ABSTRACT
The effects of three drying methods on volatile flavor components of Tuber indicum were studied. After hot air drying (AD), vacuum drying (VD), and vacuum-freeze drying (FD), flavor components were analyzed by headspace solid phase microextraction GC-MS and electronic nose (E-nose). The results from GC-MS showed that aldehydes (54.8%) and alcohols (31.4%) are the two dominant chemical species in fresh T. indicum and eight carbon (C8) compounds including 1-octen-3-ol, 3-octanol, n-octanol, 3-octanone. After dehydrating, C8 compounds, aldehyde, and ester components reduced, while alkanes, heterocyclic, and sulfur components were produced. Multivariate statistical analysis of the GC-MS revealed the components responsible for the chemical differences between fresh and three drying samples. In addition, E-nose could discriminate fresh and three drying samples. The result obtained by E-nose showed good identity compared with GC–MS. Therefore, FD was the optimal dehydrating method to preserve Tuberaceae with the most retained fresh flavor.
RESUMEN
El presente estudio se planteó investigar los efectos que tres métodos de secado tienen en los componentes de los sabores volátiles de la Tuber indicum. Los componentes de sabor fueron analizados por microextracción en fase sólida con espacio de cabeza, GC-MS y nariz electrónica después de la aplicación de secado al aire caliente (AD), secado al vacío (VD) y liofilizado al vacío (FD). Los resultados de la GC-MS mostraron que las dos especies químicas dominantes en la T. indicum fresca son los aldehídos (54.8%) y los alcoholes (31.4%), y que ésta contiene, además, ocho compuestos de carbono (C8), incluyendo 1-octa-3-ol, 3-octanol, n-octanol y 3-octanona. Se comprobó que después de efectuada la deshidratación, los compuestos C8, los aldehídos y los componentes estéricos disminuyeron, al tiempo que se produjeron alcanos, heterocíclicos y componentes de azufre. El análisis multivariado estadístico de la GC-MS dio cuenta de los componentes responsables de las diferencias químicas entre las muestras secas y aquellas obtenidas por métodos de secado. Asimismo, la nariz electrónica pudo discriminar entre las muestras frescas y las obtenidas por los tres métodos de secado. En comparación con la GC-MS, el resultado obtenido por la nariz electrónica presentó una identidad favorable. Por lo tanto, el método de deshidratación FD fue el óptimo para conservar las Tuberaceae con mayor sabor a fresco.
1. Introduction
Tuber indicum (Chinese black truffle), edible fungi found majorly underground, is not only rich in nutrients and distinctive flavor, but also showing resistance to bacteria and virus (Dahham, Al-Rawi, Ibrahim, Majid, & Majid, Citation2016; Miao et al., Citation2014). It is a popular mushroom product among consumers. However, Tuber indicum is extremely susceptible to degradation, which brings difficulty to storage and transportation, and consequently results in great loss of food quality as well as economic value (Miao et al., Citation2014). Drying is proved to be an effective method in order to extend food shelf life, and has been widely used in the processing of edible fungi (Bhattacharya, Srivastav, & Mishra, Citation2015; Huang, Zhang, Wang, Mujumdar, & Sun, Citation2012), such as the drying of mushroom (Guo, Xia, Tan, Chen, & Ming, Citation2014), Agaricus bisporus (Kumar, Singh, & Singh, Citation2013), and porcini (Aprea et al., Citation2015). There are three common drying methods currently used in massive production of dehydrated fruits and vegetables, including hot air drying (AD), vacuum drying (VD), and freeze-vacuum drying (FD) (Qiao, Fang, Huang, & Zhang, Citation2013; Saxena, Maity, Raju, & Bawa, Citation2012). Many studies have reported the changes in color, texture, rehydration capability, mechanical property, and flavor profile caused by different drying methods (Culleré, Ferreira, Venturini, Marco, & Blanco, Citation2013; Guiné & Barroca, Citation2012; Pei et al., Citation2016; Vega-Gálvez et al., Citation2012). For instance, the hardness of pepper increases as the drying temperature increases, and low temperature drying with AD has little influence on the sample color (Guiné & Barroca, Citation2012). The volatile flavor components of T. indicum are chiefly aldehydes and alcohols, and most of which are eight carbon chain (C8) compounds with distinct aroma (Culleré et al., Citation2013). A research studying the effects of FD and microwave vacuum drying (MD) on the volatile components of Agaricus bisporus concluded that drying by MD had more similar volatile components to fresh Agaricus bisporus than the ones dried by FD (Pei et al., Citation2016). The effects of four drying methods (AD, VD, MD, and FD) on the flavor components of fresh ginger slices were compared and showed that the MD retained the most amount of flavor components, followed by VD and AD (Ding et al., Citation2012). Although there are studies focusing on the effects of different drying methods on flavor profiles, research specifically about T. indicum remains unexplored. Therefore, to explore the volatile profile change of T. indicum in different drying methods, we have designed and adopted three commonly used drying methods, including AD, VD, and FD, to dehydrate T. indicum. Hence, the impacts of drying methods on flavor components of T. indicum would be compared and investigated. Moreover, the results would be used to recommend the most suitable dehydration processing in order to achieve better preservation quality of natural fresh flavors of T. indicum.
