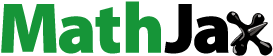
ABSTRACT
The mixtures of blue cornmeal and orange bagasse were processed in a single screw laboratory extruder. We used an experimental design, central composite rotatable, where the factors were as follows: extruder die temperature (153–187°C), feed moisture (146.4–213.6 g/kg), and orange bagasse concentration (32.7–167.3 g/kg). The results were analyzed by surface methodology response to evaluate the effect of these variables on the expansion index, bulk density, penetration force, specific mechanical energy, water absorption, and water solubility index. All independent variables had an effect on the evaluated responses (p ≤ 0.05). The highest expansion index was obtained at a feed moisture content of 146.4 g/kg, orange bagasse (100 g/kg), and 170°C at the exit die. The use of blue maize and orange bagasse can be an alternative to increase the added value of these two raw materials, and to improve the nutritional quality of extruded products ready to eat.
RESUMEN
Mezclas de maíz azul y bagazo de naranja fueron procesadas en un extrusor de laboratorio de un solo tornillo, el diseño de experimentos que se utilizó fue un central compuesto rotable, donde los factores a evaluar fueron la temperatura del dado de salida (153–187°C), el contenido de humedad (146.4–213.6 g/kg) y la concentración de bagazo de naranja (32.7–167.3 g/kg), una vez obtenidos los productos se les determinó el índice de expansión, la densidad aparente, la fuerza de penetración, la energía mecánica específica, el índice de absorción de agua e índice de solubilidad en agua. Todos los factores evaluados presentaron efecto significativo (p ≤0.05) sobre las respuestas evaluadas. El mayor índice de expansión se obtuvo a un contenido de humedad de 146.4 g/kg, una concentración de bagazo de naranja del 100 g/kg y una temperatura del dado de salida de 170°C. El uso de maíz azul y bagazo de naranja pueden ser una alternativa para desarrollar productos extrudidos directamente expandidos, e incrementar el valor agregado de estas materias primas.
1. Introduction
Snack foods are widely consumed regardless of social status, age or gender. Currently, the production of snacks in Mexico is estimated at around 450,000 tons, with annual per capita consumption of 3.8 kg, and an annual market value of 3.419 billion. Snacks fried wheat flour and corn represents 36% of the total domestic production, followed by potato chips (27%), corn chips and tostadas (19%), baked and puffed products (11%), peanuts and seeds (4%), and pork rinds (3%) (CANACINTRA, Citation2017). Extrusion is a versatile and efficient processing method, used for the manufacture of various products such as snacks, nixtamalized flour, pet, cattle and fish food; using raw materials such as maize, rice, wheat, potato flour, taro, bean flour, chickpeas and peas, among others (Berrios, Wood, Whitehand, & Pan, Citation2004; Mensa-Wilmot, Phillips, Lee, & Eitenmiller, Citation2003; Rocha-Guzmán et al., Citation2006; Rodríguez-Miranda et al., Citation2011). Mexico has about 59 different varieties of maize:yellow, red, purple, black, and blue. There are few studies in which the pigmented maizes are used as raw materials to be processed by extrusion. Most of these studies are aimed at nixtamalized flour production, mainly evaluating the changes in terms of phenolic and anthocyanins content after processing (Aguayo-Rojas et al., Citation2012; Mora-Rochin et al., Citation2010). Zazueta-Morales, Martínez-Bustos, Jacobo-Valenzuela, Ordorica-Falomir, and Paredes-López (Citation2001), developed a directly expanded snack from blue maize, fortified with calcium, while Camacho-Hernández et al. (Citation2014) developed a third generation snack with a blend of blue maize and starch; Navarro-Cortez et al. (Citation2016), developed extruded products with blue maize and different orange bagasse concentrations. In recent years, studies on waste obtained from fruits and vegetables processing have increased in popularity, because these by-products are a promising source of compounds which can be used because of their technological and nutritional properties. Waste worldwide obtained from citrus fruits, has been estimated at more than 15 million tons, which represents a serious environmental problem. This waste consists generally of the skin (albedo and flavedo), seeds and fruit pulp remaining after juice extraction (Laufenberg, Kunz, & Nystroem, Citation2003). One of the advantages of this type of waste is its content of dietary fiber, and when compared with other fiber sources such as cereals, it has a greater amount of soluble fiber (Gorinstein et al., Citation2001; Larrea, Chang, & Martínez-Bustos, Citation2005). The objective of this research was to evaluate the effect of the extrusion temperature, feed moisture and concentration of orange bagasse on the physicochemical and sensory properties of directly expanded extruded products, made from blends of blue maize and orange bagasse using a central composite rotatable design.
2. Materials and methods
2.1. Raw materials
Blue maize (Zea mays L.) obtained from a local market at Actopan, Hidalgo, Mexico. The orange bagasse was obtained from an establishment that sells fresh juice in Pachuca city, Hidalgo, Mexico.
