ABSTRACT
Antioxidant capacities of hydrophilic (phenolic compounds; H-MPE) and lipophilic (carotenoids; L-MPE) antioxidants from mango peel by ABTS and DPPH assays were determined. The ability of H-MPE and L-MPE to inhibit fish oil peroxidation by the formation of malondialdehyde (MDA) was measured and compared against synthetic antioxidant butylated hydroxytoluene (BHT) to study their antioxidant potential in the stabilization of omega-3 polyunsaturated fatty acids contained in the fish oil. H-MPE and L-MPE showed significantly higher antioxidant capacity than BHT (P < 0.05) by ABTS and DPPH assays. In addition, H-MPE significantly inhibited fish oil peroxidation compared with the control without antioxidant (P < 0.05), while L-MPE showed a prooxidant effect. On the other hand, H-MPE at 200 mg/L showed a similar effect than that of BHT on the prevention of fish oil peroxidation. Therefore, H-MPE could be used as a new alternative to BHT to prevent fish oil peroxidation.
RESUMEN
Las capacidades antioxidantes de compuestos hidrofílicos (compuestos fenólicos; H-MPE) y lipofílicos (carotenoides; L-MPE) de la cáscara de mango fue determinada por los ensayos ABTS y DPPH. La capacidad de H-MPE y L-MPE para inhibir la peroxidación en el aceite de pescado fue analizada por la formación de malonaldehído (MDA) y comparada contra el antioxidante sintético butil hidroxitolueno (BHT), para estudiar su potencial antioxidante en la estabilización de ácidos grasos poliinsaturados de la serie omega 3 presentes en el aceite de pescado. H-MPE y L-MPE mostraron mayor capacidad antioxidante que el BHT (P<0.05) mediante los ensayos ABTS y DPPH. Además, H-MPE inhibió significativamente la peroxidación del aceite de pescado en comparación con el control sin antioxidante (P<0.05), mientras que L-MPE mostró un efecto prooxidante. Contrariamente, la concentración de 200 mg/L de H-MPE mostró un efecto similar al BHT en la prevención de la peroxidación del aceite de pescado. Por lo tanto, H-MPE podría ser usado como una nueva alternativa del BHT para prevenir la peroxidación en el aceite de pescado.
1. Introduction
Fish oil is the main source of long-chain omega-3 polyunsaturated fatty acids (ω3 PUFAs) such as eicosapentaenoic acid (EPA; 20:5) and docosahexaenoic acid (DHA; 22:6) (Srigley & Rader, Citation2014), which are related with the prevention of the development of cardiovascular and inflammatory diseases (Delgado-Lista, Perez-Martinez, Lopez-Miranda, & Perez-Jimenez, Citation2012; Wall, Ross, Fitzgerald, & Stanton, Citation2010). Therefore, fish oil is commonly used as a dietary supplement. Paradoxically, the chemical characteristics of the fatty acids composition of fish oil could pose a problem. Lipid quality may be reduced by a degenerative oxidation process which lipid undergoes. Particularly, ω3 PUFAs are highly susceptible to lipid peroxidation by the action of atmospheric oxygen, light, and temperature, due to their high degree of unsaturation (Sekhon-Loodu, Warnakulasuriya, Rupasinghe, & Shahidi, Citation2013). Lipid peroxidation conduces to the formation of hydroperoxides, aldehydes, ketones, alcohols, alkanes, and alkenes, which are related to losses of nutritional values (e.g. decrease of ω3 PUFAs content), production of unpalatable flavor, and odor, shortening of shelf-life of fish oil. Likewise, lipid peroxidation can cause the formation of free radicals which may exert adverse effects on human health (Tao, Citation2015). In addition, due to the above, the synthetic antioxidant butylated hydroxytoluene (BHT) has been used for a long time to delay fish oil peroxidation rate by the oil industry. Even though the use of this synthetic antioxidant as feed additive has received increasing attention from a food safety perspective. Therefore, the use of natural antioxidants as an alternative source is recommended. The food industry has focused its attention on the use of plant extracts as natural antioxidants in preventing fish oil peroxidation. These include extracts from potato peel (Habeebullah, Nielsen, & Jacobsen, Citation2010), apple peel (Sekhon-Loodu et al., Citation2013), and pomegranate peel (Topuz et al., Citation2015), all of which have antioxidant activities comparable to that of BHT.
Mango has been reported to contain antioxidants such as carotenoids, phenolic compounds (PCs), vitamin A, and vitamin C with high antioxidant capacity (Manthey & Perkins-Veazie, Citation2009; Palafox-Carlos, Yahia, & González-Aguilar, Citation2012; Rymbai, Srivastav, Sharma, Patel, & Singh, Citation2013). Mango (Mangifera indica L. cv. Ataulfo) is used by the juice industry, generating a large amount of by-product such as peels and seed. Peels contain higher amounts of bioactive compounds such as PCs (hydrophilic antioxidants) and carotenoids (lipophilic antioxidants) (de Ancos et al., Citation2018; Sáyago-Ayerdi et al., Citation2013). Recently, we found that the cell wall bound-PCs from mango peel, after digestion have intestinal permeability similar to pure PCs (Pacheco-Ordaz, Antunes-Ricardo, Gutiérrez-Uribe, & González-Aguilar, Citation2018). Nowadays, there are multiple in vitro and in vivo studies reporting the anti-inflammatory, anti-cancer and anti-diabetic potential of mango peel antioxidants (Kim et al., Citation2016; Shah, Patel, Patel, & Parmar, Citation2010). In conjunction, these findings can be applied in the development of nutraceuticals using this important by-product from the mango processing industry. Even different studies have been reported the PCs and carotenoids present in mango peel (Pacheco-Ordaz et al., Citation2018; Palafox-Carlos et al., Citation2012). However, the antioxidant effect and possible mechanisms of hydrophilic and lipophilic fraction against lipid peroxidation have not been elucidated completely, and their possible effectiveness to reduce peroxidation in foods could be of great interest in the food industry. Therefore, the aim of this study was to analyze antioxidant mechanisms of PCs and lipophilic antioxidants from mango peels and evaluate their antioxidant potential as food additive to prevent fish oil lipid peroxidation as compared to the synthetic antioxidant BHT.
