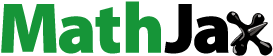
ABSTRACT
The flour film (75% w/v) with 40% w/w plasticizer/waste flour, at 2:1 w/w glycerol:sorbitol and formulated with 20% w/w potassium sorbate/waste flour, was used to cover fresh strawberries on polystyrene foam, and the quality of the fruit was monitored during storage at 5°C and 90% relative humidity for 9 days. The results revealed that the fresh strawberries covered with such film had average phenolic contents, free radical antioxidant activity, and firmness equal to 326.12 mg gallic acid/100 g fresh strawberry and 2.66 mg Trolox/g strawberry, and 0.27 N, respectively. These values were higher than those obtained for the strawberries covered with film having no added potassium sorbate, and the strawberries with no film (control), respectively. Also, among the samples studied, the total plate counts (log CFU/g) of the stored strawberries covered with film, containing 20% w/w potassium sorbate/waste flour, were lower.
Graphical Abstract
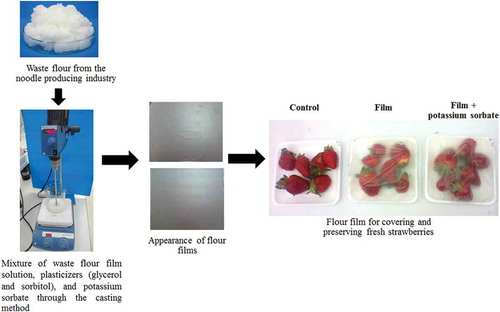
RESUMEN
En el presente estudio se utilizó una película de harina (75% p/v) con 40% p/p plastificante/harina de desperdicio, en 2:1 p/p glicerol: sorbitol, y formulada con 20% p/p sorbato potásico/harina de desperdicio, para cubrir fresas frescas en poliestireno expandible; posteriormente, se monitoreó la calidad de la fruta durante su almacenamiento a 5°C y 90% humedad relativa (HR) durante 9 días. Los resultados obtenidos permitieron constatar que las fresas frescas cubiertas con dicha película mostraron un contenido de fenólicos promedio, actividad antioxidante de radicales libres y firmeza equivalentes a 326.12 mg ácido gálico/100 g fresas frescas, 2.66 mg Trolox/g de fresas y 0.27 N, respectivamente. Estos valores superan los registrados para las fresas cubiertas con película sin sorbato potásico agregado y para las fresas sin película (control). Además de las muestras estudiadas, los recuentos por placa totales (log CFU/g) de las fresas almacenadas cubiertas con película con 20% p/p sorbato potásico/harina de desperdicio fueron más bajos.
KEYWORDS:
PALABRAS CLAVE:
Introduction
Synthetic polymer films are used in daily life. Moreover, the volumes of production and consumption of these plastics are continuously increasing. Most plastics originate from petroleum-based hydrocarbons and are, therefore, produced from nonrenewable materials. The inability of synthetic plastics to biodegrade means they must be incinerated or placed into landfill (Parra, Tadini, Ponce, & Lugão, Citation2004; Rochman et al., Citation2013; Rodríguez, Osés, Ziani, & Mate, Citation2006; Xu, Kim, Hanna, & Nag, Citation2005). Consequently, there is a growing concern about the impact of petroleum-based plastics on the environment, which has motivated several research studies on the manufacture of biodegradable films (Alves, Mali, Beléia, & Grossmann, Citation2007; Gutiérrez, Tapia, Pérez, & Famá, Citation2015; Seligra, Jaramillo, Famá, & Goyanes, Citation2016; Taqi, Askar, Nagy, Mutihac, & Stamatin, Citation2011). A suitable, naturally renewable polymer must display similar properties to those of synthetic plastics, meet the standards of a biodegradable product, and not harm the environment (Montero, Rico, Rodríguez-Llamazares, Barral, & Bouza, Citation2017; Mooney, Citation2009; Rodríguez et al., Citation2006).
Several interesting natural polymers used for the preparation of biodegradable films include polysaccharides, proteins, and lipids (Kuorwel, Cran, Sonneveld, Miltz, & Bigger, Citation2011; Pan, Jiang, Chen, & Jin, Citation2014; Shit & Shah, Citation2014; Zhao, Torley, & Halley, Citation2008). Flour is made from a starchy food material that contains a high proportion of complex carbohydrates, also known as polysaccharides. Therefore, flour can be used to produce edible and biodegradable films. Flour films have low oxygen permeability rates. When used to coat fresh-cut fruits, they aid to minimize the respiration rate of fruits. Furthermore, flour films are transparent and odorless, so, they do not affect the flavor and properties of the products (Chiumarelli & Hubinger, Citation2012; Chiumarelli, Pereira, Ferrari, Sarantópoulos, & Hubinger, Citation2010; García, Pereira, Sarantópoulos, & Hubinger, Citation2010). However, flour films have high hygroscopicity and a high water vapor transmission rate (WVTR) (Vargas, Pastor, Chiralt, McClements, & González-Martínez, Citation2008). Nevertheless, natural polysaccharides are highly diverse in nature, and polysaccharide derivatives offer opportunities to further enhance the properties of polysaccharide-based films (Kester & Fennema, Citation1986). Selection of the natural polymer(s), for producing edible and degradable films, should be based on the types of products to be contained and the storage conditions of such products. Moreover, the films should have the following properties: (1) appropriate sensory, gas transmission and mechanical properties; (2) proper biochemical, physicochemical, and microbiological stability; (3) no harmful substances and be safe for consumers; (4) simple production procedures; (5) no air pollutants; and (6) high availability and low cost for both the raw materials and production process (Fakhoury et al., Citation2012).
Flour films are probably naturally degradable, which can minimize the steps of collecting and eliminating the used films. Therefore, this study aimed to focus on the production of a natural polymer film using the waste flour from the noodle-producing industry to produce flour films, allowing reuse of the waste flour and creation of an added-value product. The type and concentration of plasticizer (glycerol and sorbitol) and their mixtures on the physical and mechanical properties of the produced films were examined. Finally, the most suitable flour films were used to cover and preserve fresh strawberries.
Materials and methods
Materials
Waste flour from the rice noodle steaming process, at the noodle-producing factory of Isariyaphon Limited Partnership in Chiang Mai, Thailand, was kept in a sealed plastic bag at 4°C. Strawberries were obtained from Rimping Supermarket, Chiang Mai, Thailand. Glycerol (99% purity, food grade), d-sorbitol (99% purity), and potassium sorbate (≥ 98%) were purchased from Union Science, Thailand. Rice flour purchased from Bangkok Inter Food Co., Ltd., Thailand, was analyzed to confirm the presence of the rice flour in the waste flour using Fourier transform infrared (FTIR) and differential scanning calorimetry (DSC).
