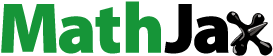
ABSTRACT
The effects of chitosan edible coating incorporated with rosmarinic acid on the quality of half-smooth tongue sole fillets were examined during 18 days of refrigerated storage (4 ± 0.5°C). The samples were analyzed by physicochemical, microbiological, and sensory characteristics. Results showed that samples coated with 1% chitosan containing 30 or 40 mg/L rosmarinic acid significantly lower the total volatile basic nitrogen, K value, free fatty acids, trimethylamine, total viable counts, and H2S-producing bacteria. Low field NMR analysis (LF-NMR) results indicated that chitosan-rosmarinic acid edible coating had positive effects on maintaining the water content and water migration. These results indicated that 1% chitosan coating with 30 mg/L rosmarinic acid was a potential alternative method to improve the quality of half-smooth tongue sole fillets during refrigerated storage.
RESUMEN
En el presente estudio se investigaron los efectos que se producen en la calidad de filetes de lenguado recubiertos con quitosano comestible al que se le agregó ácido rosmarínico. Para ello se examinaron los filetes durante los 18 días que permanecieron almacenados en frío (4 ± 0.5 °C). En este sentido, se analizaron las características fisicoquímicas, microbiológicas y sensoriales de las muestras. Los resultados dan cuenta de que las muestras recubiertas con 1% del quitosano que contiene 30 o 40 mg/L de ácido rosamarínico presentaron niveles de nitrógeno básico volátil total, valor K, ácidos grasos libres, trimetilamina, recuentos viables totales y bacterias productoras de H2S significativamente más bajos. Por otra parte, los resultados obtenidos al realizar el análisis con RMN campo bajo indican que la capa comestible de quitosano y ácido rosmarínico produjo efectos positivos en términos de mantener el contenido de agua y la migración de agua. Asimismo, dieron cuenta de que una capa de 1% del quitosano con 30 mg/L de ácido rosmarínico constituye un posible método alternativo para mejorar la calidad de los filetes de lenguado durante su almacenamiento en frío.
1. Introduction
Fish spoilage is usually dominated by microbial activities and chemical changes, especially the auto-oxidation, enzymatic hydrolysis of the lipid fraction, and tissue enzyme activity. Bioactive edible coating has been adopted to enhance the shelf-life of fresh fish due to its edibility, biodegradability, and barrier properties (Erbay, Dağtekin, Türe, Yeşilsu, & Torres-Giner, Citation2017; Gharibzahedi & Mohammadnabi, Citation2016). Chitosan is a hydrophilic biopolymer with good affinity and compatibility, which is suitable to protect fresh fish fillets (Berizi, Hosseinzadeh, Shekarforoush, & Barbieri, Citation2017; Cotaarriola et al., Citation2017). However, the limited antioxidative activities of pure chitosan coating do not satisfy consumer needs for unsaturated fatty acid-rich foods (Kakaei & Shahbazi, Citation2016). In order to overcome these shortcomings, some antioxidative agents have been incorporated in chitosan-based packaging materials (Feng, Bansal, & Yang, Citation2016; Kakaei & Shahbazi, Citation2016). Rosmarinic acid (RA) is an orthophenylphenol compound with antioxidant effect and occurs abundantly in the rosemary which is the only natural food antioxidant approved by the European Union (Xie, Vanalstyne, Uhlir, & Yang, Citation2017). Rosemary extracts could help preserve the refrigerated and frozen fish and did exhibit antioxidant effects in most cases (Choulitoudi et al., Citation2017; Li, Hu, Liac, Zhu, & Li, Citation2012).
Half-smooth tongue sole (HTS, Cynoglossus Semilaevis) is an economically important euryhaline fish species that is broadly distributed in the East Asia including coastal northern China. From the view of nutritive value, it is rich in high-quality protein, polyunsaturated free fatty acids, essential minerals as well as vitamins and popular with the consumer because of its delicacy (Liu et al., Citation2016). But the wild population of this important fishery species declined sharply year after year, due to human activities such as over-fishing, pollution, causing the relative high price in the market (Sha, Wang, Liu, & Chen, Citation2012). With the development of electronic commerce, the convenient and fast consumption mode is pursued by residents and fresh fish fillets will become the main marketing form of fish products (Yu, Xu et al., Citation2018). However, HTS decompose easily by environmental factors due to nutrient-rich character, and eventually lose edibility. Thus, the present study was aimed to evaluate the quality attribute of HTS fillets during refrigerated storage (4 ± 0.5°C) treated with different chitosan edible coating containing different concentrations of RA and the potential effects of chitosan edible coating incorporated with certain amount of RA on the quality maintenance and extension of shelf life of HTS fillets.
2. Material and method
2.1. Preparation of the edible coating solution
Bioactive edible coating solutions were prepared containing chitosan (Deacetylation degree of 80–95% and average molecular weight of 400 kDa, Sinopharm Chemical Reagent Co., Ltd., Shanghai, China) as the matrix polymer and antimicrobial agent, and rosmarinic acid (RA, Aladdin Biochemistry Technology Co., Ltd., Shanghai, China) with a purity of 97% as the antioxidant agent. Chitosan was used at concentrations of 1% (w/v) in 1% (v/v) acetic acid solution stirring with a magnetic stirrer for 2 h to dissolve completely, and RA at 20, 30 and 40 mg/L in 1% (w/v) chitosan solution. The solution was homogenized with a rotor stator (FA25, Fluko, China) at 10,000 rpm for 60 s. Deaeration was conducted in a vacuum pump for 1 h to remove air bubbles from the solution.
2.2. Preparation and immersion treatment of fillets
Twelve kilograms of fresh Half-smooth tongue sole (HTS, Cynoglossus Semilaevis, weight: 0.75 ± 0.05 kg; length: 40 ± 2 cm) were purchased from the wholesale aquatic market of Luchao Port (Shanghai, China) and immediately transported to the laboratory with ice within 30 min. The fresh HTS had excellent physical properties and the head, bone and skin were removed. Then fresh HTS were washed with tap water and portions of 6 × 4 × 1 cm from the dorsal part of fish fillets were cut. The fillets were randomly divided into six batches, and every group contain 10 fillets. The fillets were immersed in respective freshly prepared bioactive edible coating solution (ratio of coating solution to fillets samples, 2:1) for 30 s, then removed and allowed to drain at 4 °C for approximately 30 min in order to form the coatings. Briefly, the first one group without any treatment was used as control group (CK). C1 and C2 group respectively treated with 1% chitosan coating and 20 mg/L RA coating alone. The fillets of other groups were immersed in composite chitosan edible coating (C3: 1% chitosan + 20 mg/L RA; C4: 1% chitosan + 30 mg/L RA; C5: 1% chitosan + 40 mg/L RA). All the fillets samples were packed in polyethylene bags and stored at 4 °C for subsequent assessments at 2-day interval.
