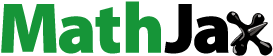
ABSTRACT
This study aims to evaluate the probiotic properties of Lactobacillus strains producing exopolysaccharides (EPS) isolated from different Malaysian fermented foods. Thirty lactobacilli species were isolated, and six of these (TAP1, PIC7, BU11, BU14, TAP16, and TEMP9) were shown to exhibit probiotic characteristics with the potential to produce EPS. The isolates were evaluated for their tolerance to low pH, bile salts, antimicrobial potential, and cell surface properties. The strains were identified using API50CHL and 16S rRNA gene sequences as Lactobacillus belonged to the species fermentum and plantarum. The Lactobacillus strains exhibited high survivability tolerance to low pH and bile salts (above 90%). All strains exhibited auto-aggregation (33.63–59.63%), co-aggregation (24.37–41.9%), and hydrophobicity (16–65.77%) ability. The strains displayed no transferrable antibiotics and antagonistic activity against pathogenic bacteria. Therefore, it indicates that Lactobacillus strains could act as promising probiotic candidates.
RESUMEN
Para el presente estudio se examinaron las propiedades probióticas de cepas de Lactobacillus productoras de exopolisacáridos (CPE) aislados de distintos alimentos fermentados en Malasia. Con este objetivo se aislaron 30 especies de lactobacilos, comprobándose que seis de las mismas (TAP1, PIC7, BU11, BU14, TAP16 y TEMP9) exhiben características probióticas y son potencialmente productoras de CPE. Los aislados fueron evaluados en términos de su tolerancia a bajos niveles de pH y bajas concentraciones de sales biliares, su potencial antimicrobiano y las propiedades de su superficie celular. En tanto Lactobacillus pertenece a las especies fermentum y plantarum, las cepas fueron identificadas usando las secuencias genéticas API50CHL y 16S rRNA. Se constató que las cepas de Lactobacillus mostraron alta tolerancia a bajos niveles de pH y de sales biliares (más de 90%) y por ende elevada tasa de supervivencia. Todas las cepas exhibieron autoagregación (33,63–59,63%), coagregación (24,37–41,9%) y capacidad de hidrofobicidad (16–65,77%). Asimismo, se evidenció que las cepas no presentaron actividad antibiótica o antagonista transferible contra bacterias patogénicas. De lo anterior se concluye que las cepas de Lactobacillus tienen el potencial de ser prometedores agentes probióticos
Introduction
Over the past few decades, there has been an increasing interest in using probiotic bacteria as health-promoting bacteria for a variety of food systems due to their safety, functional, and technological characteristics (Kanmani et al., Citation2013). According to WHO (Citation2001), probiotics can be defined as ‘live microorganisms which, when consumed in adequate amounts as part of food, confer health benefits to the host’. As such, probiotics such as lactic acid bacteria (LAB) have been associated with several health benefits including the enhancement of the human immune system, serum cholesterol reduction, anti-allergic, anticancer, antimicrobial, and antioxidative properties (Caggianiello, Kleerebezem, & Spano, Citation2016).
As the gastrointestinal tract (GIT) is acknowledged of having physical and chemical barriers against ingested microbes, a probiotic bacterium should fulfill various requirements to existing in such a harsh environment. This includes the resistance to bile and low pH, gastric juices, and the ability to adhere and colonize gut epithelial (Bao et al., Citation2010; Del Piano et al., Citation2006). Furthermore, this also includes auto-aggregation, co-aggregation, as well as microbial adhesion to solvents (MATS) (Nivoliez et al., Citation2015). Probiotics should also be resistant to antibiotics (Pinto, Franz, Schillinger, & Holzapfel, Citation2006).
LAB are a heterogeneous group of bacteria found widely in nature and have been extensively used as probiotics. Furthermore, LAB have attained Qualified Presumption of Safety (QPS) status and are Generally Recognized as Safe (GRAS) by the European Food Safety Authority Scientific Committee (EFSA, Citation2007). LAB are associated with fermented foods which produce technologically significant substances such as exopolysaccharides (EPS). In searching for interesting LAB strains with probiotic potential, several studies on fermented food products were used as source materials to identify new isolates. Previously, fermented foods were consumed for their beneficial properties such as their extended shelf life, enhanced flavor, and texture of the final product. Traditional fermented foods are considered as being abundantly available sources of edible microorganisms and several having good probiotic properties, striking functional characteristics, and favorable technological merits (Patel, Lindström, Patel, Prajapati, & Holst, Citation2012).
Regarding probiotic bacteria, LAB possess several characteristics rendering them among the most promising of candidates and exploiting all their metabolites as they can metabolize various substrates, thereby converting these to different metabolites with various applications (Mazzoli, Bosco, Mizrahi, Bayer, & Pessione, Citation2014). Metabolites such as bacteriocins, organic acids, fatty acids, hydrogen peroxides, bioactive peptides, and cell wall components, such as EPS are providing health benefits to the host. These metabolites also play a crucial role in food preservation in addition to their value as aromatic compounds (Patel & Prajapat, Citation2013). The production of extracellular metabolites is an interesting property to consider when selecting putative probiotic strains (P. Ruas-Madiedo et al., Citation2007). EPS produced from the LAB have been associated with a number of health beneficial functions (Caggianiello et al., Citation2016). These include immunostimulation (Hidalgo-Cantabrana et al., Citation2012; G. Vinderola, Perdigón, Duarte, Farnworth, & Matar, Citation2006), antioxidant activity (Polak-Berecka et al., Citation2015; Polak-Berecka, Waśko, & Kubik-Komar, Citation2014), antitumor activity (Nishimura, Citation2014), and blood cholesterol reduction (Ryan, Ross, Fitzgerald, Caplice, & Stanton, Citation2015). Even though it is yet to completely understand the physiological role that play in the ecology of the LAB, it is believed that EPS play a vital role in protecting cells of the LAB from environmental hazards and participate in adhesion and biofilm formation (Looijesteijn, Trapet, De Vries, Abee, & Hugenholtz, Citation2001). Besides, EPS have been found to play an important technological role in texture and rheology (Torino, Font De Valdez, & Mozzi, Citation2015).
Despite the considerable number of well-characterized probiotic strains found globally, many researchers remain interested in discovering new strains with unique features (Vinderola & Reinheimer, Citation2003). To date, limited to no work has been carried out on the production of EPS using Lactobacillus strains isolated from a variety of traditional Malaysian foods. Hence, this study was conducted to isolate Lactobacillus-producing EPS from several traditional Malaysian foods and to evaluate their characteristics as potential probiotics.
Materials and methods
Isolation of LAB
LAB were collected and isolated from a variety of traditional Malaysian fermented foods namely Tempeh, Tapai, pickles, and Budu in three separate batches supplied from local markets. The LAB were isolated by plating on MRS agar media (de Man, Rogosa Sharpe Oxoid Ltd., Hampshire, UK) and incubated anaerobically using an Anaerogen Gas-Pack, (Oxoid Ltd., Hampshire, UK) in an anaerobic jar at 37°C for 24–48 h. Single colonies were initially identified based on gram staining, microscopy examination, and catalase production. Accordingly, representative isolates were considered as presumptive LAB and stored in 20% glycerol at −80°C. For all subsequent assays, the LAB were activated by subculturing anaerobically in MRS broth media supplemented with 0.05% L-Cysteine at 37°C for 18–24 h. All tests were carried out in triplicate with average reading values recorded.