2. Materials and methods
2.1. Materials
Fish oil was donated for Mazindustrial SA de CV (Mazatlán, Sinaloa, México), while mango peels (Mangifera indica L. cv. Ataulfo) were collected from Pure Mango SA de CV (Escuinapa, Sinaloa, México). Fresh mango peels were dried at 50°C during 15 h in oven and ground to a particle size of 0.25 mm using a 50,703 model hammer mill (California Pellet Mill laboratory Mill Champion, Waterloo, IA, USA). Mango peel powder was stored at −20°C until analysis.
2.2. Extraction of lipophilic antioxidants from mango peel
Carotenoids were obtained following the methodology described by the AOAC (method 43.015). Mango peel powder (3 g) was homogenized in 100 mL of hexane: acetone (3:2, v/v) and 0.1 g MgCO3. The extract was filtered (Whatman No.1) under vacuum and the residue was mixed with acetone (25 mL, twice), and finally, with 25 mL of hexane until the residue was colorless. The extract was then transferred to a separating funnel and mixed with distilled water (100 mL, five times) to remove acetone. The upper layer containing carotenoids was placed in 100 mL volumetric flask containing 9 mL acetone and diluted to volume with hexane. Total carotenoids (TC) content were determined by spectrophotometry at 446 nm using β-carotene as standard, and the results were expressed as µg β-carotene equivalents (µg βE)/g of DW. Dilutions of these solutions with concentrations of 100 and 200 mg/L βE of the lipophilic antioxidants from mango peel (L-MPE) were prepared.
2.3. Extraction of hydrophilic antioxidants from mango peel
PCs were extracted according to Sekhon-Loodu et al. (Citation2013) with minor modifications. Mango peel powder (3 g) was homogenized in 100 mL of 70% ethanol, sonicated three times for 15 min in a 3510 model ultrasonic bath (Branson, Wethersfield, CT) and centrifuged (15,000 rpm, 15 min, 4°C) in an Allegra X-30R model A99470 centrifuge (Beckman Coulter, Germany). The procedure was repeated two times and was done in triplicate. Finally, extracts were concentrated to 10 mL using a rotavapor R-114 (Büchi Labortechnik AG, Flawil, Switzerland). Total phenolic content (TPC) was measured using the Folin-Ciocalteu method described by Montreau (Citation1972). Aliquots of 0.5 mL of the extract were mixed with 0.5 mL of Folin-Ciocalteu reagent for 3 min. Subsequently, the solution was mixed with 10 mL of Na2CO3 (7.5%, w/v) and 14 mL of water. The solution was placed in the dark at room temperature for 1 h. Absorbance was read at 734 in a UV-Vis spectrophotometer (Hewlett Packard, Texas, United States). A standard curve generated from gallic acid was used to calculate the TPC. Results were expressed as mg of gallic acid equivalents (mg GAE)/g of dry weight (DW). Dilutions of these solutions with concentrations of 100 and 200 mg/L GAE from mango peel (H-MPE) were prepared.
2.4. Analysis of PCs by UPLC ESI-Q-TOF/MS/MS
Identification of PCs was determined through Ultra-Performance Liquid Chromatography (UPLC) using ACQUITY UPLC; H-Class system (Waters, Milford, Mass., USA) coupled to a G2 XS Quadrupole-Time-of-Flight (Q-Tof) mass spectrometer (Agilent, Santa Clara, CA) equipped with electrospray ionization (ESI). Briefly, PCs were separated by UPLC to 40°C with a column ACQUITY BEH C18 (1.7 µm, 3.0 x 100 mm) using a mobile phase composed of 0.1% formic acid (A) and acetonitrile (B) at a flow rate of 0.3 mL/min. The gradient procedure was as follows: 0 min, 95% (A); 2.5 min, 75% (A); 3 min, 50% (A); 3.5 min, 35% (A); 5 min, 5% (A); 6 min, 95% (A); 7 min, 95% (A). An electrospray source in negative mode was used to collect mass spectra under the following conditions: nitrogen gas; desolvation temperature, 350°C; desolvation gas, 13.3 L/min; capillary voltage, 1500 V; and fragmentor voltage, 10 V.
2.5. Antioxidant capacity assay
2.5.1. ABTS radical cation scavenging capacity
ABTS radical cation (ABTS•+) scavenging capacity was measured according to Re et al. (Citation1999). The generation of the radical cation ABTS•+ was prepared with 5 mL of 7 mM ABTS [2,2-azinobis(3-ethylbenzo-thiazoline-6-sulfonate)] and 88 µL of 2.45 mM of potassium persulfate, allowing the mixture to stand in the dark at 20°C for 16 h. The reaction was adjusted to an absorbance of 0.7 ± 0.02 at 754 nm. Each sample (33 µL) was mixed with 967 µL of ABTS•+ and kept in the dark at room temperature for 6 min. Trolox was used as a standard to calculate the antioxidant capacity of different concentrations of BHT, H-MPE, and L-MPE. Absorbance was read at 754 nm, and results were expressed as mg Trolox equivalents (mg ET)/L of extract.