2.3. Weight loss (WL) and cooking loss (CL)
WL was determined as described by Ou et al. (Ou, Teng, El-Nezami, & Wang, Citation2018). Briefly, the percentage losses of the weight of fresh HTS fillets stored special time compared to the initial weight at the beginning of storage were identified as WL.
CL was measured according to Wang et al. (Wang et al., Citation2017) and the weight of fish fillets was recorded before heating and after heating.
2.4. Electrical conductivity (EC) and K value
The method of Feng et al. (Feng et al., Citation2016) was used and the supernatant was measured with an EC meter (Mettler Toledo, Switzerland) at ambient temperature.
Referring to the method as proposed by Su et al. (Su et al., Citation2014), determinations of nucleotides and related compounds were carried out by a RP-HPLC procedure (Waters 2695, Milford, USA). K value was calculated as follows:
2.5. Chemical analysis
Total volatile basic nitrogen (TVB-N) values were determined according to Yuan et al. (Yuan, Zhang, Tang, & Sun, Citation2016) and evaluated by an automatic Kjeldahl nitrogen-determination instrument (Kjeltec 8400, Foss, Denmark).
Thiobarbituric acid (TBA) values were analyzed by Wu et al. (Wu et al., Citation2017). The value was expressed in mg malonaldehyde per kg of sample.
Trimethylamine (TMA) values were determined according to the picric acid colorimetric method (Yu, Xu et al., Citation2018).
Free amino acids (FAAs) were determined according to Yu et al. (Yu, Xu et al., Citation2018) using an automatic amino acid analyzer (Hitachi L-8800, Tokyo, Japan).
Free fatty acids (FFAs) were performed as described by Natseba et al. (Natseba, Lwalinda, Kakura, Muyanja, & Muyonga, Citation2005) and expressed as the percentage of oleic acid.
Myofibrillar protein was prepared as previously described by Jiang and Wu (Jiang & Wu, Citation2018) and the -SH content was measured by total sulfhydryl assay kit (Jiancheng Bioengineering Institute, Nanjing, China).
2.6. Headspace SPME-GC/MS analysis
The volatile compounds were extracted and identified by HS-SPME/GC-MS method as previously documented (Xia, Mei, Yu, & Li, Citation2017). 2 g minced fish fillets were placed in a 20 mL headspace vial and then 5 mL NaH2PO4 (25%, w/v) were added, followed by the addition of internal standard (IS, 10 μL, C17). An automatic headspace sampling system (CTC Analytics, Zwingen, Switzerland) coupled with a DVB/CAR/PDMS 50/30 μm fiber (Supelco, PA, USA) was used to extract and concentrate the headspace volatile compounds for 25 min at 50°C. After extraction, samples were directly desorbed into the injection port of the GC which was at 250°C. The analyses of volatile compounds were identified and quantified by GC/MS (Agilent 7890A-5975C, Agilent, CA, USA) equipped with a polar fused silica capillary column (DB-Wax, J&W Scientific; 30 m × 0.25 mm 0.25 μm film thickness). The carrier gas was helium with a flow of 1.0 mL/min. The oven temperature was maintained at 40°C for 5 min, then programmed from 40°C to 250°C at the rate of 5°C/min and held at 250°C for 5 min. The GC-MS transfer line temperature was at 200°C. The MS operated in electron impact mode with electron impact energy of 70 eV; and collected data at a rate of 3.75 scans/s over a range of m/z 20–400. The volatile compounds were tentatively identified by the comparison of actual mass spectra with the published authentic spectra database in the GC/MS libraries (NIST2011), and the compounds with MS match index over 800 were reported. The identification of VOCs was further confirmed by the retention indexes (RI) and retention time (RT) portrayed by Xia et al. (Citation2017). The determination of the concentration of special individual VOC was performed by the following formula: CS = CIS × SS/SIS, where Cs indicates the relative contents of compounds in samples (μg/kg) and CIS is the concentration of IS (0.78 mg/L), and SS and SIS mean the relative peak area of samples and IS, respectively.
2.7. Low field NMR analysis (LF-NMR)
The method of Alonso et al. (Albertos et al., Citation2017) was used with the following modifications: Portions of 3 × 3 × 1 cm (about 5 g) were cut from the fish fillets and sealed with polyethylene membrane. The samples were placed in NMR tubes (7 cm diameter). T2 relaxation measurements were performed using an LF NMR analyzer (MesoMR23-060H.I, Newmai co., Ltd., CA) with a proton resonance frequency of 20 MHz. T2 was measured using the Carr-Purcell-Meiboom-Gill pulse sequence (CPMG). For each measurement 16 scans were performed with 3000 echoes, the T2 map of transverse relaxation time was obtained by iterative inversion with analytical software according to the decay curve of CPMG exponent.
2.8. Microbiological analysis
10 g of fish was aseptically homogenized in 90 ml sterile saline solution. Serial dilution was carried out and 1 ml aliquots of special serial dilutions of different treated samples were used to, respectively, inoculate on the agar (Feng, Ng, Mikš-Krajnik, & Yang, Citation2017). Briefly, the total viable counts (TVC, log CFU/g) and H2S-producing bacterial counts (log CFU/g) of the stored fillets were determined by plate count method (Zhu, Zhao, Feng, & Gao, Citation2016). Plate Count Agar plates were incubated at 37°C for 2 days and Iron Agar plates were incubated at 25 °C for 3 days, and black colonies formed by the production of H2S were enumerated.
2.9. Sensory attributes
The organoleptic properties of HTS fillets samples were carried out followed the procedure proposed by Bahram et al. (Bahram et al., Citation2016). Ten trained professional panelists (five woman and five men, aged 20–40 years) were asked to evaluate the sensory attributes from the view of color, odor, tissue morphology, elasticity and mucus using a five-point scale to determine: 5 corresponded to ‘very good’ and 1 to ‘worse’ (). The indoor environment in the sensory laboratory was limited at 20 ± 1°C and 55 ± 2% humidity, and all panelists conducted independent evaluation without interaction. Shelf life criteria assumed that rejection would occur when the final mean score of sensory attributes form every panelist declined below 3.0.
2.10. Statistical analysis
Experimental data were analyzed using SPSS software (version 19.0, IBM Company, Armonk, NY, USA). The one-way ANOVA procedure followed by Duncan’s multiple range tests was adopted to determine the significant difference (p < 0.05) between treatment means, and the results were expressed as means ± SD.
