ABSTRACT
The fruit of 18 Solanum lycopersicum var. cerasiforme accessions from Mexico were evaluated for total phenolics (TP) by the Folin-Ciocalteau assay, phenolic profiles by high performance liquid chromatography-diode array detection-mass spectrometry (HPLC-DAD-MS), antioxidant activity (AoxA) by 2,2´-azino-bis(3-ethylbenzothiazolin)-6-sulfonic acid (ABTS), 2,2-diphenil-1-pycrilhydrazyl (DPPH), and oxygen radical absorbance capacity (ORAC), and antimutagenic activity (AmuA) by the Ames assay. TP was measured as Gallic Acid Equivalents (GAE) and the AoxA as Trolox Equivalents (TE). TP varied from 37 to 86 mg GAE 100 g−1 fresh weight (fw). The AoxA by ABTS (568-1187 µmol TE 100 g−1 fw) and DPPH (157-350 µmol TE 100 g−1 fw) correlated with TP and the levels of caffeoylquinic acids and rutin. The AmuA did not correlate with the levels of phenolics. Some accessions had higher AoxA and AmuA than those reported for commercial cultivars and also showed high levels of caffeoylquinic acids and rutin; thus, their consumption could have good health promoting effects.
RESUMEN
En frutos de 18 accesiones de Solanum lycopersicum var. cerasiforme de México se evaluó contenido de fenólicos totales (FT) por el método de Folin-Ciocalteau, perfil de fenólicos por HPLC-DAD-MS, actividades antioxidante (AAox) por ABTS, DPPH y ORAC, y antimutagénica (AAmu) por el ensayo de Ames. Los FT se midieron como Equivalentes de Ácido Gálico (EAG) y la AAox como Equivalentes de Trolox (ET). Los FT variaron de 37 a 86 mg EAG 100 g−1 peso fresco (pf). Las AAox por ABTS (568-1187 µmol ET 100 g−1 pf) y DPPH (157-350 µmol ET 100 g−1 pf) correlacionaron con el contenido de FT, ácidos cafeoilquínicos y rutina. La AAmu no correlacionó con los niveles de fenólicos. Algunas accesiones presentaron AAox y AAmu mayores que las reportadas para cultivares comerciales y mostraron altos niveles de ácidos cafeoilquínicos y rutina, por lo que su consumo podría tener buenos efectos de promoción de la salud.
PALABRAS CLAVE:
Introduction
Tomato is one of the most widely consumed vegetables and an important source of bioactive compounds (e.g. vitamins A and C, carotenoids, and phenolics) that exhibit a broad range of health benefits. However, the development of the commercial tomato cultivars has reduced the levels of bioactive compounds compared to those found in traditional varieties and wild relatives (Adalid, Rosello, & Nuez, Citation2010; Boches, Peterschmidt, & Myers, Citation2011; Hanson et al., Citation2004). Genetic engineering has been successfully used to improve nutraceutical characteristics of tomato cultivars (Li et al., Citation2015; Luo et al., Citation2008), but the use of this technology is limited by the safety concerns of consumers about genetically modified organisms. Therefore, wild tomato relatives represent an important alternative source of compounds with nutritional and biological activities.
Mexico is a source of wild tomatoes, including the cherry tomato Solanum lycopersicum (S.l.) var. cerasiforme, which is derived from Solanum pimpinellifolium (Blanca et al., Citation2015) and adapts to a wide range of climatic and geographic conditions. However, few studies have documented the chemical composition and antioxidant potential of S.l. var. cerasiforme tomato from Mexico, with sampling limited to a small number of populations or accessions available in collections of international institutions (Adalid et al., Citation2010; Boches et al., Citation2011; Hanson et al., Citation2004). Specifically, there is no information about some nutraceutical properties such as antimutagenic activity of S.l. var. cerasiforme fruit. Wild and semidomesticated populations of S.l. var. cerasiforme collected from the states of Oaxaca, Guerrero, and Puebla showed significant variations in fruit quality traits such as lycopene and vitamin C content, and color parameters (Carrillo-Rodríguez & Chávez-Servia, Citation2010; Crisanto-Juárez, Vera-Guzmán, Chávez-Servia, & Carrillo-Rodríguez, Citation2010; Juárez-López et al., Citation2009). Thus, further knowledge about the chemical composition and biological activities of S.l. var. cerasiforme is required to promote its cultivation and consumption as well as its use for breeding functional tomatoes. The aim of the present study was to determine the levels of phenolic compounds and their possible relationship with the antioxidant and antimutagenic activities of S.l. var. cerasiforme accessions from Mexico.
