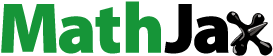
ABSTRACT
The aim of this study was to develop a culture medium based on whey for the improvement of the probiotic characteristics of Lactobacillus fermentum K73 in submerged culture. Response surface methodology was used to determine the effects of culture conditions (whey and yeast extract concentration, pH, and agitation) on growth and probiotic characteristics of the lactic acid bacterium. Results showed that whey and yeast extract concentrations were the most influential variables in the responses. The conditions for maximal final cell count (10.783 log (CFU/ml)), minimal lag phase duration (1.022 h), minimal decrease under acid stress conditions (0.097 log (CFU/ml)) and a decrease in biomass in the presence of 0.3% bile salts of 2.58 log (CFU/ml) were: 44.6 g/L of whey, 25 g/L of yeast extract, pH 5.5 and an agitation rate of 100 rpm. These results are the first to indicate that the optimization of the expression of probiotic characteristics of microorganisms is feasible.
RESUMEN
El objetivo de este estudio fue el desarrollo de un medio de cultivo basado en suero para mejorar las características probióticas de Lactobacillus fermentum K73 en cultivo sumergido. Se usó la metodología de superficie de respuesta (RSM) para determinar los efectos de las condiciones de cultivo (concentración de suero y extracto de levadura, pH y agitación) sobre el crecimiento y características probióticas de la bacteria láctica.
Los resultados mostraron que las concentraciones de suero y extracto de levadura fueron las variables de mayor importancia. Las condiciones para una máxima cuenta celular final (10.783 log (UFC/ml)), una mínima duración de la fase lag (1.022 h), una mínima disminución en concentración bajo condiciones de estrés ácido (0.097 log (UFC/ml)) and en presencia de 0.3% de sales biliares (2.58 log (UFC/ml)) fueron: 44.6 g/L de suero, 25 g/L de extracto de levadura, pH 5.5 and y una velocidad de agitación de 100 rpm. Estos resultados son los primeros en indicar que es posible optimizar la expresión de características probióticas de microorganismos.
Introduction
Probiotics have been defined by FAO/WHO as ‘live microorganism, which when administered in adequate amounts confer a health benefit on the host’ (Morelli & Capurso, Citation2012). Lactobacillus fermentum K73, a promissory probiotic strain, shows an important hypocholesterolemic effect based on the activity of the enzyme bile salt hydrolase (BSH) and cholesterol absorption (Corzo & Gilliland, Citation1999; Cueto-Vigil, Acuña-Monsalve, & Valenzuela-Riaño, Citation2010). Based on the above, its introduction in a food matrix to develop a functional food would be very important. The industry of functional foods based on probiotics has been growing at 7% per year (Dissanayake, Ramchandran, Piyadasa, & Vasiljevic, Citation2013). However, probiotic strains need different strategies for their production on a large scale, because the production process could injure the probiotic cells so the final adequate concentration in the food (106–109 CFU/ml or CFU/g) could not be reached (Shene & Bravo, Citation2007). Probiotics usually have characteristics that allow them to be produced on a large scale so they can be incorporated into food products without losing viability or functionality. Extensive research is still necessary for the optimization of biomass production by fermentation and for an optimal expression of probiotic characteristics (Annan, Borza, & Hansen, Citation2008).
Therefore, the process of biomass production must ensure that the microorganism does not change physiologically so the probiotic cells can still continue to tolerate the acidic conditions of the stomach and the bile salts presence in the small intestine without significant reductions in cell number (Calderon Santoyo, Loiseau, Rodriguez Sanoja, & Guyot, Citation2003; Lin, Hwang, Chen, & Tsen, Citation2006). These processes are influenced by the composition of the culture medium, agitation rate, and pH. Byproducts of the food industry, such as cheese whey, could enter in the composition of culture media. Whey is rich in proteins, minerals, lactose and oligosaccharides. The major proteins found in bovine whey are β-lactoglobulin (3.2–4.0 g/L), α-lactalbumin (1.2–1.5 g/L); serum albumin (0.3–0.6 g/L), lactoferrin (0.05–0.2 g/L), immunoglobulins (0.8 g/L), and in smaller proportion the proteose-peptone fraction (Harris & Sommers, Citation1968). Since whey do not contain all the necessary nutrients for the developing of lactic acid bacteria, it is necessary to supplement it with yeast extract (Amrane & Prigent, Citation1998). Previous reports indicate that supplementation of whey permeate with yeast extract had a significant effect on lactic acid concentration, volumetric productivity, and substrate conversion, but not on lactic acid yield when Lactobacillus helveticus was used (Anema, Siew, & Klostermeyer, Citation2006). Methodologies, such as the response surface methodology, have been developed to determine the effect of culture medium ingredients, pH, and agitation rate on the growth, viability, and metabolite production of different microorganisms (Guo et al., Citation2012; Jeong & Kim, Citation2009). In the case of probiotics, the optimization of biomass and lactic acid production has been reported (Annan et al., Citation2008). However, to our knowledge, no reports on the optimization of the expression of probiotic characteristics has been published.