2.2. Obtaining flour
Blue maize was milled in a turbine mill (pulvex, p200, DF, Mexico City). Orange bagasse was dehydrated at 67°C for 12 h in an oven with forced air circulation (Thermolyne 9000, USA), thereafter was ground in a blender (Oster, model BPST 02-b, USA) and sieved to a 120 µm particle size. Orange bagasse flour was mixed with blue cornmeal at different concentrations according to the experimental design; the obtained blends were adjusted at different feed moistures with distilled water ().
Table 1. Experiment design, rotatable composite central, α = 1.682.
Tabla 1. Diseño de experimentos central compuesto rotable, α = 1.682.
2.3. Extrusion process
After setting the desired moisture content, the samples were fed to a single screw laboratory extruder (Brabender Instruments Inc., model 20DN/8–235-00, CW, Germany) at a feed rate of 30 rpm/min. The extruder barrel is divided into two heating zones that kept a constant temperature of 80 and 120°C, respectively. The third zone, which corresponds to the matrix and die, was varied according to the experimental design. The screw speed was kept constant at 170 rpm, it was used a die with an outlet diameter of 3 mm and a screw with a compression ratio of 3:1.
2.4. Characterization of extruded products
2.4.1. Expansion index
The expansion index (EI) of the extruded products was calculated by dividing their diameter by the inner diameter of the exit die. 20 determinations were performed per treatment.
2.4.2. Bulk density
The bulk density (BD) (kg/m3) was calculated by dividing the weight of the extruded piece, by its apparent volume (m3). Apparent volume was obtained as follows:
where d (m) is the diameter of the extruded product and h (m) is the average length.
2.4.3. Penetration force
The force required (PF) to penetrate the expanded product was measured with a texture Analyzer TA-TX2 (Stable Micro Systems, Ltd., UK). Extruded samples were placed horizontally on a platform and penetrated with a 2 mm cylindrical flat tip probe. Probe descent rate was 2 mm s−1, and maximum penetration distance was 3 mm. Thirty measurements were taken per treatment and values were reported as newtons (N).
2.4.4. Absorption and water solubility index
The water absorption index (WAI) and water solubility index (WSI) were determined according to the method proposed by Anderson, Conway, Pfeifer, and Giffin (Citation1969), modifying the weight of sample 3 to 1 g.
2.4.5. Specific mechanical energy
The specific mechanical energy (SME) was calculated using the torque values (N*m), the screw speed (vt, rpm), and the feed flow (F, g/min) according to the next equation (Batterman-Azcona, Lawton, & Hamaker, Citation1999).
2.4.6. Sensory analysis
The hedonic sensory evaluation was performed with 80 untrained students of the Food Chemistry Degree of the Autonomous University of Hidalgo State (UAEH), 42 men and 38 women, age ranging between 19 and 23 who usually eat snacks. The students evaluated 3 extruded snacks with different orange bagasse content (A = 60, B = 100, and C = 140 g/kg). The evaluated parameters in the extruded snacks were color, texture, flavor (orange and bitter) and overall acceptability using a 7-point hedonic scale (where 1 = extremely unpleasant to 7 = extremely pleasant). Panelists rinsed their mouth with water after tasting each sample.
2.5. Experimental design and statistical analysis
A central composite rotatable experimental design with three independent variables were used: feed moisture, die temperature and orange bagasse concentration (). The dependent variables were as follows: EI, BD, penetration force (PF), SME, WAI, and WSI. The data were analyzed and adjusted to a second order regression model, and the regression coefficients were obtained. The response surface graphs were obtained using the Design Expert 7.1.6 Statistical Package (Stat-Ease, Inc., MN, USA). The significance of each term in the regression equation was examined by analysis of variance (ANOVA) for each response. To relate each answer with extrusion temperature, feed moisture, and orange bagasse concentration, the following response surface model with linear terms, quadratic and interactions was used.
3. Results
3.1. Calculated coefficients of the adjusted model
shows the coefficients of multiple linear regression and the coefficients of determination of models for the evaluated responses. These regression coefficients show that the extrusion temperature in the linear term presented statistically significant effect on EI, PF, WAI, and SME. Feed moisture and orange bagasse concentration showed statistically significant effects on the linear term for all the evaluated responses. The temperature–moisture interaction did not show a significant effect on WSI, while the temperature–orange bagasse concentration interaction presented significant effect on all the evaluated responses. Moisture–orange bagasse concentration interaction showed no significant effect on PF. Temperature, moisture, and orange bagasse concentration in their quadratic terms presented significant effect on all analyzed responses.
Table 2. Regression coefficients of the adjusted response surface models for the response variables of the extruded products of blue maize and orange bagasse.
Tabla 2. Coeficientes de regresión de los modelos ajustados de superficie de respuesta, para las variables de respuesta de los productos extrudidos de maíz azul y bagazo de naranja.
