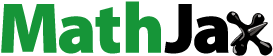
ABSTRACT
This study aimed to investigate effects of amidated low methoxyl (ALM, 0.3%, w/v) pectin on physiochemical and structural properties of polymerized whey proteins (PWP, 10%, w/v) prepared by thermal aggregation at 85°C for 10–30 min. Particle size, zeta potential, isoelectric point (IEP), surface hydrophobicity, free sulphydryl group, rheological properties and the secondary structure of all samples were analysed. ALM pectin increased mean diameter and narrowed size distribution of PWP samples (p < 0.05) regardless of heating time. Addition of ALM pectin shifted the IEP of PWP‒pectin complex to lower pH, which decreased with heating time increased. ALM pectin decreased the surface hydrophobicity of PWP heated for 30 min pronouncedly. Whey protein (WP) complexed with ALM pectin showed decreased gelation time and rheological properties of improved elasticity. ALM pectin had no considerable effect on secondary structure of PWP. Data indicated that ALM pectin could alter physiochemical properties of heat-induced polymerized WPns.
RESUMEN
El presente estudio se propuso investigar los efectos de la pectina amidada de bajo metoxilo (ALM, 0.3%, p/v) en las propiedades fisicoquímicas y estructurales de las proteínas de suero polimerizadas (PWP, 10%, w/v) y preparadas mediante el método de agregación térmica a 85°C durante 10–30 min. Asimismo, en el estudio se analizaron: el tamaño de las partículas, el potencial zeta, el punto isoeléctrico, la hidrofobicidad superficial, el grupo sulfhidrilo libre, las propiedades reológicas y la estructura secundaria de todas las muestras. Se constató que la pectina ALM aumentó el diámetro medio y estrechó la distribución de tamaños de las muestras de PWP (p < 0.05) independientemente de la duración del calentamiento. Por otra parte, la adición de pectina ALM llevó el punto isoeléctrico del complejo pectina PWP a un pH más bajo, lo cual fue disminuyendo a medida que aumentó el tiempo de calentamiento. La pectina ALM redujo drásticamente la hidrofobicidad superficial de la PWP calentada durante 30 min. La proteína de suero en complejo con la pectina ALM mostró tener un tiempo de gelificación más reducido y propiedades reológicas de mayor elasticidad. La pectina ALM no afectó sensiblemente la estructura secundaria de PWP. Los datos dan cuenta de que la pectina ALM puede alterar las propiedades fisicoquímicas de las proteínas de suero polimerizadas mediante su exposición al calor.
1. Introduction
Whey proteins (WP) have been extensively used as nutritional ingredients in food such as infant formula, sports drinks and other functional foods (Sootsman et al., Citation2008). It possesses many bioactive functions including anti-cancer activity (Bounous, Batist, & Gold, Citation1991), improving immune system (Saint-Sauveur, Gauthier, Boutin, & Montoni, Citation2008) and promoting bone health (Takada et al., Citation1997). However, its functionality is limited due to the low molecular weight (~17 kDa) and compact structure of β-lactoglobulin, which is the most abundant fraction in WP (Fuente, Singh, & Hemar, Citation2002). Physical, chemical and enzymatic methods have been developed to improve WP functional properties (Cheng et al., Citation2017). Thermal treatment of WP requires a heating step to unfold the native protein, followed by molecules cross-linking to give a protein aggregate (Nicolai, Britten, & Schmitt, Citation2011). Heat-induced WP aggregate, also termed as polymerized whey protein (PWP), showed higher viscosity, desirable gelation property (Mezaize, Chevallier, Le Bail, & de Lamballerie, Citation2009). Changes in WP molecules during thermal treatment as well as properties of polymerized WPs are modulated by many factors such as protein concentration, heating load, pH, mineral content and other ingredients (Beaulieu, Corredig, Turgeon, Wicker, & Doublier, Citation2005).
Pectin is a linear anionic polysaccharide built from polygalacturonic acid subunit which is partially esterified with methoxyl groups. Based on the degree of esterification (DE), pectin is divided into low methoxyl (LM) and high methoxyl (HM) pectin with DE lower and higher than 50%, respectively (Fu & Rao, Citation2001). Carboxylic groups of LM pectin can be amidated by a relatively simple chemical modification, obtaining amidated low-methoxyl (ALM) pectin (Ramirez, RodríGuez, Uresti, Velazquez, & VáZquez, Citation2007) which is useful in certain applications (Capel, Nicolai, Durand, Boulenguer, & Langendorff, Citation2006). It was believed that WP and LM pectin complex was formed at pH between 4 and 7 without heating mainly via electrostatic forces and hydrogen bonding (Girard, Turgeon, & Gauthier, Citation2002). In previous works, effects of LM pectin on denaturation and aggregation behaviours of WP have been studied (Krzeminski, Prell, Weiss, & Hinrichs, Citation2014; Neirynck, Van der Meeren, et al., Citation2007). LM pectin could interact with protein via electrostatic forces, which plays an important in denaturation and aggregation of WP during heating, thus influence the properties of polymerized WP (Zhang, Hsieh, & Vardhanabhuti, Citation2014; Zhang & Vardhanabhuti, Citation2014)
ALM pectin shows different physical and chemical properties from LM pectin. Amidation replaces methoxyl groups (hydrophobic) with amide groups (hydrophilic), resulting in a more hydrophilic nature (Ramirez et al., Citation2007). Compared with LM pectin, ALM pectin improved water holding capacity of fish gels (Ramirez et al., Citation2007), increased hardness, chewiness and cohesiveness of the fish mince gels (Uresti, López-Arias, Ramírez, & Vázquez, Citation2003), and improved shear stress of surimi gels (Barrera, Ramirez, Gonzalezcabriales, & Vazquez, Citation2002).
