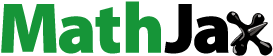
ABSTRACT
This study aimed at producing functional soymilk by optimizing the sprouting conditions of soybeans using response surface methodology. Soaking (12–24 h) and germination times (48–96 h) were optimized using central complete randomized design. Responses obtained from experimental runs were fitted into second order polynomial regression model. Significance of model parameters was tested using ANOVA and R2 was evaluated. The optimum sprouting conditions of soybeans were 12 h soaking and 52 h germination using desirability concept. Soymilk made from optimized conditions had 17% increase in total proteins, 50% reduction in phytic acid, 1.7% increase in total phenolics and a color change (∆E) of 4.89 compared with the control. There was a significant reduction in trypsin inhibitor activity (0.03 mg/g TI), with increase in total amino acids and similar rheological properties in optimized soymilk. Optimized conditions obtained are adequate in the production of soymilk with improved nutritional and quality attributes.
RESUMEN
El presente estudio se propuso producir leche de soya funcional a partir de la optimización de las condiciones de germinado de la soya, utilizando la metodología de superficies de respuesta. Con este objetivo se optimizaron la duración del remojo (12–24 h) y de la germinación (48–96 h) usando el diseño central completo aleatorizado. Las respuestas obtenidas en las pruebas experimentales fueron ajustadas al modelo de regresión polinomial de segundo orden. La significancia de los parámetros del modelo fue sometida a prueba, empleando ANOVA y evaluando R2. Partiendo del concepto de deseabilidad se determinó que las condiciones óptimas para el germinado de la soya son 12 h de remojo y 52 h de germinación. Se constató que en la leche de soya preparada bajo estas condiciones optimizadas se registró un aumento de 17% de proteínas totales, una reducción de 50% de ácido fítico, un aumento de 17% de fenólicos totales y un cambio de color de 4.89 en comparación con el control. Además, en la leche de soya optimizada se detectó una reducción significativa de la actividad inhibidora de la tripsina (0.03 mg/g TI), presentándose un incremento en los aminoácidos totales y que la misma exhibe propiedades reológicas similares. Las condiciones optimizadas logradas resultan adecuadas para la producción de leche de soya con atributos nutricionales y de calidad mejorados.
Introduction
Soybean (Glycine max) is an important plant source of major and trace nutrients, such as proteins, fatty acids, phytosterols and phenolic acids. The consumption of soybean has been found to help in preventing and combating some disease conditions including cancer, osteoporosis and cardiovascular diseases (Kim, Yang, & Chung, Citation2016). Soybean is cheap, accessible and easy to process into many fermented and non-fermented products, such as soymilk. Soymilk, an important product from soybean, is made by grinding and expression of the milk component. Soymilk has been found to be a suitable alternative for milk from animal sources, especially for vegetarians and lactose intolerants (Jiang, Cai, & Xu, Citation2013). It can be consumed directly as food and could also serve as an intermediate product in the production of other fermented and non-fermented soy products such as tofu and soy yogurt.
Sprouting of soybeans before production of soy-based products, as a means of improving the functionality of the resulting foods, has been explored (Jiang et al., Citation2013; Murugkar, Citation2014; Paucar-Menacho, Berhow, Mandarino, De Mejia, & Chang, Citation2010). Subjecting soybeans to sprouting, prior to processing into a desired product, is a cheap and effective pretreatment aimed at stimulating metabolic activities. It helps in the breakdown of complex components in the seeds into simpler, absorbable forms, hence improving nutritional quality, digestibility and overall functionality (Fernandez-Orozco et al., Citation2008). The quality of sprouted grain may be influenced by many factors including the soaking time, germination time, temperature and variety of the grain (Huang, Cai, & Xu, Citation2014; Jiang et al., Citation2013; Murugkar, Citation2014; Paucar-Menacho et al., Citation2010). Jiang et al. (Citation2013) studied the effect of varying germination time (28–72 h) of soybeans on the quality of soymilk and reported that 28 h germination resulted in the highest increase in protein and total phenolic contents. These authors also suggested that the soymilk prepared from sprouted soybean had comparable physicochemical properties to traditional soymilk. Huang et al. (Citation2014) studied the kinetics of changes in the nutrient and antioxidant capacities of soybeans at extended germination times (24–120 h). Germination for about 72–96 h was found to improve the nutrient contents of the soybean. Other optimization studies on the bioactive components in soybean reported that 45 h germination time was optimum for the production of lunasin, and reduction of lectin and lipoxygenase activities (Paucar-Menacho et al., Citation2010).
