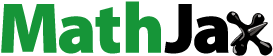
ABSTRACT
This experiment was conducted to elucidate the protein oxidation and gel properties of whey protein hydrolysates that were mixed into raw pork patties with subsequent chilled storage. Whey protein polypeptides of different size classes (Fraction I, MW > 10 k; Fraction II, MW 3–10 k; Fraction III, MW < 3 k) were separated by ultrafiltration and the pork patties were stored for 0, 2, 4, 6, and 8 days at 4°C. The contents of thiobarbituric acid-reactive substances (TBARS), carbonyl groups, and gel textural properties were determined. The results showed that there was no significant difference at the beginning of the storage period between the treatment groups that contained whey polypeptides or BHA and the control group (P > 0.05). After 2 days of storage, Fraction III (F3) was superior to Fraction I (F1), Fraction II (F2), and the control in inhibiting the oxidation of pork patties and improving gel quality. In addition, F3 also had a higher water-holding capacity and storage modulus, which was similar to the 0.02% butylated hydroxyanisole (BHA) group. The amino acid compositions showed that F3 had relatively higher contents of histidine, arginine, and proline, demonstrating a strong potential for F3 to be used as a natural antioxidant in raw pork preservation.
RESUMEN
El presente experimento se propuso elucidar la oxidación proteica y las propiedades de gel de los hidrolizados de proteína de suero mezclados en medallones de puerco y luego almacenados en frío. Se separaron los polipéptidos de la proteína de suero de distintas clases de tamaño (Fracción I, MW > 10 k; Fracción II, MW 3-10 k; Fracción III, MW < 3 k) mediante ultrafiltración; posteriormente, se almacenaron los medallones de puerco durante 0, 2, 4, 6 y 8 días a 4 °C. Se registraron los contenidos de las sustancias reactivas al ácido tiobarbitúrico (TBARS), los grupos carbonilos y las propiedades texturales de gel. Los resultados indicaron que, al comenzar el periodo de almacenamiento, no existieron diferencias significativas entre los grupos tratados con polipéptidos o BHA y el grupo de control (P > 0.05). Después de 2 días de almacenamiento se estableció que la Fracción III (F3) superaba a la Fracción I (F1), la Fracción II (F2) y el control en términos de inhibir la oxidación de los medallones de puerco y mejorar la calidad de gel. Asimismo, la F3 tenía mayor capacidad de retención de agua y módulo de almacenamiento, cualidades que se asemejan al grupo BHA (butilhidroxianisol) al 0.02%. Las composiciones de aminoácidos mostraron que la F3 exhibía contenidos relativamente más elevados de histidina, arginina y prolina, demostrando dicha fracción tiene un fuerte potencial para ser utilizada como antioxidante natural en la conservación de carne de puerco cruda.
1. Introduction
During the processing and storage of meat products, many factors, such as exposure to light, oxidation, temperature, and enzyme interaction, inevitably can impact meat quality (Turgut, Işıkçı, & Soyer, Citation2017; Utrera, Morcuende, & Estévez, Citation2014). The most notable impacts are a reduction of water-holding capacity (WHC), tenderness, flavor, and nutrient availability (Wang & Xiong, Citation2008), which may lead to economic loss in the meat processing industry (Jully, Toto, & Were, Citation2015). Previous research has shown that oxidation can result in the generation of aldehydes, ketones, hydrocarbons, esters, and ethanol, as well as other harmful components. This can result in spoilage or the deterioration of the product (Rysman, Hecke, Poucke, Smet, & Royen, Citation2016). Therefore, processing and storage strategies that reduce oxidation, which inherently affects meat quality, are highly desirable. One method of improving antioxidant capacity is the direct addition of antioxidants to meat products (Turgut, Soyer, & Işıkçı, Citation2016). In recent years, the efficiency of antioxidant peptides has been confirmed by in vivo and in vitro tests (Hwang & Winklermoser, Citation2017; Lin et al., Citation2017). Antioxidant action modes, which lend to this efficiency, include free-radical scavenging, metal chelation, lipid oxidation inhibition, and increased enzyme antioxidant activity (Farvin et al., Citation2016; Wang, Wang, & Li, Citation2016). The development and utilization of natural antioxidant polypeptides has gained tremendous attention by experts in the fields of food and medicine (Li, Kong, Xia, Liu, & Diao, Citation2013). In cooked beef, the hydrolysates prepared from potato protein had a strong inhibitory effect on lipid oxidation (And & Xiong, Citation2005). The antioxidant polypeptides isolated from milk proteins can prevent lipid oxidation during the storage of raw beef (Shelly, Zhang, Li, Wang, & Zhou, Citation2009).