Materials and methods
Plant material
Eighteen accessions of Solanum lycopersicum (S.l.) var. cerasiforme from eight states of Mexico were provided by the germplasm banks of University of Guadalajara and Autonomous University of Sinaloa (). Seeds were germinated and the seedlings were grown in vertisol soil (pelluster) under irrigation conditions at the Faculty of Agronomy Field Station of the Autonomous University of Sinaloa, Culiacan Sinaloa, Mexico, during the winter-spring season of 2010–2011. Seedlings were planted in the field with a spacing of 1 m within a row and 1.6 m between rows. Water was applied every 7–14 days as needed from transplant until harvest. The total amounts of N, P, K, Ca, and Mg supplied were 129.9, 42.8, 132.3, 56.1, and 42.1 kg ha−1, respectively. The insecticide abamectin (AGRIMEC® 1.8% CE, Syngenta) was applied twice at 0.5 L ha−1 for whitefly control. The minimum and maximum temperatures averaged 12.5 ± 3.1 and 32.5 ± 2.6°C, respectively. A completely randomized design with three replications of five plants per accession was used. A total of 10 fruits per plant were harvested at the red-ripe stage (~9 days after breaker) as defined by Yamaguchi (Citation1983). Yellow-fruited accessions were harvested based on fruit size and maximum color intensity. After washing, the whole fruits from each replicate were homogenized with a T18 basic ULTRA-TURRAX® (IKA, Staufen, Germany), lyophilized and stored at −70°C until use.
Table 1. Total phenolics content, antioxidant and antimutagenic activities of the methanol extracts from the fruit of Solanum lycopersicum var. cerasiforme accessionsa.
Tabla 1. Contenido de fenólicos totales, actividades antioxidante y antimutagénica de extractos metanólicos del fruto de accesiones de Solanum lycopersicum var. cerasiformea.
Preparation of the methanol extracts
Methanol extracts were prepared according to Gómez-Romero, Segura-Carretero, and Fernández-Gutiérrez (Citation2010) with some modifications. Lyophilized fruit tissue was ground to a fine powder and 1 g was mixed with 10 mL of methanol, sonicated for 15 min, centrifuged (28,620 × g/15 min/20°C) and the supernatant was recovered. The extraction procedure was repeated two times with the residue. Supernatants were mixed and 3 mL were used for analyses of phenolic compounds and antioxidant activity, the rest was concentrated under vacuum (40°C) with a rotary evaporator (BÜCHI R-124, Brinkmann Instruments, USA) and stored at −20°C until use.
Antioxidant activity (AoxA)
The AoxA of the methanol extracts was evaluated by three methods: 2,2´-azino-bis(3-ethylbenzothiazolin)-6-sulfonic acid (ABTS) (Re et al., Citation1999), 2,2-diphenil-1-pycrilhydrazyl (DPPH) (Brand-Williams, Cuvelier, & Berset, Citation1995), and oxygen radical absorbance capacity (ORAC) (Huang, Ou, Hampsch-Woodill, Flanagan, & Prior, Citation2002). A Trolox standard curve was used to calculate the AoxA as micromoles of Trolox Equivalents per 100 g of fresh weight (µmol TE 100 g−1 fw). For ABTS, 0.05 mL of the methanol extract, or methanol as blank, were mixed with 1.95 mL of the ABTS•+ radical solution (5 mL of 7 mmol L−1 ABTS and 0.088 mL of 140 mmol L−1 sodium persulfate), incubated for 10 min at 37°C and then the absorbance was measured at 734 nm. For DPPH, 0.2 mL of the methanol extract, or methanol as blank, were mixed with 1.8 mL of 0.15 mmol L−1 DPPH in methanol, incubated for 20 min at room temperature in darkness, and the absorbance was measured at 515 nm. The ORAC procedure was performed using 96-well microplates. Each reaction mix contained 0.025 mL of methanol extract, 0.2 mL of fluorescein (0.96 µmol L−1) and 0.075 mL of 2,2ʹ-azobis (2-amidinopropane) dihydrochloride (AAPH, 95.8 mmol L−1). The methanol extract was replaced by 0.025 mL of 75 mmol L−1 phosphate buffer (pH 7.4) in the blank. The fluorescence (485 nm (ex)/525 nm (em)) was measured for 75 min (37°C) with 1 min intervals using a Synergy 2 multimode microplate reader (BioTek Instruments, USA).