Soaking, as a factor of germination, enhances the softening of seed-coats, emergence of sprouts and reduction of antinutritional factors, such as phytates (Lestienne, Mouquet‐Rivier, Icard‐Vernière, Rochette, & Treche, Citation2005). However, soaking conditions required for sprouting of legumes, especially in combination with other factors, have been sparsely studied. As different germination times for desirable results were suggested by the previous authors, there is a need to optimize the soaking and germination times on the resulting quality attributes of soymilk. The aim of this study is to employ response surface methodology in optimizing the sprouting conditions (soaking and germination time) in the production of functional soymilk from South African soybeans, with improved nutritional and quality attributes, as an intermediate product for the production of other soy-based foods.
Materials and methods
Experimental materials
Soybeans grown in South Africa (variety: DM 5.1i RR) was obtained from Agricol, KwaZulu Natal branch. Seeds were manually sorted to remove defective ones. All chemicals and solvents used were laboratory grade.
Experimental design for sprouting conditions
The factors of sprouting studied were sprouting time and germination time. The effect of variations in these factors on some quality attributes of the resulting soymilk from each experimental run was determined using a two-factor central composite rotation design. The centres of rotation were chosen to be 5, thereby making α = ±1.414. Thirteen experimental runs were generated from the combination of factors. The experimental conditions are as presented in .
Table 1. Experimental conditions for optimization of sprouting conditions (soaking and germination times).
Tabla 1. Condiciones experimentales para la optimización de las condiciones de germinado (duración de remojo y germinación).
Sprouting of soybean seeds
For each of the experimental runs, 100 g of sound seeds were thoroughly washed with distilled water and rinsed thereafter. Sprouting of soybean seeds was carried out in the dark in a growth chamber (Hotpack 352643, Warminster, PA) and the temperature within the chamber was maintained at 25 ± 2°C. Samples were consistently rinsed and kept humid by manual wetting at 3–4 h interval throughout the germination period for each experimental run.
Preparation of soymilk from sprouted seeds
Sprouted soybeans were manually dehulled to obtain seeds and cotyledons. Distilled water (1000 ml) was added to the mixture (to obtain 1:10 w/v soybean/water ratio) and blended using a laboratory blender (Kenwood BL 380, China) until fine consistency was obtained. Raw soymilk was obtained by sieving through muslin cloth and the residue was discarded. Thereafter, the raw milk was cooked at 100°C for about 10 min and the milk was allowed to cool at ambient temperature before storing in the refrigerator at 4°C for further analyses. Soymilk produced from unsprouted seeds soaked for 12 h using the same procedure described above served as the control.
Analyses
Total protein and total solids
Total protein content of soymilk samples was determined using Kjeldahl method with the conversion factor of 6.25. Also, total solids were obtained by placing 5 g of sample into pre-weighed crucibles and then dried in a hot air oven at 105°C for 3 h. The dry matter was weighed and used to calculate the percentage total solids (Liu & Chang, Citation2013).
Determination of color parameters
The lightness, redness (or greenness) and yellowness color attributes (L*, a* and b* respectively) of each soymilk samples obtained from sprouted soybeans and control were determined using the ColourFlex EZ benchtop spectrophotometer (HunterLab, U.S.A.). Liquid samples were poured into the sample cup to fill the entire base and was covered with the lid, before the parameters were measured. The change in color (ΔE) between the optimized sprouted soymilk and the control was also determined using the equation:
Total phenolic content
The method described by B. Xu and Chang (Citation2007) was used to determine total phenolic content of the samples. Soymilk samples were frozen at −80°C for 24 h and then lyophilized in an Alpha 2–4 LDplus freeze drier (Christ, Germany) set at 1 atm and −80°C. Phenolic compounds were extracted from 0.3 g of freeze-dried samples of soymilk with a mixture of acetone, acetic acid and water, referred to as acidic acetone (Jiang et al., Citation2013) in the ratio 70:0.5:29.5. Gallic acid calibration curve was used to obtain the total phenolic content and the results were expressed in mg GAE/g of sample.