2. Materials and methods
Ascocarps of Tuber indicum, with original moisture content 76 ± 0.15% (wet base), was purchased from the local market in Kunming city, China and then refrigerated at 4°C until use. The samples were washed and cut to 5 mm slices prior to use.
2.1. Drying methods and process of T. indicum
2.1.1. Hot Air Drying
Hot air drying procedure used in this experiment was similar to that described by other researchers (Ferencz, Czukor & Cserhalmi, Citation2014; Kotwaliwale, Bakane & Verma, Citation2007; Li, Duan & Xu, Citation2014). Fresh evenly cut T. indicum slices were placed into an electronic heating air blowing dryer (Model 101-3A, Xinnuo Instrument Equipment Co. Ltd., Shanghai, China) and the dryer was heated to a temperature at 60°C and dried for 9 h.
2.1.2. Vacuum Drying
VD method was performed as described by Wu et al. with some modifications. Fresh evenly-cut T. indicum slices were placed into a vacuum dryer (Model DZF-6020, Shanghai Jinghong Equipment Co., Ltd., Shanghai, China) with a temperature at 50°C, under the vacuum pressure 90 kPa and dried for 12 h.
2.1.3. Vacuum Freeze-Drying
Fresh evenly cut T. indicum slices were frozen at a temperature at −24 ± 2°C for 24 h and the frozen samples were placed in the 12 L vacuum freeze dryer (Model, Labconco company, Kansas City, MS, USA) with heating plate temperature at 40°C, vacuum pressure 0.1 kPa and cold trap temperature at −83 ± 1°C (Pei et al., Citation2014).
2.1.4. Preparation of T. indicum powder
Upon drying to the moisture content of 5% by above mentioned three methods, that is AD, VD, and FD, the dried slices of T. indicum were stored in valve bags at room temperature. The slices were pulverized into powder by a super micro mill (Model FW100 Tianjin Taisite Equipment Co., Ltd., Tianjin, China), reaching the particle size of 200 mesh, and then were collected and used in GC-MS analysis and E-nose analysis.
2.2. Headspace solid-phase microextraction (HS-SPME) of volatile compounds
Dried samples and fresh samples were grinded adequately and weighed in a 20 ml vial with 0.05 g dry weight internal standard of 1-decanol solution (80 μg/mL) in methanol, and closed with a silicon cap. The cap was perforated for 40 min with a divinylbenzene/carboxen/polydimethylsiloxane (DVB/CAR/PDMS, 50/30 m/m) fiber holder (Supelco Ltd., Bellefonte, PA, USA) after 40 min equilibrating in a 60℃ water bath. The analytes were finally desorbed for 5 min at 250℃ in the GC injector in splitless mode.
2.3. GC-MS analysis of volatile compounds
In the analysis, the instrument for GC-MS was 7890A-5975C GC-MS (Agilent company, Santa Clara, CA, USA) with settings: HP-5MS capillary column (30 m × 0.25 mm i.d. and 0.25 μm film thickness) from (J&W Scientific, Folsom, CA, USA). Column temperature was initially set and held at 40℃ or 3 min, and then increased to 130℃ at 5 min, held for 3 min. In the second ramp, temperature increased to 200°C from 8 min to 15 min, and finally reached 250℃ at 20 min and held for 2 min. Helium was the carrier gas at a flow rate of 0.8 mL/min. Mass spectra were acquired in an electron impact mode. MS was taken at 70 eV ionization energy in the 25–450 amu mass range, and the ion source temperature was 200℃. The volatile compounds were tentatively identified by matching the mass spectra with the spectra of reference compounds in both the Wiley mass spectra library (6th edition) and the NIST/EPA/NIH mass spectra library (version 1.5a). The results from volatile analyses are provided in peak area counts of the compounds identified (Yang et al., Citation2016).