Reference Lactobacillus and pathogenic strains
Two Lactobacillus strains, namely L. rhamnosus GG (ATCC 53103) and L. Plantarum (ATCC 8014), were obtained from the American Type Culture Collection (ATCC) as reference probiotic strains. Moreover, pathogenic bacteria, namely Escherichia coli O157: H7 (ATCC 43895), Salmonella typhimurium (ATCC 13076), Methicillin-resistant Staphylococcus aureus (MRSA ATCC 6538), S. aureus (ATCC 29247), and Pseudomonas aeruginosa (ATCC 27853) were used as pathogen indicators.
Screening for EPS production
Isolated strains were screened for their capability to produce EPS using a modified method (Patel et al., Citation2012). The tested strains were first streaked on the surface of a modified MRS agar media supplemented with sucrose 5% (w/v) and incubated at 37°C for 72 h. Next, the strains with ropy or mucoid features were selected for isolation and quantification of the EPS. EPS were isolated from cell-free supernatants (CFSs) prepared by centrifugation at 8000 rpm for 15 min at 4°C. This was followed by adding a double volume of ethanol (80% v/v) and allowing to precipitate overnight at 4°C. Finally, the EPS were recovered by centrifugation at 10,000 × g for 30 min, at 4°C. The pellets were dissolved in distilled water and dialyzed using dialysis kits against distilled water for 24 h at 4°C. The pellets were then air dried for 96 h and their total weight measured. The phenol-sulfuric acid assay method was used to determine the concentration of EPS (DuBois, Gilles, Hamilton, Rebers, & Smith, Citation1956) using glucose as a standard.
Identification of the selected lactobacilli
The isolates were identified using an API 50CHL kit (BioMerieux, Marcy L’Etoile, France) following the manufacturers’ instructions. 16S rRNA gene sequencing was used for further identification. The universal primers to amplify the 16S rRNA genes were F27 (AGAGTTTGATCMTGGCTCAG) and R1492 (TACGGYTACCTTGTTACGACTT) (Lane, Citation1991; McDonald, Kenna, & Murrell, Citation1995). The amplified fragments were then sequenced and compared with those deposited in the GenBank DNA database using the Basic Local Alignment Search Tool (BLAST; http://blast.ncbi.nlm.nih.gov/Blast.cgi).
Probiotics properties
Acid and bile tolerance
The modified method of Tambekar and Bhutada (Citation2010) was used to determine acid and bile salt tolerance of the selected strains. Briefly, tested strains were grown in MRS broth adjusted to pH 2 or treated with a bile salt concentration of 0.3%. After incubating for 3 h at 37°C, resistances were estimated using viable counting numbers (CFU/mL).
Gastrointestinal transit tolerance
An in vitro assay imitated the gastrointestinal environment and used to assess the viability of the selected strains (Marteau, Minekus, Havenaar, & Huis, Citation1997). A 35 mL cell suspension for each candidate strain was prepared by harvesting the cells via centrifugation at 5000 rpm for 15 min at 10°C. These were then resuspended in the same volume of electrolyte A (containing NaCl, 6.2 g/L; KCl, 2.2 g/L; CaCl2, 0.22 g/L; and NaHCO3, 1.2 g/L, w/v) and adjusted to pH 6.2. Then, 0.10 mL of each pH-adjusted sample was used to simulate the gastric (G1–G7) and intestinal (Gi3–Gi5) conditions plated onto the MRS agar media. Their survival was calculated using a cell count (CFU/ mL), where G1 was used as a control mechanism, while G2 was used to mimic the saliva environment. Lysozyme (0.01% w/v) was mixed with the cell suspension. G3 was used to simulate gastrointestinal juice (a mixture of 3 mL of electrolyte A containing 0.3% (w/v) pepsin and the suspension adjusted to pH 5.0). The digestion steps G4, G5, G6, and G7 were obtained by reducing the pH of the aforementioned suspension from G3 gradually to 4.1, 3.0, 2.1, or 1.8, respectively. After the pH adjustment, the samples were incubated at 37°C by shaking (50 rpm for 20 min) using a shaking incubator.
To simulate the intestinal environment, pH of the samples G3, G4, and G5 were adjusted to 6.5, mixed with 4 mL of electrolyte B (containing NaCl, 5 g/L; KCl, 0.6 g/L; and CaCl2, 0.3 g/L, w/v) containing 0.45% (w/v) bile salts and 0.1% (w/v) pancreatin. The adjusted pH 8.0 was then mixed with the samples and incubated via a shaking incubator at 37°C at 50 rpm for 120 min (Gi3, Gi4, and Gi5) (Vinderola & Reinheimer, Citation2003).
Cell surface properties
Auto-aggregation
The assay was conducted as described previously (Collado, Meriluoto, & Salminen, Citation2007) with some minor modifications. Harvested cells were washed twice with PBS (pH 7.2). The cells were resuspended in PBS (McFarland standard 0.5), vortexed thoroughly, and incubated at 37°C for 5 h. Aliquots (1 mL) were taken from each sample at 0 and 5 h, and the absorbance was measured at OD660 nm. Auto-aggregation (%) was calculated using Equation (1):
where, A5 represents the absorbance at 5 h of incubation, and A0 represents the absorbance at 0 h.
Co-aggregation assay
Bacterial cell suspensions of the six selected strains, as well as individual pathogenic bacteria (namely, E. coli, S. typhimurium, Methicillin-resistant S. aureus MRSA, S. aureus, and P. aeruginosa.) were prepared based on the method that has been described in the auto-aggregation assay.
One milliliter of the cell suspension for each isolate and a pathogenic strain were mixed and incubated at 37°C. The absorbance was measured at OD600 nm at 0 and 5 h of incubation.
Co-aggregation (%) was calculated using Equation (2):
where, A0 is the absorbance of the mixed bacterial suspension at 0 h of incubation, and A5 is the absorbance of the mixed bacterial suspension after 5 h.
Bacterial hydrophobicity
The test was done as described by Doyle and Rosenberg (Citation1995) with some minor modifications. Bacterial cells were harvested and washed twice with PBS, resuspended in 5 mL of PBS, and the optical density (OD580 nm) was determined. A sample of 2 mL cell suspension was added to 2 mL of n-hexadecane, organic phase (Merck, Darmstadt, Germany), and vortexed for 2 min. The phases were allowed to separate for 30 min at room temperature. One milliliter of the water phase was discarded, and OD 580 nm was determined. The test was carried out for all of the six Lactobacillus strains, and the average OD value was determined.
Hydrophobicity (%) was calculated using Equation (3):
where, A0 is the initial OD and A1 is the final OD.
Antibiotic resistance
The standardized method for disk susceptibility testing (Oxoid, Basingstoke, UK) was applied, as previously described by D’Aimmo, Modesto, and Biavati (Citation2007). The testing used the following antibiotics: ampicillin (AMP, 10 ug/mL), erythromycin (E, 15 ug), gentamicin (GN, 10 ug/mL), metronidazole (MTZ, 5 ug/mL), cephalexin (CL, 30 ug/mL), vancomycin (VA, 30 ug), sulphafurazole (SF, 300 ug/mL), clindamycin (DA, 10 ug), nitrofurantoin (F, 300 ug/mL), bacitracin (B, 10 ug/mL), ciprofloxacin (CIP, 5 ug/mL), nalidixic acid (NA, 30 ug/mL), cefuroxime sodium (CXM, 30 ug/mL), and polymyxin B (PB, 300 ug/mL).
Bacterial suspensions equivalent to the McFarland standard value of 0.5 were spread onto Muellar Hinton agar (MHA) plates using a sterile cotton swab. The antibiotic disks were placed on the surface of the agar plates maintained at 4°C for 2 h for diffusion. The diameter (mm) of the inhibition zone was measured after incubation at 37°C for 48 h.