Polymerized whey protein and pectin have been widely used in the functional foods formulation such as Chinese Laosuannai (Wang, Gao, Zhang, Wang, & Guo, Citation2015), corn-based yogurt-like product (Wang, Zheng et al. Citation2017), and fermented goat milk (Wang, Wang et al., Citation2017). The effects of pectin on the physiochemical properties of polymerized WP will be of significance for the application in the food industry. The objectives of this study were to investigate effects of amidated LM pectin (0.3%, w/v) on the physiochemical and structural properties of polymerized WPs (10%, w/v) prepared by heating at 85°C at pH 7 for 10–30 min.
2. Materials and methods
2.1. Materials
WP isolate (WPI, 93.14% protein, 69.2% β-lactoglobulin, 14.2% α-lactalbumin, 3.3% bovine serum albumin and 2.1% immunoglobulin G, w/w) was purchased from Fonterra Co-operative Group (Auckland, New Zealand). Major ions quantified in the WP isolate are 0.70 mg/g calcium and 5.6 mg/g sodium. Citrus pectin with DE at 23% and amidation at 24% was graciously gifted by CPKELCO (Lille Skensved, Denmark). 1-Anilinonaphthalene-8-sulfonic acid (ANS), 5,5ʹ-Dithiobis-(2-nitrobenzoic acid) (DTNB) were purchased from Sigma (Sigma-Aldrich, St. Louis, MO, US). All other reagents were purchased from Beijing Works (Beijng, China). Deionized water (resistivity of 18.2 MΩ) was obtained using a Milli-Q deionization reversed osmosis system (Millipore Corp., Bedford, MA, USA).
2.2. Preparation of stock solutions
WP stock solution (20%, w/v) was prepared by dissolving WP powder slowly into deionized water at room temperature with the help of magnetic stirring for 2 h. When calculating the amount of WP powder to be added, a correction for the protein content of the powder was taken into account. Pectin solution (5%, w/v) was prepared by hydrating accurately weighed pectin powder into deionized water and then heated at 65°C for 2 h under strong stirring. Both stock solutions were stored at 4°C overnight to allow complete hydration.
2.3. Polymerization of whey protein in presence of ALM pectin
Stock solutions of WP and pectin were mixed at appropriate amount and then added with deionized water to form the final protein concentration of 10% (w/v) and pectin 0.3% (w/v). The mixtures were stirred for 30 min and then adjusted from original pH of about 6.8–7 with 1 M NaOH. A series of samples in sealed 50-mL beakers were heated at 85°C with stirring for 10, 20 and 30 min, respectively. All samples were rapidly cooled in ice bath. WPs without pectin under the same treatment were set as controls. Our previous studies showed that PWP induced by heating 10% (w/v) WP solution at pH 7.0 showed good physiochemical and functional properties (Wang et al., Citation2015; Wang, Zheng et al., Citation2017). Therefore, 10% WP solution was selected in this study. Mixture of polymerized WP and pectin was abbreviated as PWP–pectin. All samples were prepared in triplicates for three trials.
2.4. Particle size measurement
Hydrodynamic diameter of samples diluted to 1% (w/v) was determined by a Zetasizer model Nano-Z (Malvern Instruments, Malvern, Worcestershire, WR, UK) with a scattering angle of 173° at ambient temperature. Polydispersity index (PDI) calculated as the best fit between the measured scattered pattern and that predicted by light scattering theory was used to indicate the size distribution. The mean intensity-weighted size (Z-average diameter) is reported for all samples. Data analysis was performed according to the Stokes–Einstein equation:
where d(H) = diffusion coefficient, K = Boltzmann’s constant, T = absolute temperature (K), η = viscosity (mPa.s) and D = hydrodynamic diameter (nm).
2.5. Surface zeta potential and isoelectric point measurement
Surface zeta potential of all samples was measured using a Zetasizer model Nano-Z (Malvern Instruments, Malvern, Worcestershire, WR, UK). Isoelectric point (IEP) was also determined by measuring the zeta potential of all samples between pH 3 and 8 adjusted using 1 M HCl or NaOH. All measurements were performed in folded capillary cells at ambient temperature. Zeta potential was calculated based on the Henry equation:
where UE is the electrophoretic velocity; Z is the zeta potential (mV); e is the permittivity of the biopolymer mixture; h is the dispersion viscosity (mPa.s); f(ka) = Henry’s function. A value of 1.0 was used as the Huckel approximation as the zeta potential was measured in water solvent.
2.6. Surface hydrophobicity determination
All samples diluted at 0.005% (w/v) level were measured for surface hydrophobicity using ANS probe with a spectrofluorometer (RF-5301PC, Shimatzu UV, Japan). Twenty millilitres of ANS probe (8 mM) were added into 4 mL samples and then kept in dark for 15 min before determination. Emission spectra were recorded from 400 to 700 nm at an excitation wavelength of 390 nm.