2.4. Electronic nose analysis
The flavor differences of fresh and dried powder samples of T. indicum were analyzed by an electronic nose detector (E-nose) equipped with a headspace auto-sampler (Fox 3000, Alpha M.O.S., Toulouse, France) comprising an array of 12 sensors (LY2/LG, LY2/G, LY2/AA, LY2/Gh, LY2/gCTL, LY2/gCT, T30/1, P10/1, P10/2, P40/1, T70/2, PA/2). Fresh T. indicum sample (1 g) and dried powder obtained by three drying methods (0.20 g per sample) were stored in the 20 mL of headspace vials from Supelco Inc. The E-nose sampling bottles were equilibrated under the temperature of 50°C for 10 min and then were introduced by the carrier gas, which was clean and dry air, with flow rate of 150 mL/min. The sampling gas volume was 2.5 mL. The sampling introduction was finished within 1s and the temperature of injection needle was 60°C. Parameter acquisition time was 120 s and lag time was 10 min. Sensor LY2/G sensitive to alcohols, ketones and aldehydes; LY2/AA sensitive to ketones; LY2/Gh sensitive to alcohols; LY2/gCT sensitive to ethanol; T30/1 sensitive to acid compounds, PA/2 sensitive to ketones sulfur compounds and acids (Oupathumpanont & Suwonsichon, Citation2013; Yao et al., Citation2015).
2.5. Data analysis
The data were analyzed by ANOVA with SPSS 18.0. The significant difference was defined as p < 0.05. Least significant difference-test (LSD) was used in multiple comparative analyses. In addition, multivariate statistical analysis was performed using simca software version 14.1. The output data from the E-nose was analyzed by using software (AlphaSoft version 3.0.0, Toulouse, France).
3. Results and discussion
HS-SPME-GC-MS analysis was performed to analyze the volatile flavor components of fresh and processed T. indicum by AD, VD and FD. From the results shown in , total of 45 volatile compounds were detected from the fresh T. indicum samples, among which 21 compounds with the AD samples, 20 compounds with the VD samples and 18 compounds with the FD samples.
Table 1. Volatile compounds from HS-SPME-GS-MS analysis of fresh and three drying samples of T. indicum.
Tabla 1. Compuestos volátiles del análisis HS-SPME-GS-MS de muestras de T. indicum fresca y deshidratada por tres métodos.
3.1. Detection of volatile flavor components of fresh T. indicum
From the analysis of the volatile components in fresh T. indicum by HS-SPME -GC-MS, the results showed that the major chemical species were aldehydes (54.8%) and alcohols (31.4%). These results were illustrated in and they are similar to the study of Culleré et al. (Citation2013). The major flavor compounds in fresh T. indicum were compounds with C8 compounds, including 1-octen-3-ol, 3-octanol, n-octanol, and 3-octanone. The production of C8 compounds has been attributed to enzymatic degradation of unsaturated fatty acids; for instance, lipogenase existed in situ produces C8 compounds. Fresh T. indicum had the highest amount of aldehydes with low odor threshold, including 3-methylbutanal, hexanal, benzaldehyde, benzeneacetaldehyde, and nonanal. Other volatile components were detected, including phenethyl alcohol, ethyl hexanoate, ethyl hexadecanoate, and 2-pentylfuran. In addition to aforementioned C8 compounds, a few acids constitute the flavor of fresh T. indicum, showed in .
Figure 1. Flavor component analysis for fresh and three drying samples of T. indicum.
Note: AD, hot air drying; VD, vacuum drying; FD, vacuum-freeze drying.
Figura 1. Análisis de componentes de sabor en muestras de T. indicum fresca y deshidratada por tres métodos.
Nota: AD, secado al aire caliente; VD, secado al vacío; FD, liofilizado al vacío.
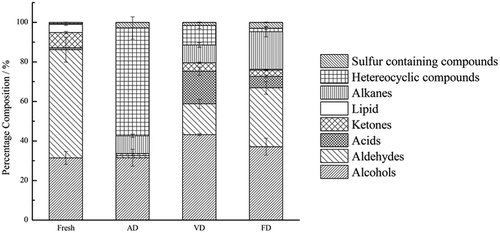
3.2. Summary of volatile profiles of T. indicum from different drying methods
HS-SPME-GC-MS is one of the most commonly used techniques in analysis of volatile compounds because chemical profiles from the headspace extraction often represent flavor components. The volatile compounds from our dehydration study of T. indicum in three drying methods (FD, AD, and VD) were summarized in the following and in .