Chemical and microbiological properties of waste flour
Proximate composition analysis
The proximate composition (moisture, ash, crude fat, crude fiber, and protein) of the waste flour was analyzed according to the Association of Analytical Chemists’ standard methods (AOAC, Citation2000). Available carbohydrate content was determined by difference. Amylose content was determined by adopting the method of Juliano (Citation1971). The results were expressed as the mean of three replicates.
FTIR analysis
FTIR spectra of the waste flour and rice flour were recorded using a Nicolet iS10 FTIR spectrometer (Thermo Fisher Scientific, USA) in the wavelength range 400–4000 cm−1. The measurements were performed on 2 mg of the completely dried film, pre-ground and dispersed in 200 mg KBr (pellet procedure). Signal averages were obtained at 4 cm−1 resolution.
Thermal analysis by DSC
The gelatinization temperatures of waste flour and rice flour were determined using a DSC823 Mettler Toledo Schwerzenbach differential scanning calorimeter (Mettler Toledo, Switzerland). A sample (5 mg each) contained in an aluminum pan was heated in the range of 45–120°C at a scanning rate of 5°C/min. Gelatinization temperature was determined from the thermogram. All samples were run in triplicate.
Microbiological analysis
Microbiological analysis was done to determine the microbial stability of waste flour over time. A 10-g aliquot of waste flour was aseptically removed from each bag and homogenized in 90 mL of sterilized physiological solution. A fivefold serial dilution was done and each dilution was plated out in triplicate on plate count agar for enumeration of aerobic mesophilic bacteria, and on potato dextrose agar for mold and yeast count using the pour plate method. The plates were allowed to cool and, then, incubated at 37°C for 48 h (bacteria) and at 25°C for 5 days (yeast and mold). Visible colonies were counted after incubation, and the results were reported as log colony-forming units (CFU)/g.
Preparation of flour film solution
Waste flour film solutions (75% w/v) were heated at 90°C, stirred, and added to glycerol, sorbitol, and glycerol:sorbitol mixtures (at ratios of 1:1, 2:1, 3:1, and 4:1 w/w), respectively. These plasticizers were tested at five concentrations: 0%, 10%, 20%, 30%, 40%, and 50% w/w plasticizer/waste flour. The mixture was stirred at 400 rpm for 15 min. It was then molded into shapes by pouring it on acrylic sheets for templates (width × length × depth, 15 × 25 × 0.10 cm) through the casting technique. The film was dried at 55°C for 24 h, then cooled to room temperature (27–30°C) for 24 h. All films were prepared in triplicate including films without plasticizers, which were used as controls. Dried flour films were peeled off to measure color (L*, a*, and b*), and total color difference (ΔE), thickness, tensile strength, elongation at break, film solubility, and water vapor permeability (WVP).
Film thickness
Film thickness (±0.001 mm) was determined with a digital micrometer (Mitutoyo Co., Japan). The thickness measurements were obtained from 15 different film areas per sample. The mean value of the measurements for each sample was then calculated.
Tensile strength and elongation at break
All of the tested films (cut to 25 × 150 mm dimensions) were equilibrated at 27 ± 2°C, 65% ± 2% relative humidity (RH), for at least 24 h before testing with an HWS Intelligent Constant Temperature and Humidity machine (Ningbo, China). Tensile strength (N) and elongation at break (%) were measured by using an Instron universal testing machine (model 1000, H1K-S, UK) according to the American Society for Testing and Materials (ASTM, D882-80a Citation1995a) at a crosshead speed of 25 mm/min and an initial gauge length of 100 mm. Tensile strength was calculated by dividing the maximum force by the initial specimen cross-sectional area, and elongation at break (%) was calculated as follows:
where is the distance between grips holding the specimen before or after the break of the specimen. Mean values of tensile strength and elongation at break were calculated from triplicate measurements of the five samples.
Film solubility in water
Five samples (2 cm diameter) were obtained from each film and oven-dried at 105°C for 24 h. The samples were weighed to determine the initial dry matter of each film (). Each sample was immersed in 30 mL of distilled water and kept at 25 ± 2°C for 24 h. The sealed beaker was stirred periodically. The insoluble portion of the film sample was removed from the soluble matter after immersion in distilled water and, then, oven-dried at 105°C for 24 h. The oven-dried samples (Wo) were reweighed to determine the weight of the solubilized dry matter. The solubility in water was calculated as the percentage of dry matter of the solubilized film after immersion in water at 25 ± 2°C for 24 h and was determined using the following equation:
Color measurement
The color parameters of the films were determined by the CIELAB L*, a*, and b* values with the Color Quest XE colorimeter. All color measurements were carried out five times per film sample. The L*, a*, and b* parameters were determined by placing the film over the white standard and ΔE was calculated according to Eq. (3).
where ,
, and
Water vapor permeability
The WVP of the films was measured gravimetrically according to the method reported by Klangmuang and Sothornvit (Citation2016). The test cup (outer diameter × inner diameter × depth, 4.50 × 4.23 × 4.0 cm) was filled with 20 mL distilled water. The cup was closed by the respective films and was placed into a cabinet at 25°C, 50% RH. The cup had an exposed film area of 14.05 cm2. The change in weight of the cup was recorded (±0.001 g) at 1-h intervals until constant weight. The lost weight of the cup was plotted as a function of time to estimate the slope by a linear equation (the correlation coefficient was 0.99). Once the slope was obtained, the WVTR and the WVP were calculated as follows:
where WVTR is the water vapor transmission rate (g/m2·day), WVP is the water vapor permeability (g mm/m2 day kPa), m is weight (g), t is time (day), is the slope of the straight line (weight loss per unit time) (g/day), L is the mean film thickness (mm), A is the test area (m2), and
is the water vapor partial pressure difference across the films (kPa).
Application of flour films for covering and preserving fresh strawberries
To produce a film that achieved optimum mechanical properties, the suitable films from the previous section thus were chosen to further develop by adding potassium sorbate at three concentrations (10%, 20%, and 30% w/w potassium sorbate/waste flour) to prevent fungi and mold growth. The film properties such as tensile strength, elongation at break, film solubility, and WVP were measured. WVP of the films was measured using a cup method at approximately 25°C, 50% RH and 5°C, 90% RH. Next, the suitable films were used to cover the fresh strawberries on a polystyrene foam tray that were subsequently stored at 5°C, 90% RH for 9 days. The quality of the strawberries was assessed at 0, 3, 6, and 9 days, for color (L*, a*, and b*), phenolic compounds, 2,2-diphenyl-1-picrylhydrazyl (DPPH) antioxidant activity, total soluble solids (°Brix), weight loss (%), texture, and microbiological analysis (i.e. total plate counts, yeast, and fungi).
