ABSTRACT
This study investigated the protective effects of astaxanthin on diabetic cardiomyopathy in rats. The rat diabetes mellitus model was treated with astaxanthin. Compared with the model group, in the 20 mg/kg astaxanthin group the LVSP (92.03 ± 11.96 mmHg), + dp/dtmax (6045.36 ± 52.74 mmHg/s) and -dp/dtmax (4956.33 ± 448.21 mmHg/s), myocardial superoxide dismutase (163.41 ± 17.42 U/mg) and glutathione peroxidase activity (148.39 ± 19.28 nmol/mg) were significantly increased (P < 0.05), and the LVEDP (1.66 ± 0.91 mmHg), blood TG (0.83 ± 0.28 mmol/L) and TC level (2.78 ± 1.45 mmol/L), serum TNF-α (1.02 ± 0.33 mol/L) and IL-6 (1.48 ± 0.26 mol/L) level and myocardial malondialdehyde level (15.78 ± 2.01 nmol/mg) were significantly decreased (P < 0.05), and ratios of p-JNK/JNK (0.92 ± 0.06), p-ERK1/2/ERK1/2 (0.22 ± 0.04) and p-p38 MAPK/p38 MAPK (0.28 ± 0.05) were significantly decreased (P < 0.05). In conclusion, astaxanthin can reduce the myocardial damage in diabetic cardiomyopathy rats, which may be related to its improving blood glucose and blood lipid metabolism, resisting oxidative stress and inflammatory reaction, and inhibiting activation of MAPK signaling pathway.
RESUMEN
El presente estudio investigó los efectos protectores de la astaxantina en ratas que padecen miocardiopatía diabética. Un grupo de ratas con diabetes mellitus fue tratado con astaxantina. Se constató que, en comparación con el grupo del modelo (ratas con diabetes mellitus sin tratar), en el grupo de ratas tratado con 20 mg/kg de astaxantina, la LVSP (92.03 ± 11.96 mmHg), + dp/dtmax (6045.36 ± 52.74 mmHg/s) y -dp/dtmax (4956.33 ± 448.21 mmHg/s), se elevaron significativamente (P < 0.05) el superóxido dismutasa miocárdico (163.41 ± 17.42 U/mg) y la actividad de glutatión peroxidasa (148.39 ± 19.28 nmol/mg). Al mismo tiempo cayeron de manera significativa (P < 0.05) los niveles de LVEDP (1.66 ± 0.91 mmHg), TG (0.83 ± 0.28 mmol/L) y TC sanguíneas (2.78 ± 1.45 mmol/L), el TNF-α en suero (1.02 ± 0.33 mol/L), el IL-6 (1.48 ± 0.26 mol/L) y el malondialdehido miocárdico. También se redujeron en forma significativa (P < 0.05) las ratios de p-JNK/JNK (0.92 ± 0.06), p-ERK1/2/ERK1/2 (0.22 ± 0.04) y p-p38 MAPK/p38 MAPK (0.28 ± 0.05). Estos resultados permiten concluir que la astaxantina puede reducir el daño miocárdico en ratas que padecen miocardiopatía diabética, lo cual puede responder a su capacidad para mejorar el metabolismo de la glucosa y los lípidos sanguíneos. Ello posibilitaría la resistencia al estrés oxidativo y la reacción inflamatoria, al tiempo que inhibe la activación de la vía de señalización MAPK.
1. Introduction
Diabetes mellitus (DM) is one of the endocrine and metabolic diseases. DM is caused by the metabolic disorder of glucose, protein, fat and other substances due to the absolute or relative lack of insulin secretion or declined sensitivity of target tissues and cells to insulin (American Diabetes Association, Citation2009). The chronic complications of DM have a very wide range, and they can almost involve all of the body tissues. Diabetic cardiomyopathy (DCM) is a heart disease caused by DM and is one of the most serious complications of DM. DCM can eventually develop into congestive heart failure, which severely threatens the patient’s life. DCM accounts for the majority of causes of death in DM patients and has become a hot and difficult issue in the medical field (Hayat, Patel, Khattar, & Malik, Citation2004). At present, there is no unified treatment scheme for DCM. The pathogenesis of DCM has not yet been fully defined. Studies have shown that many pathological changes such as glucose and lipid metabolism disorder (Brahma, Pepin, & Wende, Citation2017; Ritchie, Zerenturk, Prakoso, & Calkin, Citation2017), inflammatory reaction (Wen, Liang, Zhang, & Yang, Citation2013), microvascular lesion (Rossen, Citation1996), cell apoptosis (Sun et al., Citation2014) and so on are involved in the occurrence and development of DCM. More and more evidence confirms that the activation of the mitogen-activated protein kinase (MAPK) signaling pathway is closely related to the occurrence of DCM (Lakshmanan et al., Citation2012; Luo et al., Citation2014). Astaxanthin, namely 3,3ʹ-dihydroxy-beta,beta-carotene-4,4ʹ-dione, is one of the unsaturated terpenoids. It is separated from the shell of shrimps and crabs. A previous study (Higuera-Ciapara, Félix-Valenzuela, & Goycoolea, Citation2006) has shown that astaxanthin has potential antioxidant activity. It can enhance the immunity and prevent the occurrence and development of liver and kidney damage, cancer, cardiovascular lesions, and other diseases. It is found that astaxanthin can mitigate myocardial damage (Guo et al., Citation2015). However, the effects of astaxanthin on DCM have not been reported. This study investigated the protective effect of astaxanthin on DCM in rats. The objective was to provide a basis for clinical application of astaxanthin to prevention and treatment of DCM.