2.7. Free sulphydryl groups determination
Free sulphydryl groups were determined using Ellman’s reagent DTNB method with a UV-visible spectrophotometer (UV2550, Shimadzu, Tokyo, Japan). The free sulphhydryl group content was calculated as the following equation:
where A412 = absorbance value, D = dilution factor, C = protein concentration (g/100 mL), 73.53 is obtained from 106/1.36 × 104, 1.36 × 104 = molar extinction coefficient.
2.8. Rheological properties analysis
Rheological properties of WP and WP–pectin mixture were studied using a rheometer (DHR, TA Instruments, USA) equipped with 60 mm diameter aluminium parallel plate. The strain was 0.1% and the frequency was 1 Hz. The programmed procedure was set according to that of Sun et al. (Citation2011) with little modification. Solutions were heated from 25°C to 90°C at a rising rate of 5°C/min, maintained at 90°C for 30 min, then cooled down to 25°C at a rate of 5°C/min and kept at 25°C for 10 min.
2.9. Far-ultraviolet Circular Dichroism Spectroscopy (Far-UV CD)
The secondary structure of all samples was determined using a CD spectropolarimeter (MOS-500, Bio-logic, France). Sample dispersions (0.1 mg/mL) were obtained and recorded at 25°C in the spectral range from 190 to 260 nm using a quartz cuvette with an optical path of 0.1 cm. Mean residue ellipticity ([θ], deg· cm2 dmol−1) was determined according to the following equation (Tarhan, Spotti, Schaffter, Corvalan, & Campanella, Citation2016):
where θ corresponds to the observed ellipticity (deg); MRW is the mean residue weight, which was estimated to be 119 according to the main components of WP; d is the path length (cm); and c is the protein concentration (g/mL). The secondary structure contents of the samples were calculated using DichroWeb online http://dichroweb.cryst.bbk.ac.uk/html/home.shtml with CONTIN method.
2.10. Data analysis
All measurements were conducted in triplicates for three trials. All data obtained from analysis were expressed as mean ± SD. The significant differences of data between samples and control were calculated using version SPSS 20 (SPSS Inc. Chicago, IL, US). The significance level was set at p < 0.05. Data were checked for homogeneity by Leveneǐs test. When the data were homogeneous, one-way analysis of variance and then a least squared differences model was used. All the figures were drawn by Origin 8.0 (OriginLab Corporation, Northampton. USA).
3. Results and discussion
3.1. Effects of ALM pectin on particle size of polymerized whey protein
Interaction between pectin and WP is often studied using particle size parameters (Oweng, Erica, & Davidjulian, Citation2009). shows the effects of ALM pectin (0.3%) on mean particle size of polymerized WPs prepared at different heating times. Polymerized WPs showed diameters in the range of 40‒80 nm, which was consistent with the results reported by Dai et al. (Citation2015) and Silva et al. (Citation2010). No significant difference in particle size was found among all polymerized WP samples, p > 0.05. It was reported that heating of WP solution (10%, pH 6.8) at 80°C for 10 min may result in almost complete denaturation (Haque, Aldred, Chen, Barrow, & Adhikari, Citation2013) and prolonged heating time may result in more aggregates other than aggregates size. Compared with polymerized WPs alone, all PWP‒pectin samples showed significantly higher diameters during heating, p < 0.05. However, no significant difference was observed for PWP‒pectin mixtures treated with different heating time, p > 0.05.
Figure 1. Effects of ALM pectin on the mean particle size of polymerized whey proteins prepared by different heating time.Note: Different lower case letters denote significance between samples heated for different times at level of p < 0.05; ifferent uppercase letters denote significance between PWP and PWPectin samples at level of p < 0.05
Figura 1. Efectos de la pectina ALM en el tamaño medio de las partículas de proteínas de suero polimerizadas y preparadas mediante calentamiento de distinta duración.Nota: Las distintas letras minúsculas indican diferencias significativas entre las muestras calentadas durante diferente tiempo a un nivel de p < 0.05; las distintas letras mayúsculas indican diferencias significativas entre las muestras de PWP y pectina-PWP a un nivel de p < 0.05.
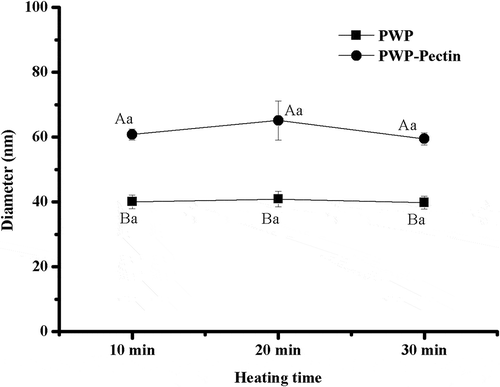
Polymerized WPs showed PDI values of 0.72 ± 0.02, 0.72 ± 0.05, and 0.70 ± 0.04 with heating time increased from 10 to 30 min, suggesting a broader size distribution for the WP aggregates. However, PWP‒pectin mixtures, having significantly lower PDI values (0.46 ± 0.06, 0.46 ± 0.04 and 0.43 ± 0.01) (p < 0.05), tended to be much narrower size distributed. WP and pectin may interact via electrostatic force during heating, which may reduce the contact frequency of WP molecules and then prevented the formation of large aggregates. Similar results were reported by Martins et al. (Citation2016).
