ABSTRACT
Evaluating the biological potential of wheat grain cultivated in northwestern Mexico is important because it mainly varies according to the cultivar and production area. The aim of the present study was to evaluate ferulic acid (FA) bioaccessibility in breads supplemented with bioprocessed bran. Bran that was bioprocessed with xylanase enzyme (EB), yeast fermentation (FB), and a combination of both treatments (FEB) showed a higher total phenol content and antioxidant capacity compared with native bran (NB), and the majority of these components were found in a bound fraction. For NB and bioprocessed bran, FA was found at higher levels compared to the other hydroxycinnamic acids evaluated. Bioprocessing increased the free FA content six-fold compared to NB. The % bioaccessibility of FA in different breads increased in the following order: FEB<NB<FB<EB. The addition of bioprocessed bran is may be the simple way to increase the amount of bioactive compounds in a bread product.
RESUMEN
La evaluación del potencial biológico de trigo cultivado en el Noroeste de México es importante porque este varía principalmente según el cultivar y área de producción. El objetivo del presente estudio fue evaluar la bioaccesibilidad del ácido ferúlico (FA) en panes adicionados con salvado bioprocesado. El salvado bioprocesado con enzima xilanasa (EB), fermentación con levadura (FB) y la combinación de ambos tratamientos (FEB) mostraron los valores más altos de contenido de fenoles totales y capacidad antioxidante que el salvado nativo (NB), en donde la fracción ligada presentó la mayor proporción respecto a la fracción libre. Tanto para el NB como para los bioprocesados, el FA se encontró en proporciones más altas respecto a los otros ácidos hidroxicinámicos evaluados. El bioprocesamiento aumentó seis veces más el contenido de FA libre en comparación con el NB. El % de bioaccesibilidad del FA en los diferentes panes se incrementó en el siguiente orden: FEB<NB<FB <EB. La adición de salvado bioprocesado puede ser una manera sencilla de aumentar los compuestos bioactivos en un producto pan.
Introduction
It is widely accepted that whole grains have many healthful properties. Several epidemiological and clinical investigations have demonstrated a significant decrease in morbidity and mortality from cardiovascular and other diseases among cereal consumers (Guo & Beta, Citation2013; Kristensen et al., Citation2012; McKeown et al., Citation2013; Slavin, Citation2004; Vitaglione, Napolitano, & Fogliano, Citation2008; Ye, Chacko, Chou, Kugizaki, & Liu, Citation2012). The positive influence of these grains is attributed to their dietary fiber and antioxidants mainly phenolic compounds. Studies have indicated in mice with diet-induced obesity that enrichment of the diet with enzymatically treated aleurone they have a tendency toward lower body weight gain, visceral adipose tissue accumulation, fasting plasma insulin, and leptin levels (Rosa et al., Citation2014). On the other hand, studies carried out in healthy men where the bioavailability from ferulic acid (FA) from the bioprocessed bread was two- to three-fold higher that from the control bread. They also found that after ex vivo stimulation with LPS the pro-anti-inflammatory cytokines in blood were significantly lower (Mateo-Anson, Aura, et al., Citation2011). For other hand, it is important to note that free FA can be absorbed directly at the gastrointestinal level, transported, biotransformed and exert its biological action. Additionally, we have to consider that FA bound to food matrix not absorbed, can be released by bacterial enzymes of microbiota present in the large intestine, creating a local antioxidant environment with different health benefits (Pekkinen et al., Citation2014).
In cereal grains, the most abundant phenolic compounds are hydroxycinnamic acids (HCA); among them, FA is the major component, followed by diferulic, sinapic, ρ-coumaric, and caffeic acids. A high portion of the FA (98%) is found in the outer parts of the wheat grain (aleurone layer and pericarp) (Andersson, Dimberg, Åman, & Landberg, Citation2014; Brewer, Kubola, Siriamornpun, Herald, & Shi, Citation2014; Ragaee, Guzar, Abdel-Aal, & Seetharaman, Citation2012).
However, in wheat, FA is linked to the α-L-arabinofuranose side-chains of cell wall arabinoxylans by ester bonds at position O-5; in wheat, FA represents up to 90% of the total phenolic acids, and 99% of this is bound, thus reducing bioaccessibility and consequent bioavailability (Itagaki et al., Citation2009; Kroon, Faulds, Ryden, Robertson, & Williamson, Citation1997; Mateo-Anson, Van Den Berg, et al. Citation2008; Rosa, Barron, Gaiani, Dufour, & Micard, Citation2013; Acosta-Estrada, Gutierrez-Uribe, & Serna-Saldivar, Citation2014). It was reported that antioxidant capacity and total phenolic contents were significantly higher in whole meal bread compared to white bread (Yu, Nanguet, & Beta, Citation2013). However, the release of phenolic compounds from the matrices of bran or increasing their accessibility has proven to be effective in improving bioavailability.
Bioprocessing can increase the bioavailability of nutrients and other compounds through chemical or enzymatic reactions that hydrolyze or release the nutrients from the food matrix (Mateo et al., 2011). The bioprocessing of wheat bran with enzymes and microbes has been performed to improve the technological properties of bran in wheat dough and bread with subsequent improvements in the end product volume, crumb texture, and shelf life (Nordlund, Katina, Aura, & Poutanen, Citation2013). Bran bioprocessing with enzymes and yeast also increases the content of bioactive compounds in bread with subsequent beneficial physiological effects; after bioprocessing with enzyme addition, the concentration of total phenols increased 20% (Coda, Rizzello, Curiel, Poutanen, & Katina, Citation2014).
Mateo-Anson, Selinheimo, et al. (Citation2009) found that the bioprocessing of wheat bran increased the content of free phenolic acids in breads compared to unprocessed control breads. The combination of fermentation and the enzymatic treatment of bran increased the amount of free FA in the bread eight-fold from 12 to 100 μg/g of dry matter. Rosa et al. (Citation2013) found that a synergistic combination of xylanase and feruloyl esterase is the most efficient enzymatic treatment, which releases up to 86% of total FA in bioaccessible forms.