2. Materials and methods
2.1. Animals
Male Sprague-Dawley rats were single-cage raised in the conditions of avoiding strong light and noise (temperature 21 ± 2°C; relative humidity 30–70%; 12/12-h day-night cycle; free to feed and water) for 1 week. Then the rats were fed with a high-glucose and high-high fat diet (20% sucrose, 10% lard, 2.5% cholesterol, 1% bile salt and 66.5% basal diet). After 4 weeks, the rats were fasted for 12 h, followed by single intraperitoneal injection of streptozotocin with dose of 55 mg/kg (streptozotocin was dissolved in pH 4.5 and 0.1 mol/L citric acid buffer at 4°C). After 72 h, the fasting blood glucose (FBG) of rats was detected, once for each day, for successive 3 times. FBG > 7.8 mmol/L presented the successful establishment of DM model. The DM rats were randomly divided into model group and low-, medium-, and high-dose astaxanthin groups, 10 rats in each group. In addition, 10 normal rats were selected as the control.
2.2. Experimental design
After establishment of the DM model, the rats in low-, medium-, and high-dose astaxanthin groups were intraperitoneally injected with astaxanthin (dissolved in olive oil), with a dose of 5, 10 and 20 mg/kg, respectively. The rats in the control and model groups were intraperitoneally injected with an equal volume of olive oil. The administration was performed once per day, for 6 weeks. During the administration, the body weight, mind state, response, activity, and diet of the rats were observed.
2.3. Determination of blood glucose, blood lipid, and inflammatory factor levels
At the end of the experiment, the rats were fasted for 12 h. The blood was taken from the tail vein. The FBG, hemoglobin A1c (HbA1c), triglyceride (TG), and total cholesterol (TC) were determined using automatic biochemical analyzer (Chen, Li, & Wu, Citation2009). The serum tumor necrosis factor-α (TNF-α) and interleukin-6 (IL-6) levels were detected using radioimmunoassay (Stannus et al., Citation2010). The procedures were in accordance to the instructions of kits (Shanghai Sangon Biological Engineering Technology And Service Co., Ltd., Shanghai, China).
2.4. Determination of cardiac function
According to the reported method (Ge, Ma, Tao, & Guan, Citation2009) with some modification, after anesthetizing using 10% chloral hydrate, the rats were fixed in a supine position. The right carotid artery was separated. The ventricular catheter was inserted into the left ventricle through the right carotid artery and was connected to the biological signal recorder to determine the left ventricular systolic pressure (LVSP), left ventricular end diastolic pressure (LVEDP), and maximum left ventricular systolic/diastolic rate (± dp/dtmax).
2.5. Determination of left ventricular weight index
Rats were sacrificed by cervical dislocation, and the heart was quickly taken. The heart was rinsed using 4°C normal saline and was weighed after drying by neutral filter paper. The atria and right ventricle were cut off, and the left ventricle including interventricular septum was weighed. The left ventricular weight index (LVWI) was calculated as follows: LVWI = (left ventricular weight/whole body weight) × 100 (Guo, Shi, Yin, & Chen, Citation2007).
2.6. Determination of myocardial oxidative stress indexes
Left ventricular tissue was homogenized on the ice, followed by centrifuging at 4°C and 3,000 r/min for 15 min. The supernatant was obtained. The myocardial superoxide dismutase (SOD) activity was determined using the xanthine oxidase method (Harrison & Bray, Citation1997). The glutathione peroxidase (GSH-Px) activity was determined using the glutathione oxidation method (Martinez et al., Citation2015). The malondialdehyde (MDA) level was determined using thiobarbituric acid method (Memon et al., Citation2012).