In the present study, response surface methodology (RSM) was used in the attainment of the best culture conditions (medium components, agitation rate, and pH) for maximal growth and tolerance to gastric pH and bile salts presence for L. fermentum K73. RSM is the method of choice for optimization in biotechnology and food science (Oyedeji et al., Citation2018). Therefore, the aim of this study was the preparation of a culture medium based on whey and yeast extract that enhances the growth and the probiotic properties of L. fermentum strain K73 so it can be used in the functional food industry.
Materials and methods
Microorganism
Lactobacillus fermentum K73 (GenBank KP784433) was previously isolated and characterized in vitro as a potentially probiotic bacterium (Corzo & Gilliland, Citation1999; Cueto-Vigil et al., Citation2010). The bacterial culture was grown in Man, Rogosa, and Sharpe (MRS) broth (Scharlau Microbiology, Barcelona, Spain) and mixed with 30% glycerol as a cryoprotectant agent. Vials with 3 mL aliquots were frozen in liquid nitrogen and kept at −70°C until used (Cueto-Vigil et al., Citation2010). The carbohydrate fermentation and assimilation pattern of the microorganism was recorded using the API 50 CH test (BioMerieux, Craponne, France).
Culture medium preparation
A culture medium based on sweet whey and yeast extract was developed. Sweet whey powder with the following composition: protein 11.67% (w/w), lipids 2.0% (w/w), lactose 51.64% (w/w), and ash 10.9% (w/w), was provided by a local food company in Sopó, Colombia. Yeast extract was purchased from Oxoid Ltd. (Basingstoke, UK). The proportions of the both ingredients, agitation rate and pH, for each run in the experimental design, are shown in . The culture medium was autoclaved at 121°C for 15 min and brought to 25°C for each run.
Table 1. Factors and response variables for response surface optimization design of the culture medium.
Tabla 1. Factores y variables de respuesta para el diseño de optimización de superficie de respuesta para el medio de cultivo.
Batch fermentation was performed in a 1 L bioreactor (Bioflo 110, New Brunswick Scientific Co. Inc., NJ, U.S.A.), which had a workload of 0.8 L. The fermentation temperature was kept constant at 37 ± 2°C. During culturing, microbial counts were taken every hour up to 10 h. Each reactor was inoculated with L. fermentum K73, 10% (v/v), previously grown in MRS broth at a final concentration of approximately 6.27 ± 0.34 Log10 CFU/mL.
The viable cell count (plate count technique) was performed immediately after inoculation and during (every hour) and at the end of the fermentation process (García-Cayuela et al., Citation2014). The growth curves were analyzed using the web version of DMFit (http://modelling.combase.cc/DMFit.aspx) (Pan et al., Citation2014). The Lag phase was assessed by adjusting the growth curves from the runs to the Baranyi and Roberts model (Pan et al., Citation2014).
Experimental design of the culture medium
The design of the culture medium was done using a D-optimal response surface design using the software Design Expert version 7.0.0 (Stat-Ease Inc., Minneapolis, MN, U.S.A.). The design included 23 runs with five replicates of the central point. The numerical factors were: whey concentration (40–80 g/L) and yeast extract concentration (1.88–25 g/L). Two-level categorical factors were proposed: acidic (5.5) and neutral (7.0) pH, and low (100 rpm) and high (300 rpm) agitation rate (). The ranges were selected according to the conditions used by different authors (Aguirre-Euzkariatza et al., Citation2010; Alonso, Herrero, Rendueles, & Díaz, Citation2014; Brinques, Do Carmo Peralba, & Ayub, Citation2010; Bulatović, Rakin, Vukašinović-Sekulić, Mojović, & Krunić, Citation2014; Rodríguez-Bernal, Serna-Jiménez, Uribe-Bohórquez, Klotz, & Quintanilla-Carvajal, Citation2014; Schepers, Thibault, & Lacroix, Citation2006). Individual effects and interactions between the numerical and categorical factors were evaluated using as responses the final cell count (Log10 CFU/mL), the lag phase duration (h), and the decrease in population in gastric pH conditions and in the presence of bile salts. The last two response variables were selected to evaluate the possible effect of the whey in the microorganism capacity to tolerate the gastrointestinal conditions. RSM was used in the experimental design to determine the optimal concentrations of sweet whey and yeast extract as well as the optimal medium pH and agitation rate.