2.5.2. DPPH radical scavenging capacity
DPPH radical (DPPH•) scavenging capacity was determined according to Brand-Williams, Cuvelier, and Berset (Citation1995) modified by Gayosso-García, Yahia, and González-Aguilar (Citation2013). The working solution was prepared freshly by mixing 2.5 mg of DPPH (2, 2-diphenyl-1-picrylhydrazyl) with 100 mL of absolute methanol. The solution was adjusted to an absorbance of 0.7 ± 0.02 at 516 nm. DPPH• solution (933 μL) was placed in a test tube and 67 μL of sample was added. The mixture was kept in the dark for 30 min. Absorbance was read at 516 nm, and results were expressed as mg ET/L of extract. Trolox was used as a standard to calculate the antioxidant capacity of different concentrations of BHT, H-MPE and L-MPE.
2.6. Determination of lipid oxidation in fish oil
L-MPE solvents were removed completely using nitrogen flow. 100 and 200 mg/L L-MPE dried compounds were dissolved with 1 mL of fish oil and vortexed by 1 min. H-MPE was rotaevaporated at 37°C to remove the ethanol and then, they were freeze-dried. 100 and 200 mg/L H-MPE dried compounds were dissolved in 200 µL of ethanol and mixed with 1 mL of fish oil and vortexed by 1 min. The ethanol was removed completely under a nitrogen flow. Concentrations used were selected according to legal restrictions imposed to BHT (100 and 200 mg/L) by the CODEX Alimentarius. BHT and control (without antioxidant) were used to compare the efficacy of antioxidants from mango peel. 100 and 200 mg/L BHT were dissolved with 1 mL of fish oil and vortexed by 1 min. The mixtures were incubated in an oven at 30°C, and peroxidation of fish oil was measured every 2 days for 14 days. Each treatment was performed in triplicate.
The lipid peroxidation of fish oil was evaluated by the formation of malondialdehyde (MDA) using an assay described by Solé, Potrykus, Fernández-Díaz, and Blasco (Citation2004) with some modifications. Briefly, 200 µL of fish oil were mixed with 1,300 µL of methyl-2-phenylindole (10.3 mM) in methanol:acetonitrile (1:3; v/v), 200 µL of water and 300 µL of 37% HCl. This mixture was incubated at 45°C for 40 min, cooled, and centrifuged at 13,000 rpm for 15 min at 4°C. Absorbance was read at 586 nm, and the amount of peroxidized lipids [mg malondialdehyde (MDA)/kg] was calculated using 1,1,3,3-tetraethoxypropane for the preparation of a standard curve of MDA.
2.7. Statistical analyses
Date were evaluated for normality (Kolmogorov–Smirnov test) and homogeneity of variance (Levene´s test) prior to the statistical analysis. Results of antioxidant capacity were analyzed using two-way analysis of variance (ANOVA). MDA data of fish oil was subjected to a two-way ANOVA with a block design. The post hoc Tukey’s test was conducted when significant differences were found (P < 0.05). All statistical analyzes were conducted using the statistical software SAS version 9.0 (SAS Institute Inc. Cary, NC, USA).
3. Results and discussion
3.1. Total PC and carotenoid contents from mango peel
The antioxidant properties of mango peel have been attributed to its bioactive compounds, being the carotenoids and PCs the most important (Pacheco-Ordaz et al., Citation2018; Palafox-Carlos et al., Citation2012). Mango peel had a TPC of 45.53 ± 0.57 mg GAE/g DW. This result was lower to that reported by García-Magaña, García, Bello-Pérez, Sáyago-Ayerdi, and de Oca (Citation2013). Probably such differences could be attributed to environmental conditions, pre- and postharvest conditions, as well as the use of different solvents and extraction methods (Kalt, Citation2005; Tomsone, Kruma, & Galoburda, Citation2012). Regarding CT, mango peel presented 132.14 µg βE/g DW. This result was low compared to those reported by Ajila, Bhat, and Rao (Citation2007) (1,400–3,945 µg EC/g DW) and Ajila, Naidu, Bhat, and Rao (Citation2007) (194–436 µg βE/g DW). These differences could be attributed to the drying method conditions used in this study (50°C, 24 h) that influenced the carotenoids content. Previous studies indicated thermal drying methods can cause losses of carotenoids and PCs in fruits, due to cell wall destruction, exposure of bioactive compounds to heat, light, and oxygen during drying (Chuyen, Roach, Golding, Parks, & Nguyen, Citation2017; İzli, Yıldız, Ünal, Işık, & Uylaşer, Citation2014). However, drying methods using ovens appear to be more convenient for the industry; it reduces the production cost of mango peel powder as an antioxidant source to prevent lipid peroxidation in fish oil.