Antimutagenic activity (AmuA)
AmuA was evaluated by the Ames microsuspension assay (Kado, Langley, & Eisenstadt, Citation1983) using Salmonella enterica serovar Typhimurium YG1024 (tester strain) and 1-nitropyrene (1-NP) at 40 ng/tube (mutagen). Toxicity and mutagenicity were evaluated for the methanol extracts at 1000 μg/plate. Tester strain was grown overnight in Oxoid Nutrient Broth No. 2 (Oxoid Ltd, Hants, UK) to approximately 1–2 × 109 cells/mL; cells were harvested by centrifugation (3000 × g, 4°C, 10 min) and resuspended (1 × 1010 cells mL−1) in ice-cold PBS (0.15 mol L−1, pH 7.4). The ingredients were added in the following order to 10 × 100 mm sterile glass culture tubes kept on ice: 0.1 mL cocktail, 0.1 mL of bacteria (1 × 1010 cells mL−1 PBS), 0.01 mL of the sample extract (100 μg/tube), and 0.005 mL of 1-NP (40 ng/tube). The mixture was incubated for 90 min at 37°C with shaking (150 rpm) and then 2 mL of molten top agar containing 90 nmol of histidine and biotin were added. The vortexed mixture was streaked onto minimal glucose, incubated for 48 h at 37°C, and the colonies were counted. Strain markers and bacterial survival were routinely monitored for each experiment. The mutagen and all samples were dissolved in dimethyl sulfoxide and sterilized by filtration (0.22 μm). AmuA was calculated as the percent inhibition (PI) of mutagenicity as follows: PI = (1 − A/B)*100, where A = number of revertants per plate in the presence of the methanol extract and B = number of revertants per plate in the absence of the extract. Antimutagenicity was classified according to the PI values: 0–20% negative, 20–40% weak, 40–60% positive, 60–90% strong, >90% suspected toxicity (Wall, Wani, Hughes, & Taylor, Citation1988).
Total phenolics (TP)
TP content was determined by the Folin-Ciocalteu reaction (Waterhouse, Citation2002). Methanol extract (20 µL) was mixed with 1.58 mL of distilled water and 0.1 mL of the Folin-Ciocalteu reagent, gently stirred for 5 min and added with 0.3 mL of a sodium carbonate saturated solution. The mixture was incubated in darkness for 30 min at 40°C and the absorbance was measured at 765 nm. A calibration curve of Gallic acid was prepared and the results were calculated as milligrams of Gallic acid equivalents (GAE) per 100 g on a fresh weight basis (mg GAE 100 g−1 fw).
Phenolic profiles by high performance liquid chromatography (HPLC)-diode array detection (DAD) and mass spectrometry (MS) analysis
HPLC analysis was according to Moco et al. (Citation2006) with some modifications. The methanol extract was passed through a syringe filter (polyvinylidene fluoride membrane, 0.45 µm, HPLC certified, Thermo Scientific, Germany) and 0.005 mL were injected into a HPLC-DAD system (ACCELA, Thermo Scientific, USA). The separation was carried out in a Fortis C18 HPLC column (3 µm, 50 × 2.1 mm) (Fortis Technologies Ltd, UK) using a linear gradient of 1% (v/v) formic acid (A) and acetonitrile (B), 0.5 to 60% of B in 40 min at a flow rate of 0.2 mL min−1. The detection was done at 280, 320, and 350 nm. Protocatechuic acid and daidzein were used as internal standards. The identification of phenolics was based on the UV-spectra, MS fragmentation, and by comparisons with MS data generated with commercial standards and reported in the literature (Gómez-Romero et al., Citation2010; Moco et al., Citation2006). Calibration curves of rutin, chlorogenic, and caffeic acid (Sigma Chemical Co., St. Louis MO, USA) were used for quantification. Flavonoids were reported as Rutin Equivalents (mg RE 100 g−1 fw) and phenolics as Caffeic Acid Equivalents (mg CAE 100 g−1 fw) or Chlorogenic Acid Equivalents (mg CGAE 100 g−1 fw). The HPLC-DAD was coupled to a mass spectrometer with an electrospray ionization source (LTQ XL, Thermo Scientific, USA). The analysis was carried out in negative mode and full scan spectra were obtained in the m/z range of 50–1500. The ions used for MSn experiments were fragmented by collision induced dissociation applying 10–45 V. The parameters of the capillary tube were 35 V and 300°C. Nitrogen and helium gases were used for drying and collision, respectively. The results were analyzed with the Xcalibur 2.2 software (Thermo Scientific, USA).