Total phytic acid content
Wade reagent was used in the determination of phytic acid from freeze dried soymilk samples. The reagent was prepared by the addition of 0.03% FeCl3ˑ6H2O and 0.3% sulfocalicyclic acid. 2.4% HCl solution was mixed with 0.3 g freeze dried samples and was shaken in an orbital shaker at 220 rpm for 6 h. Thereafter, 0.1 ml of clear supernatant was mixed with 3 ml water and 0.2 ml Wade reagent before centrifugation at 5500 rpm for 10 min. The absorbance was measured with distilled water as blank at 500 nm and the results were expressed as mg/g phytic acid/freeze-dried soymilk (Gao et al., Citation2007)
Analyses of optimized soymilk and the control
Trypsin inhibitor activity
The method of Hamerstrand, Black, and Glover (Citation1981) as illustrated by Poliseli-Scopel, Hernández-Herrero, Guamis, and Ferragut (Citation2012) was used to determine the trypsin inhibitor activity of optimized soymilk and the control. Tris buffer, Nα-Benzoyl-L-arginine 4-nitroanilide hydrochloride (L-BAPA) and trypsin solutions were prepared in line with the methods described and the samples were extracted with 0.01 N HCl solution by shaking in orbital shaker. Absorbance was measured at 410 nm against sample blanks and trypsin inhibitor content was determined.
Rheology
Viscosity and other rheological properties of soymilk were measured according to the modified method of Oyeyinka, Singh, Adebola, Gerrano, and Amonsou (Citation2015). Briefly, samples were allowed to equilibrate at 25°C for about 10 min in the sample cup of a Rheometer (Rheolab 80732808, Anton Paar, Austria). Data generated at shear rates between 750 and 1500 s−1 were fitted into the Power law equation and the rheological properties were determined as follows:
where ,
,
and
are shear stress (Pa), consistency coefficient (Pa・s)n shear rate (s−1) and flow behavior index, respectively.
Amino acid content determination
Amino acid contents were determined using the method described by Grobbelaar, Makunga, Stander, Kossmann, and Hills (Citation2014) with slight modifications. Amino acid separation and detection was performed using a Waters Acquity Ultra Performance Liquid Chromatograph (UPLC) fitted with a photodiode array (PDA) detector. Derivatization was performed using Waters AccQ Tag Ultra Derivatization kit, according to the manufacturer’s guide. Sample/standard solution (1 µl) was injected into the mobile phase (AccQ-Tag Ultra Eluent A and B (Waters)), which conveys the derivatized amino acids onto a Waters UltraTag C18 column (2.1 × 50 mm ×1.7 µm) held at 60°C.
Optimization of responses
As explained by Akinpelu et al. (Citation2014), optimization of the processing parameters was achieved using numerical optimization technique, which was aimed at simultaneous combination of parameters to find a point that maximizes the desirability functions. These desirable qualities in the resulting soymilk include the highest possible total solids, total proteins, phenolic acids and lightness and the lowest redness, yellowness and phytic acid contents. Optimized sprouting conditions (soaking and germination times) were derived from the values of responses obtained from each experimental run. Since the characteristics of each desired goal can be altered by adjusting the importance of each of the responses, maximization (for total solids, total proteins, total phenolic acid and lightness) and minimization (for redness, yellowness and phytic acid content) were preset to obtain the desired outcomes. Also, 3-dimensional response surface plots were generated to show the effects of processing parameters on the responses, with respect to the preset optimization conditions.
Statistical analyses
Design expert 10.0 software (StatEase Inc., U.S.A.) was used to generate the experimental design, with the two-independent variables; soaking time (X1) and germination time (X2). Data obtained from responses were fitted into a second order polynomial equation as shown below:
where Y is the response variable, X1 and X2 are process variables and are coefficients of regression.
Response surface methodology was used to determine the optimum sprouting conditions by using the concept of desirability and choosing the solution that best turns in the desirable levels, after the responses have either been minimized (redness, yellowness and phytic acid) or maximized (total solids, total protein, lightness and total phenolic content). Analyses of variance (ANOVA) was used to check the significant differences in responses (P < 0.05) determined in triplicates and to examine the statistical significance of the regression equation used.