Most Mexican consumers are very conservative and generally consume white bread made of 100% white flour; a smaller percentage of the population consumes bread with added bran or whole grain. The low utilization of whole grains occurs primarily because the population is unaware of the benefits to health that can result from the consumption of these products. Bioprocessing techniques, such as use of the xylanases and microbes as dough improvers, have resulted in the generation of new products with potential benefits to health for consumers. Therefore, the objectives of this study were as follows: (1) evaluate the effects of bioprocessing wheat bran on the content of total phenols, HCA, and antioxidant activity and (2) determine the bioaccessibility of total phenols and HCA in breads made from bioprocessed bran by an in vitro digestion model.
Materials and methods
Materials
Folin–Ciocalteu reagent, 2,2′-azino-bis-3-ethylbenzothiazoline-6-sulfonic acid (ABTS), 6-hydroxy-2,5,7,8 tetramethychroman-2-carboxylic acid (Trolox), and ferulic, ρ-coumaric and sinapic acids were purchased from Sigma-Aldrich Chemical Co., St. Louis, MO, U.S.A. Food-grade β-1,4-xylanase (Xyl) was obtained from Bioxylanase. ®Kerry and bakers’ yeast were purchased in a local market. All chemicals were analytical grade.
Sample preparation
Kronstad F2004 variety wheat (Triticum aestivum) was kindly donated by “Molino La Fama” S.A. de C.V. Hermosillo, Sonora, México. This is hard wheat that is commonly used for industrial bread production. Wheat grain was cleaned by passing it over a 7/64” sieve to separate broken kernels, branches, and stones; it was subsequently preconditioned to 14% moisture prior to the milling process.
Wheat grain was milled using an experimental roller mill (Model Brabender Senior Quadrumat, Germany, Duisburg) wherein white flour was separated from milling by-products to save bran. To obtain whole flour, a blade mill and corrugated shell (Model 200, Pulvex, S.A. de C.V., Mexico, D. F.) were used together with a sieve for particle sizes less than 0.5 mm. The particle size of bran was reduced to the same size as flour.
Bioprocessing of wheat bran
The bioprocessing of bran was conducted according to a procedure proposed by Mateo-Anson, Selinheimo, et al. (Citation2009) with some modifications. includes the amounts of enzyme, yeast and water added to each mixture for the bioprocessing of bran. The following three treatments were performed: (1) fermented bran (FB); (2) enzymatically treated bran (EB); and (3) fermented and enzymatically treated bran (FEB). The three treatments were placed in a proofing cabinet at 30°C with 75–80% relative humidity for 6 h. The bioprocessed brans were freeze-dried and stored at −20°C. Native bran (NB) was used as control.
Table 1. Preparation of mixtures for bran bioprocessing.a,b
Tabla 1. Preparación de las mezclas para el bioprocesamiento del salvado.a,b
Bread making procedure
The following breads were prepared: (1) white flour plus 30% native bran bread (NBB); (2) white flour plus 30% fermented bran bread (FBB); (3) white flour plus 30% enzymatic-treated bran bread (EBB); and (4) white flour plus 30% fermented and enzymatic-treated bran bread (FEBB). In order to compare with other breads traditionally consumed, additionally two breads were prepared: white flour bread (WFB); and whole-meal flour bread (WMB). The baking process was performed according to 10-10 (AACC, Citation1995)and farinographic measurements were obtained in a Brabender Farinograph/Resistograph using the constant flour weight procedure (54–21, AACC, 2000).
The breads were prepared by mixing all ingredients in a spiral mixer. The water absorption and dough development time were determined according to farinographic measurements. Subsequently, dough was fermented for 30 min at 30°C with 75–80% relative humidity. Next, a first dough degassing was performed using a dough sheeter roller (opening was 9/32 in.). The dough was fermented again for 30 min under the same conditions and was then divided in three parts before undergoing a second degassing with a 3/16-inch opening roller; then, it was molded. Molded dough pieces were fermented for 1 h and then baked for 10 min at 240°C. Loaf volume and weight were determined after 1 h of cooling. Specific volume was determined foreach experimental bread. Bread samples were freeze-dried for chemical analyses.
Free and bound phenolic compounds extraction
Free phenolic compounds from samples (bran and breads) were extracted. Briefly, one gram of each sample was mixed with methanol (80% v/v) and sonicated for 1 h, and samples were centrifuged at 100 × g for 15 min. The supernatants were filtered (Whatman No. 1 paper filter). The residues were used to repeat extraction twice. The supernatants were pooled and concentrated to dryness at 50°C on a rotatory evaporator and were then reconstituted to 5 ml with methanol (50% v/v) and stored at −20°C until analysis (Chiremba, Taylor, Rooney, & Beta, Citation2012).
Bound phenolic compounds were extracted according to the procedure of Guo & Beta (Citation2013). Briefly, the residues obtained from free phenolic compound extraction were dried at 45°C, and 100 mg of dry residue was weighed and mixed with 5 mL 2 M NaOH (degassed). Air was displaced with N2 for 30 s, and samples were sonicated for 3 h and further acidified to pH 1.5–2 with 6 M HCl. Then, liquid–liquid extractions were conducted using ethyl acetate (7 mL); this procedure was repeated three times, and the organic layer was recovered and evaporated under vacuum to dryness in a rotatory evaporator at 35°C. The residue in the bottom of the flask was reconstituted in 5 mL methanol (50% v/v) and kept at −20°C until analysis.
Total phenolic compounds (TPC) quantification
Free and bound phenolics were quantified using a method based on that of Singleton & Rossi (Citation1965), which was adapted for use with a FLUOstar OPTIMA multidetection microplate reader (BMG LABTECH, Ortenberg, Germany). Briefly, in each well, 10 μL of sample extracts were mixed with 150 μL of Folin-Ciocalteu reagent (2 N diluted 1:10 with deionized water), and 120 μL of sodium carbonate solution (7 g/L) was added to each well and mixed. After incubation in the dark for 1 h, absorbance readings were taken at 765 nm. Total phenolic content was expressed as μg GAE/g d.m. using a standard solution of gallic acid at different concentrations (0–240 μg/mL).