Statistical analysis
Data were analyzed by one and two way analysis of variance and the means were compared by the Fisher test (α = 0.05) by using the software STATGRAPHIC plus version 5.1 (Statistical Graphics Corporation™, USA). Correlation analysis was performed with PASW Statistics version 18 (SSPS Inc., Chicago, IL). The results are the mean value of three replicates.
Results
Antioxidant and antimutagenic activities
The AoxA of the methanol extracts showed a great variability with significant differences among the 18 accessions (). ABTS values varied from 568.4 µmol TE 100 g−1 in Placharosa (Sinaloa) to 1187.3 µmol TE 100 g−1 in Tumbisca (Michoacan). The AoxA by DPPH varied from 157.2 µmol TE 100 g−1 in F. Ruiz (Chiapas) to 349.9 µmol TE 100 g−1 in Tumbisca. The ORAC values of the accessions showed almost a fivefold variation and the highest activity was observed in Villamaza (Sinaloa). The average fruit weight of the accessions varied from 2.42 to 2.64 g () and it was not expected to affect significantly the activities and components evaluated.
The methanol extracts from the 18 accessions were neither toxic nor mutagenic on the tester strain up to 1000 µg/plate (data not shown). The AmuA of these extracts was assayed at a single concentration (100 µg/plate) () and the values showed a three-fold variation (19.7–61.1%). Rincon (Oaxaca) showed a strong inhibition of the mutagenic activity (61.1%), whereas most of the other accessions showed positive antimutagenicity (40–60%).
Phenolics content and its correlation with the antioxidant and antimutagenic activities
Total phenolics (TP) of the methanol extracts varied from 37.1 to 85.6 mg GAE 100 g−1 () and showed positive correlations with the AoxA determined by ABTS (r = 0.80, p < 0.001) and DPPH (r = 0.64, p < 0.01) but not with the AoxA by ORAC and the AmuA.
The methanol extracts from the 18 accessions showed similar phenolic profiles with seven peaks () but variable abundance; six of these peaks were identified as caffeoyl hexose I, chlorogenic acid (caffeoylquinic acid), rutin-pentoside (quercetin-3-O-pentosyl rutinoside), rutin (quercetin-3-O-rutinoside), and di- and tri-caffeoylquinic acids (). The most abundant phenolics (mg 100 g−1 fw) were chlorogenic acid (2.58–23.21) and rutin (3.97–21.38) (). Rincon showed the highest levels of most phenolics, followed by Tumbisca. When the levels of the six compounds identified were contrasted with the AoxA of the accessions, ABTS values correlated with chlorogenic acid (r = 0.67, p < 0.01), rutin-pentoside (r = 0.63, p < 0.01), rutin (r = 0.73, p < 0.01), and dicaffeoylquinic acid (r = 0.64, p < 0.01). DPPH values showed moderate correlations with rutin-pentoside (r = 0.48, p < 0.05), rutin (r = 0.46, p = 0.05), and dicaffeoylquinic acid (r = 0.47, p = 0.05), while ORAC values did not show any significant association. In the case of the AmuA, only the contents of rutin and dicaffeoylquinic acid showed a moderate but non-significant correlation (r = 0.37, p = 0.1) with the activity of methanol extracts evaluated at 100 µg/plate ().
Table 2. Main phenolic compounds identified by HPLC-DAD-MS in Solanum lycopersicum var. cerasiforme accessions.
Tabla 2. Principales compuestos fenólicos identificados por HPLC-DAD-MS en accesiones de Solanum lycopersicum var. cerasiforme.
Table 3. Phenolic HPLC profiles of methanol extracts from the fruit of Solanum lycopersicum var. cerasiforme accessionsa.
Tabla 3. Perfiles de fenólicos por HPLC de extractos metanólicos del fruto de accesiones de Solanum lycopersicum var. cerasiformea.
Figure 1. HPLC-DAD chromatogram of phenolics of the methanol extract from the fruit of Solanum lycopersicum var. cerasiforme accession Kilim. Similar profiles with quantitative variations were obtained for the other accessions. Peaks were identified as caffeoyl hexose I (1), chlorogenic acid (caffeoylquinic acid) (2), rutin pentoside (3), rutin (4), dicaffeoylquinic acid (5), tricaffeoylquinic acid (6), and nonidentified (7). Protocatechuic acid (P) and daidzein (D) were used as internal standards of phenolic acids and flavonoids, respectively.