3.2. Effects of ALM pectin on surface zeta potential and isoelectric point of polymerized whey protein
Electrostatic interaction was identified as the major driving force involved in complex formation between proteins and anionic polysaccharides in solution (Liu, Shim, Shen, Wang, & Reaney, Citation2017). This interaction also causes change of surface charge (Mounsey, O’Kennedy, Fenelon, & Brodkorb, Citation2008). All samples were evaluated for surface electrical properties by zeta potential and the results are shown in .
Table 1. Effects of ALM pectin on zeta potential of polymerized whey proteins prepared by different heating time.
Tabla 1. Efectos de la pectina ALM en el potencial zeta de las proteínas de suero polimerizadas y preparadas mediante calentamiento de distinta duración.
Both WP and pectin carried net negative surface charge at pH 7, which is above the IEP of WP and intrinsic pK of pectin (Neirynck, Kowalski et al., Citation2007). Polymerized WP showed the zeta potential in the range of −39.16 ± 0.21 to −41.12 ± 0.07 mV. When heated together with pectin, protein and pectin complexes are formed with more negative zeta potential ranging from −44.87 ± 0.09 to −48.84 ± 0.10 mV with heating time prolonged from 10 to 30 min, indicating more stable complex (Chen, Li, Ding, & Suo, Citation2012). Interaction between WP and pectin is a result of electrostatic attraction between negatively charged carboxylate groups of pectin and positively charged amino acid residues of WP (Jones & Mcclements, Citation2011). Pectin introduced a net charge to the system, resulting in the larger negative zeta potential magnitude than pure protein, which presumably indicates an adsorption of pectin onto WP, indicating improvement of system stability of WP by addition of pectin (Vá & Synytsya, Citation2009).
IEP is the pH at which a particular molecule carries no net electrical charge. Although the method differs from isoelectrofocusing, an ‘apparent’ IEP could be defined as the pH value where is zero charge (Guyomarc’H, Renan, Chatriot, Gamerre, & Famelart, Citation2007). shows the effects of ALM pectin on the IEP of polymerized WPs prepared by heating for different times. Polymerized WPs displayed approximated zeta potential equal to zero at pH 4.5–5, which agreed with a previous study (Krzeminski et al., Citation2014). Compared with control, IEP of protein and pectin complexes shifted to lower pH value, which may be due to more carboxyl groups (‒COO‒) introduced by ALM pectin. The IEP of PWP‒pectin complex decreased with heating time increased. Similar results for LM pectin were reported by others (Girard et al., Citation2002).
3.3. Effects of ALM pectin on free sulfhydryl groups of polymerized whey protein
β-Lactoglobulin has two intramolecular disulphide bonds and one embedded free sulohydryl group while α-lactalbumin has four intramolecular disulphide bonds and no thiol group (Qi, Wang, Chen, Guo, & Wang, Citation2016). WP aggregates mainly by forming intermolecular disulphide bonds (Kehoe, Wang, Morris, & Brodkorb, Citation2011). Thus, free sulphydryl groups content of all samples was determined and the results are shown in . Polymerized WP heated for 10 min showed free sulphydryl groups content of 2.21 ± 0.20 μmol/g. An increase observed at heating time of 20 min may be due to the deprivation of sulfpydryl group from α-lactalbumin by β-lactoglobulin, which caused more free sulphydryl to the system (And & Foegeding, Citation1999). No significant difference in free sulphydryl group content between PWP and PWP–pectin was observed for 10 min-heating samples, p > 0.05. However, significantly lower free sulphydryl group contents were found for samples heated for 20 and 30 min (p < 0.05), indicating more disulphide bonds formation. It is suggested that aggregation of WP may be improved by ALM pectin after a lag period. Similarly, Qi et al. (Citation2016) found that free sulphydryl groups of WP was mitigated by conjugating with sugar beet pectin.
Figure 3. Effects of ALM pectin on free sulphydryl group content of polymerized whey proteins prepared by different heating times.Note: Different lower case letters denote significance between samples heated for different time at level of p < 0.05; Different uppercase letters denote significance between PWP and PWPectin samples at level of p < 0.05.
Figura 3. Efectos de la pectina ALM en el contenido del grupo sulfhidrilo libre de las proteínas de suero polimerizadas y preparadas mediante calentamiento de distinta duración.Nota: Las distintas letras minúsculas indican diferencias significativas entre las muestras calentadas durante diferentes tiempos a un nivel de p < 0.05; las distintas letras mayúsculas indican diferencias significativas entre las muestras de PWP y pectina-PWP a un nivel de p < 0.05.
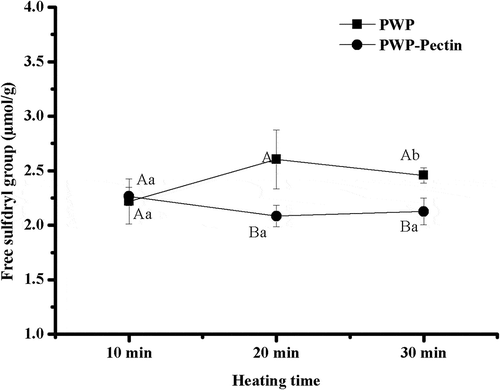
3.4. Effects of ALM pectin on surface hydrophobicity of polymerized whey protein
ANS probe is commonly used to determine the hydrophobicity due to its interaction with exposed hydrophobic groups of protein resulting in increased intensity (Valencia-Flores, Hernandez-Herrero, Guamis, & Ferragut, Citation2013). Fluorescence intensity of all samples was measured and the results are shown in .