3. Results and discussion
3.1. WL and CL
The WL of all the samples kept increasing trends during storage () and a significant differences were detected during the late storage period (p < 0.05). The addition of RA at high concentrations caused a considerable reduction in the WL value regardless of different treatments, especially C4 and C5 group. Meanwhile, the WL of C4 and C5 remained below 10% during 18-day storage, indicating the promising effects of chitosan edible coating incorporated with the high concentration of RA on the maintenance of nutrients by retarding the loss of weight. Chitosan coating could confer a physical barrier to prevent the moisture loss from HTS fillets. Interestingly, the chitosan coating had a similiar effective in suppressing the CL () and samples treated with composite chitosan edible coating incorporated 30 or 40 mg/L RA showed a lower (p < 0.05) CL than other samples, however, there were no significant differences (p > 0.05) observed between C4 and C5 during storage. Fish fillets with less CL may due to the muscle proteins, especially the increased water-holding capacity of muscle proteins, which also decreased the WL (Fan, Luo, Yin, Bao, & Feng, Citation2014). Furthermore, the increase of WL as well as CL basically demonstrated the diminishment of water holding water of HTS fillets, causing the nutrients losses and decreasing the edible value. So the above results suggested that the application of the composite chitosan edible coating can be effective in decreasing the economic loss and maintaining the excellent nutrients, especially the amount of muscle proteins.
Figure 1. Changes in weight loss (WL, a), cooking loss (CL, b), electrical conductivity (EC, c), and K value (d) of samples of control and treated with edible coating during refrigeration (CK: The control samples [coating with deionized water]; C1: 1% chitosan coating; C2: 20 mg/kg rosmarinic acid coating; C3: 1% chitosan coating incorporated with 20 mg/kg rosmarinic acid; C4: 1% chitosan coating incorporated with 30 mg/kg rosmarinic acid; C5: 1% chitosan coating incorporated with 40 mg/kg rosmarinic acid).
Figura 1. Cambios en la pérdida de peso (WL, a), pérdidas por cocción (CL, b), conductividad eléctrica (EC, c), y valor K (d) de las muestras de control y de aquellas tratadas con capas comestibles durante su refrigeración. CK: Muestras de control (capa de agua desionizada); C1: capa de 1% de quitosano; C2: capa de 20 mg/kg de ácido rosmarínico; C3: capa de 1% de quitosano con 20 mg/kg de ácido rosmarínico; C4: capa de 1% de quitosano con 30 mg/kg de ácido rosmarínico; C5: capa de 1% de quitosano con 40 mg/kg de ácido rosmarínico.
![Figure 1. Changes in weight loss (WL, a), cooking loss (CL, b), electrical conductivity (EC, c), and K value (d) of samples of control and treated with edible coating during refrigeration (CK: The control samples [coating with deionized water]; C1: 1% chitosan coating; C2: 20 mg/kg rosmarinic acid coating; C3: 1% chitosan coating incorporated with 20 mg/kg rosmarinic acid; C4: 1% chitosan coating incorporated with 30 mg/kg rosmarinic acid; C5: 1% chitosan coating incorporated with 40 mg/kg rosmarinic acid).Figura 1. Cambios en la pérdida de peso (WL, a), pérdidas por cocción (CL, b), conductividad eléctrica (EC, c), y valor K (d) de las muestras de control y de aquellas tratadas con capas comestibles durante su refrigeración. CK: Muestras de control (capa de agua desionizada); C1: capa de 1% de quitosano; C2: capa de 20 mg/kg de ácido rosmarínico; C3: capa de 1% de quitosano con 20 mg/kg de ácido rosmarínico; C4: capa de 1% de quitosano con 30 mg/kg de ácido rosmarínico; C5: capa de 1% de quitosano con 40 mg/kg de ácido rosmarínico.](/cms/asset/82ddb872-19b7-4b4f-b65d-0207389a3f3e/tcyt_a_1518344_f0001_oc.jpg)
3.2. EC and K value
EC measures the conductivity of fish leachate and can be used for evaluating fish quality during post-mortem process. As shown in , the EC of fresh fillets was 1.28 mS/cm. After 6 days, the EC of CK and C2 were significantly higher than other samples by the reason that chitosan could effectively inhibit the decomposition of nutrients and the growth of the microorganism (Cai, Leng, Cao, Cheng, & Li, Citation2018). A significant differences were detected among samples except for C4 and C5 group after the 6th day during entire refrigeration (p < 0.05). Meanwhile, it is reported that chitosan coating acts as an oxygen barrier and retards the leakage of cellular proteins and other intracellular constituents by inhibiting of microbial growth, effectively delaying the increase of EC (No, Meyers, Prinyawiwatkul, & Xu, Citation2007). Interestingly, the increase rate of the EC of C3 was slower compared with C1 and C2.
As shown in , K-values of all the samples increased during storage (p < 0.05), however, the usage of chitosan combined with 30 or 40 mg/L RA coating significantly delayed the K-values increase, which had an analogous trend with the TVB-N (). The samples with RA addition had the K-values of lower than 60% at the end of storage and still under the rejection levels of the K-value (Yu, Regenstein, & Xia, Citation2018). These results also indicated that chitosan coated with RA were effective in inhibiting the degradation of ATP and maintaining desire quality of HTS fillets during 18-day storage. The correlation analysis results also suggested that there was a close connection (p = 0.9653) to assess the quality characteristics of HTS fillets between EC and K-value, further demonstrating the application universality of above mentioned two indicators on the freshness evaluation of aquatic products.
Figure 2. Changes in d total volatile bases nitrogen (TVB-N, a), Trimethylamine (TMA-N, b), Thiobarbituric acid (TBA, c), free fatty acid (d) and sulfhydryl group (-SH, e) of samples of control and treated with edible coating during refrigeration (CK: The control samples [coating with deionized water]; C1: 1% chitosan coating; C2: 20 mg/kg rosmarinic acid coating; C3: 1% chitosan coating incorporated with 20 mg/kg rosmarinic acid; C4: 1% chitosan coating incorporated with 30 mg/kg rosmarinic acid; C5: 1% chitosan coating incorporated with 40 mg/kg rosmarinic acid).
Figura 2. Cambios en el nitrógeno volátil básico total (TVB-N, a), Trimetilamina (TMA-N, b), Ácido tiobarbitúrico (TBA, c), ácido graso libre (d) y grupo sulfhidrilo (-SH, e) de las muestras de control y las tratadas con capas comestibles durante su refrigeración. CK: Muestras de control (capa de agua desionizada); C1: capa de 1% de quitosano; C2: capa de 20 mg/kg de ácido rosmarínico; C3: capa de 1% de quitosano con 20 mg/kg de ácido rosmarínico; C4: capa de 1% de quitosano con 30 mg/kg de ácido rosmarínico; C5: capa de 1% de quitosano con 40 mg/kg de ácido rosmarínico.