Figura 1. Cromatograma HPLC-DAD de fenólicos del extracto metanólico del fruto de la accesión Kilim de Solanum lycopersicum var. cerasiforme. Todas las accesiones mostraron perfiles similares con variaciones cuantitativas. Los picos fueron identificados como cafeoil hexosa I (1), ácido clorogénico (ácido cafeoilquínico) (2), rutín pentósido (3), rutina (4), ácido dicafeoilquínico (5), ácido tricafeoilquínico (6), y no identificado (7). El ácido protocatecuico (P) y daidzeina (D) fueron usados como estándares internos de ácidos fenólicos y flavonoides, respectivamente.
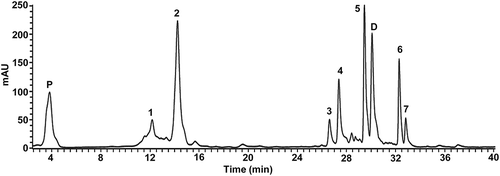
Antimutagenic activity: concentration-response effect
The antimutagenicity of methanol extracts from 10 selected accessions was evaluated in the range 0–1000 µg/plate (). The AmuA increased significantly with the extract concentration and showed the highest value in the accession Llanos. The AmuA varied from positive to strong (54.6–85.8%) at 250–1000 µg/plate and it was positive for most of the accessions at 100 µg/plate, whereas at 50 µg/plate this activity was negative or weak ().
Table 4. Concentration-response effect of methanol extracts from the fruit of Solanum lycopersicum var. cerasiforme accessions against the mutagenicity of 1-NP to Salmonella enterica serovar Typhimurium YG1024a.
Tabla 4. Efecto concentración-respuesta de extractos metanólicos del fruto de accesiones de Solanum lycopersicum var. cerasiforme en contra de la mutagenicidad de 1-NP hacia Salmonella enterica serovar Typhimurium YG1024a.
Discussion
Antioxidant activity
The AoxA values of the tomato accessions () were higher than those reported for commercial varieties. For instance, the ORAC values of the accessions were higher than those reported for some tomatoes (216–2026 µmol TE 100 g−1 fw) (Kevers et al., Citation2007; Ninfali, Mea, Giorgini, Rocchi, & Bacchiocca, Citation2005). The same was observed for the activities determined by ABTS and DPPH, where the values reported range from 274 to 562 µmol TE 100 g−1 fw and 84–180 µmol TE 100 g−1 fw, respectively (Kaur et al., Citation2013; Kevers et al., Citation2007). These results suggest that the tomato accessions analyzed in this study represent good sources of antioxidants. The present study showed positive correlation between TP and the AoxA (ABTS, DPPH) of the methanol extracts from the accessions as it has been reported previously for commercial varieties (Kevers et al., Citation2007; Ninfali et al., Citation2005). The lack of correlation between phenolics content and the AoxA by ORAC has been reported previously by other authors and has been attributed mainly to differences in the reaction mechanisms, assay conditions, and the presence of other compounds in the extract (Li et al., Citation2012). ORAC is a hydrogen atom transfer (HAT)-based assay, whereas ABTS and DPPH are commonly classified as mixed-mode methods (i.e. Electron transfer and HAT) (Apak, Özyürek, Güçlü, & Çapanoğlu, Citation2016).
Phenolic profiles
The main phenolics in the accessions were chlorogenic acid and its derivatives di- and tri-caffeoylquinic acids as well as the flavonoids rutin and rutin-pentoside (; ). These results agree with those obtained for the methanol extracts of three varieties of tomato (Gómez-Romero et al., Citation2010). Chlorogenic acid and rutin were the most abundant compounds in the methanol extracts () and their contents in some of the accessions were similar to those reported by Boches et al. (Citation2011) in five S.l. var. cerasiforme accessions selected from a core collection from Cornell University. As previously observed by these authors, high levels of di- and tri-caffeoylquinic acids (1–8 mg 100 g−1 fw) were also found in the present study, being higher than those reported for the cherry tomato cultivars Micro-Tom (0.19–0.28 mg g−1 dw) (Luo et al., Citation2008) and CLS (0.018–0.024 mg g−1 dw) (Li et al., Citation2015), considering that cherry cultivars contain 90–92% water. The association between the phenolics content and the antioxidant activity has been reinforced with data generated with transgenic tomato fruit; Micro-Tom fruit expressing the AtMYB12 transcription factor increased up to 35-fold the levels of di- and tri-caffeoylquinic acids (4.2–6.6 mg g−1 dw) (Luo et al., Citation2008), whereas the expression of AtMYB11 in the peel of the CLS cultivar increased up to 100-fold the levels of these compounds (0.25–2.39 mg g−1 dw) (Li et al., Citation2015). The higher levels of the phenolic compounds in both transgenic tomatoes were associated with three to five-fold increases in hydrophilic antioxidant activity (ABTS). The levels of di- and tri-caffeoylquinic acids in some of the accessions used in the present study () were close to those of the transgenic Micro-Tom fruit and similar to those of the transgenic CLS peels, suggesting these materials represent a nontransgenic alternative to improve this trait in tomato. Based on the positive correlation between ABTS values and phenolics content, chlorogenic acid, rutin, and their derivatives dicaffeoylquinic acid and rutin-pentoside could be the main compounds responsible for the antioxidant activity of the methanol extracts. In the case of flavonoids, their antioxidant activity has been associated with some structural characteristics, such as the ortho-dihydroxy (cathecol) in the B ring, 2,3 double bond in conjugation with a functional 4-oxo in the C ring, as well as hydroxyl groups at positions 3 and 5 that allow the formation of hydrogen bonds with the 4-oxo group. Based on these characteristics, the flavonoids with the best antioxidant activity should be quercetin and myricetin (Prochazkova, Bousova, & Wilhelmova, Citation2011). Rutin, the quercetin conjugate found at high concentrations in methanol extracts of the tomato accessions showed a good correlation with the antioxidant capacity by ABTS and DPPH.