Trolox equivalent antioxidant capacity (TEAC)
The TEAC assay is based on the ability of antioxidant molecules to decolorize the ABTS radical cation. A stable stock solution of ABTS was prepared by reacting 5 mL of an aqueous solution of 7 mM ABTS with 0.088 mL of 148 mM K2S2O8. The mixture was allowed to stand in the dark at room temperature for 16–18 h. An ABTS working solution was prepared immediately before use by diluting the stock solution in ethanol (?1:88, v/v) to obtain an absorbance value at 734 nm of 0.7 ± 0.02. The assay for the scavenging capacity of antioxidants for the non-biological radical cation ABTS+• was modified for use with a multidetection microplate reader. In the microplate wells, 280 µL of ABTS working solution was combined with 20 µL of sample (free or bound phenol extracts). The reduction of absorbance at 734 nm was monitored as the ABTS radical scavenging activity. The results were expressed as μmol TE/g d.m. using a Trolox solution for to create a calibration curve (0–120 μg/mL) (Re et al., Citation1999; Robles Sanchez et al., Citation2009).
Quantification of hydroxycinnamic acids
The phenolic acid content (free and bound extracts) was quantified using a UHPLC system (Agilent Technologies, 1260, Germany) equipped with a diode array detector (DAD). The separation was achieved with a Zorbax Eclipse Plus-C18 RRHD reversed phase column (1.8 µm particle size 2.1 × 50 mm); column temperature was set to 30°C. Binary gradient elution was employed with A (0.1% acetic acid/water) and B (0.1% acetic acid/methanol) at a flow rate of 0.7 mL/min. The solvent gradient was as follows: initial 91% A and 9% B; 0–11 min, 9% to 14% B; and 11–15 min, 15% B. Peak detection was performed at 280 nm, and quantitation was performed using commercial standards of ρ-coumaric, ferulic, and sinapic acids. The results were expressed as μg phenolic acid/g d.m. (Chiremba et al., Citation2012).
In vitro digestion
In order to evaluate the effect of bioprocessing wheat bran on bioaccessibility of FA in breads, we performed a gastrointestinal digestion in vitro assay, which consisted of a three-step procedure simulating the digestive processes in the mouth, stomach, and small intestine. The large intestinal tract was not taken into account, since in vivo food digestion and the absorption of compounds occur primarily in the small intestine (Dziki, Dziki, Baraniak, & Lin, Citation2009; Oomen et al., Citation2003).
The gastrointestinal digestion study was performed with the technique developed by Velderrain-Rodríguez et al. (Citation2016), with slight modifications. Briefly, three healthy volunteers selected from the laboratory staff chewed 1 g of each bread for 15 s. After this time, the chewed bread was deposited in tubes, and the volunteers rinsed their mouths twice with 5 ml of water for 60 s and combined these rinses with initial chewed samples. The combined samples were centrifuged at 100 × g for 15 min at 4°C, and supernatants were separated and freeze-dried.
For stomach digestion, the indigestible fraction from mouth digestion was diluted with 5 mL of KCl–HCl buffer (0.2 M pH 1.5), and the solution was then mixed with 667 µL of a solution of pepsin (300 mg/mL) in HCl/KCl buffer. The mixture was stirred for 1 h at 37°C, and the supernatant was separated and freeze-dried. Finally, the indigestible fraction from stomach digestion was used for intestinal digestion. This fraction was diluted with 9 mL phosphate buffer (pH 7.5), and the solution was then mixed with 1 mL pancreatin (17 mg/mL) in phosphate buffer (pH 7.5). For this stage, 80 mg of bile salts were added, and the mixture was stirred at 37°C for 6 h; the supernatant was separated and freeze-dried.
Calculations
The bioaccessibility (% B) of FA was calculated as the value in the intestinal digestion divided by the original value in the bread samples.
Statistical analysis
All data are reported as the means ± standard deviation for three replicates. The data were tested by ANOVA using statistical software JMP 5.0.1 (U.S.A, SAS institute, Inc.) followed by Tukey’s test; differences of p < 0.05 were considered significant.
Results and discussion
Phenolic compounds and antioxidant capacity in bioprocessed bran
Wheat cv. Kronstad is largely grown in the Northwest of Mexico; it is classified as hard wheat because this cultivar possesses physical and chemical characteristics that allow bread production with good sensory and nutritive qualities. However, little information exists regarding its bioactive compounds, such as phenolics and antioxidants, which are considered to be contributors to the reduction of chronic degenerative disease risk. There are also few studies of the biological properties of bran, which is the main by-product obtained from industrial milling this cultivar.
In this study, we first evaluated the effect of bran bioprocessing on total phenolic compounds (TPC) (). A higher proportion of bound phenols relative to free phenols was observed for all bioprocessed brans and NB (73–78%). The EB, FB, and FEB treatments increased the total quantity of bound phenolics by 32%, 42%, and 38%, respectively, compared to NB. Free phenolic compounds also increased notably for all bioprocessed brans with respect to NB (p < 0.05). Therefore, the total of phenols (free + bound) was significantly increased. The increase in free phenols using xylanase enzyme (EB treatment) was due the fragmentation of arabinoxylans, which were more soluble during methanolic extraction. The application of yeast (FB treatment) promoted a similar effect of depolymerizing arabinoxylans; it is well known that yeast and other microorganisms produce compounds with depolymerizing properties.
Table 2. Total phenolic compounds (TPC) and proportions of free and bound phenolics (%) in bioprocessed bran.
Tabla 2. Polifenoles totales (TPC) y proporciones (%) de extractos de fenoles libres y ligados en salvado bioprocesado.
