonoids than silks from red maize (RC-r and RC-dr) (), meaning that polyphenols in these silks are non-flavonoids. To confirm this, the maize-silk extracts were analyzed by TLC. The TLC plate showed that silks from white maize had mostly phenolic acids (lane 1, ), which are reveled as black spots under UV light (Fenton, Leung, & Clandinin, Citation1980). Similarly, Šukalović, Veljović-Jovanović, Maksimović, Maksimović, and Pajić (Citation2010) reported that phenolic compounds in silks of white hybrid maize are mainly non-flavonoids such as chlorogenic, ρ-cumaric and ferulic acids.
Figure 2. Thin layer chromatography (TLC) of polyphenols from maize silk extracts (100 μg mL−1). The TLC was performed on a silica gel plate run with chloroform–ethyl acetate (60:40) and visualized under ultraviolet light UV365. Rf = retention factors. Lanes: 1 = RG-w; 2 = RCr-Y; 3 = RC-wb; 4 = RC-dr; 5 = RC-r; 6 = quercetin standard.
Figura 2. Cromatografía en capa fina (TLC, por sus siglas en inglés) de polifenoles provenientes de extractos de sedas de maíz (100 μg mL−1). La TLC fue realizada en placas de silica gel y corridas con cloroformo – acetato de etílo (60:40) para ser posteriormente visualizadas bajo luz ultravioleta UV365. Rf = Factor de retención. Carriles: 1 = RG-w; 2 = RCr-y; 3 = RC-wb; 4 = RC-dr; 5 = RC-r; 6 = estándar de quercetina.
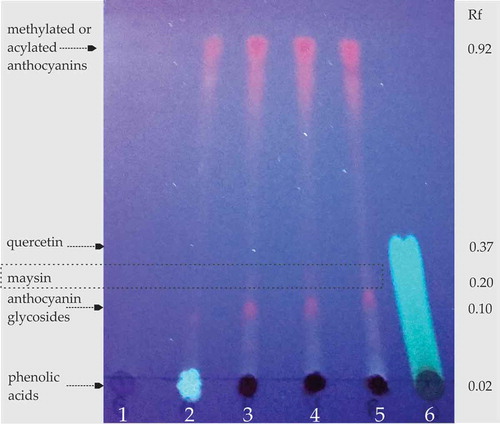
The TLC plate () also revealed pink and orange spots which are characteristics of anthocyanins (Takeoka & Dao, Citation2002) and flavonoid glycosides similar to quercetin (Marston & Hostettmann, Citation2005). These quercetin-like compounds observed in silks from colored maize (RC-r, RC-dr and RC-wb) were identified as maysin. Maysin [2″-O-α-L-rhamnosyl-6-C(-4-ketofucosyl)-luteolin] is the most abundant flavonoid glycoside reported in maize silks (Kim & Jung, Citation2001) and plays a key role in the natural resistance of silks to the maize ear worm (Snook et al., Citation1993). This flavone is synthesized via the same pathway that colored flavones in kernels and pollen (Meihls, Kaur, & Jander, Citation2012). Therefore, it is very likely to find more maysin in silks obtained from maize with colored kernels.
The concentrations of anthocyanins detected in the silks from maize races native to Mexico () were comparable to those reported for silks of purple, white and yellow hybrid maize cultivated in Thailand with 0.0539, 0.0011 and 0.0016 g C3G kg−1, respectively (Sarepoua et al., Citation2015). In a similar manner, Žilić et al. (Citation2016) found that purple silks from hybrid maize cultivated in Serbia contained 0.0149 g C3G kg−1. Silks from all the maize landraces with colored kernels (RC-r, RC-dr and RC-wb) had a high content of anthocyanins. Silks from white and yellow maizes (RG-w and RCr-Y) had in counterpart a small presence of anthocyanins (). In general, the concentration of anthocyanins observed in the silks of maize races native to Mexico was lower than reported for kernels of blue maizes from the landraces Chalqueño and Cónico (0.646 and 0.892 g C3G kg−1) (Urias-Lugo et al., Citation2015). This difference in the content of anthocyanins between silks and grains can be attributed to the migration of anthocyanins from the silks to kernels during pollination (Sarepoua et al., Citation2015).