3.2. Identification of PCs of mango peel extract
Eight PCs were identified () five of which were phenolic acids, two flavonoids, and one polyphenol xanthone. Gallic acid (m/z 169.01), 2-hydroxicinnamic acid (m/z 163.038), mangiferin (m/z 421.089), and quercetin (m/z 301.035) were identified by comparing the [M-H]- ion and fragment ions with standards. Methyl gallate (m/z, 183.027), ethyl gallate (m/z, 197.041), and isoquercitrin (m/z, 463.085) were compared with literature. While protocatechuic acid (m/z, 153.014) was confirmed with database (MassBank of North America). Our results agree with those reported by Velderrain-Rodríguez et al., Citation2015 which previously identified gallic acid, protocatechuic acid, and 2-hydroxycinnamic acid as major PCs in mango peel cv. Ataulfo. Additionally, Pacheco-Ordaz et al. (Citation2018) and Dorta, González, Lobo, Sánchez-Moreno, and de Ancos (Citation2014) have reported the presence of methyl gallate, ethyl gallate, quercetin, isoquercitrin, and mangiferin, which is according with results of this study.
Table 1. PCs detected in mango peel cv. Ataulfo extract by UPLC-ESI Q-Tof-MS/MS.
Tabla 1. PCs identificados en extracto de cáscara de mango cv. Ataulfo.
3.2. ABTS and DPPH radical scavenging capacity of L-MPE and H-MPE
Antioxidant capacity of L-MPE and H-MPE from mango peel is highly relevant because it provides information about their radical scavenging ability in a specific media and time of reaction. Using ABTS•+, the scavenging ability of synthetic antioxidant BHT, L-MPE, and H-MPE were measured at different concentrations. Higher concentrations exhibited a higher antioxidant capacity for all antioxidants (). L-MPE exhibited the highest ABTS•+ scavenging ability (1216.26 ± 15.75 mg TE/L) (P < 0.05) which was about 13-fold higher than BHT (95.86 ± 0.59 mg TE/L). H-MPE also presented significantly higher ABTS•+ scavenging ability (973.36 ± 17.75 mg TE/L) than BHT. Similarly, when DPPH• scavenging ability was evaluated, antioxidant capacity also increased with antioxidant concentration (P < 0.05) (), and L-MPE and H-MPE exhibited significantly higher DPPH• scavenging abilities 1131.51 ± 11.78 and 797.72 ± 7.63 mg TE/L, respectively) than BHT (74.47 ± 6.48 mg TE/L).
Figure 1. ABTS•+ scavenging capacity of phenolic compounds (H-MPE), carotenoids (L-MPE) and BHT at 100 and 200 mg/L. Error bars represent the standard deviation (n = 3). Letters indicate significant differences (p < 0.05) among types of antioxidants used at the same concentration. Asterisks indicate significant differences (p < 0.05) between concentrations used for each type of antioxidant.
Figura 1. Capacidad antirradical ABTS de los compuestos fenólicos (H-MPE), carotenoides (L-MPE) y BHT en 100 y 200 mg/L. Las barras de error representan la desviación estándar (n = 3). Las letras indican diferencias significativas (p < 0.05) entre tipos de antioxidantes usados en la misma concentración. Los asteriscos indican diferencias significativas entre la concentración usada por cada tipo de antioxidante.
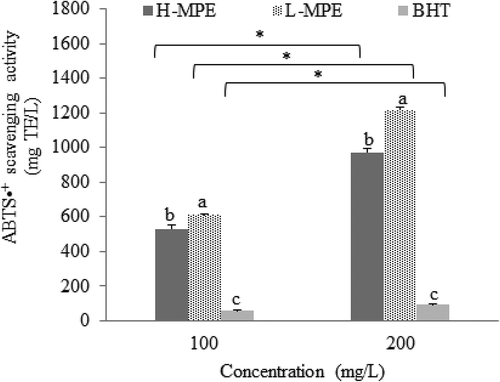
Figure 2. DPPH• scavenging capacity of phenolic compounds (H-MPE), carotenoids (L-MPE) and BHT at 100 and 200 mg/L. Error bars represent the standard deviation (n = 3). Letters indicate significant differences (p < 0.05) among types of antioxidants used at the same concentration. Asterisks indicate significant differences (p < 0.05) between concentrations used for each type of antioxidant.
Figura 2. Capacidad antirradical DPPH de los compuestos fenólicos (H-MPE), carotenoides (L-MPE) y BHT en 100 y 200 mg/L. Las barras de error representan la desviación estándar (n = 3). Las letras indican diferencias significativas (p < 0.05) entre tipos de antioxidantes usados en la misma concentración. Los asteriscos indican diferencias significativas entre la concentración usada por cada tipo de antioxidante.
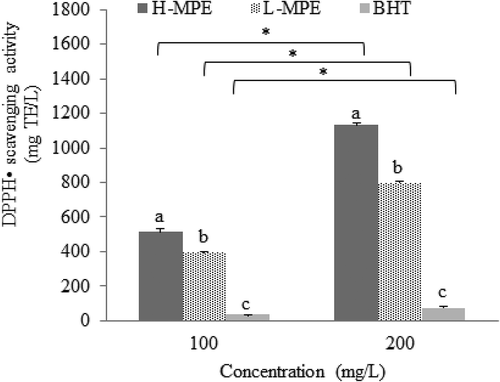
The results of antioxidant capacity assays suggest that L-MPE and H-MPE react quickly with free radicals ABTS•+ or DPPH• through the donation of hydrogen atom compared to BHT. The high antioxidant capacity of L-MPE might be attributed to the presence of carotenoids which react quickly with free radicals to produce radical adducts with a resonance stabilized carbon-centered radical (Ornelas-Paz, Yahia, Gardea, & Failla, Citation2009; Terao, Citation1989) due to their conjugated double bonds and the presence of ring structures at the end of the polyenes (Stahl & Sies, Citation2003). All-trans-β-carotene, all-trans-violaxanthin dibutyrate, and 9-cis-violaxanthin dibutyrate are the main carotenoids found in mango cv Ataulfo pulp (Ornelas-Paz et al., Citation2009), while that β-carotene, cis isomers of β-carotene, violaxanthin, lutein, neochrome, and luteoxanthin are main carotenoids identified in mango peel (Ajila, Rao, & Rao, Citation2010; Chen, Tai, & Chen, Citation2004). Gayosso-García et al. (Citation2013) reported that a mix of carotenoids (β-carotene, lycopene, and β-cryptoxanthin) promotes a synergistic effect, increasing overall antioxidant capacity relative to the individual capacity of each carotenoid. Therefore, we suggest that enhanced scavenging ability of L-MPE likely be due to the presence of a mix carotenoids, to their high reactivity with free radicals and the possible synergy among carotenoids.