3.2. Characterization of extruded products
3.2.1. Expansion index (EI) and bulk density (BD)
) shows that the highest EI (2.82) at an orange bagasse concentration of 100 g/kg was obtained at 170°C and 146.4 g/kg moisture. In ), with feed moisture set at 180 g/kg, the highest EI was obtained at 187°C and the lowest orange bagasse concentration, and the lowest EI was obtained at high orange bagasse concentrations and high die temperatures. ) shows that the highest EI was obtained at low moistures, an orange bagasse content of 100 g/kg, and a temperature of 170°C. EI experimental values obtained for all tests run were within a range of 1.65 ± 0.081 to 2.82 ± 0.108. The expansion process in extruded products is complex; it involves dough characteristics such as elasticity and flow resistance, as well as the water steam pressure on each bubble generated within the dough. When the mechanical shearing into the extruder exceeds the shearing tolerance of the bubble surface, it breaks causing a decrease in the expansion (Wang, Ganjyal, Jones, Weller, & Hanna, Citation2005). The expansion of the extruded products is governed by a change in pressure. The melt dough coming out the extruder undergoes a pressure drop, causing the sudden evaporation of moisture, generating the expansion of the extruded product (Arhalias, Bouvier, & Legrand, Citation2003), besides transforming the dough into a rigid and brittle product, losing molecular mobility and moisture. Oke, Awonorin, Asiedu, and Aiyedun (Citation2012) studied the effect of moisture content on EI and concluded that decreasing the moisture content increases the force necessary for conveying the melt dough within the barrel, exerting greater pressure on the die, allowing a greater expansion of the extruded product. The EI decrease when increasing the content of orange bagasse, this is due to a dilution effect on the total starch content, affecting the elastic properties of the matrix (Delgado-Nieblas et al., Citation2012; Robin, Bovet, Pineau, Schuchmann, & Palzer, Citation2011; Yağci & Göğüş, Citation2008). Robin et al. (Citation2012) reported a decrease in the EI values, due to a breakdown of air bubbles when their membrane becomes thinner and reaches bran particles. The breaking of air bubbles may be due to a weak or no interaction between the starch and bran molecules at the interface; similar postulates have been published by Moraru and Kokini (Citation2003), mentioning that fiber molecules alter the continuous dough structure preventing its deformation during expansion, since the fibers align as the flow into the extruder increases the mechanical resistance. The decrease in EI after 170°C has been related to an excessive breaking of the molecules that make up the starch, which weakens the network formed inside the extruded product (Meng, Threinen, Hansen, & Driedger, Citation2010). El-Dash (Citation1981) reported that the decrease in the EI at high temperatures could be due to the fact that water evaporation is very violent, breaking the structure and decreasing the expansion.
Figure 1. Response surface graphs for expansion index and bulk density. (a) Die temperature and feed moisture effect on the expansion index at a concentration of 100 g/kg orange bagasse. (b) Effect of the orange bagasse–temperature interaction on the expansion index at a moisture feed of 160 g/kg. (c) Effect of the orange bagasse–moisture feed interaction on the expansion index at a die temperature of 170°C. (d) Die temperature and moisture feed effect on the bulk density at a concentration of 100 g/kg of orange bagasse. (e) Effect of the orange bagasse–die temperature interaction at a moisture feed of 160 g/kg on the bulk density. (f) Effect of the orange bagasse–moisture feed interaction at a die temperature of 170°C on the bulk density.
Figura 1. Gráficos de superficie de respuesta para el índice de expansión y la densidad aparente. (a) Efecto de temperatura y humedad sobre el índice de expansión a una concentración de 100 g/kg de bagazo de naranja. (b) Efecto de la interacción temperatura-bagazo de naranja sobre el índice de expansión a un contenido de humedad del 160 g/kg. (c) Efecto de la interacción humedad-bagazo de naranja sobre el índice de expansión a una temperatura de 170°C. (d) Efecto de temperatura del dado y humedad de alimentación sobre la densidad aparente a una concentración de 100 g/kg de bagazo de naranja. (e) Efecto de la interacción temperatura del dado-naranja bagazo a un contenido de humedad del 160 g/kg sobre la densidad aparente. (f) Efecto de la interacción humedad de alimentación-bagazo naranja a una temperatura de 170°C, sobre la densidad aparente.