3.4 Free radical scavenging capacity
The EC50 for vitamin C (control) was 2.46 μg mL−1 or 13.96 μM (), being comparable to the values of 11.5–11.8 μM described by other authors for ascorbic acid in methanol (Sharma & Bhat, Citation2009). The concentration of maize-silks extract required to scavenge 50% of DPPH free radicals (EC50) has been reported for silks of white hybrid maize. An EC50 of 163.45 μg mL−1 was estimated for a crude ethanolic extract obtained from silks of a hybrid maize variety cultivated in China (Liu, Wang, Wang, Zhang, & Lu, Citation2011). Whereas, silk extracts from white hybrid maize grown in Iran and Egypt were described with an EC50 of 590 and 1001 μg mL−1, respectively (Ebrahimzadeh et al., Citation2008; Eman, Citation2011). Similarly, purple, pink, green and yellow silks from maize cultivated in Serbia were reported with EC50 values ranging from 151 to 239 μg mL−1 (Žilić et al., Citation2016). The ethanolic extracts obtained from the silks of Mexican maize races had a wide range of EC50 values. The highest EC50 was observed in the silks from yellow-dented maize (1069 μg mL−1), while the silks of dark red maize cobs had the lowest EC50 value (51.76 μg mL−1).
Figure 3. DPPH radical scavenging capacity of maize silk extracts obtained from different Mexican maize races. Black lines represent the regression fits and the red lines the 95% confidence bands. RG-w = race Gordo with white kernels; RC-r = race Cónico with red kernels; RC-dr = race Cónico with dark red kernels; RC-wb = race Cónico with white and blue kernels; RCr-Y = race Cristalino with a white and yellow kernels.
Figura 3. Capacidad de captación del radical libre DPPH de los extractos de sedas de maíz obtenidos de diferentes razas de maíces mexicanos. Las líneas negras representan los ajustes de regresión y las líneas rojas las bandas del 95% de confianza. RG-w = raza Gordo con granos blancos; RC-r = raza Cónico con granos rojos; RC-dr = raza Cónico con granos rojo obscuro; RC-wb = raza Cónico con granos blancos y azules; RCr-Y = raza Cristalino con granos blancos y amarillos.
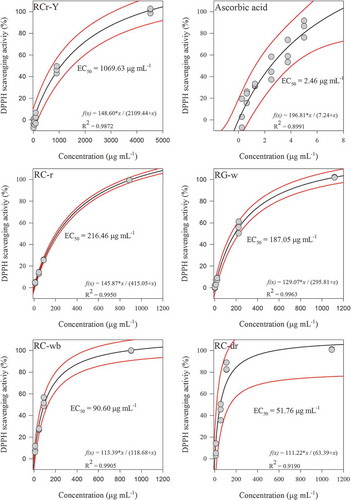
The antioxidant activity of maize-silks extracts is primarily attributed to the presence of phenolic compounds such as flavonoids and tannins (Liu et al., Citation2011; Maksimović, Malencić, & Kovacević, Citation2005). Whereas, the concentration of anthocyanins contributes to the antioxidant activity of maize-silks extracts but not significantly (Sarepoua, Tangwongchai, Suriharn, & Lertrat, Citation2013). In the present work, the green-yellow silks (RCr-Y) that had the lowest concentration of polyphenols, flavonoids, and anthocyanins () also showed the lowest antioxidant activity (highest EC50). Contradictorily, the red maize silks (RC-r) which had the highest polyphenol, flavonoid and anthocyanin content () did not exhibit the highest antioxidant activity (EC50 = 216 μg mL−1). Furthermore, the brown silks from white maize (RG-w) had a higher antioxidant activity than silks from red maize (), even with a concentration of polyphenols 10-fold lower (). These results may derive from the scarce content of polysaccharides in the brown silks and the remarkable high content of polysaccharides in the red and green-yellow silks (). Since it has been described that macromolecules such as polysaccharides strongly interact with phenolic compounds, binding non-covalently with the hydroxyl groups attached to the ring structure of phenolics (Le Bourvellec & Renard, Citation2012), which are mainly responsible for the antioxidant efficiency of flavonoids (Landete, Citation2012).
