ABSTRACT
An aqueous protocol was developed for nattokinase purification under scalable conditions using a combination of salting out, anion exchange chromatography and ultra-filtration. The enzyme was firstly washed off from the fermented soybeans. The whole proteins were precipitated by ammonium sulfate and purified by anion exchange chromatography. The purified enzyme was finally refined by size exclusion chromatography and ultra-filtration at laboratory and industry-compatible level, respectively. There was a 476.18-fold increase in enzymatic activity with a 48.3% yield at the laboratory level and 429.75-fold increase in enzymatic activity with a 42.7% yield at the industrial-compatible scale. The protocol was universally adaptive for purifying the fibrinolytic enzymes produced by bacillus tequilensis, bacillus amyloliquefaciens, and bacillus cereus, showing a purification efficiency of 329.76-fold with 42.7% yield, 221.78-fold with 32.5% yield, and 288.56-fold with 38.7% yield, respectively. All procedures are scalable, aqueous, simple, cost-effective, and suitable for fibrinolytic enzymes industrial production.
RESUMEN
Se desarrolló un protocolo acuoso para la purificación de nattoquinasa en condiciones escalables, utilizando para ello una combinación de precipitación salina, cromatografía de intercambio aniónico y ultrafiltración. Primero se lavó la enzima de las semillas de soya (soja) fermentadas. Las proteínas enteras se precipitaron con sulfato de amonio, para ser purificadas mediante cromatografía de intercambio aniónico. Finalmente, la enzima purificada fue refinada empleando cromatografía de exclusión por tamaño y ultrafiltración a nivel de laboratorio y compatible con la industria. A nivel de laboratorio se constató un aumento de la actividad enzimática en 476.18 veces, con un rendimiento de 48.3%; en la escala compatible con la industria se registró un aumento de la actividad enzimática en 429.75 veces, con un rendimiento de 42.7%. El protocolo fue universalmente adaptable a la purificación de enzimas fibrinolíticas producidas por Bacillus tequilensis, Bacillus amyloliquefaciens y Bacillus cereus, mostrando una eficiencia de purificación de 329.76 veces con un rendimiento de 42.7% para la primera, de 221.78 veces con un rendimiento de 32.5% para la segunda y de 288.56 veces con un rendimiento de 38.7% para la tercera. Todos los procedimientos son escalables, acuosos, simples, rentables y adecuados para la producción industrial de enzimas fibrinolíticas.
1. Introduction
Thrombosis is a kind of disease that is seriously harmful to human health and life. It is closely related to the level of thrombus in the blood, the greater chance of thrombus formation in vessel, the higher content of fibrinogen in plasma (Lipinski & Pretorius, Citation2013). Fibrinogen is a unique protein that is converted into an insoluble fibrin in a single enzymatic event, which is a characteristic feature of fibrinogen due to its susceptibility to fibrinolytic degradation and dissolution (Kieliszek & Lipinski, Citation2018). Nattokinase is a potent fibrinolytic enzyme that has a dual function of hydrolyzing blood thrombin directly and is involved in the conversion of plasminogen into plasmin with enzymatic activity to degrade fibrin (Liu et al., Citation2015). The pure nattokinase prepared for research purposes is a white powder with no smell; however, currently available commercial dietary nattokinase is usually not pure enough to eliminate the unique smell that is considered repulsive to most non-Japanese people. Current enzyme purification protocols mainly involve the combination of many steps of organic solvent fractionation, salting out, and several chromatography steps, which all suffer from the drawbacks of long production time, high number of operational steps, low selectivity, low activity, low recovery, and low productivity (Singh, Kumar, Mittal, & Mehta, Citation2016). These limitations have resulted in its under-exploitation, preventing the further development of high-quality nattokinase-formulated functional foods and clinical applications. Nattokinase was first purified from