2.7. Determination of protein expressions in myocardial MAPK signaling pathway
According to the previous study (Uzgare, Kaplan, & Greenberg, Citation2003), the expressions of protein in the MAPK signaling pathway in myocardial tissue were determined using western blot assay. The myocardial tissue was taken and homogenized. The protein was extracted using RIPA lysis buffer. The protein concentration was determined by BCA method. A total of 50 μg protein was used for the SDS-PAGE, then the separated protein was transferred to the PVDF membrane. After blocking using 5% BSA, the membranes were incubated with the primary antibody overnight at 4°C. After washing with TBST, the horseradish peroxidase-labeled second antibody was added, followed by incubation at 37°C for 1.5 h. After washing with TBST, the ECL luminous liquid was added, followed by developing and fixing. The intensity of bands was calculated with Image J 1.46 analysis software (European Molecular Biology Laboratory Inc., Oxford, UK). The primary and secondary antibodies for c-Jun N-terminal kinase (JNK), phosphorylated c-Jun N-terminal kinase (p-JNK), extracellular-signa1 regulated kinase 1/2 (ERK1/2), phosphorylated extracellular-signa1 regulated kinase 1/2 (p-ERK1/2), p38 mitogen-activated protein kinase (p38 MAPK), and phosphorylated p38 mitogen-activated protein kinase (p-p38 MAPK) were provided by Sigma-Aldrich Corp. (MO, USA). β-actin was used as the internal reference. The relative expression level of target protein was presented by the ratio of integral optical density of target protein to β-actin. The ratios of p-JNK/JNK, p-ERK1/2/ERK1/2, and p-p38 MAPK/p38 MAPK were calculated, respectively.
2.8. Statistical analysis
All statistical analysis was carried out using SPSS 22.0 software (SPSS Inc., Chicago, IL, USA). The data were presented as mean± SD. The difference between two groups was analyzed using one-way analysis of variance with SNK-q test. P < 0.05 was considered as statistically significant.
3. Results
3.1. General condition of rats
During the whole experiment period, the rats in the model group presented polyuria, polydipsia, and overeating. The hair gradually lost luster. The whole state was dispirited, and the action was slow. The decayed tail appeared in individual rats. The above phenomenon also occurred in the low-dose astaxanthin group, but the degree was slightly lighter than the model group. In the control and middle- and high-dose astaxanthin groups, there was no significant change in urine volume, water intake, or food intake of rats. The hair was glossy, and the eyes were flexible. The rats had sensitive responses to the surrounding changes, with relatively good state.
3.2. Effects of astaxanthin on body weight and LVWI of rats
At the end of 6 weeks, the body weight in the model group (118.34 ± 22.56 g) was significantly lower than in the control group (212.45 ± 48.12 g) (P < 0.05), and the LVWI in the model group (4.55 ± 0.48) was significantly higher than in the control group (2.54 ± 0.38) (P < 0.05). Compared with the model group, in high-dose astaxanthin groups the body weight (184.04 ± 44.64 g) was significantly increased (P < 0.05), and the LVWI (2.92 ± 0.61) was significantly decreased (P < 0.05) ().
Table 1. Efectos de la astaxantina en el peso corporal y en el índice de masa ventricular izquierda de ratas.
Tabla 1. Efectos de la astaxantina en el peso corporal y en el índice de masa ventricular izquierda de ratas.
3.3. Effects of astaxanthin on blood glucose and blood lipid level of rats
As shown in , the serum levels of FBG, HbA1c, TC and TG in the model group were 17.89 ± 2.59 mmol/L, (11.05 ± 1.25)%, 5.13 ± 1.03 mmol/L and 1.44 ± 0.41 mmol/L, which were significantly higher than 5.61 ± 1.96 mmol/L, (6.86 ± 1.14)%, 1.72 ± 0.34 mmol/L and 0.71 ± 0.24 mmol/L in the control group, respectively (P < 0.05). The FBG, HbA1c TC, and TG level in high-dose astaxanthin group were 7.34 ± 2.73 mmol/L, (6.48 ± 1.10)%, 2.78 ± 1.45 mmol/L and 0.83 ± 0.28 mmol/L, which were significantly reduced compared with the model group, respectively (P < 0.05).
Table 2. Effects of astaxanthin on blood glucose and blood lipid of rats.Tabla 2. Efectos de la astaxantina en la glucosa sanguínea y los lípidos sanguíneos de ratas.
3.4. Effects of astaxanthin on cardiac function of rats
LVSP and + dp/dtmax presented the cardiac contractility, and LVEDP and -dp/dtmax presented the cardiac diastolic function. The LVSP, + dp/dtmax, and -dp/dtmax in the model group were 56.33 ± 8.41 mmHg, 2433.51 ± 562.59 mmHg/s, and 1905.83 ± 567.34 mmHg/s, which were significantly lower than 110.78 ± 17.56 mmHg, 7689.04 ± 890.16 mmHg/s, and 5402.56 ± 789.21 mmHg/s in the control group, respectively (P < 0.05); the LVEDP in the model group was 4.33 ± 1.81 mmHg, which was significantly higher than 0.48 ± 0.11 mmHg in the control group (P < 0.05). Compared with the model group, in the high-dose astaxanthin group the LVSP, + dp/dtmax, and -dp/dtmax were 92.03 ± 11.96 mmHg, 6045.36 ± 52.74 mmHg/s, and 4956.33 ± 448.21 mmHg/s, which were significantly increased, respectively (P < 0.05), and the LVEDP was 1.66 ± 0.91 mmHg, which was significantly decreased (P < 0.05) ().
Table 3. Effects of astaxanthin on cardiac function of rats.Tabla 3. Efectos de la astaxantina en la función cardiaca de ratas.