Myofibrillar protein is abundant in pork, and it has a very strong heat-induced gelation capacity among animal proteins, which can directly impact the quality of meat products (Rysman et al., Citation2016). Protein polypeptides can contribute to the gelatinization process of myofibrillar protein through covalent and non-covalent interactions, thus influencing texture, microstructure, and WHC of the protein gel in pork products (Li et al., Citation2013). Xiong et al. (Xiong, Park, & Ooizumi, Citation2009) found that moderate oxidation of pork protein can facilitate cross-linking of proteins and promote the formation of a gel network. In contrast, excessive oxidation resulted in serious degradation of the textural properties and strength of the gel network. Decker et al. (Citation1993) reported that oxidation of myofibrillar protein from turkey resulted in decreased gel strength and that myosin oxidation led to further deterioration of the gel. Li et al. (Citation2013) reported that the addition of polypeptides from whey protein can effectively inhibit the oxidation of myofibrillar protein during freezer storage. Massimiliano et al. (Petracci, Bianchi, Mudalal, & Cavani, Citation2013) found that the addition of 8% whey protein enhanced the hardness and chewiness of sausages due to a more compact microstructure.
Previous studies have demonstrated that hydrolysates of whey protein subjected to 5 h of hydrolysis by Alcalase have excellent antioxidant capacity in vivo and in vitro (Peng, Kong, Yu, & Diao, Citation2014). After separation using a Sephadex G-10 column and purification using a protein purifier, the antioxidant polypeptides with lower molecular weights exhibited better free-radical scavenging capacity (Peng, Xiong, & Kong, Citation2009). In addition, these polypeptides with lower molecular weights also provided better protection against oxidative stress-induced damage to cells (Kong, Peng, Xiong, & Zhao, Citation2012). Due to the quantity limitations and procedural complexity of separation into polypeptide molecular weight classes employed by Peng et al. (Citation2009), an alternative separation technique was conducted with ultrafiltration membranes. In order to understand the antioxidant action mode of size-separated whey polypeptides, each separation was added to raw pork patties, and the inhibitory effect on protein oxidation and quality deterioration was determined in association with chilled storage intervals.
2. Materials and methods
2.1. Materials
Whey protein isolate (WPI, 95% protein) was purchased from Davisco Foods International Inc. (Minnesota, MN, USA) and stored at 4°C until use. Pork trims were obtained within 24 h post-mortem (pH 5.6–5.8) from Jinluo Meat Corporation (Yantai, Shandong, China), packed in ice during transport, and processed into patties on the same day. Alcalase™ (2.4 L, 2.4AU-A/g) was obtained from Novozymes Inc. (Franklinton, NC, USA). Butylated hydroxyanisole (BHA), soybean phosphatidylcholine, 2,2′-azinobis (3-ethylbenzothiazoline-6-sulfonic acid) (ABTS), 2,4-dinitrophenylhydrazine (DNPH), 5,5-dimethyl-1-pyrroline-N-oxide (DMPO, a spin trapper), riboflavin, and thiobarbituric acid (TBA) were purchased from Sigma Chemical Co. (St. Louis, MO, USA). Other chemicals and reagents used in this study were analytical grade, and were purchased from Yongxin Biological Technology Co., Ltd. (Yixing, Jiangsu, China).
2.2. Preparation and fractionation of protein hydrolysates by ultrafiltration
To produce the hydrolysates, WPI samples (45 mg/mL) were heated at 95°C in a water bath for 5 min; the enzyme:WPI ratio (E/S) was 2:100; and hydrolyzation was carried out for 5 h with Alcalase under optimal conditions (pH 8.5, 65°C), as recommended by Peng et al. (Citation2009). The hydrolysates were fractionated by ultrafiltration membranes with different molecular weight (MW) cutoffs. Three fractions, including Fraction I (F1, MW > 10 k), Fraction II (F2, MW 3–10 k), and Fraction III (F3, MW < 3 k) were obtained, and the hydrolysate fractions were lyophilized (LGJ-1 Freeze-Dryer, Shanghai, China), sealed tight, and stored at 4°C until further analysis.
2.3. Superoxide anion and ABTS radical-scavenging (ABTS+•) activity
The superoxide anion radical-scavenging activity (RSA) of each fractioned class of protein hydrolysates was measured on an ER 200D-SRC ESR spectrometer (Bruker, Germany) using an irradiated riboflavin/Ethylene diamine tetraacetic acid (EDTA) system with 0.3 mM riboflavin, 0.6 mM EDTA, and 300 mM DMPO (Peng et al., Citation2009). The RSA was calculated as (H0 − H)/H0 × 100%; where H and H0 represent the height of the first resonance peak for samples with and without protein, respectively.
The ABTS radical-scavenging (ABTS+•) activity was determined by a previous method (Jia, Kong, Liu, Diao, & Xia, Citation2012). Specifically, 20 µL of each hydrolysate sample was mixed with a diluted ABTS+• solution with an absorbance of 0.70 ± 0.01 at 734 nm. The ABTS+• activity (%) was expressed as (Ac − As) × 100/Ac, where Ac and As were the absorbance of the control (ABTS+• solution without hydrolysates) and the sample, respectively.
2.4. Preparation of pork patties
The experiment was divided into five groups, which included: a control group without any additional ingredients except 2% NaCl, which was also added to each group because of its commonality in meat products; treatment groups with the addition of F1, F2, and F3 in the amount of 15% (w/w); and a positive control group with the addition of BHA at 0.02% (w/w as its basis). Duplicate 50 g patties were also made. The patty ingredients were mixed with a Kitchen Aid food processor (St Joseph, MI, USA) for 5 min, and then patties were formed with an approximately 7.5 cm diameter and a 1.5 cm thickness. The patties were placed in food grade polypropylene trays, overwrapped with an oxygen-permeable polyvinyl chloride film (Shenzhen Qiangli Plastic Packing Products Co., Ltd., Guangdong, China), and stored at 4°C for 0, 2, 4, 6, or 8 days under fluorescent lights to simulate supermarket retail display conditions.