Antibacterial activity
Antagonistic activities of the candidates and reference strains were conducted using the agar well diffusion assay method (Peres et al. Citation2014). The pathogenic bacteria used were E. coli, S. typhimurium, methicillin-resistant S. aureus MRSA, S. aureus, P. aeruginosa, and Listeria monocytogenes. The nutrient agar (Oxoid Ltd., Hampshire, UK) was seeded with the tested pathogens and solidified onto petri dishes, with 6 mm wells formed. Forty microliters of filtered sterilized CFS of the candidate strain were added to each well and allowed to diffuse for 1 h followed by incubation at 37ºC for 24 h. The diameter of the zone of inhibition around each well was measured. The experiment was performed in triplicate.
Characterization of antibacterial substances
The isolated strains were assayed to produce inhibitory substances; bacteriocin, hydrogen peroxide, and organic acids using the same method as described for the antibacterial activity. For the organic acid assay, 5 mL from each CFS was neutralized (pH 6.5), for bacteriocin production. The supernatants were treated with a trypsin enzyme (1 mg/mL) while, to produce hydrogen peroxide, 0.5 mg/mL of catalase (from bovine liver, Sigma-Aldrich) was used. Supernatants without any supplementation were used as a means of control. The inhibitory zones around the wells were measured after the incubation period of 24 h at 37ºC. The experiment was performed in triplicate.
Organic acids profile
Organic acids were assayed for Lactobacillus strains according to the modified method employed by Tejero-Sariñena, Barlow, Costabile, Gibson, and Rowland (Citation2013). An aliquot (20 μL) of the filtered samples was injected into the high-performance liquid chromatography (HPLC) using a UV absorbance detector (Waters®2996 Photodiode Array) set at 220 nm. The column used was an Aminex-HPX 87H Column (300 × 7.8 mm; Bio-Rad, Hercules, CA, USA) maintained at 35°C. Degassed 0.002 M H2SO4 was used as the mobile phase at an applied flow rate of 0.6 mL/min. HPLC-grade lactic, acetic, butyric, isobutyric, and propionic acids (Sigma-Aldrich Co., St. Louis, MO, USA) were used as base standards.
Statistical analysis
The data collected from each experiment were expressed as a mean ± standard deviation. Data were analyzed applying the one-way analysis of variance (ANOVA) using Minitab version 16, statistical software (Minitab Inc., State College, PA, United States). The comparison observed among the treatment means was performed using Turkey’s test. The significance was considered at p ≤ 0.05.
Results and discussion
Isolation and identification of EPS-producing lactobacillus
A total of 76 bacterial strains were isolated from 4 different Malaysian fermented foods named Tapai, pickles, Budu, and Tempeh. Based on morphological characters, 30 isolates were identified as Lactobacillus species and designated as being gram positive, catalase negative, and rod-shaped. Subsequently, a preliminary screening for the isolates using turbidimetric method (OD value) was conducted and the survivability of the isolates was calculated. Six strains which had the greatest survivability values, as well as EPS production ability, were selected for further characterization.
The selected strains categorized by species were then arranged in groups by their carbohydrates fermentation profile (data not shown) and confirmed using 16S rRNA gene sequencing to identify isolated strains. Alignments were performed using BLAST which showed homology > 98%. Five strains were associated with the species fermentum (TAP1, PIC7, BU11, BU14, and TAP16) and one strain associated with plantarum (TEMP9). The gene accession numbers for the strains are KF245553.1, KC348395.1, KF312677.1, HF562939.1, KF149390.1, and JF903803.1 for TAP1, TAP16, PIC7, TEMP9, BU11, and BU14, respectively.
Production of EPS
The current study identified that the lactobacilli strain isolated from several traditional Malaysian foods exhibited a different ability to produce EPS (). The highest quantity of EPS is observed by PIC7 (750 mg/L) followed by BU14 (350 mg/ L). The lowest yield was recorded by BU11 (50 mg/L). Patel et al. (Citation2012) found that in a semi-defined media LAB strains produced EPS ranging from 250 to 900 mg/L. Moreover, Badel, Bernardi, and Michaud (Citation2011) achieved EPS yield of around 1 g/L when the culture condition was not optimized. Van Der Meulen et al. (Citation2007) observed EPS production by applying 10 LAB strains isolated from dairy and cereal products between 0.8 and 1.7 g/ L. Previous work on EPS showed that the addition of sugars as carbon sources may increase EPS yield. Ismail and Nampoothiri (Citation2010) found that EPS production by L. plantarum (MTCC 9510) was 210 mg/L when the media was supplemented with 2% sucrose. When supplementing the media with 2% sucrose, Imran et al. (Citation2016) obtained 250 mg/L EPS yield from L. plantarum (NTMI05 and NTMI20). Moreover, Sánchez, Martínez, Guillén, Jiménez-Díaz, and Rodríguez (Citation2006) found that carbon source has a marked influence on EPS production from L. pentosus (LPS26) and EPS yield of 560 mg/L was obtained when using 30 g/L glucose.
Figure 1. EPS yield (mg/mL) of the isolated Lactobacillus strains.
Figura 1. Rendimiento de CPE (mg/mL) de las cepas aisladas de Lactobacillus.
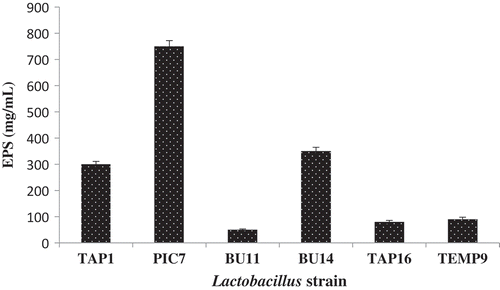
The differences in EPS production suggest that the amount of EPS is a strain-dependent feature influenced by the bacterial strain and the growth phase, including culture medium environmental conditions. The conditions include carbon, nitrogen sources, and physicochemical conditions for bacterial growth (i.e. temperature, pH, oxygen rate, etc.) (Alhudhud, Humphreys, & Laws, Citation2014; P Ruas-Madiedo, Salazar, & De Los Reyes-Gavilán, Citation2009).
In vitro characterization of probiotics potential LAB
Acid and bile salts tolerance
Potential probiotic candidates should have the ability to survive under low pH (i.e. gastric conditions) and for bile salts (i.e. in the small intestine) to successfully pass through the gut providing health benefits to the host (Anandharaj et al., Citation2015). Acidity is considered to be a major destructive factor, affecting the viability of lactobacilli (Shah & Lankaputhra, Citation1997). Singh et al. (Citation2012) observed that the pH condition of the intestinal gastric juice is 2.0–3.0 and is significantly difficult for ingested microorganisms. Therefore, this study aims to select probiotics that can withstand acidic conditions (pH 2.0) in the presence of 0.3% bile salts. The results presented in illustrate all six isolates exhibiting a high survival rate under acidic conditions (pH 2.0), and the highest value was observed by TEMP9 (90.24%). The remainder exhibited good tolerance compared with the reference strain ATCC 8014 (70.54%). This was consistent with the results by Wang, Lin, Ng, and Shyu (Citation2010) and Tulumoglu et al. (Citation2013) who observed high survivability in Lactobacillus strains isolated from the feces of breast-fed infants and children.
Table 1. Viable counts1 (log CFU/mL) and survival rate (SR) of 6 Lactobacillus strains and 2 references under low pH (pH 3.0) and 0.3% bile salts.
Tabla 1. Recuentos viables (log CFU/mL) y tasa de supervivencia (SR) de 6 cepas de Lactobacillus y 2 referencias en condiciones de bajo pH (pH 3.0) y bajas concentraciones de sales biliares (0.3%).