With respect to the increase of bound phenols, it is relevant to mention that both enzyme and yeast bran treatment promoted food matrix degradation with the consequent release of compounds, such as proteins, amino acids, carbohydrates, and flavonoids, among other compounds, which may have undergone polymerization or conjugation reactions. Similar results have been shown by Messia et al. (Citation2016), who found a nearly two-fold increase of soluble arabinoxylans in bran after pre-fermentation with lactic acid bacteria compared with control bran. Coda, Rizzello, Curiel, Poutanen, & Katina, (Citation2014) reported an increase in protein content, peptides, and extractable arabynoxilans and a decrease in starch content in fermented or enzymatically treated bran. The production of these compounds was associated with an increase in total phenols (20%). This study clearly revealed that both native and bioprocessed bran both show higher total phenol content compared with white flour or whole flour.
A study of Liyana-Pathirana, Dexter, & Shahidi (Citation2006) reported that among different fractions of wheat milling of two varieties (Canadian Western Amber Durum, Triticum turgidum L. var durum; and hard red spring Canadian Western, Triticum aestivum L), bran showed the highest phenols content, and endosperm had the lowest content. These results were reflected in their respective ability to eliminate reactive oxygen species. Additionally, Abozed, El-Kalyoubi, Abdelrashid, & Salama (Citation2014) demonstrated that the phenolic content and antioxidant capacity was higher in bran compared with whole-wheat flour. Yu et al. (Citation2013) found that whole-wheat flour showed a significantly higher antioxidant capacity compared to white flour.
It is to relevant to note that phenolic compounds include a large group of compounds that differ in their chemical and structural characteristics, but in addition to phenol compounds, several molecules, such as proteins, lipids, and carbohydrates, can be detected as phenols by the Folin-Ciocalteu assay. Therefore, we must consider that the enzymatic or fermentation processing of bran may have increased the levels of phenolic or non-phenolic molecules and the potential interactions between them.
These results were consistent with antioxidant capacity evaluated in bioprocessed brans (); higher antioxidant values corresponded to the bound phenolic fraction but not to the free phenolic fraction. Although the free phenolic fraction also increased, it was not reflected in increased antioxidant capacity values. Adom & Liu (Citation2002) reported in wheat grain, that the highest proportion of phenolic acids was bound (75%), and phenols content of 7.99 ± 0.39 μmol GAE/g.
Table 3. Antioxidant capacity (TEAC) and proportions (%) of free and bound phenolic extracts in bioprocessed bran.
Tabla 3. Capacidad antioxidante (TEAC) y proporciones (%) de extractos de fenoles libres y ligados en salvado bioprocesado.
In our study, we analyzed specific phenolics that are primarily associated with antioxidant properties, such as HCA, which are predominant in wheat bran. shows the effects of bran bioprocessing on ρ-coumaric, ferulic, and sinapic acids. These HCA were measured both in methanolic (free phenolics) and alkaline (bound phenolics) extracts. A larger proportion of HCA was found in bound phenolics compared to free phenolics for both native and bioprocessed bran. These results confirm the data of other authors, who found a higher content of bound HCA in wheat bran (Hemery et al., Citation2010).
Table 4. Hydroxycinnamic acids content (free and bound phenolic extracts) in bioprocessed bran.
Tabla 4. Contenido de ácidos hidroxicinámicos (extractos de fenoles libres y ligados) en salvado bioprocesado.
Alkaline hydrolysis is used experimentally because it breaks ester bonds linking phenolic acids in the cell wall and is therefore an effective way to release phenolic compounds from non-starch polysaccharides (Acosta-Estrada et al., Citation2014). Ragaee et al. (Citation2012) performed a study on 21 varieties of hard and soft wheat obtained from different locations in Ontario, Canada and found that most of the phenolic compounds are present in a bound form (90%).
All of the bran treatments were effective in releasing ρ-coumaric and sinapic acids because a lower quantity of these acids was found in the bound form compared to NB. In the specific case of FA, the enzymatic treatment (EB) was followed by the combined treatment (FEB) as the most effective treatments for releasing this acid; the bound fraction was reduced by 25% and 16%, respectively. Adom & Liu (Citation2002) reported that FA was found predominantly in wheat grain and that it was found mainly in the bound form. Another study by Kim, Tsao, Yang, & Cui (Citation2006) showed that in hard red and soft white wheat bran, most of the phenolic acids are bound, and they mentioned that extracts after alkaline hydrolysis contained the highest antioxidant capacity; only FA was released in a significantly higher amount (1,359–1,934 μg/g).
A reduction in phenolic acid content in the bound phenolic fraction associated with an increase in the free phenolic fraction could indicate that bioprocessing with enzymes, fermentation, or the combination had an effect on the release of these acids. In our study, this result was more evident for FA (five-fold change relative to NB). However, the reduction in the content of bound HCA by EB, FB, and FEB was not proportional to the increase of free phenolics. Apparently, the reduction of bound HCA could be attributed to the possible interaction and/or conjugation with other compounds as well as the polymerization of these phenolics.
Physical and biological characteristics of breads with bioprocessed bran added
Wheat bran is often used as an ingredient for several bakery products primarily to improve fiber dietary content, but in the last few years, the effects of adding bran on the sensory, nutritive, and biological properties of bread have been studied. In particular, bran can increase the levels of bioactive compounds, such as phenolics. However, the addition of bran has negative effects on the rheological properties of both the dough and the final product. Therefore, several technological procedures have been developed for bran to ensure an increase of the bioactive compounds without affecting the sensory properties of the product.
As part of this study, we evaluated the effects of adding bioprocessed bran to white flour on the physical and biological characteristics of breads. shows the specific volumes of experimental breads including WFB, whole meal bread (WMB), bioprocessed bran breads (EBB, FBB, and FEBB), and native bran bread (NBB). The highest specific volume was for WFB (6 mL/g). The addition of bran to flours had a negative effect on the specific volume of breads compared with WFB. When bran was treated with enzyme, fermented, or the combination was applied, the specific volume was higher than that of NB bread. However, the EBB had a higher specific volume than that of EBB or FEBB. In a study by Coda, Kärki, Nordlund, Heiniö, Poutanen & Katina (Citation2014), breads supplemented with bioprocessed bran showed a 10–40% improvement in the specific volume depending on the particle size and the fermentation time compared to breads containing control bran.