the Japanese traditional food natto in 1987 and was produced by the bacterium Bacillus subtilis natto, which is used to ferment the soybean into natto (Sumi, Hamada, Tsushima, Mihara, & Muraki, Citation1987). Since the isolation of nattokinase, several bacteria were identified to have the ability to produce similar fibrinolytic enzymes, such as Bacillus subtilis A1, Bacillus amyloliquefaciens, and Bacillus firmus NA-1 (Jeong et al., Citation2004; Peng, Huang, Zhang, & Zhang, Citation2003; Seo & Lee, Citation2004). Therefore, members of the genus Bacillus are generally considered good sources of fibrinolytic enzyme (Chang et al., Citation2000; Wang et al., Citation2009; Yin, Lin, & Jiang, Citation2010). With respect to nattokinase purification, most studies have focused on the identification of the fibrinolytic enzymes similar to nattokinase rather than on the purification protocol. The high purity level required for the enzyme identification results in low recovery and low yields of purification efficiency, leading to the slow progression of the development of a protocol that is suitable for application at the industrial-compatible scale while achieving high purity. The biochemical characteristic determination results indicated many of these enzymes have a high homogeneity in amino acid sequences but with marked differences in biochemical characteristics (Peng, Yang, & Zhang, Citation2005). As we have known, when the homology of enzymes sequence is more than 50%, the enzymes share the same overall folding (Chothia & Lesk, Citation1986). Owing to the high similarity of folding, it might be possible to develop a common strategy in purification that maintains high efficiency and yield. The developed strategy would promote the research and industrial application of these enzymes. The protocol developed in this study was designed to meet two objectives. The first was to develop a protocol for nattokinase purification at industrial level, and simultaneously maximizing yield while minimizing the operational steps. The second objective was applied and modified this protocol to the fibrinolytic enzymes produced by other Bacillus species to make it universally adaptive to purify these fibrinolytic enzymes.
2. Materials and methods
2.1. Chemicals and reagents
Fibrinogen, thrombin, urokinase, sodium carbonate, sodium bicarbonate, sodium chloride, hydrochloric acid, and precision plus protein standard were purchased from Sigma-Aldrich, St. Louis, Missouri, USA. XK 50–30 empty column, Q-Sepharose HP (1.6 × 5 cm) and Sephacryl-100 (1.6 × 60 cm) pre-packed chromatography column were purchased from GE Healthcare (Uppsala, Sweden). Bacillus subtilis natto (No. 63501) and Bacillus tequilensis (No. 11462) were obtained from the Chinese General Microbiological Culture Collection Center (CGMCC), China. Bacillus amyloliquefaciens and Bacillus cereus were isolated from soil of Zhuhai area, Guangdong Province, China, the 16S rRNA sequence of Bacillus amyloliquefaciens has the highest similarity of 99% with the sequence of Bacillus amyloliquefaciens strain BCRC (Accession number: NR_116022.1), the 16S rRNA sequence of Bacillus cereus has the highest similarity of 100% with the sequence of Bacillus cereus strain CCM2010 (Accession number: NR_115714).
The ultra-filtration centrifuge tubes (8 kDa and 30 kDa) were purchased from Merck Millipore (Darmstadt, Germany). Ultra-filtration at industrial-compatible level was performed on the bench-top quick ultra-filtration system (GE Healthcare, Uppsala, Sweden). All purification operations were performed on the AKTA purify platform (GE Healthcare, Uppsala, Sweden).
2.2. Production of nattokinase by Bacillus subtilis
The obtained strain, Bacillus subtilis natto was grown in Luria-Bertani broth medium at our laboratory, after 2 days of incubation at 37°C, one loop of the strain was transferred from Luria-Bertani broth medium to a solid medium containing 40 g soybean, 4 g MgCl2, and 20 mL of distilled water by an inoculating loop. After 72 h of aerobic and still incubation at 32°C, the fermented soybean was collected for further fibrinolytic enzyme purification.