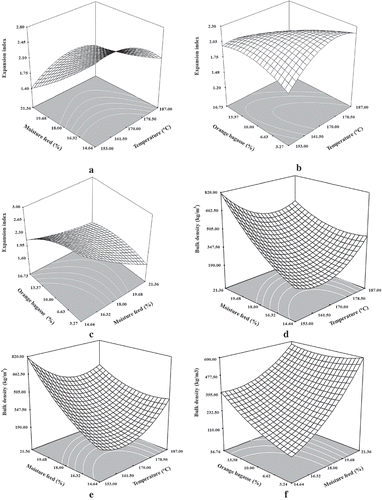
) shows the effect of the variables die temperature and feed moisture in BD at a fixed content of 100 g/kg of orange bagasse, as the feed moisture increases BD increases. At high feed moisture contents and increasing the extrusion temperature BD decreases, while at low feed moisture contents and increasing the extrusion temperature BD increases. ) shows an increase in BD by increasing the orange bagasse content and the extrusion temperature, to a constant moisture of 180 g/kg, while in ) the effect of feed moisture and orange bagasse content on BD at a temperature of 170°C is presented, observing an increase in BD due to orange bagasse and feed moisture. The BD values for all treatments are between 182.70 ± 22.95 and 594.9 ± 68.70 kg/m3, the highest BD obtained was for the product that presented the lowest EI, while the lowest one was obtained for the product with the highest EI. The decrease in BD as EI increases is due to an increase in the extruded product volume, but with a lower weight. Sacchetti, Pittia, and Pinnavaia (Citation2005) reported a decrease in BD and hardness of the extruded products, increasing the extrusion temperature. Dehghan-Shoar, Hardacre, and Brennan (Citation2010) reported that products containing fiber have the ability to absorb water, resulting in products with higher BD and lower EI, generating more compact products. Similar effects were reported for extruded products incorporating okara (Rinaldi, Ng, & Bennink, Citation2000). Meng et al. (Citation2010) reported an increase in BD by increasing the feed moisture, as well as an inverse relationship between EI and BD.
3.2.2. Penetration force (PF)
) shows the effect of temperature and feed moisture on the PF at a content of 100 g/kg orange bagasse. In this figure it can be seen that the PF increases when increasing the feed moisture and increases when increasing the extrusion temperature. ) shows the effect of orange bagasse in PF with a moisture content of 180 g/kg, which shows that the highest PF is obtained with high levels of orange bagasse and high temperatures. The hardness of the different products varied from 19.21 ± 1.355 to 48.86 ± 6.023 N. The hardness of an extruded product is inversely related to EI and directly to BD. The relationship of hardness with EI is due to the fact that more expanded products have pores with thinner walls, decreasing the force necessary to break the product (Pérez-Navarrete, González, Chel-Guerrero, & Betancur-Ancona, Citation2006), conversely, slightly expanded products have a higher number of pores per unit volume, increasing the pore thickness, fiber addition has been reported to increase the hardness of extruded products, because it interferes with the formation and growth of air bubbles (Ainsworth, Íbanoğlu, Plunkett, Íbanoğlu, & Stojceska, Citation2007). The decrease in hardness due to the effect of the orange bagasse content agrees with that reported by Altan, McCarthy, and Maskan (Citation2008), who used tomato peel as a source of fiber.
Figure 2. Graph of response surface for the penetration force. (a) Die temperature and moisture feed effect, on the force penetration at a concentration of 100 g/kg orange bagasse. (b) Effect of the orange bagasse–die temperature interaction, on the force penetration at a moisture feed 160 g/kg.
Figura 2. Gráfico de superficie de respuesta para la fuerza de penetración. (a) Efecto de temperatura del dado y humedad de alimentación a una concentración de 100 g/kg de bagazo de naranja sobre la fuerza de penetración. (b) Efecto de la interacción bagazo naranja-temperatura del dado a un contenido de humedad del 160 g/kg sobre la fuerza de penetración.
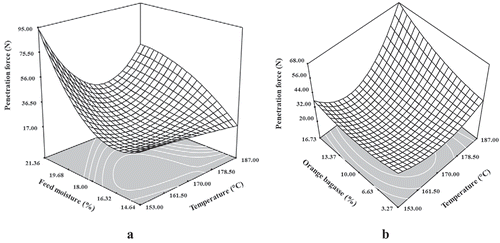
3.2.3. Specific mechanical energy (SME)
) shows the effect of the die temperature and the feed moisture on SME at a concentration of 100 g/kg orange bagasse, observing that at low temperatures (153°C) and high temperatures (187°C) SME increases with increasing feed moisture from 146.4 to 213.6 g/kg. By increasing the extrusion temperature from 153 to 170°C, the SME increases, >170°C the SME decreases. The highest values of SME were obtained both at low temperatures (153°C) and at high temperatures (187°C), at the highest moisture content tested. When the feed moisture is increased there is a greater amount of water available to be bound by the starch or the pectins present in the orange bagasse increasing the viscosity of the mixture. The effect of the temperature on SME could be related to the degree of gelatinization of the starch, where possible to 170°C the greater degree of gelatinization of the starch is obtained, higher temperatures possibly have an impact on the viscosity of the dough, decreasing the torque in the extruder motor and SME (Altan et al., Citation2008). The SME applied to the extruded material plays an important role in the conversion of the starch, greater SMEs have usually been related to a higher degree of starch gelatinization (Meng et al., Citation2010). ) shows the effect of the extrusion temperature and the concentration of the orange bagasse on SME at a moisture content in the mixture of 180 g/kg. The figure shows an increase in SME by increase the orange bagasse content and a decrease by increase the temperature of the die. The increase in the SME due to the concentration of orange bagasse is due to an increase in the viscosity of the dough, which can be attributed to the presence of thickening agents in the orange bagasse such as pectins, which have the ability to bind water and increase the viscosity of the mixture, also during the extrusion process, components such as cellulose, undergo modifications due to the mechanical shearing, exposing the hydrophilic groups of the cellulose to water, increasing the sites of binding. ) shows the effect of feed moisture and orange bagasse on SME at a temperature of 170°C. Observing an increase in the SME at the lowest moisture tested and increasing the orange bagasse content, observing similar results with low concentrations of orange bagasse as the moisture content increases. The lowest SME was obtained at a temperature of 170°C, 146.4 g/kg feed moisture, and 100 g/kg orange bagasse concentration.