Antimutagenic activity
To our knowledge, this is the first report showing the antimutagenic potential of S.l. var. cerasiforme. The methanol extracts (100 µg/plate) of some accessions showed higher inhibition values () than those reported by Valdez-Morales, Espinosa-Alonso, Espinoza-Torres, Delgado-Vargas, and Medina-Godoy (Citation2014) in the peel and seeds of some commercial tomatoes evaluated at 200 µg/plate. The AmuA of the 10 selected accessions () were higher than that obtained in a methanol extract from common beans (Phaseolus vulgaris) evaluated at 500 µg/plate (35%) using the same assay (González De Mejía, Castaño-Tostado, & Loarca-Piña, Citation1999).
The AmuA of tomato fruit has been attributed mainly to the presence of carotenoids (Rauscher, Edenharder, & Platt, Citation1998). However, the AmuA of pure lycopene was lower than that of a tomato extract (Polívková, Šmerák, Demová, & Houška, Citation2010), suggesting that other metabolites were contributing to this activity. In addition, the methanol extracts from accessions with yellow fruit exhibited high antimutagenic values ( and ) and they have very low levels of lycopene. Moreover, tomato fruit is also an important source of phenolic compounds that have AmuA (Agarwal & Rao, Citation2000; Middleton, Kandaswami, & Theoharides, Citation2000). Yamada and Tomita (Citation1996) evaluated the AmuA of caffeic acid and chlorogenic acid at 1000 µg/plate on the mutagens Trp-P-1, Glu-P-2, 4-NQO, and AF-2 using the strain TA98 of S. enterica serovar Typhimurium. The inhibition values of chlorogenic acid were 25%, 64%, 3%, and 15%, respectively; whereas those of caffeic acid were 33%, 73%, 5%, and 20%, respectively. As indicated before, the main phenolics found in the methanol extracts of the accessions studied herein were chlorogenic acid and derivatives, as well as rutin and its derivative rutin-pentoside. However, we only observed a moderate but non-significant correlation (r = 0.37, p = 0.1) between the levels of rutin and dicaffeoylquinic acid and the AmuA of the extracts evaluated at 100 µg/plate. The concentration-response effect for the antimutagenicity of methanol extracts showed the presence of active compounds; the lack of correlation between the antimutagenic activity and the content of specific phenolic compounds suggest that the activity was due either to nonidentified pure compounds or to the combined effect of different compounds.
Conclusions
Some of the Solanum lycopersicum var. cerasiforme accessions showed high content of phenolics, as well as high antioxidant and antimutagenic activities. The antioxidant activity of the accessions appears to be associated mainly with the levels of caffeoylquinic acids and rutin. Accessions with high levels of these compounds could be used as sources of antioxidants in the diet or for breeding functional tomatoes. Further studies are required to identify compounds associated with the strong antimutagenicity of some tomato accessions.
Acknowledgment
Authors thank Laura Contreras (CIAD, AC, Culiacán, Sinaloa) for her technical assistance.
Disclosure statement
No potential conflict of interest was reported by the authors.
Additional information
Funding
References
- Adalid, A. M., Rosello, S., & Nuez, F. (2010). Evaluation and selection of tomato accessions (Solanum section Lycopersicon) for content of lycopene, beta-carotene and ascorbic acid. Journal of Food Composition and Analysis, 23(6), 613–618.