2.3. Development of the purification protocol for nattokinase
Four grams of fermented soybean was dissolved in 40 mL of deionized water and centrifuged at 10,000 × g for 10 min to remove the solid debris. The enzyme was precipitated with ammonium sulfate (75% saturation), stored at −20°C for 48 h, and then dissolved in 10 mL of buffer A (0.03 M Na2CO3/NaHCO3, pH = 9.2). The insoluble polysaccharides were removed by centrifugation at 6500 × g for 10 min. The crude enzyme solution was dialyzed against buffer A using an 8-kDa membrane for 24 h. After dialysis, the crude enzyme solution was applied to a Q-Sepharose HP column (1.6 × 5 cm) pre-equilibrated with buffer A for a 17 column volume (CV), and the elution was performed at a rate of 2 mL/min by buffer A for a 5 CV followed by a linear gradient from buffer A to buffer B (buffer A containing 1 M NaCl) for a 3 CV. Fractions with fibrinolytic activity were collected and concentrated to 2.5 mL, and were then applied to a Sephacryl-100 column (1.6 × 60 cm) that was pre-equilibrated with buffer A for 1 CV. Elution was performed at 0.5 mL/min for 1 CV, and the fraction showing fibrinolytic activity was collected and lyophilized.
2.4. Four-fold and sixty-fold scale-up protocol for nattokinase purification
A 20 g sample of fermented soybean was dissolved in 200 mL of deionized water, and the suspension was filtrated by gauze to remove the solid debris. The enzyme was precipitated with ammonium sulfate (75% saturation), stored at −20°C for 48 h, and then dissolved in 200 mL buffer A. The sample was then centrifuged at 6500 ×g for 10 min to remove the insoluble polysaccharides. The crude enzyme solution was ultra-filtrated using a bench-top quick membrane separation system (GE Healthcare) with the 8-kDa membrane to remove the residual (NH4)2SO4 and concentrate the sample to a volume of 12 mL. The concentrated samples were then applied to a series connection of 4 Q HP columns (1.6 × 5 cm). The columns were pre-equilibrated with buffer A for a 17 CV, and the elution was performed at a rate of 2 mL/min by buffer A for a 5 CV followed by a linear gradient from buffer A to buffer B (buffer A containing 1 M NaCl) for a 3 CV. The fractions with specific activity were collected and concentrated. The concentrated sample was applied to a sephacryl-100 column (1.6 × 60 cm) to refine the final product. The column was pre-equilibrated with buffer A for 1 CV. Elution was performed at 0.5 mL/min for 1 CV, and the fraction showing fibrinolytic activity was collected and lyophilized.
A 160 g sample of fermented soybean was dissolved in 1.6 L of deionized water, and the suspension was filtrated by gauze to remove the solid debris. The enzyme was precipitated with ammonium sulfate (75% saturation), stored at – 20°C for 48 h, and then dissolved in 200 mL buffer A. The sample was then centrifuged at 6500 × g for 10 min to remove the insoluble polysaccharides. The crude enzyme solution was ultra-filtrated using a bench-top quick membrane separation system (GE Healthcare) with the 8-kDa membrane to remove the residual (NH4)2SO4 and concentrate the sample to a volume of 100 mL. An XK 50–30 column (GE Healthcare) was used to prepare the samples for large-scale Q HP chromatography. The samples were then loaded onto the column containing 300 mL (50 × 16 cm) of Sepharose that was previously equilibrated with buffer A for 17 CV at a flow rate of 5 mL/min after equilibration, and then eluted by buffer A for 5 CV followed by a linear gradient from buffer A to buffer B in 3 CV. The fraction showing fibrinolytic activity was collected. The maximum speed of the AKTA purifier system is 10 mL/min. In consideration of the reliability of the purifier system and column, the sample was eluted at half the maximum speed. The collected fractions were concentrated and applied to a sephacryl-100 column (1.6 × 60 cm) that was pre-equilibrated with buffer A for 1 CV. Elution was performed at 0.5 mL/min for 1 CV, and the elution peaks were recorded for purity analysis.