2.5. Measurement of lipid oxidation
The secondary product of lipid oxidation was evaluated by TBARS according to a previously developed method with minor modifications (Xia, Kong, Liu, & Liu, Citation2009). Specifically, 5 g of meat sample was homogenized (10,000 rpm, 90 s) in 15 ml of 7.5% trichloroacetic acid, 0.1% propyl gallate, and 0.1% EDTA. After filtering, 5 mL of the filtrate was mixed with an equal volume of 20 mM TBA. The mixture was incubated at 100°C in a water bath for 40 min, and the absorbance was measured at 532 nm.
2.6. Measurement of protein oxidation
2.6.1. Preparation of myofibrillar protein
Myofibrillar protein was extracted according to a previous method with an extraction solution of 100 mM NaCl, 2 mM MgCl2, 1 mM EDTA, and 10 mM K2HPO4 (pH 7.0) (Liu & Xiong, Citation1996). The solution was blended, homogenized, and centrifuged (2000 × g for 10 min). The washing solution was 100 mM NaCl. The collected samples were kept in a tightly capped bottle, stored at 4°C, and used within 24 h.
2.6.2. Protein carbonyl contents
Protein carbonyl contents were measured according to the method of Levine et. al. (Citation1994). Absorbance was measured at 365 nm for the DNPH-treated sample against an HCl control. Additionally, the absorbance of the control was measured at 280 nm to calculate protein concentration in the final pellet using bovine serum albumin (BSA) as the standard. The carbonyl content was expressed as nmol of DNPH in each mg of protein using an absorption coefficient of 22,000 M−1cm−1 for protein hydrazones.
2.7. Amino acid analysis
Amino acid compositions of F1, F2, and F3 obtained by ultrafiltration were measured using a model L-8800 amino acid analyzer (Hitachi Co., Tokyo, Japan) equipped with a Hitachi 2622 cation exchange column (4.6 nm × 60 mm) for the separation of amino acids.
2.8. The determination of gel quality
2.8.1. The preparation of gel and the determination of texture (TPA)
An appropriate amount was sampled from the original patty by taking a section from the middle, placing it in a 15 mL weighing bottle with a cover, and heating it in an 80°C water bath for 30 min. After gel formation and ambient cooling, the texture of the pork patty gels was analyzed by a texture analyzer (QTS-25 Texture Instrument Shanghai Anting Scientific Instrument Factory). The gel sample was compressed twice in succession by 50% each time using the P/36R probe. The test rate was 2 mm/s; the compression ratio was 50%; the dwell time was 5 s. The data acquisition rate was 200 pps, and the trigger value was 5 g.
2.8.2. The determination of WHC
WHC was determined via the previous method by Zhang, Yang, Tang, Chen, and You (Citation2015). After centrifuging at 3000 × g at 4°C for 10 min, the liberated liquid on top of the gel was absorbed completely by a paper towel and the weight of the remaining gel and tube was recorded. The WHC was calculated by following formula:
where W0 is the weight of the centrifuge tube, W1 is the weight of the centrifuge tube including the gel before centrifugation, and W2 is the weight of the centrifuge tube and gel after centrifugation with the free liquid removed.
2.9. The dynamic rheological properties of gels
The patty samples were stored overnight at 4°C and measured with a rheometer (Discovery DHR-1, TA, USA) according to the method of Zhao et al. (Citation2013) with modifications. Multiple samplings were used from each patty and placed between two parallel plates (upper plate: 30 mm diameter) for each measurement. By following the parameters noted above, the changes in gel storage modulus (Gʹ), or elastic response, as the temperature increased, were determined.
2.10. Statistical analysis
All analytical tests were carried out in triplicate for each patty or polypeptide-size class. Data was analyzed using Statistix 8.1 software (Analytical Software, St. Paul, MN, USA). The significant difference between groups was identified using the Turkey procedure (P < 0.05).
3. Results and discussion
3.1. Radical-scavenging capacity of whey protein fractions
In the present study, superoxide anions were produced by riboflavin/EDTA solution in the presence of ultraviolet radiation, as shown in . The superoxide anion radical-scavenging capacity of the three polypeptide-size class samples revealed an obvious order of radical-scavenging capacity of F3 > F2 > F1 with the clearance rate of 85.50%, 45.12%, and 35.50%, respectively. ABTS can be oxidized as green ABTS+• in the presence of oxidants; in contrast, its oxidation can be inhibited in the presence of antioxidants. The ABTS+• radical-scavenging rates of F1, F2, and F3 were 64.90%, 72.36%, and 77.78%, respectively, which represents an improvement of 2.01%, 13.74%, and 22.26% when compared with the control. These results show that whey polypeptide fragments with different molecular weights had different antioxidant activity, and the highest radical-scavenging capacity was shown by F3. In addition, strong antioxidant activity of polypeptides with a specific molecular weight has been widely confirmed (Peng et al., Citation2009; Wattanasiritham, Theerakulkait, Wickramasekara, Maier, & Stevens, Citation2016). For example, the polypeptides in the hydrolysates from rice bran with molecular weights of 800–2100 Da have a better oxygen absorption capacity of oxygen free radicals than other rice polypeptides (Wattanasiritham et al., Citation2016). In the current study, whey polypeptide fragments with different molecular weights had different radical-scavenging capacities, and the highest radical-scavenging capacity was observed in the polypeptide class with a molecular weight of less than 3 k, which may be due to the disruption of the tight structure upon the hydrolysis of pork protein. Furthermore, the amino acids with higher antioxidant activity were concentrated after membrane filtration (Liu, Kong, Li, Liu, & Xia, Citation2011), thereby resulting in the enhancement of antioxidant activity within the F3 polypeptides.