![Figure 2. Changes in d total volatile bases nitrogen (TVB-N, a), Trimethylamine (TMA-N, b), Thiobarbituric acid (TBA, c), free fatty acid (d) and sulfhydryl group (-SH, e) of samples of control and treated with edible coating during refrigeration (CK: The control samples [coating with deionized water]; C1: 1% chitosan coating; C2: 20 mg/kg rosmarinic acid coating; C3: 1% chitosan coating incorporated with 20 mg/kg rosmarinic acid; C4: 1% chitosan coating incorporated with 30 mg/kg rosmarinic acid; C5: 1% chitosan coating incorporated with 40 mg/kg rosmarinic acid).Figura 2. Cambios en el nitrógeno volátil básico total (TVB-N, a), Trimetilamina (TMA-N, b), Ácido tiobarbitúrico (TBA, c), ácido graso libre (d) y grupo sulfhidrilo (-SH, e) de las muestras de control y las tratadas con capas comestibles durante su refrigeración. CK: Muestras de control (capa de agua desionizada); C1: capa de 1% de quitosano; C2: capa de 20 mg/kg de ácido rosmarínico; C3: capa de 1% de quitosano con 20 mg/kg de ácido rosmarínico; C4: capa de 1% de quitosano con 30 mg/kg de ácido rosmarínico; C5: capa de 1% de quitosano con 40 mg/kg de ácido rosmarínico.](/cms/asset/8047ed2e-0929-4fcf-b786-b27d5139e493/tcyt_a_1518344_f0002_oc.jpg)
3.3. Chemical quality parameters
TVB-N is associated with fish spoilage and high TVB-N values are not desirable (Volpe et al., Citation2015). It is a general consensus that 25 mg N/100 g of fish fillets is the highest acceptable level as proposed by Giménez et al. (Gimenez, Roncales, & Beltran, Citation2002). In the present study, the TVB-N of HTS fillets increased with storage time for all the samples, especially increasing progressively with the extension of storage time after 8th day presented in (p < 0.05). The TVB-N values reached an unacceptable level (>25 mg N/100 g) for CK, C1, C2, C3, C4, C5, and C6 were at day 12, 14, 12, 16, 16, and 18, respectively, while the initial TVB-N was less than 10 mg N/100 g. TVB-N content refers produced volatile nitrogenous (such as ammonia and amine) substances based on the influence of enzymes and bacteria. These results indicated that the synergistic effect of chitosan and RA could be capable of retarding the TVB-N production. This synergistic effect could be attributed to either a more rapidly reduced bacterial population or decreased capacity of bacteria for oxidative deamination of non-protein nitrogen compounds, especially the wide spectrum of substantial biological activities of RA including antioxidant and antimicrobial properties (Li et al., Citation2012). Abdollahi et al. also reported that the chitosan/clay coating with rosemary essential oil could lower the TVB-N values of fresh silver carp fillets at 4°C for 16 days storage (Abdollahi, Rezaei, & Farzi, Citation2014).
TMA come from the bacterial breakdown of trimethylamine oxide and it is responsible for ‘fishy’ off-odor (Günlü & Koyun, Citation2013). In our study, the TMA-N value increased in all sample groups in relation to time with storage period (p < 0.05). As shown in . The TMA content at day 0 was about 0.15 mg of TMA/100 g fish muscle. Previous literature indicated that fish with less than 1.0 mg TMA-N/100 g is considered of good quality, while 5–10 mg TMA-N/100 g is considered the limit of acceptability. In the experiment, the final TMA values in CK group sharply increased to 3.7 mg TMA-N/100 g on the 18th day, while the corresponding value in C1, C2, C3, C4, and C5 were only 3.0, 3.2, 2.6, 2.2, and 2 TMA-N/100 g, respectively. TVB-N and TMA are directly related to the microbial spoilage in fish during refrigerated storage (Yu, Regenstein et al., Citation2018). The increase of TMA for HTS fillets is in line with bacterial activity; therefore, the chitosan coating with RA inhibit bacterial growth and reduce the accumulation of TMA. Similar results were also confirmed that chitosan coating incorporated with bioactive substances may effect on delaying the increasing of the TVB-N and TMA-N value (Alak, Hisar, Hisar, & Genççelep, Citation2011; Gómez-Estaca, López de Lacey, López-Caballero, Gómez-Guillén, & Montero, Citation2010; Gonca, Sükriyearas, Olcay, & Hüseyin, Citation2011; Izci et al., Citation2017).
illustrates the effect of chitosan coating with RA on TBA formation during storage. The TBA value at day 0 for the HTS fillets was 0.45 mg MDA kg−1. A similar initial TBA value of 0.43 mg MDA kg−1 was reported in fresh rainbow trout fillets (Choulitoudi et al., Citation2017). Subsequently, the TBA values of all the samples showed upward trends in the early storage period and then there were no specific trends in the later stage, which mostly due to the reaction of malondialdehyde with aldehydes and ketones (Da, Ferreira, Pintado, & Sarmento, Citation2016). The highest TBA value of the CK was 0.70 mg MDA kg−1, while the highest TBA values of the treated samples (0.64, 0.63, 0.6, 0.65, and 0.63 mg MDA kg−1 for C1, C2, C3, C4, C5, respectively) were lower than that of CK whereas there were no significant differences among the treated samples (p < 0.05).
FFA concentrations in refrigerated HTS fillets increased with storage time in all samples (). Samples treated with composite chitosan coating containing RA revealed lower FFA levels throughout storage compared to the CK, C1, and C2 group (p < 0.05). Meanwhile, a significant difference (p < 0.05) could be depicted between chitosan batches including the different concentrations of RA and increasing rate were retarded as the concentration of RA increased. FFA formation can be considered the result of endogenous enzyme and microbial activities (Oucif et al., Citation2017). In the present study, the relevant higher values found in the CK during the 8–18-day period would correspond to microbial activity.
In view of TBA value and FFA contents, all results unanimously indicated that lipid auto-oxidation occurred with the extension of storage time, and high concentrations of RA of chitosan-based coating undoubtedly maintained favorable quality properties of HTS fillets by suppressing this process, which probably owing to the excellent activities of bioactive substances in RA.
Chitosan coated with different concentrations of RA prevented the decrease of –SH significantly (p < 0.05) in HTS fillets during storage (). Clearly, compared to CK, 30 or 40 mg/L RA could significantly (p < 0.05) retard the decreasing process of –SH content. The -SH inside the protein structures is easily oxidized to the disulfide bonds during storage, which leads to structure destruction of protein and corresponding decrease in –SH content (Ma, Yi, Yu, Li, & Chen, Citation2015). Meanwhile, the content of –SH are significantly lower for C2 compared to C3, C4, and C5, indicating the synergistic effect of chitosan and RA. The mechanism of the result is closely related to the antioxidant activity and oxygen-barrier property of the chitosan (Elsabee & Abdou, Citation2013). Because RA is a naturally strong antioxidant, incorporating RA can significantly improve the antioxidant activity of the chitosan coating, resulting in lower –SH content of HTS fillets. These results were consistent with the previous studies (Gao et al., Citation2014; Wu et al., Citation2016). Besides, the thiol groups on the protein surfaces may compete with RA to trap the free radicals.