Figure 4. Effects of ALM pectin on surface hydrophobicity of polymerized whey proteins prepared by different heating times.
Figura 4. Efectos de la pectina ALM en la hidrofobicidad superficial de las proteínas de suero polimerizadas y preparadas mediante calentamiento de distinta duración.
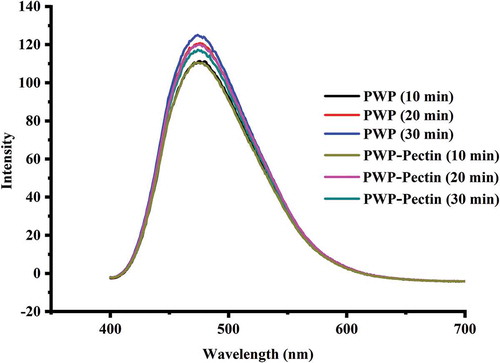
Fluorescence intensity of polymerized WP increased with prolong heating time, indicating the more exposed hydrophobic groups. All PWP–pectin mixtures showed lower hydrophobicity value in comparison with individual PWP presumably due to the hydrophilic nature of ALM pectin, indicating the influence of ALM pectin on the conformation of polymerized WP. The results also suggested that ALM pectin would lead to the increase in protein colloidal stability due to the lower surface hydrophobicity and more negative zeta potential (Ryan & Foegeding, Citation2015), especially with extended heating time (30 min).
3.5. Effects of ALM pectin on rheological properties of whey protein
Gelation of aqueous WP can be induced by a simple heating process (Wijaya, Meeren, & Patel, Citation2016), and rheological properties can reflect the gel structures and formation mechanisms. All samples were determined for the dynamic rheological properties using a temperature-controlled rheometer and the results are shown in . Storage modulus (G′) values were much greater than those of G″ during the heating and cooling process. Therefore, more focus was given to the behaviour of G′.
Figure 5. Changes of storage modulus (G’) for whey protein (a) and mixture of whey protein and pectin (b) during heating‒cooling treatment.
Figura 5. Cambios en los módulos de almacenamiento (G’) para las proteínas de suero (a) y una mezcla de proteínas de suero y pectina (b) durante el tratamiento de calentamiento-enfriamiento.
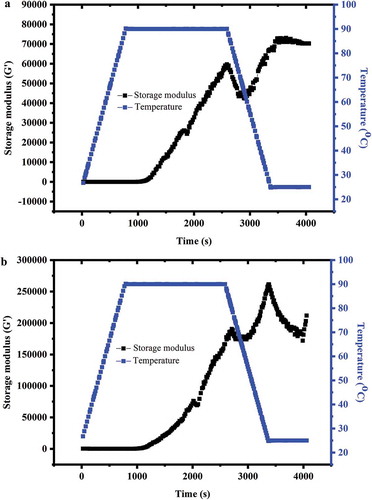
Storage modulus (G′), the indicator of material’s elasticity degree reflects the solid-like behaviour of materials. A higher G′ value indicates the predominant elastic behaviour of the sample (Sutariya & Patel, Citation2017). ) shows storage modulus of WP during heating and cooling cycle. WP showed a curve pattern similar to general globular protein (Gosal & Ross-Murphy, Citation2000). During ascending temperature, G′ was very small at almost 0 Pa.s. A surge point where G′ sharply increased was observed at approximate 2202 s, which was at 90°C, suggesting the formation of a gel-like structure. A different gelation temperature of 85°C for WP was reported by Sun et al. (Citation2011) Protein concentration and environment conditions were responsible for this difference (Fuente et al., Citation2002). During the cooling process, the G′ increased and then reached the plateau value, showing similar trend with that reported by Tavares and Jald (Citation2003).
Although ALM pectin addition did not change the curve trend, gelation time and corresponding values were quite different. WP–pectin mixture showed remarkable lower gelation time (1986 s) than that of WP (2202 s), p < 0.05. WP and pectin mixture showed almost two-fold higher G’ value than that of WP, indicating more viscoelasticity property. ALM pectin forms gel by reaction with calcium just like LM pectin (Fu & Rao, Citation2001; May, Citation1990). The network may be strengthened by junction zones created by pectin–calcium interactions (Imeson & Imeson, Citation2009). However, the current environment (low calcium content and high pH) used in this study was not sufficient for the formation of pectin gel, of which should be pH 2.5–6. Also, the dominant gel was WP due to the high protein concentration, and pectin may interact with the aggregates and favour the formation of stronger gel via electrostatic forces and hydrogen bonding (Imeson & Imeson, Citation2009). Similar result has also been reported by Li and Zhong (Citation2016). Besides the ‒OH groups, ALM pectins have three other groups: carboxylic acid (‒COOH), amide (NH2) and methoxyl (‒OCH3) (Uresti et al., Citation2003). Hydrogen bonding as minor interactions may be considered to coexist with electrostatic interaction. However, hydrophobic interactions can be neglected due to the hydrophilic backbone of ALM pectin (Ru, Wang, Lee, Ding, & Huang, Citation2012). During cooling, for both WP and mixture of WP and pectin, the storage modulus (G’) increased may be due to the formation of additional non-covalent interactions among denatured proteins and pectin molecules (i.e. the intermolecular hydrogen bands in gel structure). However, for WP alone, a decrease in storage modulus (G’) was observed before the temperature decreased to about 60°C may be due to that some of the interactions between WPaggregates were destroyed.