Figure 3. Graph of response surface for the specific mechanical energy. (a) Effect of the temperature die and moisture feed at a concentration of 100 g/kg of orange bagasse. (b) Effect of the die temperature–orange bagasse interaction at a feed moisture of 160 g/kg. (c) Effect of the moisture feed-orange bagasse interaction at a temperature of 170°C.
Figura 3. Gráfico de superficie de respuesta para energía mecánica específica. (a) Efecto de la temperatura del dado y humedad de alimentación sobre el índice de expansión a una concentración de 100 g/kg de bagazo de naranja. (b) Efecto de la interacción temperatura del dado-bagazo naranja a un contenido de humedad de 160 g/kg. (c) Efecto de la interacción bagazo de naranja-humedad de alimentación sobre la energía mecánica específica a una temperatura de 170°C.
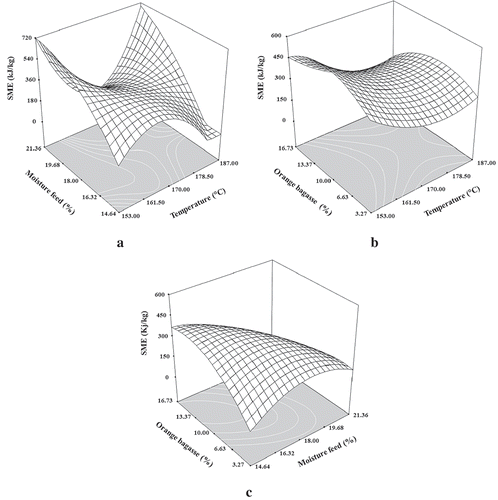
3.2.4. Water absorption index and water solubility index (WAI and WSI)
) shows the effect of the interaction between the extrusion temperature and the feed moisture at a 100 g/kg orange bagasse content, in that figure it is seen that by increasing the moisture content at low temperatures, the WAI increases, as well as by increasing the extrusion temperature at low moisture contents. At 213.6 g/kg moisture content over the entire range of studied temperatures, the WAI decreases. The increase of the WAI when increasing the feed moisture is due to the water lubricating effect; decreasing the viscosity, the residence time and mechanical shearing in the dough, reducing the starch degradation. Water acts as a plasticizer in polymers, promoting the mobility of their chains, facilitating deformation and fluidity, which leads to a decrease in viscosity (Lewicki, Citation2004; Roos & Karel, Citation1991). ) shows the effect of feed moisture and orange bagasse concentration at 170°C, in which an increase in WAI is observed by increasing the orange bagasse content at low moistures. At low concentrations of orange bagasse (32.7 g/kg) and as the moisture content increases, water absorption capacity is favored. The WAI values obtained are in the range of 3.66 ± 0.110 and 5.22 ± 0.14 g absorbed water/g sample bs. Some authors have reported a decrease in WAI by increasing the content of a fiber source due to a starch dilution effect (Robin et al., Citation2011), whereas in the present study WAI increases because the orange bagasse has pectins, which have the ability to form gels and absorb water. The ability of the fiber to absorb water depends on its physicochemical properties and the amount of soluble fiber it contains (Carrasco-Valencia, Acevedo-De la Cruz, Icochea-Alvarez, & Kallio; Zhang, Bai, & Zhang, Citation2011). Marín, Soler-Rivas, Benavente-García, Castillo, and Pérez-Alvarez (Citation2007), reported that in waste generated by the citrus processing industry, pectin content is in the range of 250–300 g/kg of total fiber and that the water absorption capacity increases with the amount of soluble fiber. Larrea et al. (Citation2005), report an increase in the water absorption capacity of the orange bagasse subjected to the extrusion process.
Figure 4. Graphs of response surface for the water absorption index and water solubility index. (a) Die temperature and moisture feed effect, on the water absorption index at a concentration of 100 g/kg of orange bagasse. (b) Effect of the die temperature–orange bagasse interaction, on the water absorption index at a moisture content of 160 g/kg. (c) Effect of die temperature–orange bagasse interaction, on the water solubility index, at a moisture content of 160 g/kg. (d) Effect of the interaction of moisture feed-orange bagasse on the water solubility index, at a temperature of 170°C.