FAAs are responsible for flavor development and some FAAs, are precursors of harmful biogenic amines. In the present study, the higher relative abundant of glycine, alanine, glutamic acid, and aspartic acid were detected in earlier periods (). The initial increase of FAAs may be due to the breakdown of amino acids and oligopeptides caused by proteolytic enzymes and the later decrease in FAAs may have been due to the degradation of FAAs by the growth of microorganisms during refrigerated storage. The total FAAs level at day 18 in chitosan coated with RA samples was significantly higher than that of CK, C1, and C2. The most abundant FAA was arginine, followed by histidine, glycine, and lysine, which was similar to raw grass carp fillets with chitosan-clove bud essential oil during refrigerated storage (Yu, Xu et al., Citation2018). As an off-taste amino acid, histidine in CK increased from 17.26 mg/100 g at day 0 to 36.27 mg/100 g at day 9, however, the corresponding contents in C1, C2, C3, C4, and C5 were only 33.98, 35.78, 18.13, 17.98, and 12.03 mg/100 g at day 9, respectively. Lower histidine content in treated samples led to a decrease in bitterness.
Table 1. The evaluation standards of sensory characteristics of half-smooth tongue sole fillet during refrigeration.
Tabla 1. Estándares de evaluación de las características sensoriales de filetes de lenguado durante su refrigeración.
Table 2. Changes in free amino acids contents (mg/100 g) in half-smooth tongue sole fillet at day 0, 9, and 18 during refrigeration.
Tabla 2. Cambios en el contenido de aminoácidos libres (mg/100 g) en los filetes de lenguado en los días 0, 9 y 18 de refrigeración.
The level of protein decomposition could be reflected in the above mentioned special indexes, including TVB-N, TMA, –SH group and FAAs. Basically, during the degradation of the macromolecular protein, peptides, and amino acids were first produced and subsequently, amines and ammonia were accumulated via further interaction of peptides and amino acids, causing the increase of TVB-N and TMA. All in all, all results indicated that the best treatment applied for HTS fillets was chitosan-based coating containing 30 mg/L RA.
3.4. Volatile compounds (VOCs)
Without taking into account aromatic compounds, hydrocarbons and amines compositions, a total of 20 main VOCs were detected: 5 aldehydes, 8 ketones and 7 alcohols (). The changes of these VOCs during storage were semi-quantitatively analyzed by comparing the peak areas.
Table 3. Identification and semi-quantification of main volatile compounds (μg/kg) in half-smooth tongue sole fillet at day 0, 9 and 18 during refrigeration.
Tabla 3. Identificación y semicuantificación de los principales compuestos volátiles (μg/kg) en los filetes de lenguado durante los días 0, 9 y 18 de refrigeración.
Some aldehydes and alcohols characterized the fresh fish flavor were detected at day 0 and the peak areas of acetoin, ethanol, benzaldehyde and methanethiol were larger than other compounds. Benzyl alcohol, phenylethyl alcohol, phenylacetaldehyde, 2-nonanone, 3-octanone, and 2-decanone were detected only at mid and/or final stages, and some compounds (including 1-octen-3-ol, 2-decanol, 3-pentanone, 2-octanone) were detected initially and decreased or even disappeared at the end storage, and the remaining 10 compounds were detected in all samples throughout storage. Alcohol, 1-hexanol, benzaldehyde, and acetoin showed a tendency to decrease gradually, however, 3-methyl-butanal, hexanal, octanal, and 2-pentanone increased during refrigerated storage. Benzaldehyde was derived from the degradation of amino acid and has a desirable effect on fish odor, and the higher relative abundance of benzaldehyde could be detected at the beginning than that of at the terminal of storage, which was in agreement with Li et al. (Li, Zhang, & Luo, Citation2018). In addition, methanethiol and phenylacetaldehyde presented an inverted V-shaped change trend. 1-Octen-3-ol showed a V-shape and associated with the enhanced activity of 12-lipoxygenase on arachidonic acid, which could be considered as the lipid oxidation marker for HTS fillets. The relative contents of benzyl alcohol, octanal, 2-nonanone, 2-pentanone, 2-heptanone and 1-octen-3-ol in the CK were higher than that of treated samples. And some of these compounds had putrid odors above threshold values, such as octanal and 1-octen-3-ol, having grassy or mushroom-like odors. The abundance of VOCs in C4 and C5 samples were lower than others, probably due to RA retarded the rate of polyunsaturated fatty acid oxidation and reduced spoilage together with chitosan (Alishahi & Aïder, Citation2012). In the present study, the fluctuation and depletion of some VOCs due to the metabolites produced by spoilage microorganisms and could also be further metabolized during storage. The accumulation of some VOCs was caused by metabolism of spoilage microorganisms and chemical reactions (Yu, Xu et al., Citation2018). So all results found in VOCs chitosan-based edible coating containing high concentrations of RA could effectively keep flavor quality of HTS fillets by retarding the amounts of spoilage microorganisms and process of chemical reactions.
3.5. Water distribution by LF NMR analysis
In the present study, T21 had little changes for all the samples during 18-day storage, however, T22 decreased and T23 had a simultaneous increase (). That is in accordance with previously reported results in hake (Zang et al., Citation2017). There was a slight increase in T21 values during 0–12 day storage and then decreased for all the samples, which is related to a population of myofibril water. However, a significant reduction of T22 (p < 0.05) was occurred, which refers to the increased free water from protein degradation. T22 of the CK was decreased from 98.31% at day 0 to 68.61% at day 18. Fish fillets with chitosan coating containing RA have lower content of free water (T23 value) than that in CK, which also may be considered to be related with the water vapor barrier of chitosan coating. The above results in LF-NMR were consistent with the WL as well as CL in this study, indicating the advantage of chitosan edible coating containing high concentrations of RA in keeping nutrient substances and extending shelf life of HTS fillets.
Table 4. Changes in the percentage of T2i in half-smooth tongue sole fillet at day 0, 6, 12, and 18 during refrigeration.
Tabla 4. Cambios en el porcentaje de T2i en filetes de lenguado en los días 0, 6, 12 y 18 de refrigeración.
3.6. Microbial growth
The evolution of TVC and H2S-producing bacteria during HTS fillets storage was shown in . The initial concentration of TVC for the fillets was 2.84 log CFU/g, indicating the excellent quality attributes of purchased fish. The TVC values dramatically increased for all samples with the extension of storage time (p < 0.05). The samples with chitosan or/and RA coating had significantly lower TVC than that of the CK during refrigerated storage (p < 0.05), especially the C4 and C5 samples did not exceed 6.0 log CFU/g at day 18. These results clearly demonstrated that chitosan coating with 30 mg/L RA exerted stronger inhibitory effects on microbial growth in HTS fillets than either treatment alone and extended the shelf life of HTS fillets.