Results and discussion
Total solids and protein contents
The total solids contents of soymilk samples obtained from sprouted beans ranged between 2.88 and 4.43 g/100 g (). Response surface plot showed that the total solid contents decreased as soaking and germination time increased (). The reduction during germination could be attributed to loss of nutrient reserves, especially at extended germination times. This seems plausible since sprouting as a metabolic process involves the breakdown of nutrients, which is accompanied with the outgrowth of cotyledons and the release of energy (Murugkar, Citation2014). Retention of adequate nutrients reserves in the form of total solids in soymilk is of critical importance to the quality of soy-based foods produced from it (Denkova & Murgov, Citation2005).
Table 2. Results of analysis of responses of sprouted soymilk for different experimental runs.
Tabla 2. Resultados del análisis de respuestas de la leche de soya germinada, obtenidos a partir de distintas pruebas experimentales.
Figure 1. Response surface plots for sprouting conditions of soybeans (a) total solids; (b) total protein; (c) lightness; (d) rightness; (e) yellowness; (f) total phenolics; (g) total phytic acid.
Figura 1. Gráficas de superficies de respuesta para las condiciones de germinado de soya (a) Sólidos totales; (b) Proteína total; (c) Claridad; (d) Exactitud [rightness]; (e) Amarillez; (f) Fenólicos totales; (g) Ácido fítico total.
![Figure 1. Response surface plots for sprouting conditions of soybeans (a) total solids; (b) total protein; (c) lightness; (d) rightness; (e) yellowness; (f) total phenolics; (g) total phytic acid.Figura 1. Gráficas de superficies de respuesta para las condiciones de germinado de soya (a) Sólidos totales; (b) Proteína total; (c) Claridad; (d) Exactitud [rightness]; (e) Amarillez; (f) Fenólicos totales; (g) Ácido fítico total.](/cms/asset/847f14b7-ba1b-4ce2-b11c-fe635a6fae3c/tcyt_a_1388292_f0001_c.jpg)
Slight increase in the total protein contents (1.24–2.44 g/100 g) () on a wet basis was recorded with increase in soaking and germination time (). Sprouting triggers the production of diverse enzymes, which could either be responsible for the biosynthesis of proteins, or breakdown of complex proteins. Previous studies similarly reported slight increase in the protein contents of sprouted soybeans (Jiang et al., Citation2013) and buckwheat for up to 72 h germination (Zhang et al., Citation2015). However, other authors working with soybean germinated for 48 h reported a decrease in protein content of the sprouted grains (Radzi, Rusydi, & Azlan, Citation2012). Hence, the protein contents of sprouted grains may be influenced by the grain variety and germination conditions. The types of enzymes produced during germination and their effect on the residual proteins of the legume may also influence the changes in protein contents of sprouted grains (Zhang et al., Citation2015).
Color
The color of soymilk as measured by lightness, redness and yellowness ranged between 79.81 and 83.58, −1.83 and −2.82 and 9.03 and 12.76, respectively (), with their response plots presented in (c–e), respectively. The least change in color attributes, with respect to the control was observed at lower soaking and germination times. This could be attributed to lower metabolic stress during the breakdown of carbohydrate components of sprouting seeds into reducing sugars. Earlier studies indicated that these sugars may result in off-color development especially at extended sprouting times (Charoenthaikij et al., Citation2009). The inclusion of cotyledons and sprouted seeds in the production of soymilk may also be implicated for color loss. However, minimal color change (∆E) was observed when the optimized soymilk was compared with the control, suggesting similar appearance of optimized soymilk to the control. Similar minimal ∆E values between soymilk and tofu from sprouted and unsprouted sources had earlier been reported (Murugkar, Citation2014).
Total phenolic and phytic acid contents
Total phenolic contents of soymilk samples varied between 12.93 and 14.07 mg GAE/g (). The phenolic contents of the samples increased with soaking and germination times () and could be due to the response of the sprouted grains to the stress induced during germination. The increase observed in soymilk produced from sprouted soybeans, as compared with the control could be seen as an advantage. Phenolic compounds have been found to exhibit bioactive properties, which may help in reducing the incidence of chronic ailments such as cancer and cardiovascular diseases (Jooyandeh, Citation2011). Phenolic compounds are synthesized and accumulated in sprouting legumes in response to the stress induced during germination, especially in the dark (Randhir, Lin, & Shetty, Citation2004).