Figura 4. Gráficos de superficie de respuesta para el índice de absorción de agua y el índice de solubilidad en agua. (a) Efecto de la temperatura de la matriz y la humedad de alimentación, sobre el índice de absorción de agua, a una concentración de 100 g/kg de bagazo de naranja. (b) Efecto de la interacción temperatura de la matriz-bagazo naranja, sobre el índice de absorción de agua con un contenido de humedad del 160 g/kg. (c) Efecto de la interacción temperatura de la matriz-bagazo naranja sobre el índice de solubilidad en agua, a un contenido de humedad del 160 g/kg. (d) Efecto de la interacción del bagazo de naranja y la humedad de alimentación sobre el índice de solubilidad en agua a una temperatura de 170°C.
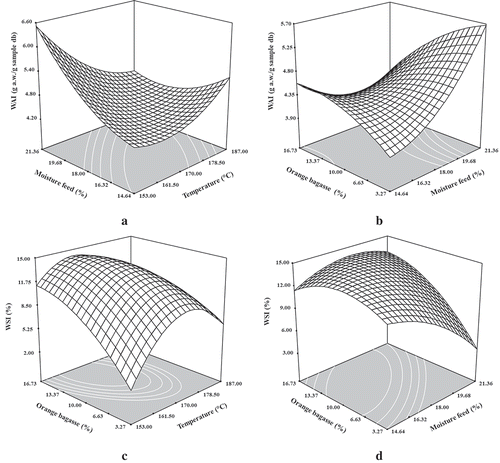
The WSI increases when the extrusion temperature increases from 153 to 170°C and by increasing the orange bagasse content, >170°C WSI decreases ( while increasing the feed moisture WSI decreases (. Altan, McCarthy, and Maskan (Citation2009), they found an increase in solubility by increasing the temperature of the extruder die around 150°C due to an increase of gelatinized starch and thus the soluble solids. During the extrusion process, the dough is subjected to mechanical shearing, high pressures, and temperatures, breaking covalent and non-covalent bonds between carbohydrates and protein associated with the fibers, as well as the breaking of the hydrogen bonds in the crystalline structure of starch, originating the formation of smaller molecular fragments that are more soluble. The WSI reduction as temperature increases could be caused by an increase in the interactions between degraded starch, protein, insoluble fiber, and lipids. These interactions could increase the molecular weight of the formed complexes, causing a decrease in WSI. A high solubility is a characteristic of over processed products, which results in an increase in the decomposition of polymers present in the raw material (starch and dietary fiber) to soluble in water forms, which is not desired, because products with a high solubility are products that can be rapidly digested and absorbed, dangerously modifying the glycemic index when consumed (Guillon & Champ, Citation2000). Some breakfast cereals have WSI values of 500 g/kg. The solubility index for the different treatments is in the range of 50.8 ± 6.34 to 160.3 ± 3.96g/kg, with the highest solubility at low moisture contents. The solubility in extruded products will depend on the processing conditions (raw material composition, screw speed, moisture used, as well as extrusion temperature and die outlet diameter).
3.2.5. Sensory analysis
Three samples out of 20 were selected for the sensory analysis, the selected samples were the ones that presented the highest EIs, the selection is mainly due to the EI correlating directly with the products hardness and therefore with their texture. The averages of the ratings given to each product by panelists are shown in . The extruded product that presented the highest color acceptability value was A with 60 g/kg of orange bagasse followed by B with 100 g/kg of orange bagasse, whereas the C with 160 g/kg of orange bagasse obtained the lowest value because it had a yellowish brown color not so pleasant to the panelist, likewise the A and B extruded snacks showed the highest values for texture. In terms of flavor, the snack with the highest content of orange bagasse showed the highest acceptability for the orange flavor but the lowest for the bitter taste, showing that the higher the content of orange bagasse the more bitter the products. The total acceptability of the extruded products was found in a range of 3.65 ± 0.42 to 5.20 ± .30, with the extruded product having 100 g/kg of orange bagasse being the one that obtained the best total acceptability.
Table 3. Scores obtained from the sensory analysis of three extruded products of blue maize with different content of orange bagasse.
Tabla 3. Calificaciones obtenidas del análisis sensorial de tres productos extruidos de maíz azul con diferente contenido de bagazo de naranja.
4. Conclusions
The results showed that it is possible to generate a directly expanded snack with agro-industrial residues and blue maize, which could bring health benefits due to the anthocyanin content of blue maize and the dietary fiber provided by the orange bagasse. This snack could be an alternative to the development of functional snacks, as well as a possible solution for the use of agro-industrial residues, giving them value and a promising use. From the technological point of view, the incorporation of orange bagasse would be a problem at concentrations higher than 100 g/kg, due to the reduction in the EI and the increase in PF, but from the nutritional and functional point of view, it is beneficial because it increases WAI and decreases WSI, which would result in an increase in the viscosity of the bolus and a smaller amount of free sugars, decreasing the glycemic index.