3.6. Effects of ALM pectin on secondary structure of polymerized whey protein
To determine the conformational changes of polymerized WP in presence of ALM pectin, CD spectroscopy from 190 to 250 nm was applied for all samples and the results are shown in . The estimated secondary structure content of WP is shown in .
Table 2. Effects of ALM pectin on secondary structure (%) of polymerized whey proteins prepared by different heating times.
Tabla 2. Efectos de la pectina ALM en la estructura secundaria (%) de las proteínas de suero polimerizadas y preparadas mediante calentamiento de distinta duración.
Figure 6. Effects of ALM pectin on secondary structure of polymerized whey proteins prepared by different heating times.
Figura 6. Efectos de la pectina ALM en la estructura secundaria de las proteínas de suero polimerizadas y preparadas mediante calentamiento de distinta duración.
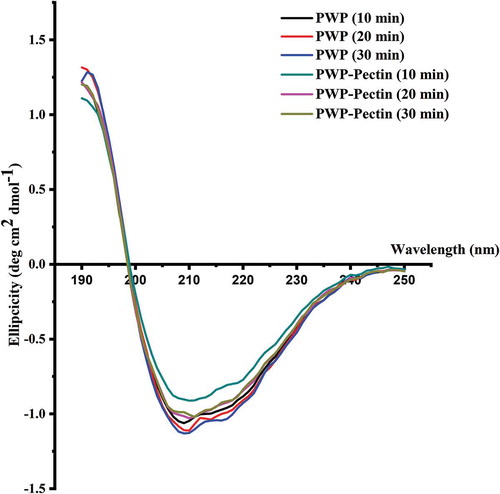
All samples showed a lower board depression with a minimum plateau from 200 to 240 nm, indicating the α-helix and β-sheet structure. Native globulins are generally characterized by a high content of β-sheet and low content of α-helix (Antonov & Takahiro, Citation2009). Thermal treatment resulted in a loss of α-helix structure and an increase in β-sheet (Zhang, Zhang, Lin, & Vardhanabhuti, Citation2012). As expected, heated WP showed low α-helix content of 3.7–3.8% and high β-sheet structures content of 39.7–40.0% ().
ALM pectin slightly decreased the α-helix and β-sheet structure of polymerized WPs by 0.1–0.4%. The secondary structure of protein is mainly maintained by hydrogen bonding. Carboxylic acid (‒COOH) and amide (NH2) in the ALM pectin may have the possibility to form hydrogen bonds with WP, resulting changes in the secondary structure content. However, the little effect of ALM on the α-helix and β-sheet content may suggest that ALM pectin interacts with WP mainly through electrostatic forces.
4. Conclusions
Presence of amidated LM pectin (0.3%) during thermal aggregation resulted in alteration of polymerized WP properties such as increased mean diameter and zeta potential, decreased IEP and surface hydrophobicity. The effects may be due to the electrostatic interactions between the WP and pectin. No considerable effect on the secondary structure of polymerized WPs was observed when heating together with pectin.
Acknowledgments
The financial support for this project was provided by the Ministry of Science and Technology of China (Project # 2013BAD18B07).
Disclosure statement
No potential conflict of interest was reported by the authors.
Additional information
Funding
References
- And, B. V., & Foegeding, E. A. (1999). Rheological properties and characterization of polymerized whey protein isolates. Journal of Agricultural & Food Chemistry, 47(9), 3649–3655.
- Antonov, Y. A., & Takahiro, S. (2009). Macromolecular complexes of the main storage protein of Vicia faba seeds with sulfated polysaccharide. Food Hydrocolloids, 23(3), 996–1006.
- Barrera, A. M., Ramirez, J. A., Gonzalezcabriales, J. J., & Vazquez, M. (2002). Effect of pectins on the gelling properties of surimi from silver carp. Food Hydrocolloids, 16(5), 441–447.
- Beaulieu, M., Corredig, M., Turgeon, S. L., Wicker, L., & Doublier, J.-L. (2005). The formation of heat-induced protein aggregates in whey protein/pectin mixtures studied by size exclusion chromatography coupled with multi-angle laser light scattering detection. Food Hydrocolloids, 19(5), 803–812.
- Bounous, G., Batist, G., & Gold, P. (1991). Whey proteins in cancer prevention. Cancer Letters, 57(2), 91–94.
- Capel, F., Nicolai, T., Durand, D., Boulenguer, P., & Langendorff, V. (2006). Calcium and acid induced gelation of (amidated) low methoxyl pectin. Food Hydrocolloids, 20(6), 901–907.
- Chen, B., Li, H., Ding, Y., & Suo, H. (2012). Formation and microstructural characterization of whey protein isolate/beet pectin coacervations by laccase catalyzed cross-linking. LWT - Food Science and Technology, 47(1), 31–38.
- Cheng, J., Xie, S., Yin, Y., Feng, X., Wang, S., Guo, M., Ni, C. (2017). Physiochemical, texture properties, and the microstructure of set yogurt using whey protein-sodium tripolyphosphate aggregates as thickening agents. Journal of the Science of Food and Agriculture, 97(9), 2819–2825.
- Dai, Q., Zhu, X., Abbas, S., Karangwa, E., Zhang, X., Xia, S., Feng, B. & Jia, C., (2015). Stable nanoparticles prepared by heating electrostatic complexes of whey protein isolate-dextran conjugate and chondroitin sulfate. Journal of Agricultural and Food Chemistry, 63(16), 4179–4189.