3.2.1. Vacuum-Freeze Drying
There were 18 volatile components characterized in FD samples, which were mainly alcohols (37.1%), aldehydes (29.8%), and alkanes (18.7%), counting on more than 85% of the total flavor compounds (). The major single compounds identified were phenethyl alcohol (26.1%), hexanal (15.3%), 2,2,4,6,6-pentamethylheptane (12.7%), and 1-octen-3-ol (10.1%).
3.2.2. Hot Air Drying
Heterocyclic volatiles (54.5%) were the major compounds identified in the AD, and they counted for more than half of the total 21 compounds (). The others were alcohols (31.6%), aldehydes (1.1%). The major compounds were 2-furanmethanol (19.5%), 2-pyrrolinone (17.7%), phenethyl alcohol (16.0%), 2,3-butanediol (12.7%), and 1-octen-3-ol (2.6%).
3.2.3. Vacuum Drying
There was total of 20 compounds identified, and the alcohol (43.2%) was the most abundant family, followed by volatile acids (16.5%) and aldehydes (15.6%). Similar to the results of FD sample, the major compounds were phenylethyl alcohol (41.5%) and the others included butanoic acid (16.5%), 1-butyl-1H-pyrrole (7.3%), hexanal (5.7%), and dodecane (5.1%). There was no 1-octen-3-ol identified and also the contents of other C8 compounds were very low. Although the boiling point of “mushroom flavor “1-octen-3-ol is 175°C at 101 kPa and 171°C at 90 kPa, it is a typical volatile compound particularly found in fungi. The possible explanation that 1-octen-3-ol was not in the profile of VD is that it is a volatile compounds and easily vaporized. Hence it is evaporated in VD that was a prolonged process (12 h) at vacuum of 90 kPa and heated (50°C) temperature.
3.3. Influence of drying methods on the volatile flavor components of T. indicum
From , it could be concluded that the three drying methods had significant influences on the flavor of T. indicum. Chemical analysis showed that after drying, most contents of alcohols, aldehydes, ketones, and esters were reduced with different extent.
There was no significant difference between fresh T. indicum and FD samples of 1-octen-3-ol concentration (p > 0.05), but significantly higher than that of AD samples (p < 0.05), and there was no 1-octen-3-ol detected from VD samples, suggesting that the FD had the most advantage among three drying methods in the retention characteristic flavor compound of T. indicum. Because the major flavor compounds in fresh T. indicum are C8 compounds, such as 1-octen-3-ol, 3-octanol, 1-octanol, and also 3-octanone, it is important to identify and profile these C8 components for flavor analyses. The HS-SPME-GC-MS data also showed the overall content of other C8 compounds, including alcohols, such as 3-octanol, (E)-2-octenal, 3-octanone, and compounds with short chain such as 1-hexanol and 1-heptanol were greatly decreased, even reached the detection limit of the instrument. Hence, the drying process had a direct impact on the loss of C8 volatile molecules by evaporation or reaction with other components. However, phenethyl alcohol content in drying samples was significantly higher than that of fresh samples (p < 0.05). The unusual increase could be explained that phenethyl alcohol was the thermal Strecker degradation product of phenylalanine, which was enhanced by the heat of drying process (Cosmai, Summo, Caponio, Paradiso, & Gomes, Citation2013).
The drying process also resulted in the loss of aldehydes. The only aldehyde survived the AD samples is hexanal, whereas there were five aldehydes in the VD samples, including hexanal, 2-phenyl-2butenal, nonenal, benzaldehyde, and benzeneacetaldehyde, and there were six aldehydes characterized in the FD samples, 3-methylbutananl, hexanal, 2-phenyl-2-butenal, nonanal, benzaldehyde, and benzeneacetaldehyde. The aldehyde content in the FD samples was the most similar to fresh T. indicum, indicating a better retention quality of the fresh flavors. This phenomenon could be explained that the high drying temperature of AD facilitated the loss of large amount of fresh flavors, comparing to the low temperature drying process as in the FD. Therefore, the most important factor in preserving the fresh flavor of T. indicum is low temperature. Similar results were reported in the research of drying methods impacting of perfume profiles.