Phytic acid contents of soymilk produced from sprouted soybeans for all experimental runs (0.19–0.23 mg/g) ( and ) were low compared with the control (0.41 mg/g). Soaking has been found to reduce the phytic acid content of legumes due to leaching into soak water (Liang, Han, Nout, & Hamer, Citation2009). The reduction in phytic acid could also be attributed to the activity of phytase released during sprouting (Murugkar, Citation2014). According to Dave, Yadav, and Tarafdar (Citation2008), phytase activity may be up to 5-fold in sprouted soybean compared with the unsprouted ones. Furthermore, the cooking process involved in the production of soymilk could also contribute to the overall reduction in its phytic acid contents. For instance, the phytic acid content of kidney beans reportedly reduced by 61% after cooking (Fabbri & Crosby, Citation2016; Shimelis & Rakshit, Citation2007). Phytic acid binds with essential minerals such as zinc, calcium and iron to form complexes, making them biologically unavailable from foods. Although phytic acid had recently been found to be a potent anti-cancer agent, they should be consumed at very low doses (Jiang et al., Citation2013; Jooyandeh, Citation2011).
Model description
The responses from different experimental runs and the mean values of total solids, total protein color, total phenolics and phytic acid contents of soymilk varied significantly (). The estimated values of parameters obtained for the second order polynomial model are presented in . Optimization of the dependent responses was carried out to obtain the best conditions for sprouting soybeans which will be used produce to produce functional soymilk that will serve as suitable intermediate for other soy-based products. Response plots are used to show the effect of processing parameters on responses (Chakraborty, Sain, Kortschot, & Ghosh, Citation2007). The effect of soaking and germination time on the responses investigated are shown in the individual 3 dimensional plots (). The significance of the model parameters was tested using ANOVA and the results are as stated in . Most parameters are significant (p < 0.05) for all the responses evaluated. The coefficient of determination, R2, which is a measure reduction in response variability using repressor variables in the model equation (Akinpelu et al., Citation2014; Esan, Sobukola, Sanni, Bakare, & Munoz, Citation2015) ranged between 0.59 and 0.91. This suggests a considerable fit of responses into the second order polynomial equation used.
Table 3. Significance of model parameters with corresponding coefficients of determination.
Tabla 3. Significado de los parámetros del modelo con los correspondientes coeficientes de determinación.
Optimized conditions for soymilk production
Numerical optimization of sprouting conditions with respect to the preset conditions of responses produced seven results and the optimum solution of responses from the result with the highest value for desirability function was selected. The selected optimized sprouting conditions were 12 h of soaking and 52 h of germination (in the dark). Optimum responses of the measured quality attributes for soymilk prepared from sprouted soybean gave total solids of 3.83 g/100 g (wet basis), total proteins of 2.40 g/100 g (wet basis), 80.30 lightness, 2.61 redness, 9.4 yellowness, 13.21 mg GAE/g total phenolic contents and 0.21 mg/g phytic acid. The values obtained for soymilk prepared from unsprouted beans were 5.07 g/100 g, total solids 2.06 g/100 g total proteins (wet basis), 84.05 lightness, −1.78 redness, 12.48 yellowness, 13.01 mg GAE/g total phenolic contents and 0.41 mg/g phytic acid. The overall change in color (∆E) of optimized sprouted soymilk, as compared to the control was 4.89. To adequately understand the effect of optimization on the sprouting parameters, soymilk from optimized sprouting conditions of seeds and the control were compared by determining their trypsin inhibitor activities, rheological properties and amino acid contents.
Comparison of soymilk from sprouted (at optimized conditions) and unsprouted (control) soybeans
Rheology
The apparent viscosity of the optimized and control increased as the shear rate increased, suggesting a shear-thickening behavior with n > 1 (). Our result contradicts earlier report where the viscosity of soymilk prepared from sprouted soybean (at 1:6 soybean-water ratio) decreased with increase in shear rate (Zhang et al., Citation2015). This difference in rheological behavior could be attributed to the soybean–water ratio used in this study (1:10). To characterize the flow properties of the soymilk samples, the power law model was used. Experimental data showed a good fit to the model with determination coefficients (R2) = 0.99 (). The power law model constants were similar for sprouted sample and the control suggesting that sprouting did not significantly affect the viscosity () of optimized soymilk.