Statistical analysis
All the analyses were performed in triplicate. Data were collected in Microsoft Excel 2013 (Microsoft (Thailand) Company Limited, Bangkok, Thailand) and analyzed statistically by analysis of variance, with Duncan’s multiple-range test, for post hoc comparison of the means, using SPSS Statistic Base version 17.0 for Windows (SPSS (Thailand) Company Limited, Bangkok, Thailand). Differences were considered to be significant at p ≤ 0.05.
Results and discussion
Proximate analysis of waste flour
The waste flour contained (w/w) 87.69% moisture, 9.17% carbohydrate, 2.85% protein, 0.06% fat, 0.02% ash, and 0.01% fiber. The amylose content was 0.71%.
The total plate counts in waste flour were below 30 CFU/g sample. Microbial analysis disclosed that there was no yeast and fungi present in the waste flour in accordance with the Thai Industrial Standard 959 (Citation1990) for noodle products, which specifies the total plate counts must not exceed 1 × 103 CFU/g sample, and the total yeast and fungi must be below 10 CFU/g.
The structure of waste flour and rice flour ()) analyzed via FTIR revealed both specimens had a peak at 3400 cm−1 attributable to –OH stretching due to the moisture in the flour. These flour samples showed the peak at 2931 cm−1 was attributed to C–H bond stretching. These results were consistent with the research conducted by Mopoung, Sirikulkajorn, Dummun, and Luethanom (Citation2012). The FTIR analysis indicated that the waste flour and rice flour showed absorption peaks in the same regions. This reveals that they possess similar functional groups and, therefore, promising for the production of flour-based films. Also, analysis of the flour samples (rice flour () and waste flour ()) by DSC revealed the gelatinization temperature of rice flour ranged between 73°C and 75°C (Huang, Chang, Chang, & Lii, Citation1994; Tapia-Blácido, Sobral, & Menegalli, Citation2010). There was no gelatinization temperature for waste flour ()), however, because the flour was removed from the noodle steaming process and, hence, already gelatinized.
Types and amounts of plasticizers suited to the flour-based film production
The type (glycerol and sorbitol) and amount (10%, 20%, 30%, 40%, and 50% w/w, plasticizer/waste flour) of plasticizer(s) suited to prepare films made from the waste flour (75% w/v) were studied. The obtained results are shown in .
Figure 2. Films produced from waste flour (75% w/v) with glycerol at (a) 10%, (b) 20%, (c) 30%, (d) 40%, and (e) 50% w/w, plasticizer/waste flour, respectively, and sorbitol at (f) 10, (g) 20, (h) 30, (i) 40, and (j) 50% w/w, plasticizer/waste flour.
Figura 2. Películas producidas de harina de desperdicio (75% p/v) con glicerol a (a) 10 (b) 20 (c) 30 (d) 40 y (e) 50% p/p, plastificante/harina de desperdicio, respectivamente, y sorbitol a (f) 10, (g) 20, (h) 30, (i) 40 y (j) 50% p/p, plastificante/harina de desperdicio.
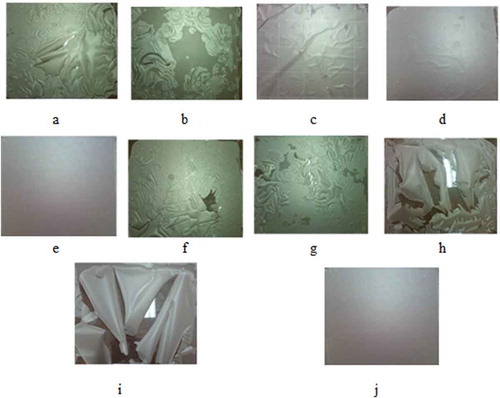
The flour-based films with 10% and 20% w/w glycerol/waste flour were breakable (, )). From , ), the flour-based films with 30% and 40% w/w glycerol/waste flour were white and flexible, and the side exposed to the air was rough. In comparison, the films with 50% w/w glycerol/waste flour ()) were more transparent, flexible, and softer. Adding plasticizers, like glycerol, during the production softens the films because molecular bonds between adjacent biopolymers within the films are demoted over plasticizer–biopolymer bonds (Sanyang, Sapuan, Jawaid, Ishak, & Sahari, Citation2015). In accordance with Laohakunjit and Noomhorm (Citation2004), films produced from rice starch with 20–35% glycerol were transparent and flexible, and one side of the films was rough, while the other was glittery. Furthermore, the authors noted that among the samples studied the films prepared with 30% and 35% glycerol were softer.
With respect to the films produced from waste flour with added sorbitol, those with 10%, 20%, 30%, and 40% w/w sorbitol/waste flour were breakable and inflexible (–i)), whereas the film with 50% w/w sorbitol/waste flour exhibited unacceptable physical appearances. The film was too dry and breakable when peeling off the templates. Therefore, at this condition, the flexible film could not be obtained and it was not possible to measure its mechanical and physical properties. However, sorbitol is one type of plasticizer that helps minimize the WVP of films. Besides, in sufficient amounts, sorbitol is able to strengthen films (Sanyang et al., Citation2015).
Laohakunjit and Noomhorm (Citation2004) prepared rice starch films with glycerol and sorbitol at 20%, 25%, 30%, 35%, 40%, and 45% w/w, respectively, and found that irrespective of the level of plasticizer added, the films with glycerol showed greater WVP and film solubility than sorbitol. Besides possessing a larger molar mass than glycerol, sorbitol has more hydroxyl groups to strongly interact with biopolymers by hydrogen bonding. Consequently, sorbitol shows a lower capacity to interact with water molecules, thereby reducing the WVP of films more effectively compared to glycerol (Dick et al., Citation2015; McHugh & Krochta, Citation1994). The effect of using mixture of glycerol and sorbitol as plasticizer on film production and application was further elucidated in subsequent sections.
Effect of glycerol: sorbitol ratio on film production from waste flour
Films were produced from waste flour (75% w/v) with 30%, 40%, and 50% w/w plasticizers (waste flour solution basis) before being adjusted to 1:1, 2:1, 3:1, and 4:1 w/w glycerol:sorbitol. The properties of the produced films disclosed that flour films with 30% w/w plasticizers/waste flour, irrespective of the glycerol:sorbitol ratio, were breakable, fragile, and inflexible (). However, the films broke into pieces when removed from the template ()). Consequently, 75% w/v waste flour with 30% w/w plasticizers/waste flour at every glycerol:sorbitol w/w ratio investigated was not suited to the production of flour-based films.