The average concentration of bile salts is proven to be 0.3%, and that food intestinal retention about 4–6 h (Prasad, Gill, Smart, & Gopal, Citation1998). The influence of 0.3% bile salts on the bacterial growth was analyzed where the results indicated that the strain TAP1 had the highest survivability value (98.15%) compared to all other isolated or reference strains. The remaining strains demonstrated the survivability rate ranging from 83.13 to 89.0%, being significantly higher compared to the ATCC8014 reference strain. Previous studies by Pan, Chen, Wu, Tang, and Zhao (Citation2009) and Tulumoglu et al. (Citation2013) observed the reduction in log CFU/mL from an initial count log CFU/mL 8.54–6.09. The survival of Lactobacillus cells at a low pH and their bile tolerance are strain-specific, with extreme variability in resistance found within species (Španová et al., Citation2015).
Gastrointestinal transit tolerance
Resistance to gastric and small intestine conditions are the main in vitro tests frequently suggested for the evaluation of the probiotic potential of an individual strain (Bao et al., Citation2010). To assess the resistance of these strains, regarding the GIT, stress conditions were simulated to model the normal physiological conditions of the GIT. The conditions included the presence of lysozyme, pepsin, sequential gastric emptying at increasingly lower pH values, and the presence of bile salts and pancreatin.
illustrates the effect of simulated gastric juice and intestinal transit on the viability of the candidates. The tested strains exhibited good viability when exposed to different GIT conditions with differences observed in the viable count between each stage. At G1, G2, and G3, the strains were stable with no significant differences found between the isolates and the reference strain under the effect of lysozyme; refer ). However, when the strains were exposed to pH 4.1 (G4), the viable count of TAP16 and BU14 decreased while negligible effects were observed on the viability of other strains. In stages G6 and G7, the strain PIC7 exhibited the highest bacterial count. Although other strains showed a relatively lower viable count, the count of these strains was still higher than the ATCC 8014 reference strain. These results are in line with those reported by Ng, Koon, Padam, and Chye (Citation2015), who found that when the pH is reduced to 1.8 simulating the last gastric emptying to the intestine, a drastic reduction of the viability takes place. Eriksen et al. (Citation2010) indicated that the actual pH of the stomach is 3.0 during food ingestion, because food ingestion has a buffering effect which raises the pH level to 3.0, whereas the pH of the stomach when fasted can be as low as pH 2.0.
Figure 2. Total viable count (log CFU/mL) of the isolated Lactobacillus and references strains in simulated gastric juice (a) and simulated intestinal juice (b) at different digestion steps (G1–G7).
Figura 2. Recuento viable total (log CFU/mL) de Lactobacillus aislada y cepas de referencia en jugos gástricos simulados (a) y jugos intestinales simulados (b) en distintas etapas de la digestión (G1- G7).
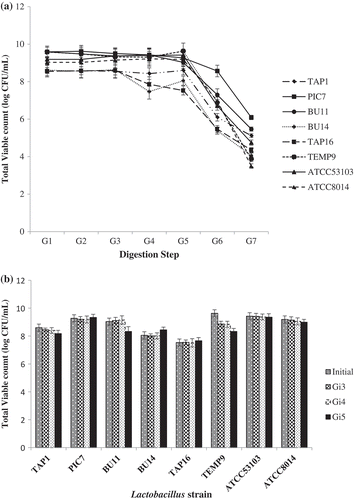
In this study, there were no differences in the survivability of the tested strain when imitating the intestinal condition (pH 8.0 with 0.30% bile salts and 0.1% pancreatin, Gi3–Gi5); refer ). All strains showed a stable pattern. These findings agree with Peres et al. (Citation2014) and Chen et al. (Citation2014) who acknowledged the high survival rate of Lactobacillus strains under intestinal conditions. Such high survival rate may be due to the role of the pepsin enzyme during the hemostasis of pH 8.0. The current study reveals that most of the candidates could survive in the upper section of the digestive tract and transit from the gastric tract to the intestine in a large number of viable potential probiotics.
Cell surface properties
Surface characteristics of the cell are essential prerequisites for probiotic strains that indicate the cell’s ability to adhere to mucosal surfaces in the GIT and to provide health benefits to the host. Bacterial adhesion attributes involve the properties of three participants: the bacterial surface, the human cell surface, and the chemical composition of the suspending medium (Kos et al., Citation2003). The capacities of the isolated strains to auto-aggregation and to adhere to hydrocarbons were examined to evaluate their ability to colonize intestinal epithelia. shows the auto-aggregation and hydrophobicity ability of the isolated strains. The figure illustrates most strains (TEMP9, TAP1, and TAP16) displaying high auto-aggregation abilities and values as compared to the reference strains (i.e. above 50%), whereas the lowest value recorded was for PIC7 (33.63%). According to Wang et al. (Citation2010), good auto-aggregation ability should be greater than 40%, and any strains with the ability less than 10% are considered to have weak auto-aggregation. Thus, the selected isolates in the current study are regarded as having auto-aggregation ability. Interestingly, a study by Todorov et al. (Citation2008) observed auto-aggregation above 70% for samples isolated from a traditional beverage produced from cereal (i.e. boza). Moreover, Nivoliez et al. (Citation2015) obtained auto-aggregation values ranging from 16% to 31% and 12% to 21% for the probiotic strain L. rhamnosus Lcr35 after 3 and 5 h, respectively. Therefore, it is difficult to articulate a standard value for a high auto-aggregation value for Lactobacillus strains. However, values reported herein can be considered relatively reliable.
Figure 3. Percentage (%) of auto-aggregation and surface hydrophobicity of the isolated Lactobacillus and references strains.
Figura 3. Porcentaje (%) de autoagregación e hidrofobicidad superficial de Lactobacillus aislada y de cepas de referencia.
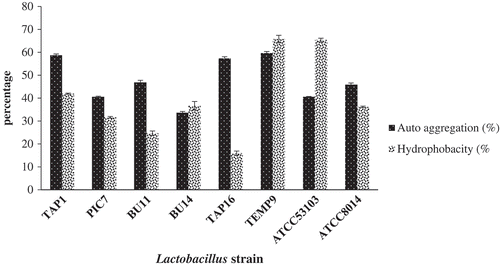
For the hydrophobicity merit, the selected isolates appeared to have various degrees of hydrophobicity ability ranging from 16% demonstrated by TAP16 to 65.77%. This was recorded by TEMP9 which was comparable to the ATCC53103 reference strain. Other strains exhibited a variable degree of hydrophobicity values ranging from 24.37% to 41.9%. A previous study by Angmo, Kumari, & Bhalla (Citation2016) identified that LAB isolated from fermented foods and a beverage of Ladakh are highly variable in hydrophobicity (< 5% to 47%) with a value of 40% for their reference strain (L. casei Shirota). In another study, L. brevis SAU105 exhibits a highly hydrophobic value of 61.0% (Ramos, Thorsen, Schwan, & Jespersen, Citation2013). Similar research shows that the cell surface hydrophobicity of some strains of L. johnsonii and L. acidophilus have values ranging between 23% and 88% and 74% and 95%, respectively (Schillinger, Guigas, & Holzapfel, Citation2005). However, several strains of lactobacilli from the L. acidophilus group show a surface hydrophobicity as low as 2–5% (Schillinger et al., Citation2005). The larger differences in cell surface hydrophobicity could, therefore, be due to a variation in the level of expression of cell surface proteins among the strains. Furthermore, this may be due to environmental conditions affecting the expression of surface proteins (Kaushik et al., Citation2009)
Co-aggregation with pathogens can be used for the initial selection of probiotic bacteria. Hence, co-aggregation properties of probiotics with pathogens are important for both food preservation and their therapeutic impact by eliminating pathogens (Ferreira, Grześkowiak, Collado, & Salminen, Citation2011). Although all tested strains showed some co-aggregation properties, these properties were strain specific. All tested strains exhibited a high ability to co-aggregate with P. aeruginosa above 50% compared with ATCC8014 reference strain; refer to . Except for BU11, the co-aggregation values with indicator pathogen S. typhimurium of all Lactobacillus strains did not differ when compared to those of reference strains. The tested strains showed co-aggregation values with E. coli higher than that of the ATCC8014 reference strain. Moreover, the tested strains had the higher co-aggregation ability with MRSA when compared with ATCC53103 reference strain except for TAP1. However, no differences in co-aggregation ability with S. aureus were noted between tested and reference strains. Tuo et al. (Citation2013) reported co-aggregation ability of 61% for L. casei 137 with E. coli. According to Del Re, Sgorbati, Miglioli, and Palenzona (Citation2000), the cell surface traits may be used for preliminary screening to identify potentially adherent isolates. The results, however, should be interpreted with caution, because this adherence does not necessarily mean in vivo cell adhesion (Bautista-Gallego et al., Citation2013)
Table 2. Co-aggregationa% ability of the Lactobacillus strains with five pathogenic bacteria.