Optimal concentrations were determined by using as a goal the maximization of the final cell count using the criterion of desirability. The criterion of desirability is a general approach for use in the optimization of multiple responses. A desirability function transforms the values of a response so they fall in the interval (0,1) where 0 stands for a non-acceptable value of the response and 1 for values where higher/lower (depending on the kind of optimization, maximization or minimization) values of the response are satisfactory (Hamme, Sannier, Piot, Didelot, & Bordenave-Juchereau, Citation2009; Henao-Ardila, Quintanilla-Carvajal, Klotz-Ceberio, & Serna-Jiménez, Citation2015). These optimal culture medium values were validated experimentally in the same bioreactor. All runs were performed in triplicate, and the regression analyses were performed at a significance level of α = 0.05.
Cell count
The cell count of L. fermentum K73 for all proposed experiments was performed by plate counting in MRS agar after culture at 37 ± 2°C for 24 h under aerobic conditions (Ha, Kim, Lee, Kim, & Kim, Citation2003). The cell count was expressed as Log10 CFU/mL for the fermentation process. The change in the bacterial cycles was determined as: Log final CFU/mL (after experiment) – Log initial CFU/mL (after the experiment).
Tolerance to gastrointestinal conditions in vitro
The tolerance to gastric pH was evaluated by taking 100 µL of the fermented culture medium obtained after each run () to inoculate MRS broth adjusted to pH 2.0 with HCl 6 M which was incubated for 2 h at 37°C. The cell count was performed at the beginning and end of the assay using the plate count technique (García-Cayuela et al., Citation2014). The resistance to bile salts was evaluated in a similar way, but the fermented culture medium aliquot was used to inoculate MRS broth with or without 0.3% (w/v) ox gall bile (Sigma-Aldrich, U.S.A.) which was incubated for 2 h at 37°C (Hernández-Ledesma, Ramos, & Gómez-Ruiz, Citation2011). The difference between the counts before and after the 2 h of incubation was calculated to evaluate the cell viability and report as: decrease in population Log10 CFU/mL.
Results
Design of culture medium
The quadratic models for the response variables Final cell count, Lag phase duration, and the change in the bacterial cycles under gastric pH conditions were statistically significant (p ≤ 0.05) with adjusted determination coefficients (R2) of 0.717, 0.8921, and 0.9596, respectively, with non-significant values of lack of fit (p ≤ 0.05). In the case of the resistance to bile salts, a 2FI model was used to fit the experimental data. However, even though the model was significant (p = 0.0139), the adjusted R2 was very low (0.4056) and the lack of fit was highly significant (p < 0.0001) so the model was not reliable and therefore not adequate for fitting these data.
The analysis of variance (ANOVA) of the regression for the response variable Final cell count showed that the first-order agitation coefficient, and the interaction coefficient between the whey and yeast extract concentrations were statistically significant (p ≤ 0.05). The coefficients of the quadratic terms of whey concentration and yeast extract were also statistically significant (p ≤ 0.05). The second-order polynomial equation in terms of coded factors is:
where Y is the predicted response, and A, B, C, and D are the coded values of whey concentration, yeast extract concentration, pH, and agitation rate, respectively.
To determine the behavior of the main ingredients concentrations on the Final cell count, the response surface plot was analyzed (). This diagram is a 3D graphical representation of the effect of the whey and yeast extract concentrations on the response variable. The particular graph for these data is known as a saddle graph and shows a relative maximum for a whey concentration in the interval of 60 and 70 g/L and a yeast extract concentration of around 1.9 g/L. The ANOVA of the regression showed that the interaction coefficient for the two concentration factors is negative indicating an antagonist effect.
Figure 1. Surface response 3D plots for the variables: Final cell count (A), lag phase duration (B), and decrease in the population under acidic pH conditions (C). Average pH and agitation rate over the experimentation interval were kept constant at the experimental average value for these graphs.
Figura 1. Gráficas 3D de superficie de respuesta para las variables: Cuenta celular final (a), Duración de la fase Lag (b) y Disminución de la población bajo condiciones de pH ácido (c). Los valores promedio del pH y la velocidad de agitación en el intervalo de experimentación se mantuvieron constantes en el valor mostrado en cada gráfica.