- Fu, J. T., & Rao, M. A. (2001). Rheology and structure development during gelation of low-methoxyl pectin gels: The effect of sucrose. Food Hydrocolloids, 15(1), 93–100.
- Fuente, M. A. D. L., Singh, H., & Hemar, Y. (2002). Recent advances in the characterisation of heat-induced aggregates and intermediates of whey proteins. Trends in Food Science & Technology, 13(8), 262–274.
- Girard, M., Turgeon, S. L., & Gauthier, S. F. (2002). Interbiopolymer complexing between β-lactoglobulin and low- and high-methylated pectin measured by potentiometric titration and ultrafiltration. Food Hydrocolloids, 16(6), 585–591.
- Gosal, W. S., & Ross-Murphy, S. B. (2000). Globular protein gelation. Current Opinion in Colloid & Interface Science, 5(3–4), 188–194.
- Guyomarc’H, F., Renan, M., Chatriot, M., Gamerre, V., & Famelart, M. (2007). Acid gelation properties of heated skim milk as a result of enzymatically induced changes in the micelle/serum distribution of the whey protein/κ-casein aggregates. Journal of Agricultural & Food Chemistry, 55(26), 10986–10993.
- Haque, M. A., Aldred, P., Chen, J., Barrow, C. J., & Adhikari, B. (2013). Comparative study of denaturation of whey protein isolate (WPI) in convective air drying and isothermal heat treatment processes. Food Chemistry, 141(2), 702–711.
- Imeson, A., & Imeson, A. (2009). Food stabilisers thickeners & gelling agents. Food Stabilisers, Thickeners and Gelling Agents, 40(1), 464–466.
- Jones, O. G., & Mcclements, D. J. (2011). Recent progress in biopolymer nanoparticle and microparticle formation by heat-treating electrostatic protein-polysaccharide complexes. Advances in Colloid & Interface Science, 167(1–2), 49–62.
- Kehoe, J. J., Wang, L., Morris, E. R., & Brodkorb, A. (2011). Formation of non-native β-lactoglobulin during heat-induced denaturation. Food Biophysics, 6(4), 487–496.
- Krzeminski, A., Prell, K. A., Weiss, J., & Hinrichs, J. (2014). Environmental response of pectin-stabilized whey protein aggregates. Food Hydrocolloids, 35(35), 332–340.
- Li, K., & Zhong, Q. (2016). Aggregation and gelation properties of preheated whey protein and pectin mixtures at pH 1.0–4.0. Food Hydrocolloids, 60, 11–20.
- Liu, J., Shim, Y. Y., Shen, J., Wang, Y., & Reaney, M. J. T. (2017). Whey protein isolate and flaxseed (Linum usitatissimum L.) gum electrostatic coacervates: Turbidity and rheology. Food Hydrocolloids, 64, 18–27.
- Martins, J. T., Santos, S. F., Bourbon, A. I., Pinheiro, A. C., González-Fernández, Á., Pastrana, L. M., Cerqueira, M. A., & Vicente, A. A (2016). Lactoferrin-based nanoparticles as a vehicle for iron in food applications – Development and release profile. Food Research International, 90, 16–24.
- May, C. D. (1990). Industrial pectins: Sources, production and applications. Carbohydrate Polymers, 12(1), 79–99.
- Mezaize, S., Chevallier, S., Le Bail, A., & de Lamballerie, M. (2009). Optimization of gluten-free formulations for French-style breads. Journal of Food Science, 74(3), E140–146.
- Mounsey, J. S., O’Kennedy, B. T., Fenelon, M. A., & Brodkorb, A. (2008). The effect of heating on β-lactoglobulin-chitosan mixtures as influenced by pH and ionic strength. Food Hydrocolloids, 22(1), 65–73.
- Neirynck, J., Kowalski, A. S., Carrara, A., Genouw, G., Berghmans, P., & Ceulemans, R. (2007). Fluxes of oxidised and reduced nitrogen above a mixed coniferous forest exposed to various nitrogen emission sources. Environmental Pollution (Barking, Essex : 1987), 149(1), 31–43.
- Neirynck, N., Van Der Meeren, P., Lukaszewicz-Lausecker, M., Cocquyt, J., Verbeken, D., & Dewettinck, K. (2007). Influence of pH and biopolymer ratio on whey protein–Pectin interactions in aqueous solutions and in O/W emulsions. Colloids and Surfaces A: Physicochemical and Engineering Aspects, 298(1–2), 99–107.
- Nicolai, T., Britten, M., & Schmitt, C. (2011). β-Lactoglobulin and WPI aggregates: Formation, structure and applications. Food Hydrocolloids, 25(8), 1945–1962.
- Oweng, J., Erica, D., & Davidjulian, M. C. (2009). Formation of biopolymer particles by thermal treatment of β-lactoglobulin-pectin complexes. Food Hydrocolloids, 23(5), 1312–1321.
- Qi, D., Wang, P., Chen, C., Guo, S., & Wang, X. (2016). Polymerization of modified diaspirin cross-linked hemoglobin (DCLHb) with 1,6-bismaleimic-hexane. Artif Cells Nanomed Biotechnol, 44(4), 1069–1074.