2.5. Nattokinase purification at scale-up level
Since size exclusion chromatography would not be suitable for large-scale purification, an ultra-filtration step using bench-top quick ultra-filtration system (GE Healthcare, Sweden) with 25-kDa pore size membrane (GE Healthcare, Sweden) was introduced in place of the size exclusion chromatography step. The filtration was collected and lyophilized for the increase of concentration and activity determination.
2.6. Verification of purification efficiency
The purification efficiency was verified with gel filtration chromatography and sodium dodecyl sulfate polyacrylamide gel electrophoresis (SDS-PAGE). The final enzyme refined by ultra-filtration was dissolved in buffer A and applied to a sephacryl-100 gel filtration column (1.6 × 60 cm) pre-equilibrated with buffer A, and eluted with the same buffer at a flow rate of 0.5 mL/min; the elution of enzyme was monitored and the fraction with the peak enzyme level was further analyzed for its purity. SDS-PAGE was performed using a 5% stacking gel and 12% separating gel according to the method described by Waśko, Kieliszek, and Targoński (Citation2012). Twenty microliters of refined enzymes was loaded into the gel, and the gel was finally stained with 0.25% Coomassie Blue R-250 for visualization of the bands and analysis.
2.7. Determination of total protein, enzyme concentration and specific activity
The protein concentration was determined by the bicinchoninic acid (BCA) method using BSA as the standard (Smith et al., Citation1985). The enzymatic activity was measured in 30 mM sodium carbonate/sodium bicarbonate buffer (pH = 10.5) at 45°C for 6 h and the specific activity was determined by the fibrin plate method (Astrup & Müllertz, Citation1952). Ruskinese was used as the standard, and the diameter of the fibrin zone showing clear solution was accurately determined. The results of two perpendicular diameters were recorded to calculate the enzyme activity.
2.8. Purification of fibrinolytic enzymes from other bacillus species
Bacillus tequilensis, Bacillus amyloliquefaciens, and Bacillus cereus were cultured in the same medium described in production of nattokinase section, and fibrinolytic enzyme purified using the same analytical-scale methods as described in development of the purification protocol used for nattokinase.
3. Results
3.1. Nattokinase purification at analytical level
Nattokinase was collected as the unbound fraction from 9 mL to 22.5 mL when purified by Q HP chromatography (). At the refinement phase, there were three peaks revealed from the sephacryl-100 chromatography (), with the retention volume of middle peak showing specific activity at 70 mL, matching the molecular weight of 27–28 kDa according to the instruction of the manufacturer. The purification efficiency showed a 476.18-fold increase in activity and 48.3% yield, with specific activity of 2618.83 U/mg ().
Table 1. Purification efficiency for nattokinase at different scale.
Tabla 1. Eficiencia de purificación para la nattoquinasa a diferentes escalas.
Figure 1. Elution profile of nattokinase by Q-HP chromatography at analytical scale; the fraction with specific activity was collected from 8 to 23 mL as unbound part (a), and elution profile of sephacryl-100 chromatography by the analytical level (b).
Figura 1. Perfil de elución de la nattoquinasa por cromatografía Q-HP a escala analítica; la fracción con actividad específica se recogió de 8–23 ml como parte no unida (a), y el perfil de elución de la cromatografía en Sefacril-100 según el nivel analítico (b).
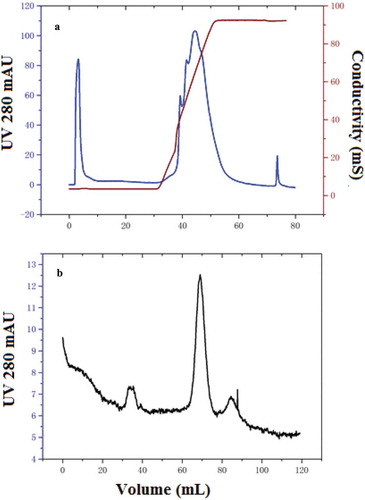
3.2. Nattokinase purification by four-fold amplification protocol
The elution profile of Q HP chromatography was similar to that obtained at the analytical level when amplified by four-fold (). The activity fraction was collected from 35 mL to 90 mL as the unbound part. The majority of the residual polysaccharides of the sample were absorbed by the column, when refined by sephacryl-100 chromatography; the retention volume of the peak showed specific activity was at 70 mL (). The final purified enzyme showed a 443.32-fold increase in activity and a 43.9% yield (), with the purification results consistent with analytical level.