Table 4. Power law coefficients for control and optimized soymilk.
Tabla 4. Coeficientes de la ley de potencias para la leche de soya de control y optimizada.
Trypsin inhibitor activity
The trypsin inhibitor activity of sprouted soymilk was significantly lower (0.03 mg/g TI) than the control (0.08 mg/g TI). The massive reduction in sprouted soymilk samples could be as a result of the presence of higher amounts of Kunitz trypsin inhibitors. These inhibitors are unstable and incorporated into protein aggregates at cooking temperatures compared to the Bowman–Birk trypsin inhibitors which are more heat stable (Z. Xu, Chen, Zhang, Kong, & Hua, Citation2012). Furthermore, the decrease in trypsin inhibition upon germination has been attributed to the synthesis of amino acids from the hydrolytic break-down of trysin inhibitors, to support the growth of sprouts (Sugawara, Ito, Akita, Oguri, & Momonoki, Citation2007). This may account for the lower values obtained for soymilk made from optimized sprouted soybeans. Jiang et al. (Citation2013) also observed that up to 91% reduction in trypsin inhibitor activity occurred between 28 and 72 h of germination.
Amino acid contents
Soymilk prepared from sprouted soybeans had higher total sum of amino acids than soymilk made from the control. Eighteen amino acids were detected, with lower values for sulfur-containing amino acids when compared to others (). Yang, Fu, and Li (Citation2012) similarly reported low values for sulfur-containing amino acids in yoghurts made from sprouted soybeans. Although there was slight reduction in the individual contents of serine, threonine, lysine, phenylalanine and methionine after sprouting, the reduction was not significant (P < 0.05). Reduction in amino acid contents after sprouting had previously been reported (Fouad & Rehab, Citation2015; Kuo, Rozan, Lambein, Frias, & Vidal-Valverde, Citation2004; Sulieman, Eltayeb, Babiker, Mustafa, & El Tinay, Citation2008; Yang et al., Citation2012). The changes observed in the amino acid contents of sprouted legumes during the first 72 h of sprouting had been attributed to hydrolysis, synthesis and rearrangement of protein moieties (Taraseviciene, Danilcenko, Jariene, Paulauskiene, & Gajewski, Citation2009). There is competition for the synthesized amino acids as energy source for germinating seeds, and this may explain the reduction in some of the amino acids (Fouad & Rehab, Citation2015; Taraseviciene et al., Citation2009). Thus, extended germination times may lead to depletion of amino acids due to extended sprout growth. The optimized sprouting conditions obtained could ensure a considerable retention of amino acids in soymilk, which makes it a suitable intermediate for other non-fermented soy-based products. Furthermore, the observed increase in some individual amino acid may be advantageous for improved microbial activities during the production of fermented products such as fermented soymilk and sufu.
Table 5. Amino acid composition of control (unsprouted) and optimized (sprouted) soymilk (µg/g).
Tabla 5. Composición de los aminoácidos de la leche de soya de control (sin germinar) y optimizada (germinada) (µg/g).
Conclusions
Process optimization of soybeans sprouting using response surface methodology showed that the optimum soaking and germination time was 12 h and 52 h. Soymilk produced from sprouted soybeans at these optimized conditions displayed improved nutritional attributes (protein, phenolic acid, total amino acids), reduced anti-nutrient activity (trypsin inhibitors and phytic acid) and compared favorably in quality attributes (color and rheology) with that which is made from unsprouted beans (control). Soymilk made from the optimized sprouting conditions of beans could be a nutritious alternative milk from plant source and an important intermediate raw material in the production of other fermented or non-fermented soy-based products.
Acknowledgment
This work is based on the research supported in part by the National Research Foundation, South Africa for the grant, Unique Grant no. 98687.
Disclosure statement
No potential conflict of interest was reported by the authors.