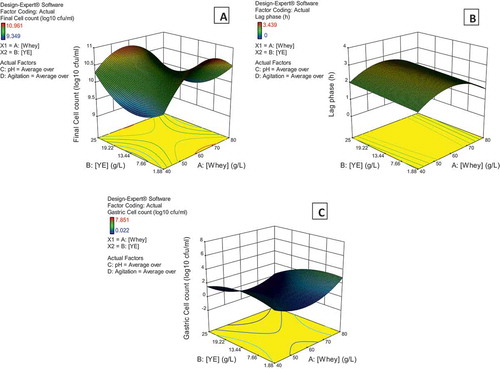
A similar analysis was performed for the response variable Lag phase duration and the quadratic model in terms of coded factors is as follows (only the significant terms are shown):
is the 3D graphical representation of the effect of the whey and yeast extract concentrations on the Lag phase duration shows a relative maximum for a whey concentration of around 60 g/L and a yeast extract concentration of around 1.9 g/L.
In the case of the response variable Change in the bacterial cycles under pH conditions, the quadratic model in terms of coded factors is as follows (only the significant terms are shown):
is the 3D graphical representation of the effect of the whey and yeast extract concentrations on the Decrease in population under acidic conditions and shows a relative maximum for a whey concentration between 70 and 80 g/L and a yeast extract concentration between 19 and 25 g/L.
The models obtained from the analysis of the RSM about the performance of the microorganism L. fermentum K73 in each run, allowed the selection of the optimal culture conditions. presents the statistical parameters of the models for the four responses studied in the culture of Lactobacillus fermentum K73 in submerged culture and shows the results of the numerical optimization of each response.
Table 2. Statistical parameters of the models for the four responses studied in the growth of Lactobacillus fermentum K73 in submerged culture.
Tabla 2. Parámetros estadísticos de los modelos para las cuatro respuestas estudiadas en el crecimiento de Lactobacillus fermentum K73 en cultivo sumergido.
Table 3. Numerical optimization of the individual response variables.
Tabla 3. Optimización numérica de las variables individuales de respuesta.
The simultaneous optimization of the three main responses included the maximization of the Final cell count, minimization of the Lag phase duration and the change in the bacterial cycles under gastric conditions. The software indicated as optimal culture two conditions. The first condition was 40.75 g/L of whey, 25 g/L of yeast extract, a pH of 5.5, and an agitation rate of 100 rpm. The predicted responses were: a Final cell count of 10.59, a Lag phase duration of 0.54, and a change in the bacterial cycles under gastric conditions of −0.692 with a desirability of 0.803. A validation run (triplicate) indicated that when these culture conditions were used: a Final cell count of 12.47 ± 0.073 Log10 CFU/mL, a Lag phase duration of 1.69 ± 0.904 h, and a decrease in cell count under gastric conditions of 3.88 ± 0.09 Log10 CFU/mL were obtained. The Final cell count was far much better than the predicted value and the Lag phase duration and Decrease of cell count under gastric conditions had values similar to the average of the lower and upper limits for these responses (1.71 h and 3.542 Log10 CFU/g).
The second condition was 80 g/L of whey, 1.89 g/L of yeast extract, a pH of 5.5. This medium composition had a desirability of 0.672 and a predicted value of 10.98 Log10 CFU/mL as Final cell count, 1.29 h as Lag phase duration and −2.13 Log10CFU/mL as change in the bacterial cycles under gastric conditions. To validate this prediction, the optimized medium was evaluated. The maximum growth of the microorganism was 9.814 ± 0.180 Log10CFU/mL, the Lag phase duration was 1.302 ± 0.313 h and the change in the bacterial cycles under gastric conditions was −0.80 ± 0.19 Log10CFU/mL.
Discussion
The aim of our study was to find the optimal culture conditions for the production and stress resistance of L. fermentum K73 during fermentation. Whey is also proposed as the principal component of the culture medium due to its proteins composition and the presence of lactose as the sole carbon source.