On the other hand, the dark red silks from the landrace Cónico with dark red kernels had by far the best antioxidant activity (51.76 μg mL−1). These results can be related to the presence of a specific flavonoid with a strong antioxidant activity such as maysin [2″-O-α-L-rhamnosyl-6-C-(deoxy-xylo-hexo-4-ulosyl)luteolin] and 3′-methoxymaysin. Due to these, flavones had a high capacity to scavenge DPPH free radicals, showing EC50 values of 22.69 and 43.71 μg mL−1 (Liu et al., Citation2011).
3.5 Principal component analysis
The multivariate analysis allowed to cluster the silks from different maize races native to Mexico by features such as antioxidant capacity, gross composition and minor constituents (). According to the PCA, all the silks from the landrace Cónico (RC-dr, RC-r and RC-wb) have similar characteristics notwithstanding the color of the silks. The main features of these silks were not only their high content of polyphenols, flavonoids, and anthocyanins but also their high content of sugars, soluble fiber and moisture. In contrast, the green-yellow silks from the race Cristalino had the lowest antioxidant activity and higher content of proteins. Finally, the brown silks from white maize (race Gordo) had an outstanding content of fiber, mostly insoluble, and the lowest content of polysaccharides.
Figure 4. Principal component analysis based on the compositional and antioxidant properties of maize silks obtained from maize races natives to Mexico. RG-w = race Gordo with white kernels; RC-r = race Cónico with red kernels; RC-dr = race Cónico with dark red kernels; RC-wb = race Cónico with white and blue kernels; RCr-Y = race Cristalino with a white and yellow kernels.
Figura 4. Análisis de componentes principales basado en las propiedades de composición y antioxidantes de las sedas de maíz obtenidas de maíces nativos de México. RG-w = raza Gordo con granos blancos; RC-r = raza Cónico con granos rojos; RC-dr = raza Cónico con granos rojo obscuro; RC-wb = raza Cónico con granos blancos y azules; RCr-Y = raza Cristalino con granos blancos y amarillos.
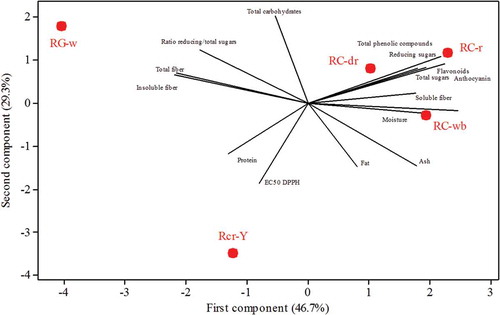
4. Conclusion
The content of polysaccharides and polyphenols in the maize silks differed significantly among the maize landraces tested. Some silks accumulated large amounts of polysaccharides like those obtained from the landrace Cristalino, which resembles those hybrid varieties of yellow sweet maize. Whereas, silks from the landrace Cónico had the highest antioxidant capacity and polyphenol content. The presence of the flavonoid maysin was confirmed by TLC in silks of the landrace Cónico but not in silks from the landraces Gordo and Cristalino. The beneficial effects of maize silks are attributed to either their polyphenol or polysaccharide content. Therefore, it is very important an adequate selection of maize silks according to their content of polysaccharides and polyphenols.
Acknowledgment
This project was supported by an internal grant from the Autonomous University of Chihuahua.
Disclosure statement
No potential conflict of interest was reported by the authors.
References
- AOAC International. (1998a). Ash of animal feed: 942.05 Official methods of analysis (16th ed., pp. 5). Gaithersburg, MD: AOAC International.
- AOAC International. (1998b). Fat (crude) in nuts and nuts products: 948.22 Official methods of analysis (16th ed., pp. 1). Gaithersburg, MD: AOAC International.
- AOAC International. (1998c). Microchemical determination of nitrogen: 960.52 Official methods of analysis (16th ed., pp. 7). Gaithersburg, MD: AOAC International.