Figure 2. Elution profile of nattokinase by Q-HP chromatography at four and sixtyfold amplification; at fourfold, the fraction with specific activity was collected from 35 to 90 mL as unbound part (a), and elution profile of sephacryl-100 chromatography (b), at sixty fold, fraction with specific activity was collected from 550 to 1450 mL as unbound part (c), and elution profile of sephacryl-100 chromatography (d).
Figura 2. Perfil de elución de la nattoquinasa por cromatografía Q-HP en amplificación a 4 y 60 veces; a 4 veces se recogieron de 35–90 ml la fracción con actividad específica como parte no unida (a) y el perfil de elución de la cromatografía en Sefacril-100 (b); a 60 veces se recogieron de 550–1 450 ml la fracción con actividad específica como parte no unida (c) y el perfil de elución de la cromatografía en Sefacril-100 (d).
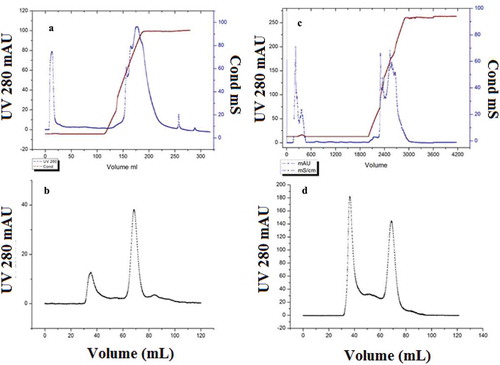
3.3. Nattokinase purification by sixty-fold amplification protocol
Nattokinase was collected from 550 mL to 1450 mL as unbound fraction when purified with Q HP chromatography (). The purity test showed two peaks and the retention volume of the peak showed a specific activity at 70 mL (). After refined by ultra-filtration, the purified enzyme showed a 429.75-fold increase in activity and a 42.7% yield ().
3.4. Purity test for nattokinase
As shown in , only 1 principal peak was observed in the elution profile. The peak was much higher with a greater area than the other minor peaks. Because of the low product amount in the other two peaks, only one band was observed in the SDS-PAGE result (). The results indicated that using ultra-filtration as the refinement method was as effective as size exclusive chromatography.
Figure 3. Purity test of ultra-filtration refined nattokinase which collected from Q-HP chromatography at sixty-fold amplification by sephacryl-100 chromatography (a) and purity test of ultra-filtration refined nattokinase by sodium dodecyl sulfate polyacrylamide gel electrophoresis SDS-PAGE (b).
Figura 3. Prueba de pureza de la nattoquinasa refinada con ultrafiltración, obtenida de la cromatografía Q-HP a una amplificación de 60 veces mediante cromatografía en Sefacril-100 (a) y prueba de pureza de la nattoquinasa refinada con ultrafiltración mediante electroforesis en gel de poliacrilamida con dodecil sulfato de sodio SDS-PAGE (b).
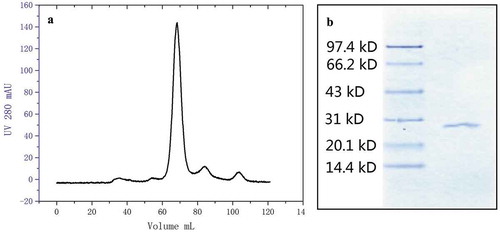
3.5. Fibrinolytic enzyme purification from Bacillus spp. applying the same protocol used for nattokinase purification
The multi-fold-increase in enzyme activity and recovery of other enzymes produced by Bacillus tequilensis and Bacillus cereus were similar to the results obtained for nattokinase. However, there was a relatively prominent decrease in activity and recovery after purification of the enzyme produced by Bacillus amyloliquefaciens (). All of the enzymes produced by this method yielded a single band after SDS-PAGE (), which indicated that the method can be generalized for the purification of other fibrinolytic enzymes produced by bacillus spp.