Design of the culture medium
Cheese whey has been used as a supplement or as culture medium for production of lactic acid bacteria biomass (Rodríguez-Bernal et al., Citation2014; Sasaki, Bosman, & Tan, Citation1995). Previous studies separated the whey components using ultrafiltration and the permeate was used as an alternative to the commercial medium; then, they pasteurized or used short times of exposure to heat treatment the permeate to finally supplement it with yeast extract or other nitrogen source because the low content of nitrogen of whey limit the growth of microorganism (Amrane & Prigent, Citation1994; Schepers, Thibault, & Lacroix, Citation2002). In contrast, we proposed the use of total whey, without additional treatment, plus a sterilization process. The sterilization is a heat treatment (>100 ºC) that induce the irreversible denaturation of β-lactoglobulin and α-lactoalbumin (Amrane & Prigent, Citation1998; Zhang, Liu, Bao, & Zhang, Citation2010), by unfolding and altering the intra-molecular hydrophobic, covalent or other interactions on the protein structure (Didelot, Bordenave-Juchereau, Rosenfeld, Piot, & Sannier, Citation2006). This denaturation may facilitate the acquisition of amino acids from the whey proteins by the microorganism. Due to this, the positive effect of whey on cell growth could be explained by the physical and chemical changes that undergo before being supplied to the microorganism.
However, some studies report that the culture medium with whey protein that has low nitrogen content, limits the growth of lactic acid bacteria, and the supplementation with yeast extract is necessary (Amrane & Prigent, Citation1994). The yeast extract is a mixture of carbohydrates, pyrimidines, vitamins B, peptides, mannitol, and some salts such as K2HPO4, NaCl and MgSO4 (Pan et al., Citation2014; Pinto et al., Citation2015), and these compounds are necessary for cell growth and development. This is reflected in the response surface plot and is why yeast extract is used as a nutritional supplement in different fermentation processes (Altaf, Naveena, Venkateshwar, Kumar, & Reddy, Citation2006).
On the other hand, the heat treatment of the whey is an alternative that facilitates the proteolytic activities of the Lactobacillus fermentum K73. Previous studies have shown that the lactobacilli isolated from milk matrices or dairy products have cell-wall proteolytic systems that help them to obtain exogenous amino acids (De Castro-Cislaghi et al., Citation2012; Guo, Kim, Nam, Park, & Kim, Citation2010). This characteristic may be present in L. fermentum K73 isolated from ‘suero costeño’ (typical Colombian coast food (Cueto-Vigil et al., Citation2010)) so the requirements of yeast extract in the culture medium are lower, because L. fermentum could present proteinase-endopeptidase activities on denatured proteins (Pinto et al., Citation2015).
Microbial growth curves revealed that the stages of cell division were directly related to the microorganism adaptation ability to a new environment, Lag phase. The Lag phase is a reflection of the transition of a microorganism from a commercial culture medium, such as MRS broth, to a media composed of food industrial waste products such as sweet whey (Foligné, Daniel, & Pot, Citation2013). For this reason, it is important to analyze the lag phase and evaluate the impact of the culture medium and the stress it may generate on the production of biomass and the conservation of probiotic properties. shows how a reduction in the concentrations of yeast extract and whey, decreases the adaptation period for L. fermentum K73. The decreasing of the sweet whey concentration, allowed microorganism adaptation to the culture medium, considering the changes in the composition of the original medium, from MRS broth to the formulated medium (Aguirre-Ezkauriatza et al., Citation2010). Although, the culture medium was complex in composition, the cells were able to use it as a substrate. The API CH50 carbohydrate fermentation pattern revealed that this microorganism uses lactose, galactose, and glucose as its main carbon sources.
The positive effect of the sterilized whey could influence the tolerance of the microorganism to gastrointestinal conditions. These proteins are denatured due to the effect of the sterilization temperature. This effect generates hydrophobic and electrostatic interactions and promotes the formation of hydrogen and disulfide bonds. This bonding is observed as aggregation, coagulation, and, finally, precipitation (Anema et al., Citation2006). As a result of this phenomenon, the following hypothesis was proposed. Mass transfer between the microorganisms and the culture medium components may be affected by protein aggregations that were not homogenized by agitation. Aggregates may protect the microorganisms against stress conditions, acting as physical barriers, similar to those found in the encapsulation processes. The whey formed a viscous layer upon the cell wall surface, protecting the microorganism from the osmotic stress generated by the culture medium and reducing the flow of acid across the cell wall and membrane avoiding the acidification of the cytoplasm (Cueto-Vigil & Aragón-Rojas, Citation2012; Martoni, Bhathena, Urbanska, & Prakash, Citation2008). It has been shown (Pan et al., Citation2014) that the interaction between soy proteins and Bifidobacterium longum is hydrophobic in nature so this phenomenon could explain also the interaction between the whey proteins in the culture medium and L. fermentum K73 as described above.