- AOAC International. (1998d). Moisture in animal feed: 934.01 Official methods of analysis (16th ed.). Gaithersburg, MD: AOAC International.
- AOAC International. (1998e). Total, soluble and insoluble dietary fiber in foods (enzymatic-gravimetric method): 991.43 Official methods of analysis (16th ed., pp. 7). Gaithersburg, MD: AOAC International.
- Bedinger, P.A., & Fowler, J.E. (2009). The maize male gametophyte. In L.J. Bennetzen & C.S. Hake (Eds.), Handbook of maize: Its biology (pp. 57–77). New York, NY: Springer.
- Blainski, A., Lopes, G., & de Mello, J. (2013). Application and analysis of the Folin Ciocalteu method for the determination of the total phenolic content from Limonium brasiliense L. Molecules, 18(6), 6852–6865. doi:10.3390/molecules18066852
- Cha, J.H., Kim, S.R., Kang, H.J., Kim, M.H., Ha, A.W., & Kim, W.K. (2016). Corn silk extract improves cholesterol metabolism in C57BL/6J mouse fed high-fat diets. Nutrition Research and Practice, 10(5), 501–506. doi:10.4162/nrp.2016.10.5.501
- Chen, S., Chen, H., Tian, J., Wang, J., Wang, Y., & Xing, L. (2014). Enzymolysis-ultrasonic assisted extraction, chemical characteristics and bioactivities of polysaccharides from corn silk. Carbohydrate Polymers, 101, 332–341. doi:10.1016/j.carbpol.2013.09.046
- Choi, C.W., Kim, S.C., Hwang, S.S., Choi, B.K., Ahn, H.J., Lee, M.Y., … Kim, S.K. (2002). Antioxidant activity and free radical scavenging capacity between Korean medicinal plants and flavonoids by assay-guided comparison. Plant Science, 163, 1161–1168. doi:10.1016/S0168-9452(02)00332-1
- Chow, P.S., & Landhäusser, S.M. (2004). A method for routine measurements of total sugar and starch content in woody plant tissues. Tree Physiology, 24(10), 1129–1136. doi:10.1093/treephys/24.10.1129
- Dong, J., Cai, L., Zhu, X., Huang, X., Yin, T., Fang, H., & Ding, Z. (2014). Antioxidant activities and phenolic compounds of cornhusk, corncob and stigma maydis. Journal of the Brazilian Chemical Society, 25, 1956–1964.
- Doo Jin, C., Sun-Lim, K., Ji Won, C., & Yong, I.P. (2014). Neuroprotective effects of corn silk maysin via inhibition of H2O2-induced apoptotic cell death in SK-N-MC cells. Life Sciences, 109(1), 57–64. doi:10.1016/j.lfs.2014.05.020
- Ebrahimzadeh, M.A., Pourmorad, F., & Hafezı, S. (2008). Antioxidant activities of Iranian corn silk. Turkish Journal of Biology, 32(1), 43–49.
- Eman, A.A. (2011). Evaluation of antioxidant and antibacterial activities of Egyptian Maydis stigma (Zea mays hairs) rich in some bioactive constituents. Journal of American Science, 7, 726–729.
- Fenton, T.W., Leung, J., & Clandinin, D.R. (1980). Phenolic components of rapeseed meal. Journal of Food Science, 45(6), 1702–1705. doi:10.1111/j.1365-2621.1980.tb07592.x
- George, G.O., & Idu, F.K. (2015). Corn silk aqueous extracts and intraocular pressure of systemic and non-systemic hypertensive subjects. Clinical & Experimental Optometry, 98(2), 138–149. doi:10.1111/cxo.12240
- Glore, S.R., Van Treeck, D., Knehans, A.W., & Guild, M. (1994). Soluble fiber and serum lipids: A literature review. Journal of the Academy of Nutrition and Dietetics, 94(4), 425–436. doi:10.1016/0002-8223(94)90099-X
- Guo, J., Liu, T., Han, L., Liu, Y., & Gou, J. (2009). The effects of corn silk on glycaemic metabolism. Nutrition & Metabolism, 6, 47. doi:10.1186/1743-7075-6-47
- Hasanudin, K., Hashim, P., & Mustafa, S. (2012). Corn silk (Stigma Maydis) in healthcare: A phytochemical and pharmacological review. Molecules, 17, 9697–9715. doi:10.3390/molecules17089697
- Kan, A., Orhan, I., Coksari, G., & Sener, B. (2012). In-vitro neuroprotective properties of the Maydis stigma extracts from four corn varieties. [Article]. International Journal of Food Sciences & Nutrition, 63(1), 1–4. doi:10.3109/09637486.2011.590797
- Karami, M., Shokerzadeh, M., Naghshvar, F., Ala, S., Fezbakhsh, R., Anahita Nosrati, A., & Makhloogh, M. (2013). An experimental model for study of the renal protective activity of corn silk against dosage induced by MDMA using in situ rat renal system. Research Opinions in Animal & Veterinary Sciences, 3(5), 143–148.