Table 2. Purification efficiency of fibrinolytic enzymes from Bacillus spp. at analytical level.
Tabla 2. Eficiencia de purificación de enzimas fibrinolíticas de Bacillusspp. a nivel analítico.
Figure 4. Sodium dodecyl sulfate polyacrylamide gel electrophoresis (SDS-PAGE) results of fibrinolytic enzymes from different sources (From left to right: Fibrinolytic enzymes produced by Bacillus cereus, Bacillus amyloliquefaciens, and Bacillus tequilensis).
Figura 4. Electroforesis en gel de poliacrilamida con dodecil sulfato de sodio (SDS-PAGE); resultados de las enzimas fibrinolíticas de diferentes fuentes (de izquierda a derecha: enzimas fibrinolíticas producidas por Bacillus cereus, Bacillus amyloliquefaciens y Bacillus tequilensis).
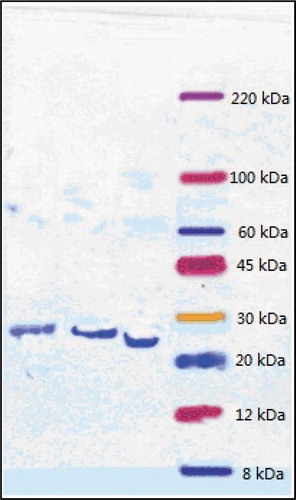
4. Discussion
The composition of a natural enzyme extract is generally quite complex, with thousands of different enzymes mixed together. The traditional method used for nattokinase purification is fractional salting out; however, this method has a quite low purification increase and recovery rate (18–50%) (Fujita et al., Citation1993). Although the salting out method is a primary purification method, it is hard to achieve the yield of pure enzyme required. Many of the other purification methods that have been developed usually rely on a combination of various chromatography columns, and the purification increase and recovery tend to decrease as the number of columns used increases. For example, the purification efficiency was reported to have increased by 4.97-fold with a yield of 6.28% with the combination of a CM FF column, sephadex-G100 column, and sepharose mono Q column (Agrebi et al., Citation2009), and was increased by 61.86-fold with 7.4% yield after using a sephacryl-100 column, CM FF column, and superdex-75 column (Ko, Yan, Zhu, & Qi, Citation2004). The most important drawback of all of these methods is that they are hard to scale up due to the large number of operational steps required and the use of size-exclusion chromatography.
In addition to the column chromatography method described herein, many other separation and purification techniques have been investigated for nattokinase, such as metal ion affinity separation, reverse micelles separation, magnetic polymer microspheres separation, and expanded bed absorption separation. The increase in enzyme activity and yield after purification was 3.9-fold and 80% using the reverse micelles extraction method (Liu, Xing, Chang, & Liu, Citation2006), and 8.7-fold and 85%, respectively when using magnetic polymer beads (Yang, Xing, Guan, & Liu, Citation2006), and 12.3-fold after using expanded bed adsorption with a mixed-mode adsorption technique (though the recovery of the enzyme was not reported in this study) (Lu et al., Citation2005). All of the methods described above are quite costly compared to the traditional chromatographic column method, and the purity of the final product was not sufficiently high according to the purification fold-change.