Tabla 2. Capacidad de coagregación1% de las cepas de Lactobacillus con cinco bacterias patogénicas
Antibiotics susceptibility tests
One of the safety considerations for a potential probiotic strain is that it does not contain transferable antibiotic resistance genes to the pathogenic microorganisms which may cause significant danger. Hence, the selected lactobacilli strains were subjected to commonly prescribed antibiotics as shown in . In this study, all isolated strains showed resistance to vancomycin, ciprofloxacin, nalidixic acid, nitrofurantoin, and gentamicin, except for TAP16 and TEMP9. However, most of the tested strains were susceptible to ampicillin, metronidazole, cephalexin, polymyxin B, bacitracin, sulphafurazole, erythromycin, clindamycin, and bacitracin. These findings are in agreement with recent results of Leite et al. (Citation2015) who found that LAB strains isolated from Brazilian kefir grains were susceptible to all analyzed antimicrobial agents. These include tetracycline, erythromycin, clindamycin, ampicillin, and aminoglycosides, except vancomycin where an intrinsic resistance is observed. J. Wang, Li, Zhou, Zhang, and Shi (Citation2016) confirmed that the strain of L. rhamnosus L1, isolated from silage, shows sensitivity traits for six antibiotics, including ampicillin, tetracycline, chloramphenicol, ciprofloxacin, and with erythromycin being slightly sensitive to kanamycin, while resistant to VA and MTZ. The resistance of Lactobacillus to VA has been previously reported by Danielsen and Wind (Citation2003). The resistance of Lactobacillus strains to some antibiotics (i.e. VA) is considered intrinsic property rather than transmissible, presenting no safety concerns in food (Domingos-Lopes, Stanton, Ross, Dapkevicius, & Silva, Citation2017). In fact, intrinsic resistance to some antibiotics could be regarded an advantage of these genera, as they can play a probiotic role in human GIT during antibiotic therapy (Sharma, Tomar, Sangwan, Goswami, & Singh, Citation2016).
Table 3. Antibioticsa susceptibility of six of Lactobacillus strains and the two reference strains.
Tabla 3. Susceptibilidad a los antibióticos1 de seis cepas de Lactobacillus y dos cepas de referencia.
Antibacterial activity tests
Antagonistic activity of LAB against pathogens is regarded as a characteristic of probiotic to maintain the gut microflora balanced and to keep the gut rid of pathogens. The inhibition of pathogenic bacteria by probiotics includes the production of nonspecific antimicrobial compounds such as short-chain fatty acids (SCFA), H2O2 and low-molecular-weight proteins known as bacteriocins and bacteriocin-like inhibitory substances.
In this study, the inhibitory activities of selected Lactobacillus strains against several pathogens revealed that selected strains showed the ability to inhibit the growth of pathogenic bacteria with different levels; refer . Lactobacillus strains TAP1, BU14, and TEMP9 exhibited excellent capabilities to inhibit indicator pathogenic bacteria and are considered better than the reference strain ATCC 8014, and more like the commercial strain ATCC5103. Different levels of inhibitory activities were reported for some other lactobacilli isolated from various sources (Hor & Liong, Citation2014; Ng et al., Citation2015; Ramos et al., Citation2013; Roberts et al., Citation1995). The prior study by Cruz-Guerrero et al. (Citation2014) highlighted that SCFA, H2O2, and bacteriocins are among the main reported compounds with antimicrobial activity produced by probiotic bacteria. In this study, the CFS of the candidates were tested for production of inhibitory compounds against the indicator strain E. Coli, by treating neutralized CFS with catalase or trypsin and comparing with an untreated controlled one. identifies the results of the inhibition zone for untreated CFS (i.e. pH unadjusted). The results ranging from 9.7 to 16.6 mm for the untreated CFS samples, 9.7–15.9 mm for CFS treated with trypsin, and 9.5 to 16.0 mm for CFS treated with catalase enzyme (). Whereas, the neutralized CFS (adjusted to pH 6.5) were not able to inhibit the growth of the indicator-pathogen indicating that inhibition by the candidates referred to the production of organic acid. These findings are in accordance with results reported by Angmo et al. (Citation2016) and Shokryazdan et al. (Citation2014). Similar levels of antagonistic activity resistance were reported by indigenous L. plantarum Lp9, resulting from the production of various organic acids by Lp9 during fermentation (Kaushik et al., Citation2009). The lethal effects of organic acids on pathogens are considered to be related to the lowering of the intracellular pH and the depletion of the cell membrane. The antagonistic effect of these bacteria varies considerably depending upon the species and strains.
Table 4. Antibacterial activities of Lactobacillus strains CFS against indicator pathogens.
Tabla 4. Actividades antibacterianas de cepas de Lactobacillus CFS contra patógenos indicadores
Table 5. Inhibitory activitya of untreated and treated (trypsin, catalase, or adjusted to pH 6.5) Lactobacillus CFS against E. coli.
Tabla 5. Actividad inhibitoria1 de Lactobacillus CFS sin tratar y tratados (tripsina, catalasa o ajustada a pH 6.5) contra E. coli.
Organic acids profiles
The primary antimicrobial effect exerted by LAB could be explained by applying different mechanisms such as the competition for limiting resources and/or production of metabolites such as bacteriocins, hydrogen peroxides, and organic acids (Buddington, Citation2009). Refer for the results of the organic acid assay performed in this study. The results reveal that lactic acid is predominantly produced by isolated strains and with the highest amount being 226.2 mM. This was generated by the strain BU11, whereas the strain BU14 demonstrated the lowest value of 138.62 mM. Nevertheless, acetic acid produced by TAP1 was recorded with the highest value of 156.05 mM, followed by PIC7 150.37 mM, and compared with BU14 which produced 134.57 mM. The remaining organic acids produced by the candidates were of a lesser amount.
Table 6. Organic acids production (mM) profile of the Lactobacillus strains.
Tabla 6. Perfil de producción (mM) de ácidos orgánicos de cepas de Lactobacillus
The antibacterial effect of LAB could be due to the production of organic acids especially SCFA, which reduce the pH of the immediate growth medium environment, rendering them unsuitable for the growth of a broad range of gram-positive bacteria (Aween, Hassan, Muhialdin, Noor, & Eljamel, Citation2012). The higher pH environment in the bacterial cytoplasm will cause the acids to dissociate and interrupt the transport process in the cells by disrupting the proton motive force (Adams & Hall, Citation1988). Furthermore, the study conducted by Servin (Citation2004) identifies that the production of SCFA (e.g. lactic, acetic, propionic, and butyric acids) by lactobacilli lowers the pH of the medium. This reduction in the pH leads to growth inhibition of pathogenic bacteria such as acetic and lactic acids and inhibits the growth of E. coli at concentrations of 1.62 and 2.97 mg mL−1, respectively (Conner & Kotrola, Citation1995).