Figure 3. Films produced from waste flour (75% w/v), with plasticizers at 30% w/w plasticizers/waste flour, using glycerol:sorbitol at (a) 1:1, (b) 2:1, (c) 3:1, and (d) 4:1 w/w ratio.
Figura 3. Películas producidas de harina de desperdicio (75% p/v), con plastificantes a 30% p/p plastificantes/harina de desperdicio, usando ratios de glicerol: sorbitol de (a) 1:1, (b) 2:1, (c) 3:1 y (d) 4:1 p/p.
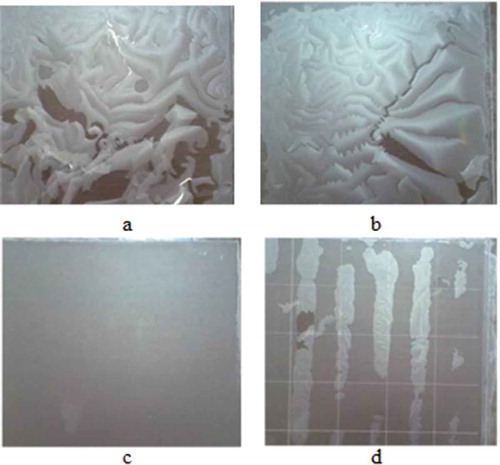
The film produced from 40% w/w plasticizers/waste flour at 1:1 w/w glycerol:sorbitol () was smooth but unable to be removed from the template. Meanwhile, films at 1:1 (50% w/w plasticizers/waste flour), and 2:1, 3:1, and 4:1 w/w glycerol: sorbitol [at 40% (), (c), and (d), respectively) and 50% w/w plasticizers/waste flour] were successfully produced, removed from the template, strong, and unbreakable (as shown in and ).
Figure 4. Films produced from waste flour (75% w/v), with 40% w/w plasticizers/waste flour, at glycerol:sorbitol w/w ratios of (a) 1:1, (b) 2:1, (c) 3:1, and (d) 4:1.
Figura 4. Películas producidas de harina de desperdicio (75% p/v), con 40% p/p plastificantes/harina de desperdicio, con ratios de glicerol: sorbitol p/p de (a) 1:1, (b) 2:1, (c) 3:1 y (d) 4:1.
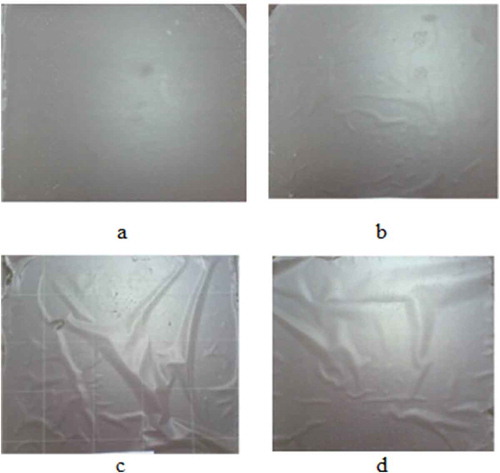
Figure 5. Films produced from waste flour (75% w/v), with 50% w/w plasticizers/waste flour, at glycerol:sorbitol w/w ratios of (a) 1:1, (b) 2:1, (c) 3:1, and (d) 4:1.
Figura 5. Películas producidas de harina de desperdicio (75% p/v), con 50% p/p plastificantes/harina de desperdicio, con ratios de glicerol: sorbitol de (a) 1:1, (b) 2:1, (c) 3:1 y (d) 4:1.
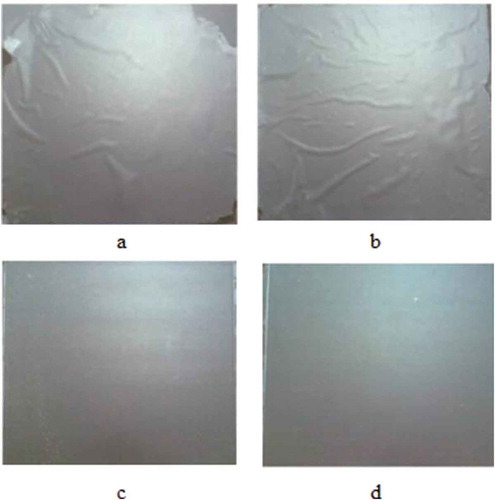
Films prepared with 1:1 (50% w/w plasticizers/waste flour), and 2:1, 3:1, and 4:1 (40% and 50% w/w, plasticizers/waste flour) w/w glycerol:sorbitol showed thickness between 0.071 and 0.076 mm () with the maximum thickness (0.076 mm) corresponding to the films having 50% w/w plasticizers/waste flour at 1:1 w/w glycerol:sorbitol (). The results illustrated slightly increase in film thickness by raising plasticizer concentration. The film thickness increased because the amount of plasticizer in the film increased while the amount of waste flour remained the same.
Table 1. Effect of glycerol:sorbitol w/w ratio on thickness, color (L*, a*, and b*), ΔE, water solubility, tensile strength, elongation at break, and water vapor permeability of waste flour (75% w/v) films produced with 40% and 50% w/w plasticizers/waste flour.
Tabla 1. Efecto de la ratio glicerol: sorbitol p/p en el grosor, color (L*, a* y b*), ΔE, solubilidad en agua, fuerza tensil, elongación de ruptura y permeabilidad de vapor de agua de películas de harina de desperdicio (75% p/v) producidas con 40 y 50% p/p plastificante/harina de desperdicio.
The L* (lightness), a* (green-red), and b* (blue-yellow) color values and ΔE of the films are tabulated in . The average color (L*, a*, and b*) and ΔE of the films with 40% w/w plasticizers/waste flour were less than the films with 50% w/w plasticizers/waste flour for the glycerol:sorbitol 2:1, 3:1, and 4:1 w/w ratios. For the films with 40% w/w plasticizers/waste flour at 2:1, 3:1, and 4:1 w/w glycerol:sorbitol, the color values were 99.84 L*, 0.60 a*, 0.44 b*; 99.87 L*, 0.61 a*, 0.38 b*, and 99.84 L*, 0.63 a*, 0.38 b*, respectively, and the corresponding ΔE averages were 0.30, 0.26, and 0.27 (). By increasing plasticizer concentration, the average value of L*, a*, and b* slightly increased. Plasticizer glycerol can produce clearer films (Farahnaky, Saberi, & Majzoobi, Citation2013); therefore, addition of glycerol to the films can improve their optical properties. Color changes due to the incorporation of glycerol can be more fully described using ΔE, which indicates the degree of total color difference from the standard color plate. The ΔE value increased with increasing plasticizer content. Although L*, a*, and b* color values and ΔE of the films increased, the changes were comparatively small.