Figure 1. Specific volume of breads.1 The bars represent the means±standard deviation (n = 3). Different letters on the bars indicate significant difference (p < 0.05). Abbreviations: WFB (white flour bread); WMB (whole meal bread); NBB (native bran bread); FBB (fermented bran bread); EBB (enzymatically treated bran bread); FEBB (fermented and enzymatically treated bran bread).
Figura 1. Volumen específico de los panes.1 Las barras representan las medias ± desviación estándar (n = 3). Diferentes letras en las barras indican diferencia significativa (p < 0.05). Abreviaturas: WFB (pan de harina blanca); WMB (pan integral); NBB (pan de salvado nativo); FBB (pan de salvado fermentado); EBB (pan de salvado tratado enzimáticamente); FEBB (pan de salvado fermentado y tratado enzimáticamente).
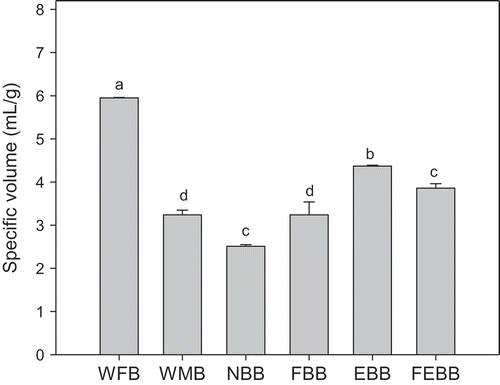
It was known that enzyme treatment with xylanase in flours destined for bread making has a positive influence on the rheological properties and final bread characteristics; the EB modifies the structures of the wall cell and promotes changes that impact water absorption and other farinograph parameters (Blandino et al., Citation2013). In our study, the addition of enzyme to the bran did not have a great influence on the absorption of water, but the time of development for the dough was greater compared to bread made with NB (data not shown). This result could be related to the higher volume obtained with EBB.
With respect to the biological characteristics of breads, we found a decrease of phenolic compounds (free and bound) compared to that found in their respective bran, these results were mainly due to the dilution effect when the bran (30%) was added to flours. Nevertheless, it was possible to observe that breads showed a similar effect to that found in bioprocessed bran. As a consequence it was observed an increase of phenolics for all breads supplemented with bioprocessed brans compared to bread with NB; both the free and bound phenolic fractions significantly increased compared to NBB (). The antioxidant capacity in bioprocessed bran breads showed consistent results with total phenolic contents; the antioxidant capacity was higher in bioprocessed bran breads compared to NBB. In addition, the major proportion of antioxidant capacity was found in the bound phenolic fraction ().
Table 5. Total phenolic compounds (TPC) and proportions (%) of free and bound phenolic extracts in different breads.
Tabla 5. Polifenoles totales (TPC) y proporciones (%) de fenoles libres y ligados en los diferentes panes.
Table 6. Antioxidant capacity (TEAC) and proportions (%) of free and bound phenolic extracts in breads.
Tabla 6. Capacidad antioxidante (TEAC) y proporciones (%) de extractos de fenoles libres y ligados en panes.
It was evident that both native and bioprocessed bran addition (30%) to flours improved the biological properties of breads compared with WFB and WMB. Similar results were shown by Blandino et al. (Citation2013), who used different wheat bran levels to supplement white flours and found that the nutritional value of the breads improved with the addition of 10% wheat bran. The bread making process may had contributed to these results, particularly because it is well known that during mixing, fermentation, and baking, several reactions can occur to enable the production of phenolic compounds (Dziki, Rozylo, Gawlik-Dziki, & Swieca, Citation2014; Han & Koh, Citation2011; Moore, Luther, Cheng, & Yu, Citation2009).
Bran bioprocessing with enzymes and yeast has also been shown to increase the content of bioactive compounds in bread with potentially positive physiological effects. After bioprocessing with enzyme addition, the concentration of total phenols increased 20% (Coda, Rizzello, Curiel, Poutanen, & Katina, Citation2014). The effect of adding bioprocessed bran to breads on HCA content was evaluated (). A decreased bound fraction was observed for all HCA for any bioprocessed bran breads compared to NBB, which was consistent with an increase of the free fraction with the exception of ρ-coumaric acid. This result could indicate that the addition of bioprocessed bran to white flour can contribute to the free phenolic acid content in bread. For all bioprocessed bran breads, the free FA content as increased to a greater extent compared with sinapic acid (24.8–28.4 μg/g vs. 15.1–16.7 μg/g); these values correspond to increases of 40–48% and 13–21%, respectively, compared to NBB.
Table 7. Hydroxycinnamic acids content (free and bound phenolic extracts) in breads.
Tabla 7. Contenido de ácidos hidroxicinámicos en extractos de fenoles libres y ligados) en panes.
Mateo et al. (2009) found that bioprocessing wheat bran increased the content of free phenolic acids in breads compared to bread controls. The combination of the fermentation and enzymatic treatment (enzyme mixture) of bran increased the amount of free FA in the bread eight-fold, from 12 to 100 μg/g of dry matter. Other studies have been performed with the bioprocessed bran of other cereals, such as rye (Nordlund et al., Citation2013) and barley (Ktenioudaki et al., Citation2015). The findings of these studies were similar to ours.
The previous results indicate that the addition of bioprocessed bran may improve the bioactive compound content in bread products; however, it is also important to determine whether these bioactive compounds that are available in the bioprocessed bran breads are truly bioaccessible and consequently bioavailable for utilization such that they can exert any beneficial effects on health, such as protecting against chronic degenerative diseases.
Ferulic acid bioaccessibility in bioprocessed bran breads
Bioaccessibility is a term that reflects the release of nutrients and other components, such as phenolic compounds, from the food matrix. Bioaccessibility can depend not only on the phenolic compounds availability in the food matrix but also on other parameters, such as food matrix complexity, conditions in the gastrointestinal tract, among others. These parameters may limit nutrient release for subsequent absorption (Hemery et al., Citation2010; Mateo et al., 2009; Saura-Calixto, Citation2011; Velderrain-Rodríguez, Citation2016). In this matter, it is very important to considered that digestion process acts as a biological extraction system in which the efficiency of extraction apparently depend of aforementionated.