Conclusions
The current study reveals that six of Lactobacillus strains isolated from a variety of traditional Malaysian foods were belonged to two species: L. plantarum and L. fermentum (TAP1, PIC7, BU11, BU14, TAP16, and TEMP9) and selected according to their ability to produce EPS. The isolates demonstrated the high capability to withstand harsh environmental conditions such as low pH, bile salts, and GIT. The strains additionally displayed cell surface properties and antibacterial activity of the tested strains being sensitive to common antibiotics. Therefore, the results of the study identified that the isolates can act as promising probiotic candidates with EPS production features.
Disclosure statement
No potential conflict of interest was reported by the authors.
Additional information
Funding
References
- Adams, M. R., & Hall, C. J. (1988). Growth inhibition of food-borne pathogens by lactic and acetic acids and their mixtures. International Journal of Food Science & Technology, 23, 287–292. doi:10.1111/j.1365-2621.1988.tb00581.x
- Alhudhud, M., Humphreys, P., & Laws, A. (2014). Development of a growth medium suitable for exopolysaccharide production and structural characterisation by Bifidobacterium animalis ssp. lactis AD011. Journal of Microbiological Methods, 100, 93–98. doi:10.1016/j.mimet.2014.02.021
- Anandharaj, M., Sivasankari, B., Santhanakaruppu, R., Manimaran, M., Rani, R. P., & Sivakumar, S. (2015). Determining the probiotic potential of cholesterol-reducing Lactobacillus and Weissella strains isolated from gherkins (fermented cucumber) and south Indian fermented koozh. Research in Microbiology, 166, 428–439. doi:10.1016/j.resmic.2015.03.002
- Angmo, K., Kumari, A., & Bhalla, T. C. (2016). Probiotic characterization of lactic acid bacteria isolated from fermented foods and beverage of Ladakh. LWT-Food Science and Technology, 66, 428–435. doi:10.1016/j.lwt.2015.10.057
- Aween, M. M., Hassan, Z., Muhialdin, B. J., Noor, H. M., & Eljamel, Y. A. (2012). Evaluation on antibacterial activity of Lactobacillus acidophilus strains isolated from honey. American Journal of Applied Sciences, 9, 807–817. doi:10.3844/ajassp.2012.807.817
- Badel, S., Bernardi, T., & Michaud, P. (2011). New perspectives for Lactobacilli exopolysaccharides. Biotechnology Advances, 29, 54–66. doi:10.1016/j.biotechadv.2010.08.11
- Bao, Y., Zhang, Y., Zhang, Y., Liu, Y., Wang, S., Dong, X., … Zhang, H. (2010). Screening of potential probiotic properties of Lactobacillus fermentum isolated from traditional dairy products. Food Control, 21, 695–701. doi:10.1016/j.foodcont.2009.10.010
- Bautista-Gallego, J., Arroyo-López, F. N., Rantsiou, K., Jiménez-Díaz, R., Garrido-Fernández, A., & Cocolin, L. (2013). Screening of lactic acid bacteria isolated from fermented table olives with probiotic potential. Food Research International, 50, 135–142. doi:10.1016/j.foodres.2012.10.004
- Buddington, R. (2009). Using probiotics and prebiotics to manage the gastrointestinal tract ecosystem. In D. Charalampopoulos & R. A. Rastall (Eds.), Prebiotics and probiotics science and technology (pp. 1–31). New York, NY: Springer.
- Caggianiello, G., Kleerebezem, M., & Spano, G. (2016). Exopolysaccharides produced by lactic acid bacteria: From health-promoting benefits to stress tolerance mechanisms. Applied Microbiology and Biotechnology, 100, 3877–3886. doi:10.1007/s00253-016-7471-2
- Chen, P., Zhang, Q., Dang, H., Liu, X., Tian, F., Zhao, J., … Chen, W. (2014). Screening for potential new probiotic based on probiotic properties and α-glucosidase inhibitory activity. Food Control, 35, 65–72. doi:10.1016/j.foodcont.2013.06.027
- Collado, M. C., Meriluoto, J., & Salminen, S. (2007). Adhesion and aggregation properties of probiotic and pathogen strains. European Food Research and Technology, 226, 1065–1073. doi:10.1007/s00217-007-0632-x
- Conner, D. E., & Kotrola, J. S. (1995). Growth and survival of Escherichia coli O157: H7 under acidic conditions. Applied and Environmental Microbiology, 61, 382–385.
- Cruz-Guerrero, A., Hernández-Sánchez, H., Rodríguez-Serrano, G., Gómez-Ruiz, L., García-Garibay, M., & Figueroa-González, I. (2014). Commercial probiotic bacteria and prebiotic carbohydrates: A fundamental study on prebiotics uptake, antimicrobials production and inhibition of pathogens. Journal of the Science of Food and Agriculture, 94, 2246–2252. doi:10.1002/jsfa.6549
- D’Aimmo, M. R., Modesto, M., & Biavati, B. (2007). Antibiotic resistance of lactic acid bacteria and Bifidobacterium spp. isolated from dairy and pharmaceutical products. International Journal of Food Microbiology, 115, 35–42. doi:10.1016/j.ijfoodmicro.2006.10.003
- Danielsen, M., & Wind, A. (2003). Susceptibility of Lactobacillus spp. to antimicrobial agents. International Journal of Food Microbiology, 82, 1–11. doi:10.1016/S0168-1605(02)00254-4
- Del Piano, M., Morelli, L., Strozzi, G. P., Allesina, S., Barba, M., Deidda, F., … Capurso, L. (2006). Probiotics: From research to consumer. Digestive and Liver Disease, 38, S248–S255. doi:10.1016/S1590-8658(07)60004-8
- Del Re, B., Sgorbati, B., Miglioli, M., & Palenzona, D. (2000). Adhesion, autoaggregation and hydrophobicity of 13 strains of Bifidobacterium longum. Letters in Applied Microbiology, 31, 438–442. doi:10.1046/j.1365-2672.2000.00845.x
- Domingos-Lopes, M. F. P., Stanton, C., Ross, P. R., Dapkevicius, M. L. E., & Silva, C. C. G. (2017). Genetic diversity, safety and technological characterization of lactic acid bacteria isolated from artisanal Pico cheese. Food Microbiology, 63, 178–190. doi:10.1016/j.fm.2016.11.014
- Doyle, R. J., & Rosenberg, M. (1995). Measurement of microbial adhesion to hydrophobic substrata. Methods in Enzymology, 253, 542–550. doi:10.1016/S0076-6879(95)53046-0
- DuBois, M., Gilles, K. A., Hamilton, J. K., Rebers, P. A., & Smith, F. (1956). Colorimetric method for determination of sugars and related substances. Analytical Chemistry, 28, 350–356. doi:10.1021/ac60111a017
- EFSA. (2007). Introduction of a Qualified Presumption of Safety (QPS) approach for assessment of selected microorganisms referred to EFSA1. Opinion of the Scientific Committee (Question No EFSA-Q-2005-293. The EFSA Journal, 587, 1–16. Retrieved from www.efsa.europa.eu/en/scdocs/doc/587.pdf