- Agarwal, S., & Rao, A. V. (2000). Tomato lycopene and its role in human health and chronic diseases. Canadian Medical Association Journal, 163(6), 739–744.
- Apak, R., Özyürek, M., Güçlü, K., & Çapanoğlu, E. (2016). Antioxidant activity/capacity measurement. 2. Hydrogen atom transfer (HAT)-based, mixed-mode (Electron transfer (ET)/HAT), and lipid peroxidation assays. Journal of Agricultural and Food Chemistry, 64(5), 1028–1045.
- Barros, L., Duenas, M., Pinela, J., Carvalho, A. M., Buelga, C. S., & Ferreira, I. C. (2012). Characterization and quantification of phenolic compounds in four tomato (Lycopersicon esculentum L.) farmers’ varieties in northeastern Portugal homegardens. Plant Foods for Human Nutrition, 67(3), 229–234.
- Blanca, J., Montero-Pau, J., Sauvage, C., Bauchet, G., Illa, E., Diez, M. J., … Canizares, J. (2015). Genomic variation in tomato, from wild ancestors to contemporary breeding accessions. BMC Genomics, 16, 257.
- Boches, P., Peterschmidt, B., & Myers, J. R. (2011). Evaluation of a subset of the Solanum lycopersicum var. cerasiforme core collection for horticultural quality and fruit phenolic content. HortScience, 46(11), 1450–1455.
- Brand-Williams, W., Cuvelier, M. E., & Berset, C. (1995). Use of a free radical method to evaluate antioxidant activity. LWT - Food Science and Technology, 28(1), 25–30.
- Carrillo-Rodríguez, J., & Chávez-Servia, J. (2010). Agromorphological characterization of tomato samples from Oaxaca. Revista Fitotecnia Mexicana, 33(4), 1–6.
- Crisanto-Juárez, A. U., Vera-Guzmán, A. M., Chávez-Servia, J. L., & Carrillo-Rodríguez, J. C. (2010). Fruit quality of wild tomatoes (Lycopersicon esculentum var. Cerasiforme Dunal) from Oaxaca, México. Revista Fitotecnia Mexicana, 33, 7–13.
- Gómez-Romero, M., Segura-Carretero, A., & Fernández-Gutiérrez, A. (2010). Metabolite profiling and quantification of phenolic compounds in methanol extracts of tomato fruit. Phytochemistry, 71(16), 1848–1864.
- González De Mejía, E., Castaño-Tostado, E., & Loarca-Piña, G. (1999). Antimutagenic effects of natural phenolic compounds in beans. Mutation Research-Genetic Toxicology and Environmental Mutagenesis, 441(1), 1–9.
- Hanson, P. M., Yang, R. Y., Wu, J., Chen, J. T., Ledesma, D., Tsou, S. C. S., & Lee, T. C. (2004). Variation for antioxidant activity and antioxidants in tomato. Journal of the American Society for Horticultural Science, 129(5), 704–711.
- Huang, D., Ou, B., Hampsch-Woodill, M., Flanagan, J. A., & Prior, R. L. (2002). High-throughput assay of oxygen radical absorbance capacity (ORAC) using a multichannel liquid handling system coupled with a microplate fluorescence reader in 96-well format. Journal of Agricultural and Food Chemistry, 50(16), 4437–4444.
- Juárez-López, P., Castro-Brindis, R., Colinas-León, T., Ramírez-Vallejo, T., Sandoval-Villa, M., Reed, D. W., … King, S. (2009). Evaluation of quality in fruits of seven native tomato (Lycopersicum esculentum var cerasiforme) genotypes. Revista Chapingo Serie Horticultura, 15, 5–9.
- Kado, N. Y., Langley, D., & Eisenstadt, E. (1983). A simple modification of the Salmonella liquid-incubation assay. Increased sensitivity for detecting mutagens in human urine. Mutation Research, 121(1), 25–32.
- Kaur, C., Walia, S., Nagal, S., Walia, S., Singh, J., Singh, B. B., & Sarika. (2013). Functional quality and antioxidant composition of selected tomato (Solanum lycopersicum L) cultivars grown in Northern India. LWT - Food Science and Technology, 50(1), 139–145.
- Kevers, C., Falkowski, M., Tabart, J., Defraigne, J. O., Dommes, J., & Pincemail, J. (2007). Evolution of antioxidant capacity during storage of selected fruits and vegetables. Journal of Agricultural and Food Chemistry, 55(21), 8596–8603.