Additionally, H-MPE also presented higher ABTS•+ and DPPH• scavenging ability than BHT. This effect is attributed to the mix of PCs (phenolic acids: gallic acid, protocatechuic acid, syringic acid, and methyl gallate; flavonoids: quercetin and isoquercitrin; mangiferin) identified in this study. Phenolic acids, flavonoids, and mangiferin are considered to be efficient hydrogen donors due to their characteristic carboxylic group, which is easily ionized to the number and position of the hydroxyl groups and the type of substituent on the aromatic rings (Balasundram, Sundram, & Samman, Citation2006). Other studies have reported that gallic acid reduces from three to six molecules of DPPH•, while BHT reduces around three molecules of DPPH•. Therefore, the scavenging ability of gallic acid is higher than that of BHT (Brand-Williams et al., Citation1995). Another possible reason for the higher antioxidant capacity observed of PCs from MPE compared with that of BHT might be due to its synergistic effect. Antioxidants can chemically reduce each other, acting in synergy to scavenging reactive oxygen species. For example, binary combination of PCs such as myricetin-kaempferol, quercetin-rutin, resveratrol-catechin, gallic-protocatechuic acids, increased their antioxidant capacity (hydrogen atoms transfer and electron transfer), compared with the theoretical sum of its individual antioxidant capacity (Ornelas-Paz et al., Citation2009; Skroza, Mekinić, Svilović, Šimat, & Katalinić, Citation2015). About, we suggested that the mix of PCs present in MPE exerted a synergic effect which was reflected in its high antioxidant capacity compared to BHT.
3.3. Inhibition of fish oil peroxidation by H-MPE
The MDA is a secondary product of lipid peroxidation considered as the most abundant aldehyde resulting from hydroperoxides oxidation (primary lipid oxidation product). The MDA formation is of great interest because this compound can adversely affect the PUFAs content (Yun & Surh, Citation2012). Mango peel extracts contain a mix of PCs with high antioxidant capacity, which could counter the effects previously mentioned. Because of that, H-MPE and BHT were added at 100 and 200 mg/L into bulk fish oil and its effect on lipids peroxidation was evaluated. According to the results, MDA content indicated that 100 and 200 mg/L H-MPE significantly inhibited fish oil peroxidation compared to that of control without antioxidant (P < 0.05) (). In general, it was observed MDA content increased after storage time up to 14 days (P < 0.05). Regarding BHT, no significant differences (P < 0.05) between H-MPE and BHT at 200 mg/L was observed. Additionally, the ability of antioxidants to prevent peroxidation of fish oil varied with the type and concentration of antioxidant (P < 0.05). Topuz et al. (Citation2015) reported that PCs of pomegranate peel at a concentration of 1,000 mg/L had a similar effect as BHT in controlling peroxidation of fish oil stored to 60°C for 12 days. However, PCs from potato peel at concentrations lower than 1,600 mg/L did not prevent lipid peroxidation in fish oil stored 55°C with magnetic stirring for 3 days (Habeebullah et al., Citation2010). Sekhon-Loodu et al. (Citation2013) observed that the flavonol-rich fractions of apple peel were better able to inhibit fish oil peroxidation (experimental conditions: 50°C using shaker oven for 3 h) than PCs from crude apple peel extracts. The effectiveness of PCs may be related to their localization in bulk fish oil as well. Yehye et al. (Citation2015) reported that hydrophilic antioxidants (e.g. PCs) delay lipid peroxidation because these antioxidants concentrate at the oil–water interface in bulk oils, which is where most of the lipid peroxidation takes place due to the high concentration of oxygen and prooxidants. On the other hand, the ability of different PCs to prevent lipid oxidation also depends on their mechanism of action, which can include chelation of transition metals, free radicals, and quenching of reactive oxygen species (Bravo, Citation1998). Therefore, we hypothesize that the efficacy of mango peel PCs could arise from a combined effect of their concentration, chemical structures, mechanisms of action, and localization in the bulk oil. We recently reported the synergism of PCs present in mango ‘Ataulfo’ to stabilize different radicals and its effectiveness was in function of its chemical structure and position and OH number (Palafox-Carlos et al., Citation2012; Velderrain-Rodríguez et al., Citation2015).
Figure 3. Effect of different concentrations of phenolic compounds from mango peel extract (H-MPE) and BHT on the malondialdehyde (MDA) content, a lipid peroxidation product in fish oil, when stored at 30°C for 14 days. Error bars represent the standard deviation (n = 3).
Figura 3. Efecto de diferentes concentraciones de compuestos fenólicos de extracto de cáscara de mango (H-MPE) y BHT sobre el contenidode malonaldehído (MDA), un producto de la peroxidación lipídica en aceite de pescado almacenado a 30°C por 14 días. Las barras de error representan la desviación estándar (n = 3).