- Eriksen, E. K., Holm, H., Jensen, E., Aaboe, R., Devold, T. G., Jacobsen, M., & Vegarud, G. E. (2010). Different digestion of caprine whey proteins by human and porcine gastrointestinal enzymes. British Journal of Nutrition, 104, 374–381. doi:10.1017/S0007114510000577
- Ferreira, C. L., Grześkowiak, Ł., Collado, M. C., & Salminen, S. (2011). In vitro evaluation of Lactobacillus gasseri strains of infant origin on adhesion and aggregation of specific pathogens. Journal of Food Protection, 74, 1482–1487. doi:10.4315/0362-028X.JFP-11-074
- Hidalgo-Cantabrana, C., López, P., Gueimonde, M., De Los Reyes-Gavilán, G., Suárez, A., Margolles, A., & Ruas-Madiedo, P. (2012). Immune modulation capability of exopolysaccharides synthesised by lactic acid bacteria and bifidobacteria. Probiotics and Antimicrobial Proteins, 4, 227–237. doi:10.1007/s12602-012-9110-2
- Hor, Y. Y., & Liong, M. T. (2014). Use of extracellular extracts of lactic acid bacteria and bifidobacteria for the inhibition of dermatological pathogen Staphylococcus aureus. Dermatologica Sinica, 32, 141–147. doi:10.1016/j.dsi.2014.03.001
- Imran, M. Y. M., Reehana, N., Jayaraj, K. A., Ahamed, A. A. P., Dhanasekaran, D., Thajuddin, N., … Muralitharan, G. (2016). Statistical optimization of exopolysaccharide production by Lactobacillus plantarum NTMI05 and NTMI20. International Journal of Biological Macromolecules, 93, 731–745. doi:10.1016/j.ijbiomac.2016.09.007
- Ismail, B., & Nampoothiri, K. M. (2010). Production, purification and structural characterization of an exopolysaccharide produced by a probiotic Lactobacillus plantarum MTCC 9510. Archives of Microbiology, 192, 1049–1057. doi:10.1007/s00203-010-0636-y
- Kanmani, P., Satish Kumar, R., Yuvaraj, N., Paari, K. A., Pattukumar, V., & Arul, V. (2013). Probiotics and its functionally valuable products – A review. Critical Reviews in Food Science and Nutrition, 53, 641–658. doi:10.1080/10408398.2011.553752
- Kaushik, J. K., Kumar, A., Duary, R. K., Mohanty, A. K., Grover, S., Batish, V. K., & Sechi, L. A. (2009). Functional and probiotic attributes of an indigenous isolate of Lactobacillus plantarum. PLoS ONE, 4(12), e8099. doi:10.1371/journal.pone.0008099
- Kos, B. V. Z. E., Šušković, J., Vuković, S., Šimpraga, M., Frece, J., & Matošić, S. (2003). Adhesion and aggregation ability of probiotic strain Lactobacillus acidophilus M92. Journal of Applied Microbiology, 94, 981–987. doi:10.1046/j.1365-2672.2003.01915.x
- Lane, D. J. (1991). 16S/23S rRNA sequencing. In E. Stackebrandt & M. Goodfellow (Eds.), Nucleic Acid Techniques in Bacterial Systematics(pp. 115–175). Chichester: Wiley.
- Leite, A. M. O., Miguel, M. A. L., Peixoto, R. S., Ruas-Madiedo, P., Paschoalin, V. M. F., Mayo, B., & Delgado, S. (2015). Probiotic potential of selected lactic acid bacteria strains isolated from Brazilian kefir grains. Journal of Dairy Science, 98, 3622–3632. doi:10.3168/jds.2014-9265
- Looijesteijn, P. J., Trapet, L., De Vries, E., Abee, T., & Hugenholtz, J. (2001). Physiological function of exopolysaccharides produced by Lactococcus lactis. International Journal of Food Microbiology, 64(1–2), 71–80. doi:10.1016/S0168-1605(00)00437-2
- Marteau, P. M., Minekus, M., Havenaar, R., & Huis, J. H. J. (1997). Survival of lactic acid bacteria in a dynamic model of the stomach and small intestine: Validation and the effects of bile. Journal of Dairy Science, 80, 1031–1037. doi:10.3168/jds.S0022-0302(97)76027-2
- Mazzoli, R., Bosco, F., Mizrahi, I., Bayer, E. A., & Pessione, E. (2014). Towards lactic acid bacteria-based biorefineries. Biotechnology Advances, 32, 1216–1236. doi:10.1016/j.biotechadv.2014.07.005
- McDonald, I. R., Kenna, E. M., & Murrell, J. C. (1995). Detection of methanotrophic bacteria in environmental samples with the PCR. Applied and Environmental Microbiology, 61, 116-121.
- Ng, S. Y., Koon, S. S., Padam, B. S., & Chye, F. Y. (2015). Evaluation of probiotic potential of lactic acid bacteria isolated from traditional Malaysian fermented Bambangan (Mangifera pajang). CyTA-Journal of Food, 13, 563–572. doi:10.1080/19476337.2015.1020342
- Nishimura, J. (2014). Exopolysaccharides Produced from Lactobacillus delbrueckii subsp. bulgaricus. Advances in Microbiology, 4, 1017–1023. doi:10.4236/aim.2014.414112
- Nivoliez, A., Veisseire, P., Alaterre, E., Dausset, C., Baptiste, F., Camarès, O., … Bornes, S. (2015). Influence of manufacturing processes on cell surface properties of probiotic strain Lactobacillus rhamnosus Lcr35®. Applied Microbiology and Biotechnology, 99, 399–411. doi:10.1007/s00253-014-6110-z
- Pan, X., Chen, F., Wu, T., Tang, H., & Zhao, Z. (2009). The acid, bile tolerance and antimicrobial property of Lactobacillus acidophilus NIT. Food Control, 20, 598–602. doi:10.1016/j.foodcont.2008.08.019
- Patel, A., & Prajapat, J. B. (2013). Food and health applications of exopolysaccharides produced by lactic acid bacteria. Advances in Dairy Research, 1, 1–8. doi:10.4172/2329-888x.1000107.
- Patel, A. R., Lindström, C., Patel, A., Prajapati, J. B., & Holst, O. (2012). Screening and isolation of exopolysaccharide producing lactic acid bacteria from vegetables and indigenous fermented foods of Gujarat, India. International Journal of Fermented Foods, 1, 87–101.
- Peres, C. M., Alves, M., Hernandez-Mendoza, A., Moreira, L., Silva, S., Bronze, M. R., … Malcata, F. X. (2014). Novel isolates of lactobacilli from fermented Portuguese olive as potential probiotics. LWT-Food Science and Technology, 59, 234–246. doi:10.1016/j.lwt.2014.03.003
- Pinto, M. G. V., Franz, C. M., Schillinger, U., & Holzapfel, W. H. (2006). Lactobacillus spp. with in vitro probiotic properties from human faeces and traditional fermented products. International Journal of Food Microbiology, 109, 205–214. doi:10.1016/j.ijfoodmicro.2006.01.029
- Polak-Berecka, M., Choma, A., Waśko, A., Górska, S., Gamian, A., & Cybulska, J. (2015). Physicochemical characterization of exopolysaccharides produced by Lactobacillus rhamnosus on various carbon sources. Carbohydrate Polymers, 117, 501–509. doi:10.1016/j.carbpol.2014.10.006
- Polak-Berecka, M., Waśko, A., & Kubik-Komar, A. (2014). Optimization of culture conditions for exopolysaccharide production by a probiotic strain of Lactobacillus rhamnosus E/N. Polish Journal of Microbiology, 63, 253–257.