Figure 1. ESR spectra of superoxide anion radical-scavenging signals (A) and ABTS radical-scavenging activity (B) of control (without hydrolysates), F1, F2, and F3. Means with different letters (a–c) at the same storage time revealed a significant difference (P < 0.05).
Figura 1. Espectro ESR de señales de eliminación de radicales por medio del anión superóxido (A) y de actividad de eliminación de radicales mediante ABTS (B) del control (sin hidrolizados), F1, F2 y F3. Las medias señaladas con distintas letras (a-c) para la misma duración de almacenamiento indican una diferencia significativa (P < 0.05).
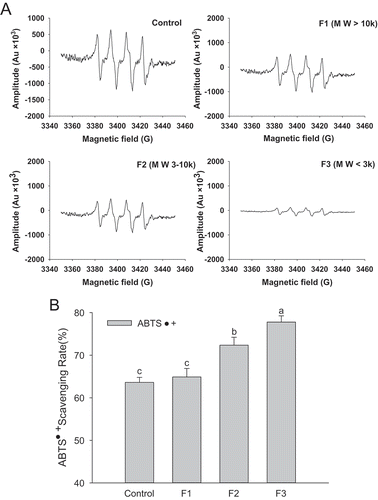
3.2. Thiobarbituric acid-reactive substance (TBARS) values of lipid oxidation in pork patties
The TBARS value reflects the production of malondialdehyde (MDA) as the secondary product of lipid oxidation (Xiong et al., Citation2015). A higher TBARS value usually suggests more severe lipid oxidation (Moudache, Nerín, Colon, & Zaidi, Citation2017). As the storage time increased (), the TBARS value in each treatment group showed an increasing trend. The TBARS value in the control group reached 2.85 mg/kg on the 8th day, indicating that during the storage period the patties had obvious lipid oxidation and substantial accumulation of MDA. There was no significant difference among treatment groups and the control group at day 0; there were significant differences among treatment groups on the 2nd day and thereafter. On the 8th day of storage, the TBARS values in F1, F2, and F3 groups and the BHA group were 2.54, 2.55, 1.39, and 1.47 mg/kg respectively, with an MDA reduction of 10.38%, 10.53%, 51.23%, and 48.42%, compared with the control patties. This suggests that the treatment with F3 polypeptides had the best inhibitory effect of lipid oxidation.
Figure 2. Effects of F1, F2, and F3 as well as BHA on TBARS values of pork patties during chilled storage compared to control patties.
Figura 2. Efectos de F1, F2, F3 y BHA en los valores TBARS de los medallones de puerco durante el almacenamiento en frío, en comparación con los medallones de control.
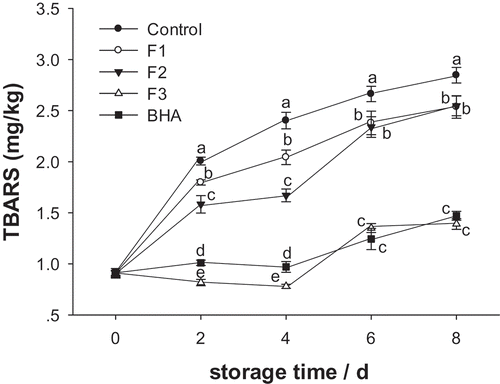
3.3. Inhibition of protein oxidation in pork patties
As shown in , the contents of carbonyl increased during the entire storage period. On Day 0, there was no significant difference among treatment groups or the control group. No significant difference was observed compared to the control on the 0, 2nd, and 4th day for the F1 patties. On the 6th day, F3 had a similar effect compared to the BHA group (P > 0.05), and both the F3 and BHA groups were better than F2 and F1 groups (P < 0.05). On the 8th day, the contents of carbonyl in the F1, F2, F3, and BHA groups showed a reduction by 4.3%, 4.0%, 11%, and 8.9%, respectively, from the 8th day control group. Therefore, whey protein fractions may retard the formation of carbonyls by inhibiting pork patty oxidation.
Figure 3. Effects of F1, F2, F3, and BHA on the contents of carbonyl in pork patties during chilled storage compared to control patties.
Figura 3. Efectos de F1, F2, F3 y BHA en los contenidos de carbonilo de los medallones de puerco durante el almacenamiento en frío, en comparación con los medallones de control.