Additional information
Funding
References
- Akinpelu, O. R., Idowu, M. A., Sobukola, O. P., Henshaw, F., Sanni, S. A., Bodunde, G., … Munoz, L. (2014). Optimization of processing conditions for vacuum frying of high quality fried plantain chips using response surface methodology (RSM). Food Science and Biotechnology, 23(4), 1121–1128.
- Chakraborty, A., Sain, M. M., Kortschot, M. T., & Ghosh, S. B. (2007). Modeling energy consumption for the generation of microfibres from bleached kraft pulp fibres in a PFI mill. BioResources, 2(2), 210–222.
- Charoenthaikij, P., Jangchud, K., Jangchud, A., Piyachomkwan, K., Tungtrakul, P., & Prinyawiwatkul, W. (2009). Germination conditions affect physicochemical properties of germinated brown rice flour. Journal of Food Science, 74(9), C658–C665.
- Dave, S., Yadav, B., & Tarafdar, J. (2008). Phytate phosphorus and mineral changes during soaking, boiling and germination of legumes and pearl millet. Journal of Food Science and Technology -Mysore, 45(4), 344–348.
- Denkova, Z., & Murgov, I. (2005). Soy milk yoghurt. Biotechnology & Biotechnological Equipment, 19(1), 193–195.
- Esan, T., Sobukola, O., Sanni, L., Bakare, H., & Munoz, L. (2015). Process optimization by response surface methodology and quality attributes of vacuum fried yellow fleshed sweetpotato (Ipomoea batatas L.) chips. Food and Bioproducts Processing, 95, 27–37.
- Fabbri, A. D., & Crosby, G. A. (2016). A review of the impact of preparation and cooking on the nutritional quality of vegetables and legumes. International Journal of Gastronomy and Food Science, 3, 2–11.
- Fernandez-Orozco, R., Frias, J., Zielinski, H., Piskula, M. K., Kozlowska, H., & Vidal-Valverde, C. (2008). Kinetic study of the antioxidant compounds and antioxidant capacity during germination of Vigna radiata cv. emmerald, Glycinemax cv. jutro and Glycine max cv. merit. Food Chemistry, 111(3), 622–630.
- Fouad, A. A., & Rehab, F. (2015). Effect of germination time on proximate analysis, bioactive compounds and antioxidant activity of lentil (Lens culinaris Medik.) sprouts. Acta Scientiarum Polonorum Technologia Alimentaria, 14(3), 233–246. doi:10.17306/J.AFS
- Gao, Y., Shang, C., Maroof, M., Biyashev, R., Grabau, E., Kwanyuen, P., … Buss, G. R. (2007). A modified colorimetric method for phytic acid analysis in soybean. Crop Science, 47(5), 1797–1803.
- Grobbelaar, M. C., Makunga, N. P., Stander, M. A., Kossmann, J., & Hills, P. N. (2014). Effect of strigolactones and auxins on growth and metabolite content of Sutherlandia frutescens (L.) R. Br. microplants in vitro. Plant Cell, Tissue and Organ Culture (PCTOC), 117(3), 401–409.
- Hamerstrand, G., Black, L., & Glover, J. (1981). Trypsin inhibitors in soy products: Modification of the standard analytical procedure. Cereal Chemistry, 58(1), 42-45.
- Huang, X., Cai, W., & Xu, B. (2014). Kinetic changes of nutrients and antioxidant capacities of germinated soybean (Glycine max L.) and mung bean (Vigna radiata L.) with germination time. Food Chemistry, 143, 268–276.
- Jiang, S., Cai, W., & Xu, B. (2013). Food quality improvement of soy milk made from short-time germinated soybeans. Foods, 2(2), 198–212.
- Jooyandeh, H. (2011). Soy products as healthy and functional foods. Middle-East Journal of Scientific Research, 7(1), 71–80.
- Kim, S.-H., Yang, Y.-S., & Chung, I.-M. (2016). Effect of acetic acid treatment on isoflavones and carbohydrates in pickled soybean. Food Research International, 81, 58–65.
- Kuo, Y.-H., Rozan, P., Lambein, F., Frias, J., & Vidal-Valverde, C. (2004). Effects of different germination conditions on the contents of free protein and non-protein amino acids of commercial legumes. Food Chemistry, 86(4), 537–545.