3.5. Effects of astaxanthin on serum tnf-α and IL-6 levels of rats
As shown in , the serum TNF-α and IL-6 levels in the model group were 2.78 ± 0.445 and 3.33 ± 0.45 mol/L, which were significantly higher than 0.78 ± 0.21 and 1.12 ± 0.11 mol/L in the control group, respectively (P < 0.05). The TNF-α and IL-6 levels in the high-dose astaxanthin group were 1.02 ± 0.33 and 1.48 ± 0.26 mol/L, which were significantly lower than the model group, respectively (P < 0.05).
Table 4. Effects of astaxanthin on serum tumor necrosis factor-α and interleukin-6 levels of rats.Tabla 4. Efectos de la astaxantina en la necrosis tumoral en suero factor-α y en el nivel de interleucina-6 en ratas.
3.6. Effects of astaxanthin on myocardial SOD and gsh-px activity and MDA level in rats
As shown in , the myocardial SOD and GSH-Px activity in the model group were 129.72 ± 14.26 U/mg and 103.78 ± 21.83 nmol/mg, which were significantly lower than 172.43 ± 20.11 U/mg and 154.67 ± 29.82 nmol/mg in the control group, respectively (P < 0.05); the myocardial MDA level in the model group was 18.45 ± 2.91 nmol/mg, significantly higher than 11.59 ± 2.56 nmol/mg in the control group (P < 0.05). Compared with the model group, in the high-dose astaxanthin group the myocardial SOD and GSH-Px activity were 163.41 ± 17.42 U/mg and 148.39 ± 19.28 nmol/mg, which were significantly increased, respectively (P < 0.05), and the myocardial MDA level was 15.78 ± 2.01 nmol/mg, which was significantly decreased (P < 0.05).
Table 5. Effects of astaxanthin on myocardial superoxide dismutase and glutathione peroxidase activity and malondialdehyde level in rats.Tabla 5. Efectos de la astaxantina en el superóxido dismutasa miocárdico, la actividad de glutatión peroxidasa y el nivel de malondialdehido en ratas.
3.7. Effects of astaxanthin on myocardial MAPK signaling pathway in rats
Ratios of p-JNK/JNK, p-ERK1/2-ERK1/2, and p-p38 MAPK/p38 MAPK reflected the phosphorylation level of MAPK signaling pathway. The ratios of p-JNK/JNK, p-ERK1/2/ERK1/2, and p-p38 MAPK/p38 MAPK in the model group were 1.42 ± 0.11, 0.59 ± 0.08, and 0.58 ± 0.07, which were significantly higher than 0.88 ± 0.05, 0.18 ± 0.02, and 0.27 ± 0.06 in the control group, respectively (P < 0.05). Compared with the model group, the ratios of p-JNK/JNK, p-ERK1/2/ERK1/2 and p-p38 MAPK/p38 MAPK in the high-dose astaxanthin group were 0.92 ± 0.06, 0.22 ± 0.04 and 0.28 ± 0.05, respectively, which were significantly decreased, (P < 0.05) (-.
Figure 1. Effects of astaxanthin on myocardial JNK and p-JNK protein expression in rats. aP < 0.01 compared with control group; bP < 0.05 compared with model group; cP < 0.05 compared with low-dose astaxanthin group. JNK, c-Jun N-terminal kinase; p-JNK, phosphorylated c-Jun N-terminal kinase.Figura 1. Efectos de la astaxantina en las expresiones proteicas JNK miocárdica y p-JNK en ratas. aP < 0.01 comparado con el grupo de control; bP < 0.05 comparado con el grupo del modelo; cP < 0.05 comparado con el grupo de astaxantina de baja dosis. JNK, cinasa c-Jun N-terminal; p-JNK, cinasa fosforilada c-Jun N-terminal.
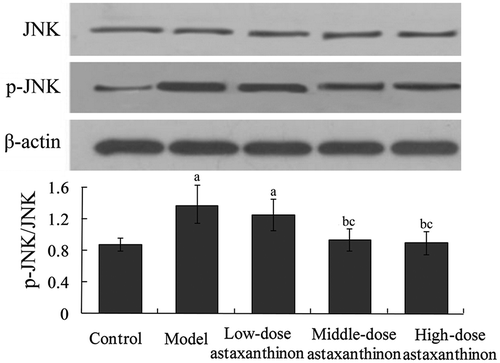
Figure 2. Effects of astaxanthin on myocardial ERK1/2 and p-ERK1/2 protein expression in rats. aP < 0.01 compared with control group; bP < 0.05 compared with model group; cP < 0.05 compared with low-dose astaxanthin group; dP < 0.05 compared with middle-dose astaxanthin group. ERK1/2, extracellular-signa1 regulated kinase 1/2; p-ERK1/2, phosphorylated extracellular-signa1 regulated kinase 1/2.Figura 2. Efectos de la astaxantina en las expresiones proteicas ERK1/2 miocárdica y p-ERK1/2 en ratas. aP < 0.01 comparado con el grupo de control; bP < 0.05 comparado con el grupo del modelo; cP < 0.05 comparado con el grupo de astaxantina de baja dosis; dP < 0.05 comparado con el grupo de astaxantina de dosis media. ERK1/2, cinasa 1/2 extracelular-signa1 regulada; p-ERK1/2, cinasa 1/2 fosforilada extracelular-signa1 regulada.