In our study, we evaluated the behavior of free FA in both native and bioprocessed bran breads during an in vitro digestion model, and the results are shown in . Compared with the initial values of free FA (), the mouth digestion process promoted the release free FA from NBB (14.7 vs. 26.3 μg/g d.m + 44%), FBB (24.9 vs. 73.83 μg/g d.m. +66%), EBB (24.8 vs. 58.06 μg/g d.m. +58%), and FEBB (28.4 vs. 52.15 μg/g d.m. +45%). The free FA values were increased to a greater extent under gastric digestion conditions (stomach) for NBB (14.7 vs. 64.4 μg/g d.m. +77%), FBB (24.9 vs. 67.5 μg/g d.m. +63%), EBB (24.8 vs. 77.9 μg/g d.m. +68%), and FEBB (28.4 vs. 71.4 μg/g d.m. +60%).
Figure 2. In vitro digestion-mediated release of ferulic acid from breads.
Figura 2. Liberación de ácido ferúlico mediada por digestión in vitro de panes.
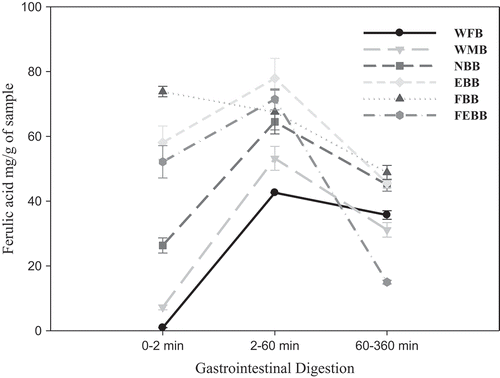
Finally, in intestinal digestion, the free FA values decreased with respect to mouth and gastric digestions but with higher levels than those initials of free ferulic for NBB (14.7 vs. 45.0 μg/g d.m. +67%), FBB (24.9 vs. 48.8 μg/g d.m. +45%), and EBB (24.8 vs. 45.5 μg/g d.m. +48%). The exception was the FEBB sample, which showed a reduction compared with initial values (28.4 vs. 15.0 μg/g d.m. −47%). The free FA levels in WFB and WMB samples showed similar behavior during the digestion process, the values were always less than that obtained with bioprocessed bran bread.
Several studies on wheat bran have been performed to improve the biological potential that is attributed to its phenolic acids; the results have been consistent with the behavior of these acids during in vitro digestion. Hemery et al. (Citation2010) studied the effects of dry-fractionated wheat bran added to white flours for bread elaboration on the FA content during in vitro digestion and reported a maximal amount of this acid during the gastric phase; they observed decreased levels when the samples were digested under intestinal conditions. Similar results were obtained by Mateo et al. (2009), who made breads with wheat bran (16%) treated with commercial enzymes including xylanases and ferulic esterase; they evaluated the effect of these treatments on in vitro digestion, and the results were similar to those obtained in our study.
During the bread in vitro digestion, we assume that the mouth digestion promoted a release of FA of food matrix higher to that of initial values due to break of food matrix by chewing effect which increase the surface of contact for salival enzyme (amylase) which catalyze the starch hydrolysis improving the solubilization of FA. The gastric digestion conditions played an important role in the release of FA independently of the technological procedure applied to wheat bran. The acidic conditions and enzymatic action presents during gastric digestion promoted the release of free ferulic additional to mouth digestion.
However, it is important to highlight that the release of FA during intestinal digestion although it was lower than that found in mouth and gastric digestion, is a pivotal event because absorption occurs in this phase. The above results can be understood better when the FA bioaccessibility percentage is calculated.
shows the FA (%) bioaccessibility for each bread sample. The WFB shows the highest FA bioaccessibility value (93%); the bioaccessibility values decreased notably in all bread containing bran. WMB and NBB samples showed the same bioaccessibility value (10%), whereas in bioprocessed bran breads, the bioaccessibility was higher for EBB samples (15%), followed by FBB (13%) and FEBB samples (4%).
Table 8. Amount of bioaccesible ferulic acid (μg/g bread intake) recovered after intestinal digestion of the different breads and % bioaccessibility of ferulic acid.
Tabla 8. Cantidad de ácido ferúlico bioaccesible (μg/g de pan consumido) recuperada después de la digestión intestinal de los diferentes panes y % de bioaccesibilidad de ácido ferúlico.
The FA in the WFB sample was mainly in the bound form, but when this sample was digested, practically all of this acid was released. For this reason, we obtained a high % B value. We assumed that the simplicity of the food matrix contributed to this result because this sample consists of starchy endosperm (~90%) that can be easily digested by gastrointestinal enzymes to release FA. In contrast, the bran added to white flour modified the food matrix composition and made it more complex and more difficult to degrade by digestive enzymes, which was reflected in a lower % B compared with WFB. Hemery et al. (Citation2010) and Mateo et al. (2009) found a 10.2 and 4.9% B, respectively, for WFBs. Compared with whole meal breads, they found a reduction of 3.5 – and 4.4-fold, respectively, whereas we found a 9.3-fold reduction in our study.
In our study, bran addition to flours resulted in bioaccessibility values ranging from 4% to 15% B; the higher values were for bread samples with enzymatically treated bran. Hemery et al. (Citation2010) reported FA bioaccessibility values in the range from 2.5% to 5.1% B for breads with bran added, and the higher values were for bread prepared with bran fractionated by electrostatic separation. Mateo et al. (2009) found FA bioaccessibility values for breads with bran added in the range of 1.1–5.5% B, and the higher values corresponded to bread prepared with bioprocessed bran (combined fermentation and enzymatic treatment). The use of bioprocessing techniques has been shown to also be a good approach for improving the bioaccessibility of health-promoting compounds in bran (Coda, Rizzello, Curiel, Poutanen, & Katina, Citation2014). Therefore, the incorporation of wheat bran into cereal-based products is an interesting and low-cost strategy to improve nutritional properties and physiological effects (Hemdane et al., Citation2015).