- Prasad, J., Gill, H., Smart, J., & Gopal, P. K. (1998). Selection and characterisation of Lactobacillus and bifidobacterium strains for use as probiotics. International Dairy Journal, 8, 993–1002. doi:10.1016/S0958-6946(99)00024-2
- Ramos, C. L., Thorsen, L., Schwan, R. F., & Jespersen, L. (2013). Strain-specific probiotics properties of Lactobacillus fermentum, Lactobacillus plantarum and Lactobacillus brevis isolates from Brazilian food products. Food Microbiology, 36, 22–29. doi:10.1016/j.fm.2013.03.010
- Roberts, C. M., Fett, W. F., Osman, S. F., Wijey, C., O’Connor, J. V., & Hoover, D. G. (1995). Exopolysaccharide production by Bifidobacterium longum BB-79. Journal of Applied Bacteriology, 78, 463–468. doi:10.1111/j.1365-2672.1995.tb03085.x
- Ruas-Madiedo, P., Moreno, J. A., Salazar, N., Delgado, S., Mayo, B., Margolles, A., & De Los Reyes-Gavilan, C. G. (2007). Screening of exopolysaccharide-producing Lactobacillus and Bifidobacterium strains isolated from the human intestinal microbiota. Applied and Environmental Microbiology, 73, 4385–4388. doi:10.1128/AEM.02470-06
- Ruas-Madiedo, P., Salazar, N., & De Los Reyes-Gavilán, C. G. (2009). Exopolysaccharides produced by lactic acid bacteria in food and probiotic applications. In A. P. Moran, O. Holst, P. J. Brennan, & M. von Itzstein (Eds.), Microbial Glycobiology, Structures, Relevance and Applications (pp. 887–902). London: Academic Press.
- Ryan, P. M., Ross, R. P., Fitzgerald, G. F., Caplice, N. M., & Stanton, C. (2015). Sugar-coated: Exopolysaccharide producing lactic acid bacteria for food and human health applications. Food & Function, 6, 679–693. doi:10.1039/C4FO00529E
- Sánchez, J. I., Martínez, B., Guillén, R., Jiménez-Díaz, R., & Rodríguez, A. (2006). Culture conditions determine the balance between two different exopolysaccharides produced by Lactobacillus pentosus LPS26. Applied and Environmental Microbiology, 72, 7495–7502. doi:10.1128/AEM.01078-06
- Schillinger, U., Guigas, C., & Holzapfel, W. H. (2005). In vitro adherence and other properties of lactobacilli used in probiotic yoghurt-like products. International Dairy Journal, 15, 1289–1297. doi:10.1016/j.idairyj.2004.12.008
- Servin, A. L. (2004). Antagonistic activities of lactobacilli and bifidobacteria against microbial pathogens. Fems Microbiology Reviews, 28, 405-440. doi: 10.1016/j.femsre.2004.01.003
- Shah, N. P., & Lankaputhra, W. E. (1997). Improving viability of Lactobacillus acidophilus and Bifidobacterium spp. in yogurt. International Dairy Journal, 7, 349–356. doi:10.1016/S0958-6946(97)00023-X
- Sharma, P., Tomar, S. K., Sangwan, V., Goswami, P., & Singh, R. (2016). Antibiotic resistance of Lactobacillus sp. isolated from commercial probiotic preparations. Journal of Food Safety, 36, 38–51. doi:10.1111/jfs.12211
- Shokryazdan, P., Sieo, C. C., Kalavathy, R., Liang, J. B., Alitheen, N. B., Jahromi, M. F., & Ho, Y. W. (2014). Probiotic potential of Lactobacillus strains with antimicrobial activity against some human pathogenic strains. BioMed Research International, 2014, 1–16. doi:10.1155/2014/927268
- Singh, T. P., Kaur, G., Malik, R. K., Schillinger, U., Guigas, C., & Kapila, S. (2012). Characterization of intestinal Lactobacillus reuteri strains as potential probiotics. Probiotics and Antimicrobial Proteins, 4, 47–58. doi:10.1007/s12602-012-9090-2
- Španová, A., Dráb, V., Turková, K., Špano, M., Burdychová, R., Šedo, O., … Rittich, B. (2015). Selection of potential probiotic Lactobacillus strains of human origin for use in dairy industry. European Food Research and Technology, 241, 861–869. doi:10.1007/s00217-015-2511-1
- Tambekar, D. H., & Bhutada, S. A. (2010). An evaluation of probiotic potential of Lactobacillus sp. from milk of domestic animals and commercial available probiotic preparations in prevention of enteric bacterial infections. Recent Research in Science and Technology, 2, 82–88.
- Tejero-Sariñena, S., Barlow, J., Costabile, A., Gibson, G. R., & Rowland, I. (2013). Antipathogenic activity of probiotics against Salmonella typhimurium and Clostridium difficile in anaerobic batch culture systems: Is it due to synergies in probiotic mixtures or the specificity of single strains? Anaerobe, 24, 60–65. doi:10.1016/j.anaerobe.2013.09.011
- Todorov, S. D., Botes, M., Guigas, C., Schillinger, U., Wiid, I., Wachsman, M. B., Holzapfel, W. H., & Dicks, L. M. T. (2008). Boza, a natural source of probiotic lactic acid bacteria. Journal of Applied Microbiology, 104, 465–477. doi:10.1111/j.1365-2672.2007.03558.x
- Torino, M. I., Font de Valdez, G., & Mozzi, F. (2015). Biopolymers from lactic acid bacteria. Novel applications in foods and beverages. Frontier in Microbiolology, 6, 1–16. doi:10.3389/fmicb.2015.00834
- Tulumoglu, S., Yuksekdag, Z. N., Beyatli, Y., Simsek, O., Cinar, B., & Yaşar, E. (2013). Probiotic properties of lactobacilli species isolated from children’s feces. Anaerobe, 24, 36–42. doi:10.1016/j.anaerobe.2013.09.006
- Tuo, Y., Yu, H., Ai, L., Wu, Z., Guo, B., & Chen, W. (2013). Aggregation and adhesion properties of 22 Lactobacillus strains. Journal of Dairy Science, 96, 4252–4257. doi:10.3168/jds.2013-6547
- Van der Meulen, R., Grosu-Tudor, S., Mozzi, F., Vaningelgem, F., Zamfir, M., De Valdez, G. F., & De Vuyst, L. (2007). Screening of lactic acid bacteria isolates from dairy and cereal products for exopolysaccharide production and genes involved. International Journal of Food Microbiology, 118, 250–258. doi:10.1016/j.ijfoodmicro.2007.07.014
- Vinderola, C. G., & Reinheimer, J. A. (2003). Lactic acid starter and probiotic bacteria: A comparative “in vitro” study of probiotic characteristics and biological barrier resistance. Food Research International, 36, 895–904. doi:10.1016/S0963-9969(03)00098-X
- Vinderola, G., Perdigón, G., Duarte, J., Farnworth, E., & Matar, C. (2006). Effects of the oral administration of the exopolysaccharide produced by Lactobacillus kefiranofaciens on the gut mucosal immunity. Cytokine, 36, 254–260. doi:10.1016/j.cyto.2007.01.003
- Wang, C.-Y., Lin, P.-R., Ng, C.-C., & Shyu, Y.-T. (2010). Probiotic properties of Lactobacillus strains isolated from the feces of breast-fed infants and Taiwanese pickled cabbage. Anaerobe, 16, 578–585. doi:10.1016/j.anaerobe.2010.10.003
- Wang, J., Li, B., Zhou, L., Zhang, X., & Shi, P. (2016). Probiotic potential and function of a Lactobacillus strain L1 isolated from Silage. Journal of Food Safety. doi:10.1111/jfs.12338
- WHO. (2001). Health and nutritional properties of probiotics in food including powdered milk with live lactic acid bacteria: A joint FAO/WHO expert consultation report. Retrieved December 3, 2016, from World Health Orgnization (WHO) http://isappscience.org/wp-content/uploads/2015/12/FAO-WHO-2001-Probiotics-Report.pdf