The criterion of desirability was used to estimate the optimal culture conditions to improve the growth and survival of the microorganism. The optimal parameters proposed by this design are comparable with those of other authors who have assessed the appropriate growth conditions for L. fermentum. L. fermentum IMDO 130,101 was isolated from a sourdough matrix and it was determined that its optimum pH for growth was 5.5 (Vrancken, Rimaux, De Vuyst, & Leroy, Citation2008). Similar results were reported for L. fermentum strain F6, which was isolated from koumiss (Zhang et al., Citation2010). This characteristic is typical of fermented foods microbiota; as fermentation generates acids, the microorganisms are forced to adapt to the environment (Simanca, Arteaga, Pérez, Soto, & Salcedo, Citation2010; Yamal, Sharmila, Rao, & Pardha-Saradhi, Citation2013). L. fermentum K73 was isolated from coastal serum (a fermented milk food) at pH 4.96 (Shahbazi et al., Citation2005), which explains its tolerance to acidic conditions close to this value (). This fact suggested that the microorganism developed a different transcriptional response; this includes the positive regulation of H+-ATPase (Al-Sheraji et al., Citation2013).
Finally, the RSM design allows the optimization of resources and generates economic benefits for the industry, as previously reported (Ha et al., Citation2003). These authors determined that the concentration of yeast extract had a 38% of contribution to the lactic acid production. Therefore, the second culture condition proposed by the software could be used in the industrial process because the amount of the yeast extract is low and may decrease the cost production.
It is possible to conclude that the design of a culture medium with RSM allowed for the discovery of the optimal whey concentration, yeast extract concentration, pH level and agitation rate for the growth of L. fermentum K73 and enhancement of the probiotic viability. The culture medium designed from whey was favorable for the growth of L. fermentum K73 and allowed for attaining a very high final cell concentration with a good adaptation to the culture medium and resistance to the stress conditions provided by the gastric environment.
Disclosure statement
No potential conflict of interest was reported by the authors.
Acknowledgments
The authors appreciate the support for this work by the Research Department of the University of La Sabana (grant numbers ING 136-2013). The Ph. D. studies of one of the authors were supported by Colciencias doctoral national scholarship 6172.
Additional information
Funding
References
- Aguirre-Ezkauriatza, E. J., Aguilar-Yáñez, J. M., Ramírez-Medrano, A., & Alvarez, M. M. (2010). Production of probiotic biomass (Lactobacillus casei) in goat milk whey: Comparison of batch, continuous and fed-batch cultures. Bioresource Technology, 101(8), 2837–2844.
- Alonso, S., Herrero, M., Rendueles, M., & Díaz, M. (2014). Physiological heterogeneity in Lactobacillus casei fermentations on residual yoghurt whey. Process Biochemistry, 49(5), 732–739.
- Al-Sheraji, S. H., Ismail, A., Manap, M. Y., Mustafa, S., Yusof, R. M., & Hassan, F. A. (2013). Prebiotics as functional foods: A review. Journal of Functional Foods, 5(4), 1542–1553.
- Altaf, M., Naveena, B. J., Venkateshwar, M., Kumar, E. V., & Reddy, G. (2006). Single step fermentation of starch to l(+) lactic acid by Lactobacillus amylophilus GV6 in SSF using inexpensive nitrogen sources to replace peptone and yeast extract – Optimization by RSM. Process Biochemistry, 41(2), 465–472.
- Amrane, A., & Prigent, Y. (1994). Lactic acid production from lactose in batch culture: Analysis of the data with the help of a mathematical model; relevance for nitrogen source and preculture assessment. Applied Microbiology and Biotechnology, 40(5), 644–649.
- Amrane, A., & Prigent, Y. (1998). Influence of yeast extract concentration on batch cultures of Lactobacillus helveticus: Growth and production coupling. World Journal of Microbiology and Biotechnology, 14, 529–534.
- Anema, S. G., Siew, K. L., & Klostermeyer, H. (2006). Effect of protein, nonprotein-soluble components, and lactose concentrations on the irreversible thermal denaturation of β-lactoglobulin and α-lactalbumin in skim milk. Journal of Agricultural and Food Chemistry, 54(19), 7339–7348.
- Annan, N. T., Borza, A. D., & Hansen, L. T. (2008). Encapsulation in alginate-coated gelatin microspheres improves survival of the probiotic Bifidobacterium adolescentis 15703T during exposure to simulated gastro-intestinal conditions. Food Research International, 41(2), 184–193.
- Brinques, G. B., Do Carmo Peralba, M., & Ayub, M. A. Z. (2010). Optimization of probiotic and lactic acid production by Lactobacillus plantarum in submerged bioreactor systems. Journal of Industrial Microbiology and Biotechnology, 37(2), 205–212.