Disclosure statement
No potential conflict of interest was reported by the authors.
References
- Aguayo-Rojas, J., Mora-Rochín, S., Cuevas-Rodríguez, E. O., Serna-Saldivar, S. O., Gutiérrez-Uribe, J. A., Reyes-Moreno, C., & Milán-Carrillo, J. (2012). Phytochemicals and antioxidant capacity of tortillas obtained after lime cooking extrusion process of whole pigmented Mexican maize. Plants Foods for Human Nutrition, 67(2), 178–185.
- Ainsworth, P., Íbanoğlu, Ş., Plunkett, A., Íbanoğlu, E., & Stojceska, V. (2007). Effect of brewers spent grain addition and screw speed on the selected physical and nutritional properties of an extruded snack. Journal of Food Engineering, 81, 702–709.
- Altan, A., McCarthy, K. L., & Maskan, M. (2008). Evaluation of snack foods from barley tomato pomace blends. Journal of Food Engineering, 84, 231–242.
- Altan, A., McCarthy, K. L., & Maskan, M. (2009). Effect of extrusion cooking on functional properties and in vitro starch digestibility of barley-based extrudates from fruit and vegetable by-products. Food Engineering Physical Properties, 74(2), E77–E86.
- Anderson, R. A., Conway, H. F., Pfeifer, V. F., & Giffin, E. L. (1969). Rolland extrusion cooking of grain sorghum grits. Cereal Science Today, 14(11), 371–376.
- Arhalias, A., Bouvier, J. M., & Legrand, J. (2003). Melt growth and shrinkage at the exit of the die in the extrusion cooking process. Journal of Food Engineering, 60, 185–192.
- Batterman-Azcona, S. J., Lawton, J. W., & Hamaker, B. R. (1999). Effect of specific mechanical energy on rotein bodies and α zeins in flour corn extrudates. Cereal Chemistry, 76(2), 316–320.
- Berrios, J. J., Wood, D. F., Whitehand, L., & Pan, J. (2004). Sodium bicarbonate and the microstructure, expansion and color of extruded black beans. Journal of Food Process and Preservation, 28, 321–335.
- Camacho-Hernández, I. L., Zazueta-Morales, J. J., Gallegos-Infante, J. A., Aguilar-Palazuelos, E., Rocha-Guzmán, N. E., Navarro-Cortez, R. O., … Gómez-Aldapa, C. A. (2014). Effect of extrusion conditions on the physicochemical characteristics and anthocyanin content of blue-corn third generation snacks. CyTA-Journal of Food. doi:10.1080/19476337.2013.861517
- CANACINTRA. (2017, July 25). Datos de la industria de las botanas. Retrieved from http://www.botanas.org.mx/botanas/index.php/industria/datos-de-la-industria
- Dehghan-Shoar, Z., Hardacre, A. K., & Brennan, C. S. (2010). The physicochemical characteristics of extruded snacks enriched with tomato lycopene. Food Chemistry, 123(4), 1117–1122.
- Delgado-Nieblas, C., Aguilar-Palazuelos, E., Gallegos-Infante, A., Rocha-Guzmán, N., Zazueta-Morales, J., & Caro-Corrales, J. (2012). Characterization and optimization of extrusión cooking for the manufacture of third generation snacks with Winter squash (Cucurbita moschata D.) flour. Cereal Chemistry, 89(1), 65–72.
- El-Dash, A. A. (1981). Application and control of thermoplastic extrusion of cereal for food and industrial uses. In Y. Pomeranz & L. Munch (Eds.), Cereals: A renewable resource, theory and practice American Association of Cereal Chemists (pp. 165–216). Minnesota: CRC press.
- Gorinstein, S., Martin-Belloso, O., Park, Y. S., Haruenkit, R., Lojek, A., Ciz, M., … Trakhtenberg, S. (2001). Comparison of some biochemical characteristics of different citrus fruits. Food Chemistry, 74, 309–315.
- Guillon, F., & Champ, M. (2000). Structural and physical properties of dietary fibres, amd consequences of processing on human physiology. Food Research International, 33, 233–245.
- Larrea, M. A., Chang, Y. K., & Martínez-Bustos, F. (2005). Effect of some operational extrusion parameters on the constituents of orange pulp. Food Chem, 89, 301–308.
- Laufenberg, G., Kunz, B., & Nystroem, M. (2003). Transformation of vegetable waste into value added products: (A) the upgrading concept; (B) practical implementations. Bioresource Technology, 87, 167–198.
- Lewicki, P. P. (2004). Water as the determinant of food engineering properties: A review. Journal of Food Engineering, 61, 483–495.
- Marín, F. R., Soler-Rivas, C., Benavente-García, O., Castillo, J., & Pérez-Alvarez, J. A. (2007). By-products from different citrus processes as a source of customized functional fibres. Food Chemistry, 100, 736–741.