The content of long chain alkanes and heterocyclic compounds containing nitrogen increased significantly. Alkanes were majorly produced by lipid oxidation (Sucan & Weerasinghe, Citation2005). Heterocyclic compounds were formed by Maillard reaction in the drying process, which could produce oxygen-, nitrogen-, and sulfur-containing heterocyclic species (Sucan & Weerasinghe, Citation2005). There were ten major heterocyclic compounds generated in AD processing such as 2-furanmethanol (19.5%), 2-pyrrolidinone (17.7%), 2-acetylpyrrole (4.6%), 2-(5H)-furanone (2.6%), trimethylpyrazine (3.3%), 2-ethenyl-6-methylpyrazine (2.5%), 2-acetylfuran (1.8%), 2,3-dimethylpyrazine (0.8%), and dihydro-5-pentyl-2-(3H)-furanone (0.7%). In the VD samples, three major heterocyclic compounds were detected, including 1-butyl-1H-pyrrole (7.3%) and two minor ones, 2-(5H)-furanone (1.4%) and dihydro-5-pentyl-2-(3H)-furanone (1.2%). There was only one compound, 2-pentylfuran, which was a lipid oxidation product generated in the FD process with small content (1.7%) compared to other flavor compounds in the FD samples. High temperature in the AD process facilitated Maillard reaction, Strecker degradation and aldol condensation, which generated heterocyclic aroma compounds. In other words, the generation of some volatile compounds, such as pyrazine molecules, requires high temperature, and the drying temperature in the methods of VD and FD was too low to form these pyrazine products.
In summary, with HS-SPME-GC-MS technique, 45 volatile compounds were identified from fresh T. indicum. The effects of three different drying methods on these fresh volatile compounds were investigated. The flavor profile of drying T. indicum from FD processing was the closest to the fresh T. indicum, compared to the large amount of pyrazine compounds formed by AD processing and the great loss of aldehyde species in VD processing. Therefore, the FD is the most proper way among the three evaluated processes to preserve the original fresh flavor of T. indium in the presented study.
3.4. Principal component analysis of flavor components
Principal component analysis (PCA) is a multivariate statistical analysis method that transfers multiple indicators to a few comprehensive indicators by reducing dimensions. The intention of this method is data simplification and a clear demonstration of the relationships among variables. PCA is adopted to analyze the volatile composition. The object of data analysis is the volatile components of four samples mentioned above (samples from fresh T. indicum, and AD, VD and FD). The analysis results are illustrated in . The first three principal components accounted for 98.7% of the total variance, with values for PC1, PC2 and PC3 of 49.8%, 31.1% and 17.8%, respectively. Fresh T. indicum, AD, VD, and FD samples formed four clusters. Fresh T. indicum and three drying samples separate along PC1 where fresh T. indicum are located on negative PC1 while three drying samples occupy positive PC1 (. The three drying samples were similar along PC1, but there was clear separation on the PC2 or PC3 axis ((a,b)). Moreover, AD samples were obvious difference from VD, and FD samples. To analyze the variables responsible for the clustering, the loading plot of PCA was performed (Figure 2(c,d)). The results showed chemical composition contributing to the separation of drying samples were 2,3-Butanediol, 2-Furanmethanol, 2,3-Dimethylpyrazine, 2-acetylfuran, Trimethylpyrazine, 2-Ethenyl-6-methylpyrazine, 2-Acetylpyrrole, 2-Pyrrolidinone and 2,3-Dihydrothiophene, Phenethyl alcohol, 2-Phenyl-2-butenal, 2-Methyl-3-octanone, 3-Methylbutanoic acid, Tetradecane, 1-Butyl-1H-pyrrole, Dihydro-5-pentyl-2(3H)-furanone, 2(5H)-Thiophenone. Their intensities increased with duration of drying. 2,3-Butanediol, 2-Furanmethanol, 2,3-Dimethylpyrazine, 2-acetylfuran, Trimethylpyrazine, 2-Ethenyl-6-methylpyrazine, 2-Acetylpyrrole, 2-Pyrrolidinone and 2,3-Dihydrothiophene were markers of AD. VD was positively associated with Phenethyl alcohol, 2-Phenyl-2-butenal, 2-Methyl-3-octanone, 3-Methylbutanoic acid, Tetradecane, 1-Butyl-1H-pyrrole, Dihydro-5-pentyl-2(3H)-furanone, 2(5H)-Thiophenone. Fresh T. indicum samples were characterized by higher content of 1-Hexanol, 3-Octanol, 1-Octanol, 3-Methylbutanal, Benzaldehyde, Benzeneacetaldehyde, (E)-2-Octenal, 3-Octanone, 3-Octen-2-one, 2-Undecanone, Ethyl 3-methylbutanoate, Ethyl hexanoate, Isobutyl octyl phthalate, Ethyl hexadecanoate, Styrene.
Figure 2. Score plot of PC1 vs. PC2 (a) and PC1 vs. PC3 (b), loading plot of PC1 vs. PC2 (c) and PC1 vs. PC3 (d) for fresh and three drying samples of T. indicum.