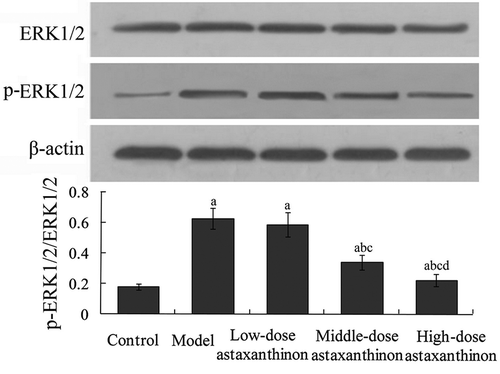
Figure 3. Effects of astaxanthin on myocardial p38 MAPK and p-p38 MAPK protein expression in rats. aP < 0.01 compared with control group; bP < 0.05 compared with model group; cP < 0.05 compared with low-dose astaxanthin group; dP < 0.05 compared with middle-dose astaxanthin group. p38 MAPK, p38 mitogen-activated protein kinase; p-p38 MAPK, phosphorylated p38 mitogen-activated protein kinase.Figura 3. Efectos de la astaxantina en las expresiones proteicas p38 MAPK cardíaca y p-p38 MAPK en ratas. aP < 0.01 comparado con el grupo de control; bP < 0.05 comparado con el grupo del modelo; cP < 0.05 comparado con el grupo de astaxantina de baja dosis; dP < 0.05 comparado con el grupo de astaxantina de dosis media. p38 MAPK, proteína cinasa p38 activada por mitógenos; p-p38 MAPK, proteína cinasa p38 fosforilada activada por mitógenos.
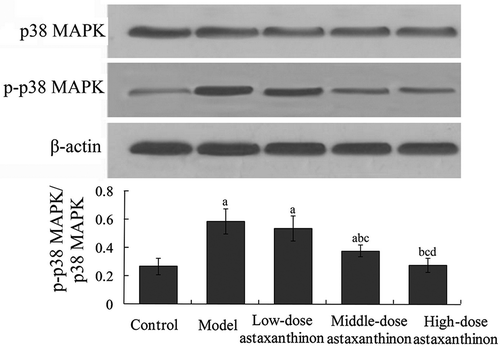
4. Discussion
It is found that changes of cardiac function and myocardial structure often occur in DM patients. This is different from hypertension, coronary heart disease, and other known heart diseases (Russell et al., Citation2008). DCM is the myocardial structure abnormity due to cardiac microvascular lesion, myocardial metabolic disturbance, and fibrosis caused by DM. DCM will lead to the left ventricular hypertrophy and diastolic and (or) systolic dysfunction. It is one of the most important causes of death in DM patients (Hayat et al., Citation2004). Therefore, DCM has become the focus and hot spot of the current medical research. At present, the mechanism of DCM is not yet clear, and the treatment of DCM often uses traditional drugs such as digitalis, angiotensin converting enzyme inhibitors, β-blockers, etc. (Hayat et al., Citation2004). It is of great significance to study the mechanism and treatment scheme of DCM. As a natural medicine, astaxanthin has been applied to the treatment of DM (Mashhadi et al., Citation2018) and related diseases such as diabetic nephropathy (Naito et al., Citation2004), cognition deficits (Zhou et al., Citation2015), sickness behavior (Ying et al., Citation2015), etc. However, the effects of astaxanthin on DCM have not been reported. In this study, the DM model was established and was intervened with astaxanthin. Results showed that at the end of 6 weeks, compared with the model group, the serum FBG, HbA1c, TC, and TG level in the astaxanthin group with certain doses were significantly reduced. This indicates that astaxanthin has an obvious lowering effect on blood glucose and blood lipid in DM rats. Pei et al. (Citation2012) found that astaxanthin can reduce the lipid levels of hyperlipidemia rats. In the study by Hussein et al. (Citation2007), astaxanthin can decrease the FBG level in SHR/NDmcr-cp (cp/cp) rat model. This basically conforms to the results in our study. In addition, compared with the model group, the body weight was significantly increased; the LVWI was significantly decreased; the LVSP, + dp/dtmax, and -dp/dtmax were significantly increased; and the LVEDP was significantly decreased. This is not reported in the previous studies. It is indicated that astaxanthin has obvious protective effects on the heart of DCM rats. A recent study has found that the oxidative stress plays an important role in the occurrence and development of DM and its complications (Opara, Citation2002). Oxidative stress refers to the tissue cell damage caused by excessive production of reactive oxygen species (ROS). ROS are the main substances that induce oxidative stress in the body, including superoxide anion, hydrogen peroxide, hydroxyl radicals, etc. When the production rate of ROS exceeds the clearance rate, the oxidative stress will occur (Higashi & Yoshizumi, Citation2004). The reaction of ROS with polyunsaturated fatty acid produces the lipid peroxide such as MDA. Therefore, the content of MDA can reflect the degree of oxidative stress damage (Pirinccioglu, Gökalp, Pirinccioglu, Kizil, & Kizil, Citation2010). SOD and GSH-Px can scavenge superoxide products and convert them into oxygen and water, so they are the detection indicators of intracellular reduction level (Djordjevic, Spasic, Jovanovic-Galovic, Djordjevic, & Grubor-Lajsic, Citation2004). It is found that, in mice with exercise-induced myocardial injury, astaxanthin can enhance the ability to scavenge oxygen free radicals and reduce the content of lipid peroxide in myocardial cells, thus playing a role in protecting myocardial cells (Aoi et al., Citation2003). In addition, Yang, Zhang, Zhu, Ruan, and Fu (Citation2009) found that astaxanthin can significantly increase the SOD and GSH-Px levels and decrease the MDA level in acetic acid-induced gastric ulcer rats. Results of this study showed that, compared with the model group, in the high-dose astaxanthin group the myocardial SOD and GSH-Px activity were significantly increased, and the myocardial MDA level was significantly decreased. This is basically identical with the results of the above studies.