- Meng, X., Threinen, D., Hansen, M., & Driedger, D. (2010). Effect of extrusion conditions on system parameters and physical properties of a chickpea flour based snack. Food Research International, 43, 650–658.
- Mensa-Wilmot, Y., Phillips, R. D., Lee, J., & Eitenmiller, R. R. (2003). Formulation and evaluation of cereal legume based weaning food supplements. Plant Foods for Human Nutrition, 58, 1–14.
- Mora-Rochin, S., Gutiérrez-Uribe, J. A., Serna-Saldívar, S. O., Sánchez-Peña, E., Reyes-Moreno, C., & Milán-Carrillo, J. (2010). Phenolic content and antioxidant activity of tortillas produced from pigment maize processed by conventional nixtamalization or extrusion cooking. Journal of Cereal Science, 52, 502–508.
- Moraru, C. I., & Kokini, J. L. (2003). Nucleation expansion during extrusion and microwave heatings of cereal foods. Journal of Food Science and Food Safety, 2, 120–137.
- Navarro-Cortez, R. O., Gómez-Aldapa, C. A., Aguilar-Palazuelos, E., Delgado-Licon, E., Castro-Rosas, J., Hernández-Ávila, J., … Medrano-Roldán, H. (2016). Blue corn (Zea mays L.) with added orange (Citrus sinensis) fruit bagasse: Novel ingredients for extruded snacks. CyTA-Journal of Food, 14(2), 349–358.
- Oke, M. O., Awonorin, S. O., Asiedu, R., & Aiyedun, P. O. (2012). Effect of extrusion variables on the extrudates properties of water yam flour a response surface analysis. Journal of Food Process. doi:10.1111/j.1745-4549.2011.00661.x
- Pérez-Navarrete, C., González, R., Chel-Guerrero, L., & Betancur-Ancona, D. (2006). Effect of extrusion on nutritional quality of maize and Lima bean flour blends. Journal Science of Food and Agriculture, 86(14), 2477–2484.
- Rinaldi, V. E. A., Ng, P. K. W., & Bennink, M. R. (2000). Effects pf extrusion on dietary fiber and isoflavone content of wheat extrudades enriched with okara. Cereal Chemistry, 77(2), 237–240.
- Robin, F., Bovet, N., Pineau, N., Schuchmann, H. P., & Palzer, S. (2011). Online shear viscosity measurement of starchy melts enriched in wheat bran. Journal of Food Science, 76, E405–E412.
- Robin, F., Dattinger, S., Boire, A., Forny, L., Horvat, M., Schumman, H. P., & Palzer, S. (2012). Elastic properties of extruded starchy melt containing wheat bran using on line rheology and dynamic mechanical thermal analysis. Journal of Food Engineering, 109, 414–423.
- Rocha-Guzmán, N. E., Gallegos-Infante, J. A., González-Laredo, R. F., Castillo-Antonio, P. A., Delgado-Licon, E., & Ibarra-Perez, F. (2006). Functional properties of three common bean (Phaseolus vulgaris) cultivars stored under accelerated conditions followed by extrusion. Journal of Food Science and Technology, 39(1), 6–10.
- Rodríguez-Miranda, J., Ruiz-Lopez, I. I., Herman-Lara, E., Martínez-Sánchez, C. E., Delgado-Licon, E., & Vivar-Vera, M. A. (2011). Development of extruded snacks using taro (Colocasia esculenta) and nixtamalized maize (Zea mays) flour blends. Journal of Food Science and Technology, 44, 673–680.
- Roos, Y., & Karel, M. (1991). Plasticizing effect of water on thermal behavior and crystallization of amorphous food models. Journal of Food Science, 56(1), 38–43.
- Sacchetti, G., Pittia, P., & Pinnavaia, G. G. (2005). Effect of extrusion temperature and drying tempering on both the kinetics of hydration and textural changes in extruded ready to eat breakfast cereals during soaking semi-skimmed milk. International Journal of Food Science and Technology, 40, 655–663.
- Wang, L., Ganjyal, G. M., Jones, D. D., Weller, C. L., & Hanna, M. A. (2005). Modelling of bubble growth dynamic and nonisothermal expansion in starch based foam during extrusion. Advances in Polymer Technology, 24(1), 29–45.
- Yağci, S., & Göğüş, F. (2008). Development of extruded snack from food by-products: A response surface analysis. Journal of Food Process and Engineering, 32, 565–586.
- Zazueta-Morales, J. J., Martínez-Bustos, F., Jacobo-Valenzuela, N., Ordorica-Falomir, C. A., & Paredes-López, O. (2001). Effect of the addition of calcium hydroxide on some characteristics of extruded products from blue maize (Zea mays L) using response surface methodology. Journal of Food Science and Agricultural, 81(14), 1379–1386.
- Zhang, M., Bai, X., & Zhang, Z. (2011). Extrusion process improves the functionality of soluble fiber in oat bran. Journal of Cereal Science, 54, 98–103.