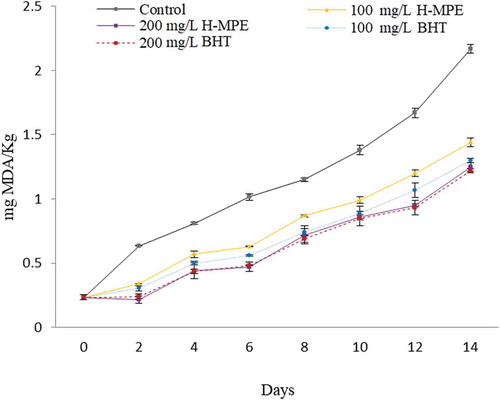
3.4. Inhibition of fish oil peroxidation by L-MPE
L-MPE and BHT were incorporated at two concentrations (100 and 200 mg/L) into the bulk fish oil to compare their effectiveness at preventing fish oil peroxidation. MDA content for all samples increased as the storage time increased over 14 days (P < 0.05) (). The ability of antioxidants to prevent peroxidation in fish oil varied with the type of antioxidant and the concentration used (P < 0.05). At both concentrations tested, L-MPE exhibited higher MDA content (P < 0.05) than BHT or the control, indicating that L-MPE acted as a prooxidant rather than an antioxidant. Jacobsen (Citation2010) reported that the scavenging of peroxyl and alkoxyl radicals is the most important mechanism for lipid peroxidation prevention. In this sense, carotenoids inhibit autoxidation of lipid systems by its capacity to quench peroxyl and alkoxy radicals, through hydrogen donation and delocalization of unpaired electrons in its conjugated polyene, at low oxygen concentration (Choe & Min, Citation2009). However, the prooxidative behavior of carotenoids has been reported as well. For example, purified carotenoids as β-carotene at 20 and 500 mg/L acts as a prooxidant in rapeseed oil and safflower seed oil, respectively (Haila & Heinonen, Citation1994; Henry, Catignani, & Schwartz, Citation1998). This effect results as a consequence of peroxyl and β-carotene reaction, where a β-carotene radical is formed and readily autoxidized which then produces lipid radicals that propagate the chain reaction of lipid peroxidation (Subagio & Morita, Citation2001). In this study, the prooxidant effect observed in fish oil could be due to the presence of β-carotene in MPE, being the major carotenoid identified in both pulp and mango peel (Ajila et al., Citation2010; Chen et al., Citation2004), which could have led to the formation of highly reactive radicals.
Figure 4. Effect of different concentrations of carotenoids from mango peel extract (L-MPE) and BHT on the malondialdehyde (MDA) content, a lipid peroxidation product in fish oil, when stored at 30°C for 14 days. Error bars represent the standard deviation (n = 3).
Figura 4. Efecto de diferentes concentraciones de carotenoides de extracto de cáscara de mango (L-MPE) y BHT sobre el contenidode malonaldehído (MDA), un producto de la peroxidación lipídica en aceite de pescado almacenado a 30°C por 14 días. Las barras de error representan la desviación estándar (n = 3).
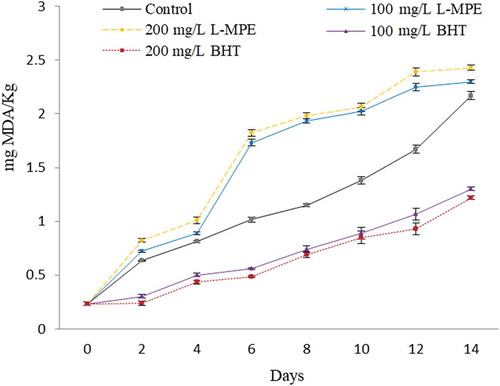
4. Conclusions
H-MPE and L-MPE exhibited high ABTS•+ and DPPH• scavenging. 200 mg/L H-MPE had a strong inhibitory effect on fish oil peroxidation, which showed a similar effect than that of 200 mg/L BHT. However, carotenoids exhibited a prooxidant effect in the concentrations tested. Therefore, results of this study suggest that 200 mg/L H-MPE have the potential to be used as natural antioxidants and a good alternative to BHT in the prevention of fish oil peroxidation which is commonly used as dietary supplement.
Currently, there are lots of bioactive compounds with antioxidant properties, which are considered as safe and can be extracted from low-cost sources such as agro-industrial by-products. In this sense, further investigations are needed to study their application and subsequently the commercialization of these compounds in fish oil, even in foods with a high proportion of polyunsaturated fatty acids.
Acknowledgments
Cynthia E. Lizárraga-Velázquez thanks CONACYT for a doctoral fellowship. We thank Alexis Emus-Medina and Laura Aracely Contreras Angulo for their technical support on the UPLC ESI-Q-TOF/MS/MS analysis.
Disclosure statement
No potential conflict of interest was reported by the authors.
Additional information
Funding
References
- Ajila, C., Bhat, S., & Rao, U. P. (2007). Valuable components of raw and ripe peels from two Indian mango varieties. Food Chemistry, 102(4), 1006–1011.
- Ajila, C., Naidu, K., Bhat, S., & Rao, U. P. (2007). Bioactive compounds and antioxidant potential of mango peel extract. Food Chemistry, 105(3), 982–988.