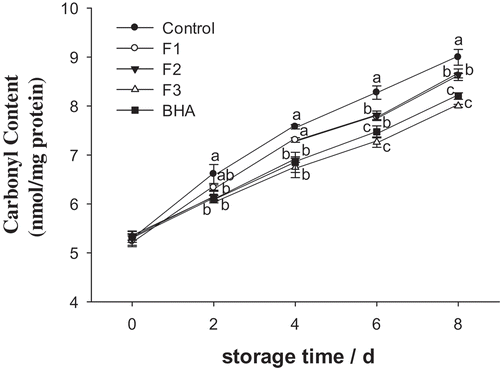
Many studies have demonstrated that carbonyl groups can be generated by the α-amidation pathway by direct oxidation of side chains, by a reducing sugar reaction, or by the binding of nonprotein carbonyl compounds (Botsoglou, Govaris, Ambrosiadis, Fletouris, & Botsoglou, Citation2014). In meat products, carbonyl compounds can be formed by the oxidation of side chains in lysine, proline, arginine, and histidine. Carbonyl content increases with the degree of protein oxidation (Turgut et al., Citation2016). In the present study, whey antioxidant polypeptides led to the reduced degradation of pork proteins compared to the control. This suggests that antioxidant polypeptides could protect against oxidative degradation of amino acids and prevent the formation of carbonyl groups during storage. In addition to environmental factors, both transition metals and oxidized lipids are potential contributors to protein oxidation (Ran et al., Citation2016). Preliminary studies have demonstrated that whey polypeptides have an excellent radical-scavenging ability, metal ion-chelating capacity, and lipid peroxidation-inhibitory capacity (Peng et al., Citation2014, Citation2009). Therefore, whey polypeptides may inhibit the oxidation of meat proteins through these noted mechanisms.
3.4. Effect of whey antioxidant polypeptides on the texture of pork patties
As shown in , there was no significant difference in springiness, chewiness, hardness, and WHC between treatment groups and control group in the beginning. However, on the 2nd day, a significant difference was observed. On the 6th and 8th days, BHA and F3 exhibited the same effect, and both maintained substantially better texture properties than the other treatments (P < 0.05). With the extension of storage time, the hardness in each treatment group began to reveal a significant increase on the 4th day from the 2nd day. By the 8th day, this represented a reduction by 6.29%, 10.57%, 14.04%, and 18.30%, respectively, for F1, F2, F3, and BHA groups, when compared with the control group. On the 4th day, BHA, F3, and F2 maintained better WHC than F1 and the control (P < 0.05). On the 8th day, BHA and F3 maintained better WHC than F2, F1, and the control (P < 0.05).
Table 1. The effects of F1, F2, F3, and BHA on the quality and textural properties of raw pork patties during storage, compared to control patties.
Tabla 1. Los efectos de F1, F2, F3 y BHA en la calidad y las propiedades texturales de medallones de puerco crudos durante el almacenamiento, en comparación con los medallones de control.
This illustrates that in the late storage, F1, F2, F3, and BHA all inhibited, to some degree, the trend of increasing hardness expected with increased storage time. The treatment groups also resisted, to some degree, the decreasing trend of chewiness, springiness, and WHC associated with longer storage time. Among them, F3 and BHA have similar effects. The data suggests that both whey antioxidant polypeptides and BHA treatments have a distinguished effect on the maintenance of texture in pork patties during storage.
Gel formation in meat products is primarily due to the three-dimensional structure created by protein–protein cross-linkages. If oxidation of myofibrillar protein occurs, denaturation can result in the degradation of the cross-linkages, thus resulting in the reduction of WHC, softness, juiciness, and texture-related capacities, leading to a direct impact on product nutrition and gel quality (Xiong et al., Citation2009). In the present study, gel hardness, chewiness, springiness, and WHC showed a tendency to deteriorate from ideal parameters as storage time was extended. This is due to the reduction of hydrogen bonds between proteins, and damage to the natural shape conformity of the proteins (Intarasirisawat, Benjakul, Visessanguan, & Wu, Citation2014). In this study, F3 in pork patties revealed a beneficial antioxidant effect, which may be due to their interaction in the formation of a fine network structure with myofibrillar protein after whey antioxidant polypeptides were added to the pork patty mixture. A more cohesive network structure may enhance the WHC through capillary action and protein hydration. At the same time, the addition of protein hydrolysates can prevent the degradation of the three-dimensional network structure by inhibiting protein oxidation, thus attenuating the deterioration of the gel quality, leading to the maintenance of textural properties.
3.5. Effect of whey antioxidant polypeptides on storage modulus of pork patties
As shown in , during the storage process, the G’ in each treatment was similar and concurrently showed similar trends as the temperature increased. From the 2nd day, the G’ in each treatment group revealed a slow decrease at low temperature, while the G’ value in each treatment group began to increase when the temperature exceeded 40°C. At the temperature of 46°C, the G’ value produced a small peak, followed by a slight dip, and then substantially increased to reach a maximum G’ value from 60°C to 80°C. During the large G’ increase phase, the continuous increase of the G’ value was mainly due to the enhanced aggregation of interacting proteins, leading to an accelerated formation of a gel network. However, the rate of change of the G’ value exhibited a significant difference as storage time progressed, indicating that the addition of hydrolysates and BHA had a significant impact on G’. During the entire storage period, the reduction of G’ value in the control and F1 groups was obvious. The F2 group occupied the middle range, suggesting some degree of improvement. The highest values were observed in the BHA and F3 groups during the same storage period, indicating that both BHA and F3 effectively protected the rheological properties of the patties.