- Bulatović, M. L., Rakin, M. B., Vukašinović-Sekulić, M. S., Mojović, L. V., & Krunić, T. Ž. (2014). Effect of nutrient supplements on growth and viability of Lactobacillus johnsonii NRRL B-2178 in whey. International Dairy Journal, 34(1), 109–115.
- Calderon Santoyo, M., Loiseau, G., Rodriguez Sanoja, R., & Guyot, J. P. (2003). Study of starch fermentation at low pH by Lactobacillus fermentum Ogi E1 reveals uncoupling between growth and a-amylase production at pH 4.0. International Journal of Food Microbiology, 80(1), 77–87.
- Corzo, G., & Gilliland, S. E. (1999). Bile salt hydrolase activity of three strains of Lactobacillus acidophilus. Journal of Dairy Science, 82(3), 472–480.
- Cueto-Vigil, C., Acuña-Monsalve, Y., & Valenzuela-Riaño, J. (2010). In vitro of probiotic potential of lactic acid bacteria isolated from coastal serum. Actualidades Biológicas, 32(93), 129–138.
- Cueto-Vigil, C., & Aragón-Rojas, S. (2012). Evaluation of probiotic potential of lactic acid bacteria to reduce in vitro cholesterol. Sciencia Agropecuaria, 1, 45–50.
- De Castro-Cislaghi, F. P., Silva, C. D. R. E., Fritzen-Freire, C. B., Lorenz, J. G., Sant’Anna, E. S., Sant’Anna, E. S., & Sant’Anna, E. S. (2012). Bifidobacterium Bb-12 microencapsulated by spray drying with whey: Survival under simulated gastrointestinal conditions, tolerance to NaCl, and viability during storage. Journal of Food Engineering, 113(2), 186–193.
- Didelot, S., Bordenave-Juchereau, S., Rosenfeld, E., Piot, J.-M., & Sannier, F. (2006). Peptides released from acid goat whey by a yeast-lactobacillus association isolated from cheese microflora. The Journal of Dairy Research, 73(2), 163–170.
- Dissanayake, M., Ramchandran, L., Piyadasa, C., & Vasiljevic, T. (2013). Influence of heat and pH on structure and conformation of whey proteins. International Dairy Journal, 28(2), 56–61.
- Foligné, B., Daniel, C., & Pot, B. (2013). Probiotics from research to market: The possibilities, risks and challenges. Current Opinion in Microbiology, 16(3), 284–292.
- García-Cayuela, T., Díez-Municio, M., Herrero, M., Martínez-Cuesta, M. C., Peláez, C., Requena, T., & Moreno, F. J. (2014). Selective fermentation of potential prebiotic lactose-derived oligosaccharides by probiotic bacteria. International Dairy Journal, 38(1), 11–15.
- Guo, C. F., Zhang, L. W., Han, X., Yi, H. X., Li, J. Y., Tuo, Y. F., … Yang, L. (2012). Screening for cholesterol-lowering probiotic based on deoxycholic acid removal pathway and studying its functional mechanisms in vitro. Anaerobe, 18(5), 516–522.
- Guo, X., Kim, J.-M., Nam, H.-M., Park, S.-Y., & Kim, J.-M. (2010). Screening lactic acid bacteria from swine origins for multistrain probiotics based on in vitro functional properties. Anaerobe, 16(4), 321–326.
- Ha, M.-Y., Kim, S.-W., Lee, Y.-W., Kim, M.-J., & Kim, S.-J. (2003). Kinetics analysis of growth and lactic acid production in pH-controlled batch cultures of Lactobacillus casei KH-1 using yeast extract/corn steep liquor/glucose medium. Journal of Bioscience and Bioengineering, 96(2), 134–140.
- Hamme, V., Sannier, F., Piot, J.-M., Didelot, S., & Bordenave-Juchereau, S. (2009). Crude goat whey fermentation by Kluyveromyces marxianus and Lactobacillus rhamnosus: Contribution to proteolysis and ACE inhibitory activity. The Journal of Dairy Research, 76(2), 152–157.
- Harris, R. F., & Sommers, L. E. (1968). Plate-dilution frequency technique for assay of microbial ecology. Applied and Environmental Microbiology, 16(2), 330.
- Henao-Ardila, A., Quintanilla-Carvajal, M. X., Klotz-Ceberio, B., & Serna-Jiménez, J. (2015). Evaluation of inhibition of the Candida intermedia due to the effect of supernatants from bioprotector and their synergistics effect. Revista Mexicana de Ingeniería Química, 14(2), 373–381.
- Hernández-Ledesma, B., Ramos, M., & Gómez-Ruiz, J. Á. (2011). Bioactive components of ovine and caprine cheese whey. Small Ruminant Research, 101(1–3), 196–204.