Figure 3. Changes in total viable counts (TVC, a) and H2S-producing bacteria (b) of samples of control and treated with edible coating during refrigeration (CK: The control samples [coating with deionized water]; C1: 1% chitosan coating; C2: 20 mg/kg rosmarinic acid coating; C3: 1% chitosan coating incorporated with 20 mg/kg rosmarinic acid; C4: 1% chitosan coating incorporated with 30 mg/kg rosmarinic acid; C5: 1% chitosan coating incorporated with 40 mg/kg rosmarinic acid).
Figura 3. Cambios en recuentos viables totales (TVC, a) y bacterias productoras de H2S (b) en las muestras de control y en las tratadas con capas comestibles durante su refrigeración. CK: Muestras de control (capa de agua desionizada); C1: capa de 1% de quitosano; C2: capa de 20 mg/kg de ácido rosmarínico; C3: capa de 1% de quitosano con 20 mg/kg de ácido rosmarínico; C4: capa de 1% de quitosano con 30 mg/kg de ácido rosmarínico; C5: capa de 1% de quitosano con 40 mg/kg de ácido rosmarínico.
![Figure 3. Changes in total viable counts (TVC, a) and H2S-producing bacteria (b) of samples of control and treated with edible coating during refrigeration (CK: The control samples [coating with deionized water]; C1: 1% chitosan coating; C2: 20 mg/kg rosmarinic acid coating; C3: 1% chitosan coating incorporated with 20 mg/kg rosmarinic acid; C4: 1% chitosan coating incorporated with 30 mg/kg rosmarinic acid; C5: 1% chitosan coating incorporated with 40 mg/kg rosmarinic acid).Figura 3. Cambios en recuentos viables totales (TVC, a) y bacterias productoras de H2S (b) en las muestras de control y en las tratadas con capas comestibles durante su refrigeración. CK: Muestras de control (capa de agua desionizada); C1: capa de 1% de quitosano; C2: capa de 20 mg/kg de ácido rosmarínico; C3: capa de 1% de quitosano con 20 mg/kg de ácido rosmarínico; C4: capa de 1% de quitosano con 30 mg/kg de ácido rosmarínico; C5: capa de 1% de quitosano con 40 mg/kg de ácido rosmarínico.](/cms/asset/cf33301a-ca3f-4c48-88f0-86ff00211f89/tcyt_a_1518344_f0003_oc.jpg)
H2S-producing bacteria are one of the specific spoilage organisms (SSOs) because of producing H2S and reducing TMAO to TMA. H2S-producing bacteria were also increased for all samples during entire storage (p < 0.05). The chitosan coating containing more than 20 mg/L RA could effectively inhibit the H2S-producing bacteria growth and the C3, C4 and C 5 samples did not exceed 6.0 log CFU/g at day 18. The results may be owing to the strong antimicrobial capacity of chitosan by the interaction of the positively charged amine group with the negatively charged cell wall and membrane components, resulting in increased leakage of cell material from tissue and permeability of the membranes (Wu et al., Citation2016). At the same time, antimicrobial activities of RA could improve the antimicrobial capacity of composite chitosan edible coating.
It was known that Microbial growth played an important role in assessing the quality of aquatic products, and the results of TVC and H2S-producing bacteria showed the strong correlation with other physicochemical indexes, which further verified the potential application values of chitosan coating including RA in HTS fillets.
3.7. Sensory evaluation
Changes in the sensory evaluation values (color, odor, tissue, elasticity, and mucus) in all the samples are given in . The best evaluations of sensory were reported in the C4 and C5. The quality of physical appearance was reduced following 18 days of refrigeration, this was noticed by panelists. The CK and C2 quickly deteriorated and reached an unacceptable level at day 10, which was in accordance with the TVB-N values (>25 mg N/100 g). However, if any of the sensory scores for the HTS fillets was less than 3, all the scores were not shown in the figures. The odor and tissue qualities of all the samples were acceptable during the 18 days of storage in C4 and C5 (p ˂ 0.05). And higher appearance score of chitosan-RA coated HTS fillets may result from the protective coating preventing the color deterioration (Abdallah, Mohmaed, Mohamed, & Emara, Citation2017). Besides, the elasticity and mucus in all chitosan-RA coated HTS fillets were also improved.
Figure 4. Radar map in sensory scores of samples of control and treated with edible coating during refrigeration (CK: The control samples [coating with deionized water]; C1: 1% chitosan coating; C2: 20 mg/kg rosmarinic acid coating; C3: 1% chitosan coating incorporated with 20 mg/kg rosmarinic acid; C4: 1% chitosan coating incorporated with 30 mg/kg rosmarinic acid; C5: 1% chitosan coating incorporated with 40 mg/kg rosmarinic acid).
Figura 4. Imagen de radar de las puntuaciones sensoriales de las muestras de control y las tratadas con capas comestibles durante su refrigeración. CK: Muestras de control (capa de agua desionizada); C1: capa de 1% de quitosano; C2: capa de 20 mg/kg de ácido rosmarínico; C3: capa de 1% de quitosano con 20 mg/kg de ácido rosmarínico; C4: capa de 1% de quitosano con 30 mg/kg de ácido rosmarínico; C5: capa de 1% de quitosano con 40 mg/kg de ácido rosmarínico.
![Figure 4. Radar map in sensory scores of samples of control and treated with edible coating during refrigeration (CK: The control samples [coating with deionized water]; C1: 1% chitosan coating; C2: 20 mg/kg rosmarinic acid coating; C3: 1% chitosan coating incorporated with 20 mg/kg rosmarinic acid; C4: 1% chitosan coating incorporated with 30 mg/kg rosmarinic acid; C5: 1% chitosan coating incorporated with 40 mg/kg rosmarinic acid).Figura 4. Imagen de radar de las puntuaciones sensoriales de las muestras de control y las tratadas con capas comestibles durante su refrigeración. CK: Muestras de control (capa de agua desionizada); C1: capa de 1% de quitosano; C2: capa de 20 mg/kg de ácido rosmarínico; C3: capa de 1% de quitosano con 20 mg/kg de ácido rosmarínico; C4: capa de 1% de quitosano con 30 mg/kg de ácido rosmarínico; C5: capa de 1% de quitosano con 40 mg/kg de ácido rosmarínico.](/cms/asset/22b8bcc6-3ba4-4f8a-9312-65ae431d0148/tcyt_a_1518344_f0004_oc.jpg)
4. Conclusion
The ability of 1% chitosan coating incorporated with 20, 30 or 40 mg/L RA to improve the quality of HTS fillets at 4°C for 18 days is discussed in the present study. The results of the physicochemical (WL, CL, TVB-N, TMA, EC, TBA, K values, FFAs and FAAs), microbiological (TVC and H2S-producing bacteria), and sensory analyses revealed that 1% chitosan coating with 30 mg/L RA sufficiently inhibited the water loss, protein decomposition, lipid oxidation, microbial growth, and maintained better sensory characteristics and flavor quality during refrigerated storage. Therefore, the combination of 1% chitosan coating with 30 mg/L RA could become a promising alternative for improving the storage quality of fresh HTS fillets.