As shown in , for all glycerol:sorbitol w/w ratios studied, the average water solubility of the films were less at 40% w/w plasticizers/waste flour (20.01%, 30.84%, and 31.19% for 2:1, 3:1, and 4:1, respectively) than at 50% w/w (31.60%, 30.99%, 33.70%, and 33.95%, for 1:1, 2:1, 3:1, and 4:1, respectively). The film produced, using glycerol:sorbitol at 4:1 w/w with 50% w/w plasticizers/waste flour, showed the highest water solubility because glycerol and sorbitol are water-soluble, flexible substances that promoted the solubility of the polysaccharide molecules through the extensive hydrogen bonding, enabled by their high contents in the film. Similarly, Müller, Yamashita, and Laurindo (Citation2008) documented increased water solubility of tapioca starch-based films with increased content of sorbitol and, in particular, glycerol, due to its relatively higher mobility (smaller molar mass) and hygroscopicity than sorbitol (Dick et al., Citation2015; Mathew & Dufresne, Citation2002; Sanyang et al., Citation2015).
The film with the highest tensile strength (1.65 MPa) and elongation at break (63.61%) was prepared using 40% w/w plasticizers/waste flour at 2:1 w/w glycerol:sorbitol (). In comparison, at 3:1 and 4:1 w/w glycerol:sorbitol, the tensile strength (1.33 and 1.26 MPa, respectively) and elongation at break (55.61% and 54.79%, respectively) of the films decreased. From , films with 50% w/w plasticizers/waste flour at 1:1, 2:1, 3:1, and 4:1 w/w glycerol:sorbitol presented lower tensile strength (1.30, 1.53, 1.14, and 1.45 MPa, respectively) and elongation at break (48.75%, 38.18%, 34.67%, and 34.49%, respectively) than films with 40% w/w plasticizers/waste flour, at all glycerol:sorbitol ratios.
The 1:1, 2:1, 3:1, and 4:1 w/w glycerol:sorbitol ratios were achieved by increasing the glycerol content (adjusted weight ratios shown in ). Increasing the glycerol helped soften the film structure, and the obtained films were flexible plastics, thus, glycerol effectively decreased the tensile strength but increased the elongation at break. McHugh and Krochta (Citation1994), and Laohakunjit and Noomhorm (Citation2004) reported similar results for rice starch-based films. However, the current study results showed that both tensile strength and elongation at break of the films were likely to decrease. The tensile strength of flour-based films decreased as plasticizer concentration increased. This phenomenon can be attributed to the role of plasticizers in weakening the strong intermolecular attraction and enhancing the formation of hydrogen bonds between plasticizers and biopolymer molecules. Therefore, it reduces the tensile strength of plasticized films by subsequently diminishing the hydrogen bonds interaction between biopolymer chains (Sanyang et al., Citation2015). Meanwhile, the decrease in elongation at break of films can be explained through glycerol migration from film matrix. The migration of glycerol from the film network enables the strong intermolecular forces along biopolymer chains, thus, reducing the elongation at break of the films. This phenomenon occurs when the plasticizer concentration exceeds its compatibility levels (Sanyang et al., Citation2015).
According to the WVP results (), irrespective of the glycerol:sorbitol w/w ratio (2:1, 3:1, and 4:1), the films with 40% w/w plasticizers/waste flour showed lower WVP than films with 50% w/w, plasticizers/waste flour. At 2:1 w/w glycerol:sorbitol, the films with 40% w/w plasticizers/waste flour showed the lowest WVP (average 141.85 g mm/m2 day kPa), which increased to 148.32 and 159.24 g mm/m2 day kPa at 3:1 and 4:1 w/w glycerol:sorbitol, respectively.
Films with 50% w/w plasticizers/waste flour at 1:1, 2:1, 3:1, and 4:1 w/w glycerol:sorbitol showed increasing WVP averages of 169.96, 180.86, 189.44, and 192.88 g mm/m2 day kPa, respectively. However, the WVP of the 3:1 and 4:1 w/w glycerol:sorbitol films were not significantly different (p > 0.05) ().
The results of Cerqueira, Souza, Teixeira, and Vicente (Citation2012) demonstrated that partial replacement of glycerol by sorbitol lowered the WVP of chitosan-based films because, as above-mentioned, sorbitol is a larger molecule and has lower hygroscopicity than glycerol. Thus, increasing the glycerol content allowed the films to absorb more water, resulting in an increase in the WVP. Similar findings were documented for chitosan–rice flour films (Bourtoom & Chinnan, Citation2008) and plasticized waxy maize starch films (Mathew & Dufresne, Citation2002).
In summary, a mixture of glycerol and sorbitol for film production from waste flour revealed that film with 75% w/v waste flour and 40% w/w plasticizers/waste flour was the most suitable film, particularly when a combination of glycerol and sorbitol was used at 2:1 w/w glycerol:sorbitol. This film possessed the highest tensile strength (1.65 ± 0.15 MPa) and elongation at break (63.61% ± 0.96%), along with the least water solubility (20.01% ± 2.05%), and WVP (141.85 ± 6.04 g mm/m2 day kPa) ().
Application of flour-based films for covering the fresh strawberries and quality of strawberries preserved by flour films
The film with the optimal mechanical properties (i.e. this prepared using 75% w/v waste flour with 40% w/w plasticizers/waste flour and 2:1 w/w glycerol: sorbitol) was selected to investigate their ability to cover and preserve fresh strawberries during storage. The antimicrobial agent potassium sorbate (10%, 20%, and 30% w/w potassium sorbate/waste flour, respectively) was incorporated into the selected film before analysis.
The tensile strength of the film was decreased while the elongation at break of the film was increased by the addition of potassium sorbate (). Perhaps, potassium sorbate filled the intermolecular spaces within the film decreasing in intermolecular forces in the films and further increasing the mobility of the biopolymer molecules (Barzegar, Azizi, Barzegar, & Hamidi-Esfahani, Citation2014). With regard to water solubility and WVP (), the results showed that the water solubility of the films decreased with increasing potassium sorbate content (0%, 10%, 20%, and 30% w/w potassium sorbate/waste flour). Adding potassium sorbate would increase the polarity of the films, and their ability to absorb water suggesting that the water solubility of films would be higher. However, in this research, the films showed lower solubility as less potassium sorbate dissolved in the film dissolutions or potassium sorbate presented a solid form in the film dissolutions. Upon filtration to determine the solubility, the potassium sorbate crystals might block the holes of the filter paper interfering with the ability of the film solutions to flow through the filter paper, which resulted in a lower water solubility.