Conclusion
The addition of wheat milling by-products, such as bran, is a feasible method to increase the content of bioactive compounds in products, such as bread, while adding functional value due to the increased antioxidant phenolic acid capacity. Bran bioprocessing with EB improved the specific volume of breads and the FA content, which was reflected in increased bioaccessibility. Further studies could focus on human interventions to determine whether the intake of bread prepared with bioprocessed bran has positive effects on biomarkers of chronic diseases, such as obesity, diabetes, and cardiovascular disease.
Acknowledgments
María Fernanda Amaya Villalva received scholarship from CONACyT (National Research and Technology Council), Mexico.
Disclosure statement
No potential conflict of interest was reported by the authors.
Additional information
Funding
References
- Abozed, S. S., El-Kalyoubi, M., Abdelrashid, A., & Salama, M. F. (2014). Total phenolic contents and antioxidant activities of various solvent extracts from whole wheat and bran. Annals of Agricultural Science, 59, 63–67.
- Acosta-Estrada, B. A., Gutierrez-Uribe, J. A., & Serna-Saldivar, S. O. (2014). Bound phenolics in foods, a review. Food Chemistry, 152, 46–55.
- Adom, K. K., & Liu, R. H. (2002). Antioxidant activity of grains. Journal of Agricultural and Food Chemistry, 50, 6182–6187.
- American Association of Cereal Chemists (AACC). (1995). Approved methods of the american association of cereal chemists (9th ed.). Minneapolis, USA: American Association of Cereal Chemists.
- Andersson, A. A. M., Dimberg, L., Åman, P., & Landberg, R. (2014). Recent findings on certain bioactive components in whole grain wheat and rye. Journal of Cereal Science, 59, 294–311.
- Blandino, M., Sovrani, V., Marinaccio, F., Reyneri, A., Rolle, L., Giacosa, S., … Arlorio, M. (2013). Nutritional and technological quality of bread enriched with an intermediated pearled wheat fraction. Food Chemistry, 141, 2549–2557.
- Brewer, L. R., Kubola, J., Siriamornpun, S., Herald, T. J., & Shi, Y. C. (2014). Wheat bran particle size influence on phytochemical extractability and antioxidant properties. Food Chemistry, 152, 483–490.
- Chiremba, C., Taylor, J. R. N., Rooney, L. W., & Beta, T. (2012). Phenolic acid content of sorghum and maize cultivars varying in hardness. Food Chemistry, 134, 81–88.
- Coda, R., Giuseppe Rizzello, C., Curiel, J. A., Poutanen, K., & Katina, K. (2014). Effect of bioprocessing and particle size on the nutritional properties of wheat bran fractions. Innovative Food Science and Emerging Technologies, 25, 19–27.
- Coda, R., Kärki, I., Nordlund, E., Heiniö, R. L., Poutanen, K., & Katina, K. (2014). Influence of particle size on bioprocess induced changes on technological functionality of wheat bran. Food Microbiology, 37, 69–77.
- Dziki, D., Rozylo, R., Gawlik-Dziki, U., & Swieca, M. (2014). Current trends in the enhancement of antioxidant activity of wheat bread by the addition of plant materials rich in phenolic compounds. Trends in Food Science & Technology, 40, 48–61.
- Dziki, U., Dziki, D., Baraniak, B., & Lin, R. (2009). The effect of simulated digestion in vitro on bioactivity of wheat bread with Tartary buckwheat flavones addition. Food Science and Technology, 42, 137–143.
- Guo, W., & Beta, T. (2013). Phenolic acid composition and antioxidant potential of insoluble and soluble dietary fibre extracts derived from select whole-grain cereals. Food Research International, 51, 518–525.
- Han, H. M., & Koh, B. K. (2011). Antioxidant activity of hard wheat flour, dough and bread prepared using various processes with the addition of different phenolic acids. Journal of Science and Food Agricultural, 91, 604–608.
- Hemdane, S., Leys, S., Jacobs, P. J., Dornez, E., Delcour, J. A., & Courtin, C. M. (2015). Wheat milling by-products and their impact on bread making. Food Chemistry, 187, 280–289.
- Hemery, Y. M., Mateo Anson, N., Havenaar, R., Haenen, G. R. M. M., Noort, M. W. J., & Rouau, X. (2010). Dry-fractionation of wheat bran increases the bioaccessibility of phenolic acids in breads made from processed bran fractions. Food Research International, 43, 1429–1438.
- Itagaki, S., Kurokawa, T., Nakata, C., Saito, Y., Oikawa, S., Kobayashi, M., … Iseki, K. (2009). In vitro and in vivo antioxidant properties of ferulic acid: A comparative study with other natural oxidation inhibitors. Food Chemistry, 114, 466–471.
- Kim, K. H., Tsao, R., Yang, R., & Cui, S. W. (2006). Phenolic acid profiles and antioxidant activities of wheat bran extracts and the effect of hydrolysis conditions. Food Chemistry, 95, 466–473.
- Kristensen, M., Toubro, S., Jensen, M. G., Ross, A. B., Riboldi, G., Petronio, M., … Astrup, A. (2012). Whole grain compared with refined wheat decreases the percentage of body fat following a 12-week, energy-restricted dietary intervention in postmenopausal women. The Journal of Nutrition, 142, 710–716.
- Kroon, P. A., Faulds, C. B., Ryden, P., Robertson, J. A., & Williamson, G. (1997). Release of covalently bound ferulic acid from fiber in the human colon. Journal of Agricultural and Food Chemistry, 45, 661–667.
- Ktenioudaki, A., Alvarez-Jubete, L., Smythc, T. J., Kilcawley, K., Rai, D. K., & Gallagher, E. (2015). Application of bioprocessing techniques (sourdough fermentation and technological aids) for brewer’s spent grain breads. Food Research International, 73, 107–116.
- Liyana-Pathirana, C., Dexter, J., & Shahidi, F. (2006). Antioxidant properties of wheat as affected by pearling. Journal of Agricultural and Food Chemistry, 54, 17.