- Lestienne, I., Mouquet‐Rivier, C., Icard‐Vernière, C., Rochette, I., & Treche, S. (2005). The effects of soaking of whole, dehulled and ground millet and soybean seeds on phytate degradation and Phy/Fe and Phy/Zn molar ratios. International Journal of Food Science & Technology, 40(4), 391–399.
- Liang, J., Han, B.-Z., Nout, M. R., & Hamer, R. J. (2009). Effect of soaking and phytase treatment on phytic acid, calcium, iron and zinc in rice fractions. Food Chemistry, 115(3), 789–794.
- Liu, Z. S., & Chang, S. K. (2013). Nutritional profile and physicochemical properties of commercial soymilk. Journal of Food Processing and Preservation, 37(5), 651–661.
- Murugkar, D. A. (2014). Effect of sprouting of soybean on the chemical composition and quality of soymilk and tofu. Journal of Food Science and Technology, 51(5), 915–921.
- Oyeyinka, S. A., Singh, S., Adebola, P. O., Gerrano, A. S., & Amonsou, E. O. (2015). Physicochemical properties of starches with variable amylose contents extracted from bambara groundnut genotypes. Carbohydrate Polymers, 133, 171–178.
- Paucar-Menacho, L. M., Berhow, M. A., Mandarino, J. M. G., De Mejia, E. G., & Chang, Y. K. (2010). Optimisation of germination time and temperature on the concentration of bioactive compounds in Brazilian soybean cultivar BRS 133 using response surface methodology. Food Chemistry, 119(2), 636–642.
- Poliseli-Scopel, F. H., Hernández-Herrero, M., Guamis, B., & Ferragut, V. (2012). Comparison of ultra high pressure homogenization and conventional thermal treatments on the microbiological, physical and chemical quality of soymilk. LWT-Food Science and Technology, 46(1), 42–48.
- Radzi, M., Rusydi, M., & Azlan, A. (2012). Effect of germination on total phenolic, tannin and phytic acid contents in soy bean and peanut. International Food Research Journal, 19(2), 673–677.
- Randhir, R., Lin, Y.-T., & Shetty, K. (2004). Stimulation of phenolics, antioxidant and antimicrobial activities in dark germinated mung bean sprouts in response to peptide and phytochemical elicitors. Process Biochemistry, 39(5), 637–646.
- Shimelis, E. A., & Rakshit, S. K. (2007). Effect of processing on antinutrients and in vitro protein digestibility of kidney bean (Phaseolus vulgaris L.) varieties grown in East Africa. Food Chemistry, 103(1), 161–172.
- Sugawara, M., Ito, D., Akita, M., Oguri, S., & Momonoki, Y. (2007). Kunitz soybean trypsin inhibitor is modified at its C-terminus by novel soybean thiol protease (protease T1). Plant Production Science, 10(3), 314–321.
- Sulieman, M. A., Eltayeb, M. M., Babiker, E. E., Mustafa, A. I., & El Tinay, A. H. (2008). Effect of sprouting on chemical composition and amino acid content of Sudanese lentil cultivars. Journal of Applied Sciences, 8(12), 2337–2340.
- Taraseviciene, Z., Danilcenko, H., Jariene, E., Paulauskiene, A., & Gajewski, M. (2009). Changes in some chemical components during germination of broccoli seeds. Notulae Botanicae Horti Agrobotanici Cluj-Napoca, 37(2), 173.
- Xu, B., & Chang, S. (2007). A comparative study on phenolic profiles and antioxidant activities of legumes as affected by extraction solvents. Journal of Food Science, 72(2), S159–S166.
- Xu, Z., Chen, Y., Zhang, C., Kong, X., & Hua, Y. (2012). The heat-induced protein aggregate correlated with trypsin inhibitor inactivation in soymilk processing. Journal of Agricultural and Food Chemistry, 60(32), 8012–8019.
- Yang, M., Fu, J., & Li, L. (2012). Rheological characteristics and microstructure of probiotic soy yogurt prepared from germinated soybeans. Food Technology and Biotechnology, 50(1), 73.
- Zhang, G., Xu, Z., Gao, Y., Huang, X., Zou, Y., & Yang, T. (2015). Effects of germination on the nutritional properties, phenolic profiles, and antioxidant activities of buckwheat. Journal of Food Science, 80(5), H1111–H1119.