Table 2. Properties of films produced from 75% w/v waste flour with 40% w/w plasticizers/waste flour and 2:1 w/w glycerol:sorbitol, with or without potassium sorbate.
Tabla 2. Propiedades de películas producidas de 75% p/v harina de desperdicio con 40% p/p plastificante/harina de desperdicio y 2:1 p/p glicerol: sorbitol, con o sin sorbato potásico.
From , the WVP of the films was increased by the incorporation of potassium sorbate, which helped increase the polarity of films, enabling them to better absorb the water, thereby increasing the WVP. Nevertheless, there was no significant difference (p > 0.05) in the WVP of the films with 10%, 20%, and 30% w/w potassium sorbate/waste flour.
Based on the above-mentioned study results, the film produced from 75% w/v waste flour and 40% w/w plasticizers/waste flour at 2:1 w/w glycerol:sorbitol with 20% w/w potassium sorbate/waste flour was used to cover fresh strawberries on a foam tray (). This film was flexible with a maximum average elongation at break of 73.01%. The quality of the covered fresh strawberries was monitored during storage at 5°C and 90% RH for 9 days.
Figure 6. Application of waste flour-based films for covering and preserving fresh strawberries stored at 5°C and 90% RH for 9 days.
Figura 6. Aplicación de películas basadas en harina de desperdicio para cubrir y conservar fresas frescas almacenadas a 5°C y 90% HR durante 9 días.
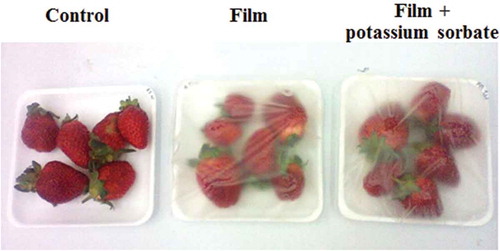
Strawberry is a non-climacteric fruit, which must be harvested when about 75% of the whole fruit is red and still firm (Nunes, Brecht, Morais, & Sargent, Citation2006). Maintaining the quality of strawberries, before reaching the customer, may help minimize customer rejection and thereby fruit waste.
The quality of the strawberries was analyzed every 3 days. These analyses included the color (L*, a*, and b*), phenol components, DPPH antioxidant activity, water-soluble solids, weight loss, firmness, and total plate counts. Uncovered fresh strawberries preserved under the same conditions were used as a control. The results () revealed that the color parameters (L*, a*, and b*) of fresh strawberries preserved by no film (control), the film with 2:1 w/w glycerol:sorbitol, and the film with 2:1 w/w glycerol:sorbitol and 20% (w/w) potassium sorbate/waste flour were not significantly different (p > 0.05). For all samples, the lightness (L*) and yellow tone (b*) tended to decrease, whereas the red tone (a*) tended to increase when preserved for 3 and 6 days; the red tone (a*) decreased, when preserved for 9 days.
Table 3. The colors (L*, a*, and b*) of fresh strawberries preserved with no film (control), film with 2:1 w/w glycerol:sorbitol, and film with 2:1 w/w glycerol:sorbitol and 20% w/w potassium sorbate/waste flour (film + ks).
Tabla 3. Colores (L*, a* y b*) de fresas frescas conservadas sin película (control), con película de 2:1 p/p glicerol: sorbitol, y película con 2:1 p/p glicerol: sorbitol y 20% p/p sorbato potásico/harina de desperdicio (película+ks).
shows the phenol content, DPPH antioxidant activity, water-soluble solids, weight loss, firmness, and total plate counts of the fresh strawberries preserved by the three different treatments. The phenol contents and DPPH antioxidant activity increased on day 3, then, decreased with further storage. The strawberries covered with films produced from 2:1 w/w glycerol: sorbitol and 20% w/w potassium sorbate/waste flour had an average phenol content of 326.12 mg gallic acid/100 g fresh strawberry, and an average DPPH antioxidant activity of 2.66 mg Trolox/g fresh strawberry. These values were higher (p ≤ 0.05) than the fresh strawberries covered with no film (control) and strawberries covered with films having 2:1 w/w glycerol:sorbitol, respectively.
Table 4. Phenol content, DPPH antioxidant activity, water-soluble solids, weight loss, firmness, and total plate counts of fresh strawberry preserved with no film (control), film having 2:1 w/w glycerol:sorbitol, and film having 2:1 w/w glycerol:sorbitol and 20% w/w potassium sorbate (ks)/waste flour (film + ks).
Tabla 4. Contenido de fenol, actividad antioxidante DPPH, sólidos solubles en agua, pérdida de peso, firmeza y recuento de placas totales de fresas frescas conservadas sin película (control), con película con 2:1 p/p glicerol: sorbitol y 20% p/p sorbato potásico (ks)/harina de desperdicio (película+ks).
From , the water-soluble solids increased with increased storage duration. The water-soluble solids of the strawberries with no film had the highest average of 14.67 °Brix, followed by the strawberries covered by the film with 2:1 w/w glycerol:sorbitol (12.67 °Brix), then the strawberries with the film containing 2:1 w/w glycerol:sorbitol and 20% w/w potassium sorbate/waste flour (11.67 °Brix). The soluble solids of strawberry were consistent with the weight loss of the fruit, which averaged 49.99% (control), 26.31% (2:1 w/w glycerol: sorbitol), and 24.50% (2:1 w/w glycerol:sorbitol and 20% w/w potassium sorbate/waste flour). Irrespective of the treatment, the firmness of the strawberries was not significantly different (p > 0.05) during storage with averages of 0.22 (control), 0.25 (2:1 w/w glycerol:sorbitol), and 0.27 N (2:1 w/w glycerol:sorbitol and 20% w/w potassium sorbate/waste flour) ().
Additionally, irrespective of the treatment, the total plate counts of the strawberry fruit increased with increased storage duration. The strawberry coated with films having 2:1 w/w glycerol:sorbitol had the highest total plate counts (average of 6.07 log CFU/g), probably caused by a contamination of the coating films, which affected the preserved strawberry. No yeast and fungi were present in any of the fruit throughout the 9-day storage.
Shin, Ryu, Liu, Nock, and Watkins (Citation2008) reported that the overall quality of strawberry (firmness, color, flavonoids, phenol components, and antioxidant activity) kept at 10°C for 12 days, decreased according to the storage duration. Likewise, Peretto et al. (Citation2014) studied the quality (weight loss, appearance, firmness, color, water-soluble solids, phenol components, antioxidant activity, and inhibition of microorganisms) of strawberries preserved by films produced from strawberry puree with added carvacrol, methyl, and cinnamate, and kept at 10°C for 10 days, and found that the firmness and lightness decreased, whereas the water-soluble solids, phenol contents, antioxidant activity, and inhibition of microorganisms increased throughout storage.