MAPK is a serine/threonine protein kinase widely distributed in vertebrate cells. It is involved in regulating cellular growth, proliferation, differentiation, apoptosis, and other physiological processes (Seger & Krebs, Citation1995). p38 MAPK, JNK, and ERK1/2 are the main members of the MAPK family. p38 MAPK and JNK can be activated by stimuli of high-glucose ischemia-reperfusion, oxidative stress, and inflammatory factors (Wagner & Nebreda, Citation2009). ERK1/2 mainly regulates the mitogen-induced cell growth (Murakami-Mori, Mori, Bonavida, & Nakamura, Citation1999). It is found that the activation of the p38 MAPK signaling pathway is the common pathway of vasculopathy and retinopathy, neuralgia, nephropathy, and other complications of DM, and the phosphorylation of p38 MAPK is the key to activate this pathway (Riad et al., Citation2007). p-p38 MAPK can directly promote the activation and nuclear translocation of the downstream transcription factor NF-κB which initiates or enhances the transcription and expression of TNF-α, IL-6, and other inflammatory related genes, thus aggravating the damage of DCM (Qiao et al., Citation2012). In the study by Yan, Zhao, Zhang, and Lin (Citation2016), astaxanthin may inhibit acetaldehyde-induced apoptosis through blocking the activation of p38MAPK. In Li et al.’s study (Citation2015), astaxanthin can reduce the immune liver injury in ConA-induced autoimmune hepatitis by downregulation of JNK/p-JNK-mediated apoptosis and autophagy. In Kim, Koh, and Kim’s study (Citation2010), astaxanthin can down-regulate the activation of p-ERK1/2, thus regulating IL-6 production in activated microglial cells. In our study, compared with the model group, in the high-dose astaxanthin group the ratios of p-JNK/JNK, p-ERK1/2-ERK1/2, and p-p38 MAPK/p38 MAPK were significantly decreased. This indicates that astaxanthin can inhibit the activation of the MAPK signaling pathway, thereby exerting the cardioprotective effects. This is basically identical with the finding of the above studies.
4. Conclusions
Astaxanthin has protective effects on myocardial damage in DCM rats. The possible mechanism may be related to its improving blood glucose and blood lipid metabolism, resisting oxidative stress and inflammatory reaction, and inhibition of the activation of the MAPK signaling pathway. This study has provided an experimental basis for clinical application of astaxanthin to prevention and treatment of DCM. Whether there are other mechanisms in protective effects of astaxanthin on DCM needs to be further confirmed.
Disclosure statement
No potential conflict of interest was reported by the authors.
Additional information
Funding
References
- Aoi, W., Naito, Y., Sakuma, K., Kuchide, M., Tokuda, H., Maoka, T., Yoshikawa, T. (2003). Astaxanthin limits exercise-induced skeletal and cardiac muscle damage in mice. Antioxidants & Redox Signaling, 5(1), 139–144.
- Association, A. D. (2009). Diagnosis and classification of diabetes mellitus. Diabetes Care, 32(Suppl 1), S62–S67.
- Brahma, M. K., Pepin, M. E., & Wende, A. R. (2017). My sweetheart is broken: Role of glucose in diabetic cardiomyopathy. Diabetes & Metabolism Journal, 41(1), 1–9.
- Chen, F. P., Li, B. G., & Wu, X. M. (2009). Significance of detection of GLU, HbA1c and blood lipid in type 2 diabetes patients. China Tropical Medicine, 9(6), 1059–1060. In Chinese
- Djordjevic, A., Spasic, S., Jovanovic-Galovic, A., Djordjevic, R., & Grubor-Lajsic, G. (2004). Oxidative stress in diabetic pregnancy: SOD, CAT and GSH-Px activity and lipid peroxidation products. Journal of Maternal-Fetal & Neonatal Medicine, 16(6), 367–372.