- Ramirez, J. A., RodríGuez, N. R., Uresti, R. M., Velazquez, G., & VáZquez, M. (2007). Fiber-rich functional fish food from striped mullet (Mugil cephalus) using amidated low methoxyl pectin. Food Hydrocolloids, 21(4), 527–536.
- Ru, Q., Wang, Y., Lee, J., Ding, Y., & Huang, Q. (2012). Turbidity and rheological properties of bovine serum albumin/pectin coacervates: Effect of salt concentration and initial protein/polysaccharide ratio. Carbohydrate Polymers, 88(3), 838–846.
- Ryan, K. N., & Foegeding, E. A. (2015). Formation of soluble whey protein aggregates and their stability in beverages. Food Hydrocolloids, 43(43), 265–274.
- Saint-Sauveur, D., Gauthier, S. F., Boutin, Y., & Montoni, A. (2008). Immunomodulating properties of a whey protein isolate, its enzymatic digest and peptide fractions. International Dairy Journal, 18(3), 260–270.
- Silva, M. R, Rodrigues, D. F, Lana, F. D. C, Silva, V. D. M, Morais, H. A, & Silvestre, M. P. C. (2010). Peptide profile of enzymatic hydrolysates from whey protein concentrate obtained by action of pancreatin and papain. Food and Nutrition, 35(3), 97-114.
- Sootsman, J. R., Kong, H., Uher, C., D’Angelo, J. J., Wu, C. I., Hogan, T. P., Caillat, T., & Kanatzidis, M. G. (2008). Large enhancements in the thermoelectric power factor of bulk PbTe at high temperature by synergistic nanostructuring. Angewandte Chemie (International Ed. In English), 47(45), 8618–8622.
- Sun, W. W., Yu, S. J., Yang, X. Q., Wang, J. M., Zhang, J. B., Zhang, Y., Zhang, Y., & Zheng, E. L. (2011). Study on the rheological properties of heat-induced whey protein isolate–Dextran conjugate gel. Food Research International, 44(10), 3259–3263.
- Sutariya, S., & Patel, H. (2017). Effect of hydrogen peroxide on improving the heat stability of whey protein isolate solutions. Food Chemistry, 223, 114–120.
- Takada, Y., Matsuyama, H., Kato, K., Kobayashi, N., Yamamura, J. I., Yahiro, M., & Ace, S. (1997). Milk whey protein enhances the bone breaking force in ovariectomized rats. Nutrition Research, 17(11), 1709–1720.
- Tarhan, O., Spotti, M. J., Schaffter, S., Corvalan, C. M., & Campanella, O. H. (2016). Rheological and structural characterization of whey protein gelation induced by enzymatic hydrolysis. Food Hydrocolloids, 61, 211–220.
- Tavares, C., & Jald, S. (2003). Rheology of galactomannan–Whey protein mixed systems. International Dairy Journal, 13(8), 699–706.
- Uresti, R. M., López-Arias, N., Ramírez, J. A., & Vázquez, M. (2003). Effect of Amidated Low Methoxyl Pectin on the Mechanical Properties and Colour Attributes of Fish Mince. Food Technology & Biotechnology, 41(2), 131–136.
- Vá, R. K., & Synytsya, A. (2009). Characterisation of whey proteins-pectin interaction in relation to emulsifying properties of whey proteins. Czech Journal of Food Sciences, 27(6), s4–s8.
- Valencia-Flores, D. C., Hernandez-Herrero, M., Guamis, B., & Ferragut, V. (2013). Comparing the effects of ultra-high-pressure homogenization and conventional thermal treatments on the microbiological, physical, and chemical quality of almond beverages. Journal of Food Science, 78(2), E199–205.
- Wang, C., Gao, F., Zhang, T., Wang, Y., & Guo, M. (2015). Physiochemical, textural, sensory properties and probiotic survivability of Chinese Laosuan Nai (protein‐fortified set yoghurt) using polymerised whey protein as a co-thickening agent. International Journal Dairy Technological, 68(2), 261–269.
- Wang, C., Zheng, H., Liu, T., Wang, D., & Guo, M. (2017). Physiochemical properties and probiotic survivability of symbiotic corn-based yogurt-like product. Journal of Food Science, 82(9), 2142–2150.
- Wang, H., Wang, C., Wang, M., & Guo, M. (2017). Chemical, physiochemical, and microstructural properties, and probiotic survivability of fermented goat milk using polymerized whey protein and starter culture kefir mild 01. Journal of Food Science, 82(11), 2650–2658.
- Wijaya, W., Meeren, P. V. D., & Patel, A. R. (2016). Cold-set gelation of whey protein isolate and low-methoxyl pectin at low pH. Food Hydrocolloids, 65, 35–45.
- Zhang, S., Hsieh, F. H., & Vardhanabhuti, B. (2014). Acid-induced gelation properties of heated whey protein–Pectin soluble complex (Part I): Effect of initial pH. Food Hydrocolloids, 36(5), 76–84.
- Zhang, S., & Vardhanabhuti, B. (2014). Acid-induced gelation properties of heated whey protein−pectin soluble complex (Part II): Effect of charge density of pectin. Food Hydrocolloids, 39(2), 95–103.
- Zhang, S., Zhang, Z., Lin, M., & Vardhanabhuti, B. (2012). Raman spectroscopic characterization of structural changes in heated whey protein isolate upon soluble complex formation with pectin at near neutral pH. Journal of Agricultural & Food Chemistry, 60(48), 12029.