Note: AD, hot air drying; VD, vacuum drying; FD, vacuum-freeze drying.
Figura 2. Diagrama de puntos de PC1 vs. PC2 (A) y PC1 vs. PC3 (B), diagrama de carga de PC1 vs. PC2 (C) y PC1 vs. PC3 (D) para muestras de T. indicum fresca y deshidratada por tres métodos.
Nota: AD, secado al aire caliente; VD, secado al vacío; FD, liofilizado al vacío.
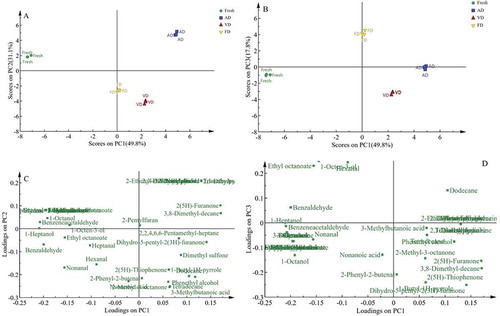
3.5. E-nose analysis
E-nose technology provides comprehensive information about volatile profiles, which is commonly used to differentiate and analyze complicated flavor components. The volatile profile of samples subjected to the three drying methods and fresh samples were also performed by Fox 3000 E-nose. The original response data generated by 12 sensors of E-nose were collected and transformed to radar graphs, as shown in . Generally, the similar shape of these radar graphs implied some similarities among these samples. The response values of LY2/LG, T30/1, P10/1, P10/2, P40/1, T70/2 and PA/2 sensors to fresh T. indicum and three drying samples were positive, LY2/G, LY2/AA, LY2/Gh, LY2/gCTL, LY2/gCT were negative. The tends of connect shapes were different between fresh sample and three drying samples, and three drying samples were in substantial agreement.
Figure 3. Radar map of fresh and three drying samples of T. indicum.
Note: Sensor: 1: LY2/LG; 2: LY2/G; 3: LY2/AA; 4: LY2/Gh; 5: LY2/gCTL; 6: LY2/gCT; 7: T30/1; 8: P10/1; 9: P10/2; 10: P40/1; 11: T70/2; 12: PA/2. AD, hot air drying; VD, vacuum drying; FD, vacuum-freeze drying.
Figura 3. Mapa radar de muestras de T. indicum fresca y deshidratada por tres métodos.
Nota: Sensor: 1: LY2/LG; 2: LY2/G; 3: LY2/AA; 4: LY2/Gh; 5: LY2/gCTL; 6: LY2/gCT; 7: T30/1; 8: P10/1; 9: P10/2; 10: P40/1; 11: T70/2; 12: PA/2. Nota: AD, secado al aire caliente; VD, secado al vacío; FD, liofilizado al vacío.
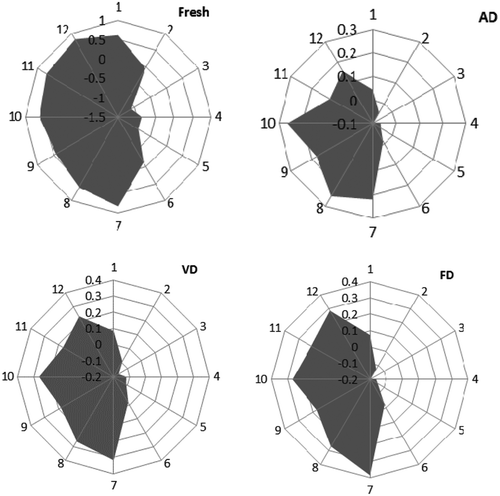
Compared with the fresh T. indicum, the response values of sensor decreased of the three drying samples, indicating that the drying caused the loss of fresh T. indicum flavor. The response values of LY2/G, LY2/AA, LY2/Gh, LY2/gCT of FD samples was higher than AD samples, those sensors (LY2/G, LY2/AA, LY2/Gh, LY2/gCT) sensitive with ethanol, aldehyde and ketone, so the result indicated that content of ethanol, aldehydes, ketones of FD samples higher than AD samples. The flavor profiles generated by E-nose were in accordance with the experimental data detected by GC–MS.