Disclosure statement
No potential conflict of interest was reported by the authors.
Acknowledgments
The authors gratefully acknowledge the funding from National Natural Science Foundation of China (31601414), National Key Research and Development Program (2016YFD0400106), and also supported by Construction Project of Public Service Platform for Shanghai Municipal Science and Technology Commission (17DZ2293400). The authors would like to express their profound gratitude to Wenjuan Yu from Instrumental Analysis Center of Shanghai Jiao Tong University for her technical assistance.
Additional information
Funding
References
- Abdallah, M. R. S., Mohmaed, M. A., Mohamed, H. M. H., & Emara, M. M. T. (2017). Improving the sensory, physicochemical and microbiological quality of pastirma (A traditional dry cured meat product) using chitosan coating. LWT - Food Science and Technology, 86, 247–253.
- Abdollahi, M., Rezaei, M., & Farzi, G. (2014). Influence of chitosan/clay functional bionanocomposite activated with rosemary essential oil on the shelf life of fresh silver carp. International Journal of Food Science & Technology, 49(3), 811–818.
- Alak, G., Hisar, S. A., Hisar, O., & Genççelep, H. (2011). Biogenic amines formation in Atlantic bonito (Sarda sarda) fillets packaged with modified atmosphere and vacuum, wrapped in chitosan and cling film at 4 °C. European Food Research and Technology, 232(1), 23–28.
- Albertos, I., Martin-Diana, A. B., Cullen, P. J., Tiwari, B. K., Ojha, S. K., Bourke, P., & Rico, D. (2017). Shelf-life extension of herring (Clupea harengus) using in-package atmospheric plasma technology. Innovative Food Science & Emerging Technologies. In Press, doi:10.1016/j.ifset.2017.09.010
- Alishahi, A., & Aïder, M. (2012). Applications of chitosan in the seafood industry and aquaculture: A review. Food & Bioprocess Technology, 5(3), 817–830.
- Bahram, S., Rezaie, M., Soltani, M., Kamali, A., Abdollahi, M., Ahmadabad, M. K., & Nemati, M. (2016). Effect of whey protein concentrate coating cinamon oil on quality and shelf life of refrigerated beluga sturegeon (Huso huso). Journal of Food Quality, 39, 743–749.
- Berizi, E., Hosseinzadeh, S., Shekarforoush, S. S., & Barbieri, G. (2017). Microbial, chemical, textural and sensory properties of coated rainbow trout by chitosan combined with pomegranate peel extract during frozen storage. International Journal of Biological Macromolecules, 106, 1004–1013.
- Cai, L., Leng, L., Cao, A., Cheng, X., & Li, J. (2018). The effect of chitosan‐essential oils complex coating on physicochemical, microbiological, and quality change of grass carp (Ctenopharyhgodon idella) fillets. Journal of Food Safety, 38(1), e12399.
- Choulitoudi, E., Ganiari, S., Tsironi, T., Ntzimani, A., Tsimogiannis, D., Taoukis, P., & Oreopoulou, V. (2017). Edible coating enriched with rosemary extracts to enhance oxidative and microbial stability of smoked eel fillets. Food Packaging & Shelf Life, 12, 107–113.
- Cota-Arriola, O., Plascencia-Jatomea, M., Lizardi-Mendoza, J., Robles-Sánchez, R. M., Ezquerra-Brauer, J. M., Ruíz-García, J., … Cortez-Rocha, M. O. (2017). Preparation of chitosan matrices with ferulic acid: Physicochemical characterization and relationship on the growth of aspergillus parasiticus. CyTA - Journal of Food, 15(1), 1–10.
- Da, S. S., Ferreira, D., Pintado, M., & Sarmento, B. (2016). Chitosan-based nanoparticles for rosmarinic acid ocular delivery–In vitro tests. International Journal of Biological Macromolecules, 84, 112–120.
- Elsabee, M. Z., & Abdou, E. S. (2013). Chitosan based edible films and coatings: A review. Materials Science & Engineering C, 33(4), 1819–1841.
- Erbay, E. A., Dağtekin, B. B., Türe, M., Yeşilsu, A. F., & Torres-Giner, S. (2017). Quality improvement of rainbow trout fillets by whey protein isolate coatings containing electrospun poly (ε-caprolactone) nanofibers with Urtica dioica L. extract during storage. LWT - Food Science and Technology, 78, 340–351.
- Fan, H., Luo, Y., Yin, X., Bao, Y., & Feng, L. (2014). Biogenic amine and quality changes in lightly salt- and sugar-salted black carp (Mylopharyngodon piceus) fillets stored at 4 °C. Food Chemistry, 159, 20–28.
- Feng, X., Bansal, N., & Yang, H. (2016). Fish gelatin combined with chitosan coating inhibits myofibril degradation of golden pomfret (Trachinotus blochii) fillet during cold storage. Food Chemistry, 200, 283–292.
- Feng, X., Ng, V. K., Mikš-Krajnik, M., & Yang, H. (2017). Effects of fish gelatin and tea polyphenol coating on the spoilage and degradation of myofibril in fish fillet during cold storage. Food & Bioprocess Technology, 10, 1–14.
- Gao, M., Feng, L., Jiang, T., Zhu, J., Fu, L., Yuan, D., & Li, J. (2014). The use of rosemary extract in combination with nisin to extend the shelf life of pompano (Trachinotus ovatus) fillet during chilled storage. Food Control, 37(1), 1–8.
- Gharibzahedi, S. M., & Mohammadnabi, S. (2016). Effect of novel bioactive edible coatings based on jujube gum and nettle oil-loaded nanoemulsions on the shelf-life of Beluga sturgeon fillets. International Journal of Biological Macromolecules, 95, 769–777.
- Gimenez, B., Roncales, P., & Beltran, J. A. (2002). Modified atmosphere packaging of filleted rainbow trout. Journal of the Science of Food and Agriculture, 82, 1154–1159.
- Gómez-Estaca, J., López de Lacey, A., López-Caballero, M. E., Gómez-Guillén, M. C., & Montero, P. (2010). Biodegradable gelatin–Chitosan films incorporated with essential oils as antimicrobial agents for fish preservation. Food Microbiology, 27(7), 889–896.
- Gonca, A., Sükriyearas, H., Olcay, H., & Hüseyin, G. (2011). Biogenic amines formation in Atlantic bonito (Sarda sarda) fillets packaged with modified atmosphere and vacuum, wrapped in chitosan and cling film at 4 °C. European Food Research & Technology, 232(1), 23–28.
- Günlü, A., & Koyun, E. (2013). Effects of vacuum packaging and wrapping with chitosan-based edible film on the extension of the shelf life of sea bass (Dicentrarchus labrax) fillets in cold storage (4 °C). Food & Bioprocess Technology, 6(7), 1713–1719.