- Li, H. Y., Deng, Z. Y., Wu, T., Liu, R. H., Loewen, S., & Tsao, R. (2012). Microwave-assisted extraction of phenolics with maximal antioxidant activities in tomatoes. Food Chemistry, 130(4), 928–936.
- Li, Y., Chen, M., Wang, S., Ning, J., Ding, X., & Chu, Z. (2015). AtMYB11 regulates caffeoylquinic acid and flavonol synthesis in tomato and tobacco. Plant Cell, Tissue and Organ Culture, 122, 309–319.
- Luo, J., Butelli, E., Hill, L., Parr, A., Niggeweg, R., Bailey, P., … Martin, C. (2008). AtMYB12 regulates caffeoyl quinic acid and flavonol synthesis in tomato: Expression in fruit results in very high levels of both types of polyphenol. The Plant Journal, 56(2), 316–326.
- Middleton, E., Jr., Kandaswami, C., & Theoharides, T. C. (2000). The effects of plant flavonoids on mammalian cells: Implications for inflammation, heart disease, and cancer. Pharmacological Reviews, 52(4), 673–751.
- Moco, S., Bino, R. J., Vorst, O., Verhoeven, H. A., De Groot, J., Van Beek, T. A., … De Vos, C. H. R. (2006). A liquid chromatography-mass spectrometry-based metabolome database for tomato. Plant Physiology, 141(4), 1205–1218.
- Ninfali, P., Mea, G., Giorgini, S., Rocchi, M., & Bacchiocca, M. (2005). Antioxidant capacity of vegetables, spices and dressings relevant to nutrition. British Journal of Nutrition, 93(2), 257–266.
- Polívková, Z., Šmerák, P., Demová, H., & Houška, M. (2010). Antimutagenic effects of lycopene and tomato puree. Journal of Medicinal Food, 13(6), 1443–1450.
- Prochazkova, D., Bousova, I., & Wilhelmova, N. (2011). Antioxidant and prooxidant properties of flavonoids. Fitoterapia, 82(4), 513–523.
- Rauscher, R., Edenharder, R., & Platt, K. L. (1998). In vitro antimutagenic and in vivo anticlastogenic effects of carotenoids and solvent extracts from fruits and vegetables rich in carotenoids. Mutation Research-Genetic Toxicology and Environmental Mutagenesis, 413(2), 129–142.
- Re, R., Pellegrini, N., Proteggente, A., Pannala, A., Yang, M., & Rice-Evans, C. (1999). Antioxidant activity applaying an improved ABTS radical cation decolorization assay. Free Radical Biology and Medicine, 26, 1231–1237.
- Ribas-Agustí, A., Cáceres, R., Gratacós-Cubarsí, M., Sárraga, C., & Castellari, M. (2012). A validated HPLC-DAD method for routine determination of ten phenolic compounds in tomato fruits. Food Analytical Methods, 5(5), 1137–1144.
- Valdez-Morales, M., Espinosa-Alonso, L. G., Espinoza-Torres, L. C., Delgado-Vargas, F., & Medina-Godoy, S. (2014). Phenolic content and antioxidant and antimutagenic activities in tomato peel, seeds, and byproducts. Journal of Agricultural and Food Chemistry, 62(23), 5281–5289.
- Vallverdú-Queralt, A., Medina-Remón, A., Martínez-Huélamo, M., Jáuregui, O., Andres-Lacueva, C., & Lamuela-Raventos, R. M. (2011). Phenolic profile and hydrophilic antioxidant capacity as chemotaxonomic markers of tomato varieties. Journal of Agricultural and Food Chemistry, 59(8), 3994–4001.
- Wall, M. E., Wani, M. C., Hughes, T. J., & Taylor, H. (1988). Plant antimutagenic agents, 1. General bioassay and isolation procedures. Journal of Natural Products, 51(5), 866–873.
- Waterhouse, A. L. (2002). Determination of total phenolics. In R. E. Wrolstad (Ed.), Current protocols in food analytical chemistry (pp. I1.1.1–I1.1.8). New York, NY: Wiley.
- Yamada, J., & Tomita, Y. (1996). Antimutagenic activity of caffeic acid and related compounds. Bioscience, Biotechnology and Biochemistry, 60(2), 328–329.
- Yamaguchi, M. (1983). Solanaceous fruits: Tomato, eggplant, peppers, and others. In M. Yamaguchi (Ed.), World vegetables (pp. 291–311). Westport: AVI Publishing Company, Inc.