Figure 4. Effects of F1, F2, F3, and BHA on storage modulus of pork patties during chilled storage compared to control patties.
Figura 4. Efectos de F1, F2, F3 y BHA en los módulos de almacenamiento de los medallones de puerco durante el almacenamiento en frío, en comparación con los medallones de control.
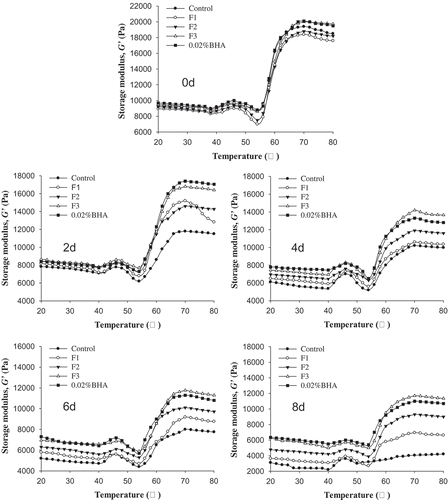
Within rheological properties, the G’ reflects the elasticity of the protein and the transition temperature of the protein gel (Ersch et al., Citation2016). A larger G’ can result in better elasticity and toughness of the gel. Beginning at 20°C, the G’ value revealed a gradual decrease with increasing temperature, which may be due to the dissociation of light-chain subunits in myosin, resulting in corresponding enhanced molecular mobility. A small peak appeared at 46°C, perhaps because the gel network in myosin initially cross-linked to form a weak gel. Sequentially, the G’ value was reduced to the minimum level at 54°C, which may be due to the degeneration of the light chains in myosin, thus resulting in the increased mobility and reduced elasticity (Choi & Kim, Citation2009). However, the G’ value exhibited a rapid increase at a temperature higher than 54°C, up to the highest point at 70°C followed by a gentle reduction toward 80°C. This is mainly due to the unfolding of myosin and the enhancement of cross-linking between proteins, thus generating a strong and irreversible heat-induced gel (Wu, Xiong, Chen, Tang, & Zhou, Citation2010). With respect to storage time, 0.02% BHA and F3 had a relatively smaller reduction of the G’ value, suggesting that BHA and F3 can interact with myofibrillar protein to ensure and maintain the formation of the gel matrix and to improve gel quality within the patties.
3.6. Analysis of amino acid compositions in each fraction from membrane separation
As shown in , the overall change in total amino acid content in the three fractions of whey protein subjected to membrane filtration was not obvious. The contents of leucine and glutamic acid in F1 and F2 were relatively higher than F3; however, the percentage of histidine, arginine, and proline in F3 revealed an increase by 200%, 42%, and 83%, and 108%, 46%, and 76%, when compared with those in F1 and F2, respectively. Polypeptides with different amino acid compositions and molecular weights generated from proteolysis also have different physiological functions. According to previous reports (Lin et al., Citation2017), the polypeptides containing histidine, lysine, arginine, and proline obtained from hydrolysis have excellent radical-scavenging capacity. Similarly, another report demonstrated that amino acids including alanine, glycine, glutamic acid, and arginine are rich in antioxidant polypeptides from the hydrolysis of Atlantic cod (Farvin et al., Citation2016), which is in agreement with our results. In the present study, F3 had relatively higher contents of histidine, arginine, and proline, which revealed a compositional difference with respect to these amino acids when compared to the F1 and F2 fractions. Antioxidant activity may also be correlated with amino acid types and acidity in polypeptides. According to a previous research (Liu et al., Citation2011) which measured TBARS values and hydroxyl radical scavenging activity, F3 (MW < 3 k) possessed the highest antioxidant activity. So, the antioxidant activity may be correlated with the molecular weight of the polypeptides.
Table 2. Amino acid compositions of F1, F2, and F3. The contents of aspartic acid and glutamic acid include asparagine and glutamine, respectively.
Tabla 2. Composiciones de aminoácidos de F1, F2 y F3. Los contenidos del ácido aspártico y el ácido glutámico incluyen asparagina y glutamina, respectivamente.
4. Conclusions
In conclusion, Fraction III (F3, MW < 3 k) hydrolysates of whey protein showed higher antioxidant activity similar to 0.02% BHA. The addition of F3 significantly inhibited lipid and protein oxidation, improved texture, and improved the G’ values of pork patties during chilled storage. These findings suggest that F3 has potential as a natural antioxidant source for meat product quality preservation. Additional research is needed to investigate the structure and specific amino acid sequences of F3 that are responsible for this preservation and its practical application in the processed meat industry.
Acknowledgments
This study was supported by the National Natural Science Foundation of China [Grant No. 31401491], the National Undergraduate Innovation and entrepreneurship training program and the Natural Science Foundation of Shandong Province, China [Grant No. ZR2017PC006].
Disclosure statement
No potential conflict of interest was reported by the authors.