- Ajila, C., Rao, L. J., & Rao, U. P. (2010). Characterization of bioactive compounds from raw and ripe Mangifera indica L. peel extracts. Food and Chemical Toxicology, 48(12), 3406–3411.
- Balasundram, N., Sundram, K., & Samman, S. (2006). Phenolic compounds in plants and agri-industrial by-products: Antioxidant activity, occurrence, and potential uses. Food Chemistry, 99(1), 191–203.
- Brand-Williams, W., Cuvelier, M.-E., & Berset, C. (1995). Use of a free radical method to evaluate antioxidant activity. LWT-Food Science and Technology, 28(1), 25–30.
- Bravo, L. (1998). Polyphenols: Chemistry, dietary sources, metabolism, and nutritional significance. Nutrition Reviews, 56(11), 317–333.
- Chen, J, Tai, C, & Chen, B. (2004). Improved liquid chromatographic method for determination of carotenoids in taiwanese mango (mangifera indica l.). Journal Of Chromatography a, 1054(1–2), 261–268.
- Choe, E., & Min, D. B. (2009). Mechanisms of antioxidants in the oxidation of foods. Comprehensive Reviews in Food Science and Food Safety, 8(4), 345–358.
- Chuyen, H. V., Roach, P. D., Golding, J. B., Parks, S. E., & Nguyen, M. H. (2017). Effects of four different drying methods on the carotenoid composition and antioxidant capacity of dried Gac peel. Journal of the Science of Food and Agriculture, 97(5), 1656–1662.
- de Ancos, B., Sánchez-Moreno, C., Zacarías, L., Rodrigo, M. J., Ayerdí, S. S., Benítez, F. J. B., … González-Aguilar, G. A. (2018). Effects of two different drying methods (freeze-drying and hot air-drying) on the phenolic and carotenoid profile of ‘Ataulfo’mango by-products. Journal of Food Measurement and Characterization, 12(3), 1–13.
- Delgado-Lista, J., Perez-Martinez, P., Lopez-Miranda, J., & Perez-Jimenez, F. (2012). Long chain omega-3 fatty acids and cardiovascular disease: A systematic review. British Journal of Nutrition, 107(S2), S201–S213.
- Dorta, E., González, M., Lobo, M. G., Sánchez-Moreno, C., & de Ancos, B. (2014). Screening of phenolic compounds in by-product extracts from mangoes (Mangifera indica L.) by HPLC-ESI-QTOF-MS and multivariate analysis for use as a food ingredient. Food Research International, 57, 51–60.
- García-Magaña, M., García, H. S., Bello-Pérez, L. A., Sáyago-Ayerdi, S. G., & de Oca, M.-M.-M. (2013). Functional properties and dietary fiber characterization of mango processing by-products (Mangifera indica L., cv Ataulfo and Tommy Atkins). Plant Foods for Human Nutrition, 68(3), 254–258.
- Gayosso-García, L. E., Yahia, E. M., & González-Aguilar, G. A. (2013). Contribution of major hydrophilic and lipophilic antioxidants from papaya fruit to total antioxidant capacity. Food and Nutrition Sciences, 4(08), 93.
- Habeebullah, S. F. K., Nielsen, N. S., & Jacobsen, C. (2010). Antioxidant activity of potato peel extracts in a fish-rapeseed oil mixture and in oil-in-water emulsions. Journal of the American Oil Chemists’ Society, 87(11), 1319–1332.
- Haila, K., & Heinonen, M. (1994). Action of β-carotene on purified rapeseed oil during light storage. LWT-Food Science and Technology, 27(6), 573–577.
- Henry, L., Catignani, G., & Schwartz, S. (1998). The influence of carotenoids and tocopherols on the stability of safflower seed oil during heat-catalyzed oxidation. Journal of the American Oil Chemists’ Society, 75(10), 1399–1402.
- İzli, N., Yıldız, G., Ünal, H., Işık, E., & Uylaşer, V. (2014). Effect of different drying methods on drying characteristics, colour, total phenolic content and antioxidant capacity of goldenberry (Physalis peruviana L.). International Journal of Food Science & Technology, 49(1), 9–17.
- Jacobsen, C. (2010). Challenges when developing omega-3 enriched foods. Oléagineux, Corps gras, Lipides, 17(4), 251–258.
- Kalt, W. (2005). Effects of production and processing factors on major fruit and vegetable antioxidants. Journal of Food Science, 70(1), R11–R19.
- Kim, H, Banerjee, N, Ivanov, I, Pfent, C. M, Prudhomme, K. R, Bisson, W. H, & Mertens-Talcott, S. U. (2016). Comparison of anti-inflammatory mechanisms of mango (mangifera indica l.). And Pomegranate (Punica Granatum L.) in a Preclinical Model Of Colitis. Molecular Nutrition & Food Research, 60(9), 1912-1923.
- Manthey, J. A., & Perkins-Veazie, P. (2009). Influences of harvest date and location on the levels of β-carotene, ascorbic acid, total phenols, the in vitro antioxidant capacity, and phenolic profiles of five commercial varieties of mango (Mangifera indica L.). Journal of Agricultural and Food Chemistry, 57(22), 10825–10830.
- MassBank of North America. (n.d.) Retrieved from http://mona.fiehnlab.ucdavis.edu/
- Montreau, F. (1972). Sur le dosage des composés phénoliques totaux dans les vins par la methode Folin-Ciocalteau. Connaiss Vigne Vin, 24, 397–404.
- Ornelas-Paz, J., Yahia, E., Gardea, A., & Failla, M. L. (2009). Carotenoid composition in’Ataulfo’mango and their bioavailability and bioconversion to vitamin A. VI International Postharvest Symposium (pp. 877).