- Izci, L., Ekici, F., Günlü, A., Izci, L., Ekici, F., & Günlü, A. (2017). Coating with chitosan film of sea bream (Sparus aurata) fillets: Determining shelf life in refrigerator conditions. Food Sciences Technological (Oline). doi:10.1590/1678-457x.38416
- Jiang, L., & Wu, S. (2018). Pullulan suppresses the denaturation of myofibrillar protein of grass carp (Ctenopharyngodon idella) during frozen storage. International Journal of Biological Macromolecules, 112, 1171–1174.
- Kakaei, S., & Shahbazi, Y. (2016). Effect of chitosan-gelatin film incorporated with ethanolic red grape seed extract and Ziziphora clinopodioides essential oil on survival of Listeria monocytogenes and chemical, microbial and sensory properties of minced trout fillet. LWT - Food Science and Technology, 72, 432–438.
- Li, Q., Zhang, L., & Luo, Y. (2018). Changes in microbial communities and quality attributes of white muscle and dark muscle from common carp (Cyprinus carpio) during chilled and freeze-chilled storage. Food Microbiology, 73, 237–244.
- Li, T., Hu, W., Liac, J., Zhu, J., & Li, X. (2012). Coating effects of tea polyphenol and rosemary extract combined with chitosan on the storage quality of large yellow croaker (Pseudosciaena crocea). Food Control, 25(1), 101.
- Liu, F., Yang, Y., Li, Y., Guo, H., Dai, H., Gao, J., … Chen, S. (2016). Phenotypic and genetic parameter estimation of juvenile growth and bottom color traits in half-smooth tongue sole, Cynoglossus semilaevis. Acta Oceanologica Sinica, 35(10), 83–87.
- Ma, X. S., Yi, S. M., Yu, Y. M., Li, J. R., & Chen, J. R. (2015). Changes in gel properties and water properties of nemipterus virgatus surimi gel induced by high-pressure processing. Lwt - Food Science and Technology, 61(2), 377–384. doi:10.1016/j.lwt.2014.12.041
- Natseba, A., Lwalinda, I., Kakura, E., Muyanja, C. K., & Muyonga, J. H. (2005). Effect of pre-freezing icing duration on quality changes in frozen Nile perch (Lates niloticus). Food Research International, 38(4), 469–474.
- No, H. K., Meyers, S. P., Prinyawiwatkul, W., & Xu, Z. (2007). Applications of chitosan for improvement of quality and shelf life of foods: A review. Journal of Food Science, 72(5), R87–R100.
- Ou, J., Teng, J., El-Nezami, H. S., & Wang, M. (2018). Impact of resveratrol, epicatechin and rosmarinic acid on fluorescent ages and cytotoxicity of cookies. Journal of Functional Foods, 40, 44Ou, J., Teng, J., El-Nezami, H. S., & Wang, M. (2018). Impact of resveratrol, epicatechin and rosmarinic acid on fluorescent AGEs and cytotoxicity of cookies. Journal of Functional Foods, 40, 44-50.50. doi:10.1016/j.jff.2017.10.042
- Oucif, H., Miranda, J. M., Mehidi, S. A., Abi-Ayad, E. A., Barros-Velázquez, J., & Aubourg, S. P. (2017). Effectiveness of a combined ethanol-aqueous extract of alga Cystoseira compressa for the quality enhancement of a chilled fatty fish species. European Food Research & Technology, 244(2), 291–299.
- Sha, Z. X., Wang, Q. L., Liu, Y., & Chen, S. L. (2012). Identification and expression analysis of goose-type lysozyme in half-smooth tongue sole (Cynoglossus semilaevis). Fish & Shellfish Immunology, 32(5), 914–921.
- Su, H., Chen, W., Fu, S., Wu, C., Li, K., Huang, Z., … Li, J. (2014). Antimicrobial effect of bayberry leaf extract for the preservation of large yellow croaker (Pseudosciaena crocea). Journal of the Science of Food and Agriculture, 94, 935–942.
- Volpe, M. G., Siano, F., Paolucci, M., Sacco, A., Sorrentino, A., Malinconico, M., & Varricchio, E. (2015). Active edible coating effectiveness in shelf-life enhancement of trout (Oncorhynchus mykiss) fillets. LWT - Food Science and Technology, 60(1), 615–622.
- Wang, T., Li, Z., Mi, N., Yuan, F., Zou, L., Lin, H., & Pavase, T. (2017). Effects of brown algal phlorotannins and ascorbic acid on the physiochemical properties of minced fish (Pagrosomus major) during freeze-thaw cycles. International Journal of Food Science & Technology, 52, 706–713.
- Wu, C., Li, Y., Wang, L., Hu, Y., Chen, J., Liu, D., & Ye, X. (2016). Efficacy of chitosan-gallic acid coating on shelf life extension of refrigerated pacific mackerel fillets. Food & Bioprocess Technology, 9(4), 675–685.
- Wu, T., Wu, C., Fang, Z., Ma, X., Chen, S., & Hu, Y. (2017). Effect of chitosan microcapsules loaded with nisin on the preservation of small yellow croaker. Food Control, 79, 317–324.
- Xia, Q., Mei, J., Yu, W., & Li, Y. (2017). High hydrostatic pressure treatments enhance volatile components of pre-germinated brown rice revealed by aromatic fingerprinting based on HS-SPME/GC-MS and chemometric methods. Food Research International, 91, 103–114.
- Xie, J., Vanalstyne, P., Uhlir, A., & Yang, X. (2017). A review on rosemary as a natural antioxidation solution. European Journal of Lipid Science & Technology, 119, 1600439.
- Yu, D., Regenstein, J. M., & Xia, W. (2018). Bio-based edible coatings for the preservation of fishery products: A review. Critical Reviews in Food Science & Nutrition, (Just-Accepted), 1–46. doi:10.1080/10408398.2018.1457623
- Yu, D., Xu, Y., Regenstein, J. M., Xia, W., Yang, F., Jiang, Q., & Wang, B. (2018). The effects of edible chitosan-based coatings on flavor quality of raw grass carp (Ctenopharyngodon idellus) fillets during refrigerated storage. Food Chemistry, 242(21), 412–420.
- Yuan, G., Zhang, X., Tang, W., & Sun, H. (2016). Effect of chitosan coating combined with green tea extract on the melanosis and quality of pacific white shrimp during storage in ice. CyTA - Journal of Food, 14(1), 35–40.
- Zang, X., Lin, Z., Zhang, T., Wang, H., Cong, S., Song, Y., … Tan, M. (2017). Non-destructive measurement of water and fat contents, water dynamics during drying and adulteration detection of intact small yellow croaker by low field NMR. Journal of Food Measurement & Characterization, 11(4), 1550–1558.
- Zhu, J., Zhao, A., Feng, L., & Gao, H. (2016). Quorum sensing signals affect spoilage of refrigerated large yellow croaker (Pseudosciaena crocea) by Shewanella baltica. International Journal of Food Microbiology, 217, 146–155.