Additional information
Funding
References
- And, L. L. W., & Xiong, Y. L. (2005). Inhibition of lipid oxidation in cooked beef patties by hydrolyzed potato protein is related to its reducing and radical scavenging ability. Journal of Agricultural & Food Chemistry, 53(23), 9186–9192. doi:10.1021/jf051213g
- Botsoglou, E., Govaris, A., Ambrosiadis, I., Fletouris, D., & Botsoglou, N. (2014). Effect of olive leaf (olea europea, l.) extracts on protein and lipid oxidation of long-term frozen n −3 fatty acids-enriched pork patties. Meat Science, 98(2), 150–157. doi:10.1016/j.meatsci.2014.05.015
- Choi, Y. M., & Kim, B. C. (2009). Muscle fiber characteristics, myofibrillar protein isoforms, and meat quality. Livestock Science, 122(2–3), 105–118. doi:10.1016/j.livsci.2008.08.015
- Decker, E. A., Xiong, Y. L., Calvert, J. T., Crum, A. D., & Blanchard, S. P. (1993). Chemical, physical, and functional properties of oxidized turkey white muscle myofibrillar proteins. Journal of Agricultural & Food Chemistry, 41(2), 186–189. doi:10.1021/jf00026a007
- Ersch, C., Meinders, M. B. J., Bouwman, W. G., Nieuwland, M., Linden, E. V. D., Venema, P., Martin A. H. (2016). Microstructure and rheology of globular protein gels in the presence of gelatin. Food Hydrocolloids, 55, 34–46. doi:10.1016/j.foodhyd.2015.09.030
- Farvin, K. H. S., Andersen, L. L., Otte, J., Nielsen, H. H., Jessen, F., & Jacobsen, C. (2016). Antioxidant activity of cod (gadus morhua) protein hydrolysates: Fractionation and characterization of peptide fractions. Food Chemistry, 204, 409–419. doi:10.1016/j.foodchem.2016.02.145
- Hwang, H. S., & Winklermoser, J. K. (2017). Antioxidant activity of amino acids in soybean oil at frying temperature: Structural effects and synergism with tocopherols. Food Chemistry, 221, 1168–1177. doi:10.1016/j.foodchem.2016.11.042
- Intarasirisawat, R., Benjakul, S., Visessanguan, W., & Wu, J. (2014). Effects of skipjack roe protein hydrolysate on properties and oxidative stability of fish emulsion sausage. LWT - Food Science and Technology, 58(1), 280–286. doi:10.1016/j.lwt.2014.02.036
- Jia, N., Kong, B., Liu, Q., Diao, X., & Xia, X. (2012). Antioxidant activity of black currant (ribes nigrum, l.) extract and its inhibitory effect on lipid and protein oxidation of pork patties during chilled storage. Meat Science, 91(4), 533–539. doi:10.1016/j.meatsci.2012.03.010
- Jully, K. M. M., Toto, C. S., & Were, L. (2015). Antioxidant effect of spent, ground, and lyophilized brew from roasted coffee in frozen cooked pork patties. LWT - Food Science and Technology, 66, 244–251. doi:10.1016/j.lwt.2015.10.046
- Kong, B., Peng, X., Xiong, Y. L., & Zhao, X. (2012). Protection of lung fibroblast mrc-5 cells against hydrogen peroxide-induced oxidative damage by 0.1-2.8 kda antioxidative peptides isolated from whey protein hydrolysate. Food Chemistry, 135(2), 540–547. doi:10.1016/j.foodchem.2012.04.122
- Levine, R. L., Williams, J. A., Stadtman, E. R., & Shacter, E. (1994). Carbonyl assays for determination of oxidatively modified proteins. Methods in Enzymology, 233(233), 346–357. doi:10.1016/S0076-6879(94)33040-9
- Li, Y., Kong, B., Xia, X., Liu, Q., & Diao, X. (2013). Structural changes of the myofibrillar proteins in common carp (cyprinus carpio) muscle exposed to a hydroxyl radical-generating system. Process Biochemistry, 48(5–6), 863–870. doi:10.1016/j.procbio.2013.03.015
- Lin, S., Liang, R., Xue, P., Zhang, S., Liu, Z., & Dong, X. (2017). Antioxidant activity improvement of identified pine nut peptides by pulsed electric field (pef) and the mechanism exploration. LWT - Food Science and Technology, 75, 366–372. doi:10.1016/j.lwt.2016.09.017
- Liu, G., & Xiong, Y. L. (1996). Contribution of lipid and protein oxidation to rheological differences between chicken white and red muscle myofibrillar proteins. Journal of Agricultural & Food Chemistry, 44(3), 779–784. doi:10.1021/jf9506242
- Liu, Q., Kong, B., Li, G., Liu, N., & Xia, X. (2011). Hepatoprotective and antioxidant effects of porcine plasma protein hydrolysates on carbon tetrachloride-induced liver damage in rats. Food & Chemical Toxicology, 49(6), 1316–1321. doi:10.1016/j.fct.2011.03.013
- Moudache, M., Nerín, C., Colon, M., & Zaidi, F. (2017). Antioxidant effect of an innovative active plastic film containing olive leaves extract on fresh pork meat and its evaluation by raman spectroscopy. Food Chemistry, 229, 98–103. doi:10.1016/j.foodchem.2017.02.023