- Kato-Yamakake, T.Á., Mapes-Sánchez, C., Mera-Ovando, L.M., Serratos-Hernández, J.A., & Bye-Boettler, R.A. (Eds.). (2009). El origen y diversificación del maíz: Una revisión analítica. México, DF: Universidad Nacional Autónoma de México, Comisión Nacional para el Conocimiento y Uso de la Biodiversidad (CONABIO).
- Kim, S.L., & Jung, T.W. (2001). Maysin and other flavonoid contents in corn silks. Korean Journal Breeder, 33(4), 338–343.
- Krivorotova, T., & Sereikaite, J. (2014). Determination of fructan exohydrolase activity in the crude extracts of plants. Electronic Journal of Biotechnology, 17(6), 329–333. doi:10.1016/j.ejbt.2014.09.005
- Landete, J.M. (2012). Updated knowledge about polyphenols: Functions, bioavailability, metabolism, and health. Critical Reviews in Food Science and Nutrition, 52(10), 936–948. doi:10.1080/10408398.2010.513779
- Le Bourvellec, C., & Renard, C.M. (2012). Interactions between polyphenols and macromolecules: Quantification methods and mechanisms. Critical Reviews in Food Science and Nutrition, 52(3), 213–248. doi:10.1080/10408398.2010.499808
- Lee, E., Kim, S., Kang, H., Kim, M., Ha, A., & Kim, W. (2016). High maysin corn silk extract reduces body weight and fat deposition in C57BL/6J mice fed high-fat diets. Nutrition Research and Practice, 10(6), 575–582. doi:10.4162/nrp.2016.10.6.575
- Lee, J. (2005). Determination of total monomeric anthocyanin pigment content of fruit juices, beverages, natural colorants, and wines by the pH differential method: Collaborative study. Journal of AOAC International, 88(5), 1269–1278.
- Liu, J., Wang, C., Wang, Z., Zhang, C., & Lu, S. (2011). The antioxidant and free-radical scavenging activities of extract and fractions from corn silk (Zea mays L.) and related flavone glycosides. Food Chemistry, 126, 261–269. doi:10.1016/j.foodchem.2010.11.014
- Maksimović, Z., Malencić, D., & Kovacević, N. (2005). Polyphenol contents and antioxidant activity of Maydis stigma extracts. Bioresource Technology, 96, 873–877. doi:10.1016/j.biortech.2004.09.006
- Marston, A., & Hostettmann, K. (2005). Separation and quantification of flavonoids. In M.A. Oyvind & T.W. Keenan (Eds.), Flavonoids: Chemistry, biochemistry and applications (pp. 1–36). Boca Raton, FL: CRC Press Taylor and Francis Group.
- Meihls, L., Kaur, H., & Jander, G. (2012). Natural variation in maize defense against insect herbivores. Paper presented at the Cold Spring Harbor symposia on quantitative biology. doi:10.1101/sqb.2012.77.014662
- Sánchez-Moreno, C., Larrauri, A.J., & Saura-Calixto, F. (1998). A procedure to measure the antiradical efficiency of polyphenols. Journal of the Science of Food and Agriculture, 76, 270–276. doi:10.1002/(SICI)1097-0010(199802)76:2<270::AID-JSFA945>3.0.CO;2-9
- Sarepoua, E., Tangwongchai, R., Suriharn, B., & Lertrat, K. (2013). Relationships between phytochemicals and antioxidant activity in corn silk. International Food Research Journal, 20(5), 2073–2079.