- Mateo-Anson, N., Aura, A. M., Selinheimo, E., Mattila, I., Poutanen, K., Van Den Berg, R., … Haenen, G. R. M. M. (2011). Bioprocessing of wheat bran in whole wheat bread increases the bioavailability of phenolic acids in men and exerts anti-inflammatory effects ex vivo.. Journal of Nutrition, 141, 137–143.
- Mateo-Anson, N., Selinheimo, E., Havenaar, R., Aura, A. M., Mattila, I., Lehtinen, P., … Haenen, G. R. M. M. (2009). Bioprocessing of wheat bran improves in vitro bioaccessibility and colonic metabolism of phenolic compounds. Journal of Agricultural and Food Chemistry, 57, 6148–6155.
- Mateo-Anson, N., Van Den Berg, R., Havenaar, R., Bast, A., & Haenen, G. R. M. M. (2008). Ferulic acid from aleurone determines the antioxidant potency of wheat grain (Triticum aestivum L.). Journal of Agricultural and Food Chemistry, 56, 5589–5594.
- McKeown, N. M., Jacques, P. F., Seal, C. J., Vries, J., Jonnalagadda, S. S., Clemens, R., … Marquart, L. F. (2013). Whole grains and health: From theory to practice—highlights of the grains for health foundation’s whole grains summit. Journal of Nutrition, 143, 744S–758S.
- Messia, M. C., Reale, A., Maiuro, L., Candigliota, T., Sorrentino, E., & Marconi, E. (2016). Effects of pre-fermented wheat bran on dough and bread Characteristics. Journal of Cereal Science, 69, 138–144.
- Moore, J., Luther, M., Cheng, Z., & Yu, L. (2009). Effects of baking conditions, dough fermentation, and bran particle size on antioxidant properties of whole-wheat pizza crusts. Journal of Agricultural and Food Chemistry, 57, 832–839.
- Nordlund, E., Katina, K., Aura, A. M., & Poutanen, K. (2013). Changes in bran structure by bioprocessing with enzymes and yeast modifies the in vitro digestibility and fermentability of bran protein and dietary fibre complex. Journal of Cereal Science, 58, 200–208.
- Oomen, A. G., Rompelberg, C. J. M., Bruil, M. A., Dobbe, C. J. G., Pereboom, D. P. K. H., & Sips, A. J. A. M. (2003). Development of an in vitro digestion model for estimating the bioaccessibility of soil contaminants. Archives of Environmental Contamination and Toxicology, 44, 281–287.
- Pekkinen, J., Rosa, N. N., Savolainen, O. I., Rahkonen, P. K., Mykkänen, H., Poutanen, K., … Hanhineva, K. (2014). Disintegration of wheat aleurone structure has an impact on the bioavailability of phenolic compounds and other phytochemicals as evidenced by altered urinary metabolite profile of diet-induced obese mice. Nutrition & Metabolism, 11, 1.
- Ragaee, S., Guzar, I., Abdel-Aal, E.-S., & Seetharaman, M. (2012). Bioactive components and antioxidant capacity of Ontario hard and soft wheat varieties. Canada Journal of Plant Science, 92, 19–30.
- Re, R., Pellegrini, N., Proteggentee, A., Pannala, A., Yang, M., & Rice-Evans, C. (1999). Antioxidant activity applying an improved ABTS radical cation de colorization assay. Free Radical Biology and Medicine, 26, (9–10), 1231–1237.
- Robles Sánchez, R. M., Islas Osuna, M. A., Astiazarán García, H. F., Vázquez Ortiz, F. A., Martín Belloso, O., Gorinstein, S., & González Aguilar, G. A. (2009). Quality index, consumer acceptability, bioactive compounds, and antioxidant activity of fresh-cut “Ataulfo” mangoes (Mangifera indica L.) as affected by low-temperature storage. Journal of Food Science, 74, 126–134.
- Rosa, N. N., Barron, C., Gaiani, C., Dufour, C., & Micard, V. (2013). Ultra-fine grinding increases the antioxidant capacity of wheat bran. Journal of Cereal Science, 57, 84–89.
- Rosa, N. N., Pekkinen, J., Zavala, K., Fouret, G., Korkmaz, A., Feillet-Coudray, C., … Micard, V. (2014). Impact of wheat aleurone structure on metabolic disorders caused by a high-fat diet in mice. Journal of Agricultural and Food Chemistry, 62, 10101–10109.
- Saura-Calixto, F. (2011). Dietary fiber as a carrier of dietary antioxidants: An essential physiological function. Journal of Agricultural and Food Chemistry, 59, 43–49.
- Singleton, V., & Rossi, J. A. (1965). Colorimetry of total phenolics with phosphomolybdic-phosphotungstic acid reagents. American Journal of Enology and Viticulture, 16(3), 144.
- Slavin, J. (2004). Whole grains and human health. Nutrition Research Reviews, 17, 99–110.
- Velderrain-Rodríguez, G., Quirós-Sauceda, A., Mercado-Mercado, G., Ayala-Zavala, J. F., Astiazarán-García, H., Robles-Sánchez, R. M., … González-Aguilar, G. A. (2016). Effect of dietary fiber on the bioaccessibility of phenolic compounds of mango, papaya and pineapple fruits by an in vitro digestion model. Food Science and Technology, 36, 188–194.
- Vitaglione, P., Napolitano, A., & Fogliano, V. (2008). Cereal dietary fibre: A natural functional ingredient to deliver phenolic compounds into the gut. Trends in Food Science & Technology, 19, 451–463.
- Ye, E. Q., Chacko, S. A., Chou, E. L., Kugizaki, M., & Liu, S. (2012). Greater whole-grain intake is associated with lower risk of type 2 diabetes, cardiovascular disease, and weight gain. The Journal of Nutrition, 142, 1304–1313.
- Yu, L., Nanguet, A. L., & Beta, T. (2013). Comparison of antioxidant properties of refined and whole wheat flour and bread. Antioxidants, 2, 370–383.