Considering the strawberry quality, based on phenol content, DPPH antioxidant activity and firmness, throughout the storage (5°C and 90% RH, for 9 days) (), the flour-based film shows promising potential for application as a coating for fresh strawberry (). Throughout the storage, the strawberry coated with film produced from 75% w/v waste flour solution having 40% w/w plasticizers/waste flour at 2:1 w/w glycerol:sorbitol with potassium sorbate showed higher phenol contents, DPPH antioxidant activity, and firmness than the strawberry coated with film produced from 75% w/v waste flour solution having 40% w/w plasticizers/waste flour at 2:1 w/w glycerol:sorbitol (with no potassium sorbate), and the control (strawberries with no film), respectively. In addition, the fresh strawberry coated with film containing potassium sorbate showed average total plate counts of 4.95 log CFU/g, which was less than the fruit coated with films containing no potassium sorbate.
Conclusions
Waste flour from rice noodle-producing industry can be used to produce flour film, commonly used as a natural packaging material. Films prepared with 75% w/v waste flour having 40% w/w plasticizers/waste flour at 2:1, 3:1, and 4:1 w/w glycerol:sorbitol showed the possibility of producing useful films. However, at 2:1 w/w glycerol: sorbitol, the film was the most suitable film that possessed the optimal tensile strength (1.65 MPa) and elongation at break (63.61%), along with the least water solubility (20.01%) and WVP (141.85 g mm/m2 day kPa). The developed film with the optimal mechanical properties, formulated with 20% w/w potassium sorbate/waste flour, can be applied for wrapping and preserving fresh strawberries over a foam tray, during storage at 5°C and 90% RH for 9 days. The film was able to better preserve the quality of the fruit, such as firmness, antioxidants, and phenol content, than the film with no potassium sorbate. Additionally, the strawberries covered with film containing potassium sorbate had total plate counts (log CFU/g) less than the strawberries covered with film that was not formulated with potassium sorbate. Thus, waste flour film could be a promising alternative material to reduce the use of nonbiodegradable synthetic polymer films in food applications.
Acknowledgment
The authors thank the Graduate School of Chiang Mai University for their financial support.
Disclosure statement
No potential conflict of interest was reported by the authors.
References
- Alves, V. D., Mali, S., Beléia, A., & Grossmann, M. V. E. (2007). Effect of glycerol and amylose enrichment on cassava starch films properties. Journal of Food Engineering, 78, 941–946. doi:10.1016/j.jfoodeng.2005.12.007
- AOAC. (2000). Official methods of analysis (17th ed.). Gaithersburg, MD: Association of Official Analytical Chemists.
- ASTM. (1995a). Standard test method for tensile properties of thin plastic sheeting. ASTM D882-80a. In Annual book of ASTM standards. Philadelphia, PA: American Society for Testing and Materials.
- Barzegar, H., Azizi, M. H., Barzegar, M., & Hamidi-Esfahani, Z. (2014). Effect of potassium sorbate on antimicrobial and physical propertiesof starch–Clay nanocomposite films. Carbohydrate Polymers, 110, 26–31. doi:10.1016/j.carbpol.2014.03.092
- Bourtoom, T., & Chinnan, M. S. (2008). Preparation and properties of rice starch–Chitosan blend biodegradable film. LWT - Food Science and Technology, 41, 1633–1641. doi:10.1016/j.lwt.2007.10.014
- Cerqueira, M. A., Souza, B. W. S., Teixeira, J. A., & Vicente, A. A. (2012). Effects of interactions between the constituents of chitosan-edible films on their physical properties. Food and Bioprocess Technology, 5, 3181–3192. doi:10.1007/s11947-011-0663-y
- Chiumarelli, M., & Hubinger, M. D. (2012). Stability, solubility, mechanical and barrier properties of cassava starch – Carnauba wax edible coatings to preserve fresh-cut apples. Food Hydrocolloids, 28, 59–67. doi:10.1016/j.foodhyd.2011.12.006
- Chiumarelli, M., Pereira, L. M., Ferrari, C. C., Sarantópoulos, C. I. G. L., & Hubinger, M. D. (2010). Cassava starch coating and citric acid to preserve quality parameters of fresh-cut “Tommy Atkins” mango. Journal of Food Science, 75, E297–E304. doi:10.1111/j.1750-3841.2010.01636.x
- Dick, M., Costa, T. M. H., Gomaa, A., Subirade, M., de Oliveira Rios, A., & Flôres, S. H. (2015). Edible film production from chia seed mucilage: Effect of glycerol concentration on its physicochemical and mechanical properties. Carbohydrate Polymers, 130, 198–205. doi:10.1016/j.carbpol.2015.05.040
- Fakhoury, F. M., Martelli, S. M., Bertan, L. C., Yamashita, F., Mei, L. H. I., & Queiroz, F. P. C. (2012). Edible films made from blends of manioc starch and gelatin – Influence of different types of plasticizer and different levels of macromolecules on their properties. LWT - Food Science and Technology, 49, 149–154. doi:10.1016/j.lwt.2012.04.017
- Farahnaky, A., Saberi, B., & Majzoobi, M. (2013). Effect of glycerol on physical and mechanical properties of wheat starch edible films. Journal of Texture Studies, 44, 176–186. doi:10.1111/jtxs.12007
- García, L. C., Pereira, L. M., Sarantópoulos, C. I. G. D. L., & Hubinger, M. D. (2010). Selection of an edible starch coating for minimally processed strawberry. Food and Bioprocess Technology, 3, 834–842. doi:10.1007/s11947-009-0313-9
- Gutiérrez, T. J., Tapia, M. S., Pérez, E., & Famá, L. (2015). Structural and mechanical properties of edible films made from native and modified cush-cush yam and cassava starch. Food Hydrocolloids, 45, 211–217. doi:10.1016/j.foodhyd.2014.11.017
- Huang, R. M., Chang, W. H., Chang, Y. H., & Lii, C. Y. (1994). Phase transitions of rice starch and flour gels. Cereal Chemistry, 71, 202–207.
- Juliano, B. O. (1971). A simplified assay for milled-rice amylose. Cereal Science Today, 16, 334–340.
- Kester, J. J., & Fennema, O. R. (1986). Edible films and coatings: A review. Food Technology, 40(12), 47–59.