- Ge, M., Ma, S., Tao, L., & Guan, S. (2009). The effect of gypenosides on cardiac function and expression of cytoskeletal genes of myocardium in diabetic cardiomyopathy rats. American Journal of Chinese Medicine, 37(6), 1059–1068.
- Guo, S. X., Zhou, H. L., Huang, C. L., You, C. G., Fang, Q., Wu, P., … Han, C. M. (2015). Astaxanthin attenuates early acute kidney injury following severe burns in rats by ameliorating oxidative stress and mitochondrial-related apoptosis. Marine Drugs, 13(4), 2105–2123.
- Guo, Y., Shi, D. Z., Yin, H. J., & Chen, K. J. (2007). Effects of Tribuli saponins on ventricular remodeling after myocardial infarction in hyperlipidemic rats. American Journal of Chinese Medicine, 35(2), 309–316.
- Harrison, R., & Bray, R. C. (1997). Xanthine oxidase: Enzymology and pathophysiology. Biochemical Society Transactions, 25(3), 749–750.
- Hayat, S. A., Patel, B., Khattar, R. S., & Malik, R. A. (2004). Diabetic cardiomyopathy: Mechanisms, diagnosis and treatment. Clinical Science, 107(6), 539–557.
- Higashi, Y., & Yoshizumi, M. (2004). Oxidative stress, reactive oxygen species. Nihon Rinsho, 62(1), 49–55. Article in Japanese
- Higuera-Ciapara, I., Félix-Valenzuela, L., & Goycoolea, F. M. (2006). Astaxanthin: A review of its chemistry and applications. Critical Reviews in Food Science and Nutrition, 46(2), 185–196.
- Hussein, G., Nakagawa, T., Goto, H., Shimada, Y., Matsumoto, K., Sankawa, U., & Watanabe, H. (2007). Astaxanthin ameliorates features of metabolic syndrome in SHR/NDmcr-cp. Life Sciences, 80(6), 522–529.
- Kim, Y. H., Koh, H. K., & Kim, D. S. (2010). Down-regulation of IL-6 production by astaxanthin via ERK-, MSK-, and NF-κB-mediated signals in activated microglia. International Immunopharmacology, 10(12), 1560–1572.
- Lakshmanan, A. P., Harima, M., Sukumaran, V., Soetikno, V., Thandavarayan, R. A., Suzuki, K., … Watanabe, K. (2012). Modulation of AT-1R/AMPK-MAPK cascade plays crucial role for the pathogenesis of diabetic cardiomyopathy in transgenic type 2 diabetic (Spontaneous Diabetic Torii) rats. Biochemical Pharmacology, 83(5), 653–660.
- Li, J., Xia, Y., Liu, T., Wang, J., Dai, W., Wang, F., … Guo, C. (2015). Protective effects of astaxanthin on ConA-induced autoimmune hepatitis by the JNK/p-JNK pathway-mediated inhibition of autophagy and apoptosis. PLoS One, 10(3), e0120440.
- Luo, B., Li, B., Wang, W., Liu, X., Liu, X., Xia, Y., … An, F. (2014). Rosuvastatin alleviates diabetic cardiomyopathy by inhibiting NLRP3 inflammasome and MAPK pathways in a type 2 diabetes rat model. Cardiovascular Drugs and Therapy, 28(1), 33–43.
- Martinez, P. F., Bonomo, C., Guizoni, D. M., Junior, S. A., Damatto, R. L., Cezar, M. D., … Okoshi, M. P. (2015). Influence of N- acetylcysteine on oxidative stress in slow-twitch soleus muscle of heart failure rats. Cellular Physiology & Biochemistry, 35(1), 148–159.
- Mashhadi, N. S., Zakerkish, M., Mohammadiasl, J., Zarei, M., Mohammadshahi, M., & Haghighizadeh, M. H. (2018). Astaxanthin improves glucose metabolism and reduces blood pressure in patients with type 2 diabetes mellitus. Asia Pacific Journal of Clinical Nutrition, 27(2), 341–346.
- Memon, A. A., Wahid, H., Rosnina, Y., Goh, Y. M., Ebrahimi, M., & Nadia, F. M. (2012). Effect of antioxidants on post thaw microscopic, oxidative stress parameter and fertility of Boer goat spermatozoa in Tris egg yolk glycerol extender. Animal Reproduction Science, 136(1–2), 55–60.
- Murakami-Mori, K., Mori, S., Bonavida, B., & Nakamura, S. (1999). Implication of TNF receptor-I-mediated extracellular signal-regulated kinases 1 and 2 (ERK1/2) activation in growth of AIDS-associated Kaposi’s sarcoma cells: A possible role of a novel death domain protein MADD in TNF-alpha-induced ERK1/2 activation in Kaposi’s sarcoma cells. Journal of Immunology, 162(6), 3672–3679.
- Naito, Y., Uchiyama, K., Aoi, W., Hasegawa, G., Nakamura, N., Yoshida, N., … Yoshikawa, T. (2004). Prevention of diabetic nephropathy by treatment with astaxanthin in diabetic db/db mice. Biofactors, 20(1), 49–59.