- Pacheco-Ordaz, R., Antunes-Ricardo, M., Gutiérrez-Uribe, J. A., & González-Aguilar, G. A. (2018). Intestinal permeability and cellular antioxidant activity of phenolic compounds from mango (Mangifera indica cv. Ataulfo) peels. International Journal of Molecular Sciences, 19(2), 514.
- Palafox-Carlos, H., Yahia, E., & González-Aguilar, G. (2012). Identification and quantification of major phenolic compounds from mango (Mangifera indica, cv. Ataulfo) fruit by HPLC–DAD–MS/MS-ESI and their individual contribution to the antioxidant activity during ripening. Food Chemistry, 135(1), 105–111.
- Re, R., Pellegrini, N., Proteggente, A., Pannala, A., Yang, M., & Rice-Evans, C. (1999). Antioxidant activity applying an improved ABTS radical cation decolorization assay. Free Radical Biology and Medicine, 26(9), 1231–1237.
- Rymbai, H., Srivastav, M., Sharma, R., Patel, C., & Singh, A. (2013). Bio-active compounds in mango (Mangifera indica L.) and their roles in human health and plant defence–A review. The Journal of Horticultural Science and Biotechnology, 88(4), 369–379.
- Sáyago-Ayerdi, S. G., Moreno-Hernández, C. L., Montalvo-González, E., García-Magaña, M. L., de Oca, -M.-M.-M., Torres, J. L., & Pérez-Jiménez, J. (2013). Mexican ‘Ataulfo’mango (Mangifera indica L) as a source of hydrolyzable tannins. Analysis by MALDI-TOF/TOF MS. Food Research International, 51(1), 188–194.
- Sekhon-Loodu, S., Warnakulasuriya, S. N., Rupasinghe, H. P. V., & Shahidi, F. (2013). Antioxidant ability of fractionated apple peel phenolics to inhibit fish oil oxidation. Food Chemistry, 140(1), 189–196.
- Shah, K. A., Patel, M. B., Patel, R. J., & Parmar, P. K. (2010). Mangifera Indica (Mango). Pharmacognosy Reviews, 4(7), 42–48.
- Skroza, D., Mekinić, I. G., Svilović, S., Šimat, V., & Katalinić, V. (2015). Investigation of the potential synergistic effect of resveratrol with other phenolic compounds: A case of binary phenolic mixtures. Journal of Food Composition and Analysis, 38, 13–18.
- Solé, M., Potrykus, J., Fernández-Díaz, C., & Blasco, J. (2004). Variations on stress defences and metallothionein levels in the Senegal sole, Solea senegalensis, during early larval stages. Fish Physiology and Biochemistry, 30(1), 57–66.
- Srigley, C. T., & Rader, J. I. (2014). Content and composition of fatty acids in marine oil omega-3 supplements. Journal of Agricultural and Food Chemistry, 62(29), 7268–7278.
- Stahl, W., & Sies, H. (2003). Antioxidant activity of carotenoids. Molecular Aspects of Medicine, 24(6), 345–351.
- Subagio, A., & Morita, N. (2001). Instability of carotenoids is a reason for their promotion on lipid oxidation. Food Research International, 34(2–3), 183–188.
- Tao, L. (2015). Oxidation of polyunsaturated fatty acids and its impact on food quality and human health. Advancement Food Technological Nutritional Sciences Open Journal, 1(6), 135–142.
- Terao, J. (1989). Antioxidant activity of β‐carotene‐related carotenoids in solution. Lipids, 24(7), 659–661.
- Tomsone, L., Kruma, Z., & Galoburda, R. (2012). Comparison of different solvents and extraction methods for isolation of phenolic compounds from horseradish roots (Armoracia rusticana). World Academy of Science, Engineering and Technology, 64, 903–908.
- Topuz, O. K., Yerlikaya, P., Uçak, İ., Gümüş, B., Büyükbenli, H. A., & Gökoğlu, N. (2015). Influence of pomegranate peel (Punica granatum) extract on lipid oxidation in anchovy fish oil under heat accelerated conditions. Journal of Food Science and Technology, 52(1), 625–632.
- Velderrain-Rodríguez, G. R, Ovando-Martínez, M, Villegas-Ochoa, M, Ayala-Zavala, J. F, Wall-Medrano, A, Álvarez-Parrilla, E, & González-Aguilar, G. A. (2015). Antioxidant capacity and bioaccessibility of synergic mango (cv. Ataulfo) Peel Phenolic Compounds in Edible Coatings Applied to Fresh-cut Papaya. Food and Nutrition Sciences, 6(3), 365.
- Wall, R., Ross, R. P., Fitzgerald, G. F., & Stanton, C. (2010). Fatty acids from fish: The anti-inflammatory potential of long-chain omega-3 fatty acids. Nutrition Reviews, 68(5), 280–289.
- Yehye, W. A., Rahman, N. A., Ariffin, A., Abd Hamid, S. B., Alhadi, A. A., Kadir, F. A., & Yaeghoobi, M. (2015). Understanding the chemistry behind the antioxidant activities of butylated hydroxytoluene (BHT): A review. European Journal of Medicinal Chemistry, 101, 295–312.
- Yun, J.-M., & Surh, J. (2012). Fatty acid composition as a predictor for the oxidation stability of Korean vegetable oils with or without induced oxidative stress. Preventive Nutrition and Food Science, 17(2), 158.