3.6. Principle component analysis from E-nose data
The PCA from E-nose data of the flavor compounds of fresh and three different drying processed samples of T. indicum was conducted on a E-nose software and the volatile flavor component analysis of the original response values was performed for principle component analysis. As illustrated in , the cumulative variance contribution rates of PC1 and PC2 of T. indicum samples were 99.505% and 0.466%, respectively, which indicated that PC1 and PC2 had a large amount of information and could reflect the whole information of the samples. The discriminate index (DI) is the representation value of the degree of sample discrimination provided by data statistics software of E-nose. The DI value of is 98, indicating that the electronic nose can significantly differentiate samples of different drying mothdes. The volatile odor difference from fresh samples and samples of three drying methods is apparent, and this difference can be displayed on the platform of PC1 and PC2. The flavor of the fresh T. indicum is the closest to that of the FD samples, which indicates proximity of flavor similarity between the two samples and is also consistent with the results detected by HS-SPME-GC-MS.
Figure 4. Principal component analysis for fresh and three drying samples of T. indicum.
Note: AD, hot air drying; VD, vacuum drying; FD, vacuum-freeze drying.
Figura 4.Análisis de los componentes principales de muestras de T. indicum fresca y deshidratada por tres métodos.
Nota: AD, secado al aire caliente; VD, secado al vacío; FD, liofilizado al vacío.
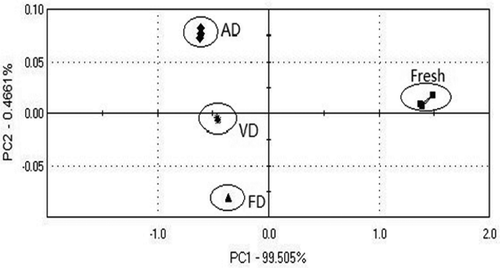
4. Conclusions
This study compared the volatile components of fresh, VD, AD and FD samples by using HS-SPME-GC-MS. In fresh T. indicum, 45 volatile components were detected, among which aldehydes and alcohols were prevalent in fresh T. indicum. The main flavor components included C8 compounds (1-octen-3-ol, 3-octanol, n-octanol, 3-octanone), 2-methylbutanal and hexanal. After dehydrating, C8 compounds, aldehyde and ester components reduced significantly, while alkanes, heterocyclic and sulfur volatile components were produced. Compared to AD and VD samples, flavor components of FD samples were the most similar to the fresh T. indicum, which maintained the main volatile components, aldehydes and alcohols and C8 molecules. The alkanes and heterocyclic content of AD samples were in the largest amount because of Maillard, Strecker and oxidation reactions. According to the analysis of E-nose, different T. indicum samples could be distinguished and the flavor profile of FD samples was similar to the fresh T. indicum. The volatile components of FD, AD and VD samples were significantly different. Therefore, FD was the most suitable processing method in preparation of dehydrated T. indicum in order to keep the flavor quality.
Acknowledgments
The authors acknowledge financial support from China Agriculture Research System (CARS-20) and the Priority Academic Program Development of Jiangsu Higher Education Institutions (PAPD).
Disclosure statement
No potential conflict of interest was reported by the authors.
Additional information
Funding
References
- Aprea, E., Romano, A., Betta, E., Biasioli, F., Cappellin, L., Fanti, M., & Gasperi, F. (2015). Volatile compound changes during shelf life of dried Boletus edulis: Comparison between SPME-GC-MS and PTR-ToF-MS analysis. Biological Mass Spectrometry, 50(50), 56–64.
- Bhattacharya, M., Srivastav, P. P., & Mishra, H. N. (2015). Thin-layer modeling of convective and microwave-convective drying of oster mushroom (Pleurotus ostreatus). Journal of food science and technology, 52(4), 2013–2022.
- Cosmai, L., Summo, C., Caponio, F., Paradiso, V. M., & Gomes, T. (2013). Influence of the thermal stabilization process on the volatile profile of canned tomato‐based food. Journal of Food Science, 78(12), C1865–C70.
- Culleré, L., Ferreira, V., Venturini, M. E., Marco, P., & Blanco, D. (2013). Potential aromatic compounds as markers to differentiate between Tuber melanosporum and Tuber indicum truffles. Food Chemistry, 141(1), 105–110.
- Dahham, S. S., Al-Rawi, S. S., Ibrahim, A. H., Majid, A. S. A., & Majid, A. M. S. A. (2016). Antioxidant, anticancer, apoptosis properties and chemical composition of black truffle Terfezia claveryi. Saudi Journal of Biological Sciences, 12(8), 98–103.
- Ding, S. H., An, K. J., Zhao, C. P., Li, Y., Guo, Y. H., & Wang, Z. F. (2012). Effect of drying methods on volatiles of Chinese ginger (Zingiber officinale Roscoe). Food Bioprod Processing Transac Insti, 90(C3), 515–524.
- Ferencz, S., Czukor, B., & Cserhalmi, Z. (2014). Combined with hot-air drying and compared with freeze- and hot-air drying by the quality of the dried apple product. Periodica Polytechnica Chemical Engineering, 58(2), 111–116.