- Peng, X., Kong, B., Yu, H., & Diao, X. (2014). Protective effect of whey protein hydrolysates against oxidative stress in d-galactose-induced ageing rats. International Dairy Journal, 34(1), 80–85. doi:10.1016/j.idairyj.2013.08.004
- Peng, X. Y., Xiong, Y. L. L., & Kong, B. H. (2009). Antioxidant activity of peptide fractions from whey protein hydrolysates as measured by electron spin resonance. Food Chemistry, 113(1), 196–201. doi:10.1016/j.foodchem.2008.07.068
- Petracci, M., Bianchi, M., Mudalal, S., & Cavani, C. (2013). Functional ingredients for poultry meat products. Trends in Food Science & Technology, 33(1), 27–39. doi:10.1016/j.tifs.2013.06.004
- Ran, Y., Yan, B., Li, Z., Ding, Y., Shi, Y., & Le, G. (2016). Dityrosine administration induces novel object recognition deficits in young adulthood mice. Physiology & Behavior, 164(Pt A), 292–299. doi:10.1016/j.physbeh.2016.06.019
- Rysman, T., Hecke, T. V., Poucke, C. V., Smet, S. D., & Royen, G. V. (2016). Protein oxidation and proteolysis during storage and in vitro, digestion of pork and beef patties. Food Chemistry, 209, 177–184. doi:10.1016/j.foodchem.2016.04.027
- Shelly, H., Zhang, L., Li, J., Wang, H., & Zhou, K. (2009). Development of antioxidant rich peptides from milk protein by microbial proteases and analysis of their effects on lipid peroxidation in cooked beef. Food Chemistry, 117(3), 438–443. doi:10.1016/j.foodchem.2016.02.145
- Turgut, S. S., Işıkçı, F., & Soyer, A. (2017). Antioxidant activity of pomegranate peel extract on lipid and protein oxidation in beef meatballs during frozen storage. Meat Science, 129, 111–119. doi:10.1016/j.meatsci.2017.02.019
- Turgut, S. S., Soyer, A., & Işıkçı, F. (2016). Effect of pomegranate peel extract on lipid and protein oxidation in beef meatballs during refrigerated storage. Meat Science, 116, 126–132. doi:10.1016/j.meatsci.2016.02.011
- Utrera, M., Morcuende, D., & Estévez, M. (2014). Temperature of frozen storage affects the nature and consequences of protein oxidation in beef patties. Meat Science, 96(3), 1250–1257. doi:10.1016/j.meatsci.2013.10.032
- Wang, C., Wang, B., & Li, B. (2016). Bioavailability of peptides from casein hydrolysate in vitro: Amino acid compositions of peptides affect the antioxidant efficacy and resistance to intestinal peptidases. Food Research International, 81, 188–196. doi:10.1016/j.foodres.2015.12.013
- Wang, L. L., & Xiong, Y. L. (2008). Inhibition of oxidant-induced biochemical changes of pork myofibrillar protein by hydrolyzed potato protein. Journal of Food Science, 73(6), 482–487. doi:10.1111/j.1750-3841.2008.00802.x
- Wattanasiritham, L., Theerakulkait, C., Wickramasekara, S., Maier, C. S., & Stevens, J. F. (2016). Isolation and identification of antioxidant peptides from enzymatically hydrolyzed rice bran protein. Food Chemistry, 192, 156–162. doi:10.1016/j.foodchem.2015.06.057
- Wu, M., Xiong, Y. L., Chen, J., Tang, X., & Zhou, G. (2010). Rheological and microstructural properties of porcine myofibrillar protein-lipid emulsion composite gels. Journal of Food Science, 74(4), E207–E217. doi:10.1111/j.1750-3841.2009.01140.x
- Xia, X., Kong, B., Liu, Q., & Liu, J. (2009). Physicochemical change and protein oxidation in porcine longissimus dorsi as influenced by different freeze-thaw cycles. Meat Science, 83(2), 239–245. doi:10.1016/j.meatsci.2009.05.003
- Xiong, Y. L., Park, D., & Ooizumi, T. (2009). Variation in the cross-linking pattern of porcine myofibrillar protein exposed to three oxidative environments. Journal of Agricultural & Food Chemistry, 57(1), 153–159. doi:10.1021/jf8024453
- Xiong, Z., Sun, D. W., Pu, H., Xie, A., Han, Z., & Luo, M. (2015). Non-destructive prediction of thiobarbituricacid reactive substances (TBARS) value for freshness evaluation of chicken meat using hyperspectral imaging. Food Chemistry, 179(1), 175–181. doi:10.1016/j.foodchem.2015.01.116
- Zhang, Z., Yang, Y., Tang, X., Chen, Y., & You, Y. (2015). Chemical forces and water holding capacity study of heat-induced myofibrillar protein gel as affected by high pressure. Food Chemistry, 188, 111–118. doi:10.1016/j.foodchem.2015.04.129
- Zhao, J., Lv, W., Wang, J., Li, J., Liu, X., & Zhu, J. (2013). Effects of tea polyphenols on the post-mortem integrity of large yellow croaker (pseudosciaena crocea) fillet proteins. Food Chemistry, 141(3), 2666–2674. doi:10.1016/j.foodchem.2013.04.126