- Klangmuang, P., & Sothornvit, R. (2016). Combination of beeswax and nanoclay on barriers, sorption isotherm and mechanical properties of hydroxypropyl methylcellulose-based composite films. LWT - Food Science and Technology, 65, 222–227. doi:10.1016/j.lwt.2015.08.003
- Kuorwel, K. K., Cran, M. J., Sonneveld, K., Miltz, J., & Bigger, S. W. (2011). Antimicrobial activity of biodegradable polysaccharide and protein-based films containing active agents. Journal of Food Science, 76, R90–R102. doi:10.1111/j.1750-3841.2011.02102.x
- Laohakunjit, N., & Noomhorm, A. (2004). Effect of plasticizers on mechanical and barrier properties of rice starch film. Starch/Starke, 56, 348–356. doi:10.1002/star.200300249
- Mathew, A. P., & Dufresne, A. (2002). Plasticized waxy maize starch: Effect of polyols and relative humidity on material properties. Biomacromolecules, 3(5), 1101–1108. doi:10.1021/bm020065p
- McHugh, T. H., & Krochta, J. M. (1994). Sorbitol- vs glycerol-plasticized whey protein edible films: Integrated oxygen permeability and tensile property evaluation. Journal of Agricultural and Food Chemistry, 42(4), 841–845. doi:10.1021/jf00040a001
- Montero, B., Rico, M., Rodríguez-Llamazares, S., Barral, L., & Bouza, R. (2017). Effect of nanocellulose as a filler on biodegradable thermoplastic starch films from tuber, cereal and legume. Carbohydrate Polymers, 157, 1094–1104. doi:10.1016/j.carbpol.2016.10.073
- Mooney, B. P. (2009). The second green revolution? Production of plant-based biodegradable plastics. Biochemical Journal, 418, 219–232. doi:10.1042/BJ20081769
- Mopoung, S., Sirikulkajorn, A., Dummun, D., & Luethanom, P. (2012). Nanocarbonfibril in rice flour charcoal. International Journal of Physical Sciences, 7, 214–221. doi:10.5897/IJPS11.1615
- Müller, C. M. O., Yamashita, F., & Laurindo, J. B. (2008). Evaluation of the effects of glycerol and sorbitol concentration and water activity on the water barrier properties of cassava starch films through a solubility approach. Carbohydrate Polymers, 72, 82–87. doi:10.1016/j.carbpol.2007.07.026
- Nunes, M. C. N., Brecht, J. K., Morais, A. M., & Sargent, S. A. (2006). Physicochemical changes during strawberry development in the field compared with those that occur in harvested fruit during storage. Journal of the Science of Food and Agriculture, 86, 180–190. doi:10.1002/jsfa.2314
- Pan, H., Jiang, B., Chen, J., & Jin, Z. (2014). Blend-modification of soy protein/lauric acid edible films using polysaccharides. Food Chemistry, 151, 1–6. doi:10.1016/j.foodchem.2013.11.075
- Parra, D. F., Tadini, C. C., Ponce, P., & Lugão, A. B. (2004). Mechanical properties and water vapor transmission in some blends of cassava starch edible films. Carbohydrate Polymers, 58, 475–481. doi:10.1016/j.carbpol.2004.08.021
- Peretto, G., Du, W. X., Avena-Bustillos, R. J., Sarreal, S. B. L., Hua, S. S. T., Sambo, P., & McHugh, T. H. (2014). Increasing strawberry shelf-life with carvacrol and methyl cinnamate antimicrobial vapors released from edible films. Postharvest Biology and Technology, 89, 11–18. doi:10.1016/j.postharvbio.2013.11.003
- Rochman, C. M., Browne, M. A., Halpern, B. S., Hentschel, B. T., Hoh, E., Karapanagioti, H. K., … Thompson, R. C. (2013). Policy: Classify plastic waste as hazardous. Nature, 494, 169–171. doi:10.1038/494169a
- Rodríguez, M., Osés, J., Ziani, K., & Mate, J. I. (2006). Combined effect of plasticizers and surfactants on the physical properties of starch based edible films. Food Research International, 39, 840–846. doi:10.1016/j.foodres.2006.04.002
- Sanyang, M. L., Sapuan, S. M., Jawaid, M., Ishak, M. R., & Sahari, J. (2015). Effect of plasticizer type and concentration on tensile, thermal and barrier properties of biodegradable films based on sugar palm (Arenga pinnata) starch. Polymers, 7, 1106–1124. doi:10.3390/polym7061106
- Seligra, P. G., Jaramillo, C. M., Famá, L., & Goyanes, S. (2016). Biodegradable and non-retrogradable eco-films based on starch–Glycerol with citric acid as crosslinking agent. Carbohydrate Polymers, 138, 66–74. doi:10.1016/j.carbpol.2015.11.041
- Shin, Y., Ryu, J. A., Liu, R. H., Nock, J. F., & Watkins, C. B. (2008). Harvest maturity, storage temperature and relative humidity affect fruit quality, antioxidant contents and activity, and inhibition of cell proliferation of strawberry fruit. Postharvest Biology and Technology, 49, 201–209. doi:10.1016/j.postharvbio.2008.02.008
- Shit, S. C., & Shah, P. M. (2014). Edible polymers: Challenges and opportunities. Journal of Polymers. doi:10.1155/2014/427259
- Tapia-Blácido, D. R., Sobral, P. J. A., & Menegalli, F. C. (2010). Potential of Amaranthus cruentus BRS Alegria in the production of flour, starch and protein concentrate: Chemical, thermal and rheological characterization. Journal of the Science of Food and Agriculture., 90(7), 1185–1193. doi:10.1002/jsfa.3946
- Taqi, A., Askar, K. A., Nagy, K., Mutihac, L., & Stamatin, I. (2011). Effect of different concentrations of olive oil and oleic acid on the mechanical properties of albumen (egg white) edible films. African Journal of Biotechnology, 10, 12963–12972. doi:10.5897/AJB11.1971
- Thai Industrial Standards Institute (TISI), Ministry of Industry. (1990). Industrial products standards 959–1990 of TIS rice noodles. Bangkok, Thailand: Author.
- Vargas, M., Pastor, C., Chiralt, A., McClements, D. J., & González-Martínez, C. (2008). Recent advances in edible coatings for fresh and minimally processed fruits. Critical Reviews in Food Science and Nutrition, 48, 496–511. doi:10.1080/10408390701537344
- Xu, Y. X., Kim, K. M., Hanna, M. A., & Nag, D. (2005). Chitosan–Starch composite film: Preparation and characterization. Industrial Crops and Products, 21, 185–192. doi:10.1016/j.indcrop.2004.03.002
- Zhao, R., Torley, P., & Halley, P. J. (2008). Emerging biodegradable materials: Starch- and protein-based bio-nanocomposites. Journal of Materials Science, 43, 3058–3071. doi:10.1007/s10853-007-2434-8