- Jeong, I. J., & Kim, K. J. (2009). An interactive desirability function method to multiresponse optimization. European Journal of Operational Research, 195(2), 412–426.
- Lin, W.-H., Hwang, C.-F., Chen, L.-W., & Tsen, H.-Y. (2006). Viable counts, characteristic evaluation for commercial lactic acid bacteria products. Food Microbiology, 23(1), 74–81.
- Martoni, C., Bhathena, J., Urbanska, A. M., & Prakash, S. (2008). Microencapsulated bile salt hydrolase producing Lactobacillus reuteri for oral targeted delivery in the gastrointestinal tract. Applied Microbiology and Biotechnology, 81(2), 225–233.
- Morelli, L, & Capurso, L. (2012). Fao/who guidelines on probiotics 10 years later. Journal Of Clinical Gastroenterology, 46, S1-S2.
- Oyedeji, A.B, Mellem, J.J, & Ijabadeniyi, O.A. (2018). Improvement of some quality attributes of soymilk through optimization of selected soybean sprouting parameters using response surface methodology. Cyta - Journal Of Food, 16, 230-237.
- Pan, Q., Zhou, B., Liu, L., Wang, L., Yuan, F., & Gao, Y. (2014). The aggregation of soy protein isolate on the surface of Bifidobacterium. Food Research International, 64, 323–328.
- Pinto, S., Fritzen-Freire, C., Benedetti, S., Murakami, F., Petrus, J. C., Prudêncio, E., & Amboni, R. (2015). Potential use of whey concentrates and prebiotics as carrier agents to protect Bifidobacterium-BB-12 microencapsulated by spray drying. Food Research International, 67, 400–408.
- Rodríguez-Bernal, J. M., Serna-Jiménez, J. A., Uribe-Bohórquez, M. A., Klotz, B., & Quintanilla-Carvajal, M. X. (2014). Aplicación de la metodología de superficie de respuesta para evaluar el efecto de la concentración de azúcar y de cultivos iniciadores comerciales sobre la cinética de fermentación del yogurt. Revista Mexicana de Ingeniería Química, 13(1), 213–225.
- Sasaki, M., Bosman, B. W., & Tan, P. S. (1995). Comparison of proteolytic activities in various lactobacilli. The Journal of Dairy Research, 62(4), 601–610.
- Schepers, A., Thibault, J., & Lacroix, C. (2002). Lactobacillus helveticus growth and lactic acid production during pH-controlled batch cultures in whey permeate/yeast extract medium. Part I. multiple factor kinetic analysis. Enzyme and Microbial Technology, 30(2), 176–186.
- Schepers, A., Thibault, J., & Lacroix, C. (2006). Continuous lactic acid production in whey permeate/yeast extract medium with immobilized Lactobacillus helveticus in a two-stage process: Model and experiments. Enzyme and Microbial Technology, 38(3–4), 324–337.
- Shahbazi, A., Mims, M., Li, Y., Shirley, V., Ibrahim, S., & Morris, A. (2005). Lactic acid production from cheese whey by immobilized bacteria. Applied Biochemistry and Biotechnology, 122, 529–540.
- Shene, C., & Bravo, S. (2007). Whey fermentation by Lactobacillus delbrueckii subsp. bulgaricus for exopolysaccharide production in continuous culture. Enzyme and Microbial Technology, 40(6), 1578–1584.
- Simanca, M., Arteaga, M., Pérez, Y., Soto, M., & Salcedo, J. (2010). Characterization and study of spontaneous fermentation of fermented milk product (suero costeño) produced in Monteria. Revista MVZ Córdoba, 15(1), 1944–1953.
- Vrancken, G., Rimaux, T., De Vuyst, L., & Leroy, F. (2008). Kinetic analysis of growth and sugar consumption by Lactobacillus fermentum IMDO 130101 reveals adaptation to the acidic sourdough ecosystem. International Journal of Food Microbiology, 128(1), 58–66.
- Yamal, G., Sharmila, P., Rao, K. S., & Pardha-Saradhi, P. (2013). Yeast Extract Mannitol medium and its constituents promote synthesis of Au nanoparticles. Process Biochemistry, 48(3), 532–538.
- Zhang, Y., Liu, Y., Bao, Y., & Zhang, H. (2010). influence of ph, heat and enzymatic treatments on the activity of antibacterial substance in MRS and Milk Media produced by Lactobacillus fermentum F6. Agricultural Sciences in China, 9(6), 911–920.