- Opara, E. C. (2002). Oxidative stress, micronutrients, diabetes mellitus and its complications. The Journal of the Royal Society for the Promotion of Health, 122(1), 28–34.
- Pei, L. P., Bai, Y., Huang, H. Q., Li, Z. Y., Liu, W. Z., & Hui, B. D. (2012). Effect of astaxanthin on the lipid levels of hyperlipidemia rat. Chinese Journal of Gerontology, 32(18), 1936–3938. In Chinese
- Pirinccioglu, A. G., Gökalp, D., Pirinccioglu, M., Kizil, G., & Kizil, M. (2010). Malondialdehyde (MDA) and protein carbonyl (PCO) levels as biomarkers of oxidative stress in subjects with familial hypercholesterolemia. Clinical Biochemistry, 43(15), 1220–1224.
- Qiao, H., Zhang, X., Zhu, C., Dong, L., Wang, L., Zhang, X., … Cao, X. (2012). Luteolin downregulates TLR4, TLR5, NF-κB and p-p38MAPK expression, upregulates the p-ERK expression, and protects rat brains against focal ischemia. Brain Research, 1448, 71–81.
- Riad, A., Unger, D., Du, J., Westermann, D., Mohr, Z., Sobirey, M., … Tschöpe, C. (2007). Chronic inhibition of p38MAPK improves cardiac and endothelial function in experimental diabetes mellitus. European Journal of Pharmacology, 554(1), 40–45.
- Ritchie, R. H., Zerenturk, E. J., Prakoso, D., & Calkin, A. C. (2017). Lipid metabolism and its implications for type 1 diabetes-associated cardiomyopathy. Journal of Molecular Endocrinolgy, 58(4), R225–R240.
- Rossen, J. D. (1996). Abnormal microvascular function in diabetes: Relationship to diabetic cardiomyopathy. Coronary Artery Disease, 7(2), 133–138.
- Russell, N. E., Foley, M., Kinsley, B. T., Firth, R. G., Coffey, M., & McAuliffe, F. M. (2008). Effect of pregestational diabetes mellitus on fetal cardiac function and structure. American Journal of Obstetrics and Gynecology, 199(3), 312.e1-312.e7.
- Seger, R., & Krebs, E. G. (1995). The MAPK signaling cascade. FASEB Journal, 9(9), 726–735.
- Stannus, O., Jones, G., Cicuttini, F., Parameswaran, V., Quinn, S., Burgess, J., & Ding, C. (2010). Circulating levels of IL-6 and TNF-α are associated with knee radiographic osteoarthritis and knee cartilage loss in older adults. Osteoarthritis and Cartilage, 18(11), 1441–1447.
- Sun, X., Chen, R. C., Yang, Z. H., Sun, G. B., Wang, M., Ma, X. J., … Sun, X. B. (2014). Taxifolin prevents diabetic cardiomyopathy in vivo and in vitro by inhibition of oxidative stress and cell apoptosis. Food and Chemical Toxicology, 63, 221–232.
- Uzgare, A. R., Kaplan, P. J., & Greenberg, N. M. (2003). Differential expression and/or activation of p38MAPK, Erkl/2, and Jnk during the initiation and progression of prostate cancer. Prostate 55: 128-139. Prostate, 55, (2), 128–139. doi:10.1002/pros.10212
- Wagner, E. F., & Nebreda, A. R. (2009). Signal integration by JNK and p38 MAPK pathways in cancer development. Nature Reviews Cancer, 9(8), 459–537.
- Wen, H. L., Liang, Z. S., Zhang, R., & Yang, K. (2013). Anti-inflammatory effects of triptolide improve left ventricular function in a rat model of diabetic cardiomyopathy. Cardiovascular Diabetology, 12, 50.
- Yan, T., Zhao, Y., Zhang, X., & Lin, X. (2016). Astaxanthin inhibits acetaldehyde-induced cytotoxicity in SH-SY5Y cells by modulating Akt/CREB and p38MAPK/ERK signaling pathways. Marine Drugs, 14(3), In Chinese 56–58.
- Yang, Q., Zhang, Z., Zhu, X., Ruan, H., & Fu, Y. (2009). Therapeutic effect of astaxanthin on acetic acid-induced gastric ulcer in rats. Acta Pharmaceutica Sinica, 44(5), 558–560. In Chinese
- Ying, C. J., Zhang, F., Zhou, X. Y., Hu, X. T., Chen, J., Wen, X. R., … Song, Y. J. (2015). Anti-inflammatory effect of astaxanthin on the sickness behavior induced by diabetes mellitus. Cellular & Molecular Neurobiology, 35(7), 1027–1037.
- Zhou, X., Zhang, F., Hu, X., Chen, J., Wen, X., Sun, Y., … Song, Y. (2015). Inhibition of inflammation by astaxanthin alleviates cognition deficits in diabetic mice. Physiology & Behavior, 151, 412–420.