- Guiné, R. P., & Barroca, M. J. (2012). Effect of drying treatments on texture and color of vegetables (pumpkin and green pepper). Food and Bioproducts Processing, 90(1), 58–63.
- Guo, X. H., Xia, C. Y., Tan, Y. R., Chen, L., & Ming, J. (2014). Mathematical modeling and effect of various hot-air drying on mushroom (Lentinus edodes). Journal of Integrative Agriculture, 13(1), 207–216.
- Huang, L. L., Zhang, M., Wang, L. P., Mujumdar, A. S., & Sun, D. F. (2012). Influence of combination drying methods on composition, texture, aroma and microstructure of apple slices. Lwt - Food Science and Technology, 47(1), 183–188.
- Kotwaliwale, N., Bakane, P., & Verma, A. (2007). Changes in textural and optical properties of oyster mushroom during hot air drying. Journal of Food Engineering, 78(4), 1207–1211.
- Kumar, A., Singh, M., & Singh, G. (2013). Effect of different pretreatments on the quality of mushrooms during solar drying. Journal of Food Science and Technology, 50(1), 165–170.
- Li, W., Duan, J., & Xu, J. (2014). Different drying methods on nutrients and antioxidant activities of Agaricus bisporus. Advanced Sciences and Engineering, 6(2), 35–39.
- Maga, J. A. (1981). Mushroom flavor. Journal of agricultural and food chemistry, 29(1), 1–4.
- Miao, Y., Chen, C., Ma, Q., Wang, Y., Zhang, X., Guo, F., … Yong, B. (2014). Effects of soaking with natural additives in combinations with vacuum or modified atmosphere packaging on microbial populations and shelf life of fresh truffles (Chinese Tuber indicum). Journal of Food Science, 79(10), M2040–M2047.
- Oupathumpanont, O., & Suwonsichon, T. (2013). Change of sensory characteristic of fermented rice flour and fermented rice noodle (kanom-jeen) during fermentation by lactobacillus plantarum a1. Journal Applied Sciences Research, 9(12), 6063–6071.
- Pei, F., Yang, W., Ma, N., Fang, Y., Zhao, L., An, X., … Hu, Q. (2016). Effect of the two drying approaches on the volatile profiles of button mushroom (Agaricus bisporus) by headspace GC–MS and electronic nose. LWT - Food Science and Technology, 72, 343–350.
- Pei, F., Yang, W., Shi, Y., Sun, Y., Mariga, A. M., Zhao, L.-Y., … Hu, Q. (2014). Comparison of freeze-drying with three different combinations of drying methods and their influence on colour, texture, microstructure and nutrient retention of button mushroom (Agaricus bisporus) slices. Food and Bioprocess Technology, 7, 702–710.
- Qiao, F., Fang, C., Huang, L., & Zhang, S. (2013). The effect of different heating patterns on vacuum freeze-drying of litchi pulp. Journal of Food Process Engineering, 36(4), 407–411.
- Saxena, A., Maity, T., Raju, P. S., & Bawa, A. S. (2012). Degradation kinetics of colour and total carotenoids in jackfruit (Artocarpus heterophyllus) bulb slices during hot air drying. Food and Bioprocess Technology, 5(2), 672–679.
- Sucan, M. K., & Weerasinghe, D. K. (2005). Process and reaction flavors: An overview. In D. K. Weerasinghe & M. K. Sucan (Eds.), Process and Reaction Flavors (Vol. 905, pp. 1–23). New York, NY: Ameican Chemical Society.
- Vega-Gálvez, A., Ah-Hen, K., Chacana, M., Vergara, J., Martínez-Monzó, J., García-Segovia, P., … Di, S. K. (2012). Effect of temperature and air velocity on drying kinetics, antioxidant capacity, total phenolic content, colour, texture and microstructure of apple (var. Granny Smith) slices. Food Chemistry, 132(1), 51–59.
- Yang, W., Yu, J., Pei, F., Mugambi, A., Ma, N., Fang, Y., & Hu, Q. (2016). Effect of hot air drying on volatile compounds of flammulina velutipes detected by hs-spme-gc-ms and electronic nose. Food Chemistry, 196(1), 860–866.
- Yao, Y., Pan, S., Fan, G., Dong, L., Ren, J., & Zhu, Y. (2015). Evaluation of volatile profile of sichuan dongcai, a traditional salted vegetable, by spme-gc-ms and e-nose. LWT - Food Science and Technology, 64(2), 528–535.