- Sarepoua, E., Tangwongchai, R., Suriharn, B., & Lertrat, K. (2015). Influence of variety and harvest maturity on phytochemical content in corn silk. [Article]. Food Chemistry, 169, 424–429. doi:10.1016/j.foodchem.2014.07.136
- Scanlon, M.J., & Takacs, E.M. (2009). Kernel Biology. In L.J. Bennetzen & C.S. Hake (Eds.), Handbook of maize: Its biology (pp. 121–143). New York, NY: Springer.
- Shahzadi, H., Sheikh, M.A., Hameed, A., Jamil, A., & Ur-Rehman, S. (2015). Comparative antioxidant potential and bioactivity of maize (Zea mays) ear tissues from different genotypes. [Article]. International Journal of Agriculture & Biology, 17(3), 539–546. doi:10.17957/IJAB/17.3.14.572
- Sharma, O.P., & Bhat, T.K. (2009). DPPH antioxidant assay revisited. Food Chemistry, 113(4), 1202–1205. doi:10.1016/j.foodchem.2008.08.008
- Snook, M.E., Gueldner, R.C., Widstrom, N.W., Wiseman, B.R., Himmelsbach, D.S., Harwood, J.S., & Costello, C.E. (1993). Levels of maysin and maysin analogs in silks of maize germplasm. Journal of Agricultural and Food Chemistry, 41(9), 1481–1485. doi:10.1021/jf00033a024
- Šukalović, V.H.-T., Veljović-Jovanović, S., Maksimović, J.D., Maksimović, V., & Pajić, Z. (2010). Characterisation of phenol oxidase and peroxidase from maize silk. Plant Biology, 12(3), 406–413. doi:10.1111/j.1438-8677.2009.00237.x
- Sukandar, E.Y., Sigit, J.I., & Adiwibowo, L.F. (2013). Study of kidney repair mechanisms of corn silk (Zea mays L. hair)-Binahong (Anredera cordifolia (Ten.) steenis) leaves combination in rat model of kidney failure. International Journal of Pharmacology, 9, 12–23. doi:10.3923/ijp.2013.12.23
- Takeoka, G., & Dao, L. (2002). Anthocyanins. In W.J. Hurst (Ed.), Methods of analysis for functional foods and nutraceuticals (pp. 224–246). Boca Raton, FL: CRC Press.
- Urias-Lugo, D.A., Heredia, J.B., Serna-Saldivar, S.O., Muy-Rangel, M.D., & Valdez-Torres, J.B. (2015). Total phenolics, total anthocyanins and antioxidant capacity of native and elite blue maize hybrids (Zea mays L.). Cyta - Journal of Food, 13(3), 336–339. doi:10.1080/19476337.2014.980324
- Vielle-Calzada, J.P., & Padilla, J. (2009). The Mexican landraces: Description, classification and diversity. In L.J. Bennetzen & C.S. Hake (Eds.), Handbook of maize: Its biology (pp. 543–561). New York, NY: Springer.
- Vollbrecht, E., & Schmidt, R.J. (2009). Development of the inflorescences. In L.J. Bennetzen & C.S. Hake (Eds.), Handbook of maize: Its biology (pp. 13–40). New York, NY: Springer.
- Zhao, W., Yin, Y., Yu, Z., Liu, J., & Chen, F. (2012). Comparison of anti-diabetic effects of polysaccharides from corn silk on normal and hyperglycemia rats. International Journal of Biological Macromolecules, 50, 1133–1137. doi:10.1016/j.ijbiomac.2012.02.004
- Žilić, S., Janković, M., Basić, Z., Vančetović, J., & Maksimović, V. (2016). Antioxidant activity, phenolic profile, chlorophyll and mineral matter content of corn silk (Zea mays L): Comparison with medicinal herbs. Journal of Cereal Science, 69, 363–370. doi:10.1016/j.jcs.2016.05.003