Another important aspect of the purification of nattokinase and similar fibrinolytic enzymes is the removal of polysaccharides, which usually comprise a large proportion of the metabolic product of bacteria and can easily be combined with enzymes (Ng, Citation1998). Like enzymes, polysaccharides are also electrically charged, and can therefore be absorbed by ion exchange chromatography. The common purification protocol for nattokinase rarely involves the removal of the polysaccharides from the enzyme, resulting in inaccurate determination of the biochemical characteristics. It is known that most of the fibrinolytic enzymes produced by bacillus spp. show high homology with respect to similarity in amino acid sequences; however, their biochemical characteristics are quite different. The working pH range for nattokinase is pH 6–12, whereas those for choggokkinase, subtilisin DFE, and subtilisin QK2 are pH 6–10, pH 7–10.5, and pH 3–12. In general, when the homology of the enzymes’ amino acid sequences is greater than 50%, the enzymes can be said to share the same overall folding structure (Chothia & Lesk, Citation1986). Polysaccharides will effectively enhance the acid endurance of nattokinase; consequently, we hypothesized that if the polysaccharides are not removed, the biochemical characteristics of fibrinolytic enzymes will be inaccurately determined. Thus, the difficulty in polysaccharide removal is a common defect of all of the purification protocols for nattokinase that have been reported to date. The protocol described in this paper is also not sufficient to completely remove all of the polysaccharides, since after absorption by Q HP chromatography, about 20% of the polysaccharides remained in the enzyme. Because polysaccharides cannot be dissolved at low temperature, we tried to freeze the precipitation of (NH4)2SO4 that was salted out, which was then re-dissolved in cold water; therefore, the polysaccharides would be removed as undissolved debris. The results showed that a quite long freezing period was required to achieve this effect, usually 2–7 days under −20°C. Due to the long time needed, direct separation by chromatography could be used to achieve the desired purity level under the specific requirements.
In the purification process of fibrinolytic enzymes, traditional technologies, such as organic solvent fractionation, salting out and various techniques of chromatography were used mainly. The drawbacks of these traditional methods are the requirement of long production time, high number operation units, low selectivity, low activity recovery and low productivity. These together contribute to a high cost of fibrinolytic enzymes production. More importantly, the traditional procedure involves organic solvent extraction and gel chromatography steps, which are not compatible with large industrial production scale as well as drastically decreasing the activity of the enzyme through this kind of multi-step purification procedures. The current protocol is completely aqueous and industrial-compatible, involving only three operational steps. The first is enzyme extraction, obtained by (NH4)2SO4 precipitation and ultra-filtration. The second step is chromatographic purification. In this study, Q HP chromatography was adopted owing to its property of ease in scaling up. The last refinement was finished with a sephacryl-100 size exclusion column at the lab scale and a bench-top ultra-filtration system at the industrial level. The purification efficiency remained consistent when scaled up to a large level. This protocol is not only applicable and effective for the purification of nattokinase but can also be extended to the purification of other fibrinolytic enzymes produced by bacillus spp.
5. Conclusion
The protocol established in this study was completely aqueous and industrial compatible and only three operation units were contained. The fibrinolytic enzymes thus produced will be completely natural, environmentally friendly and cost effective, also is ready to formulate into foods to create a series of fibrinolytic functional foods.
Abbreviations
(NH4)2SO4 ppt, ammonium sulfate precipitation; MgCl2, magnesium chloride; Na2CO3, sodium carbonate; NaHCO3, sodium bicarbonate, NaCl, sodium chloride; BCA, bicinchoninic acid, BSA, bovine serum albumin; QFF, Q Sepharose fast flow ion exchange chromatography; CV, column volume; SDS-PAGE, sodium dodecyl sulfate polyacrylamide gel electrophoresis.
Author Contributions
XX; ZC and BL designed the experiments; XX performed the experiments, analyzed the data, and wrote the paper; RR, ZC and BL critically revised the manuscript.
Acknowledgments
The authors would like to acknowledge a research grant no. R201102 supported by Beijing Normal University-Hong Kong Baptist University United International College, Zhuhai, China.
Disclosure statement
No potential conflict of interest was reported by the authors.
Additional information
Funding
References
- Agrebi, R., Haddar, A., Hmidet, N., Jellouli, K., Manni, L., & Nasri, M. (2009). BSF1 fibrinolytic enzyme from a marine bacterium Bacillus subtilis A26: Purification, biochemical and molecular characterization. Process Biochemistry, 44(11), 1252–1259.
- Astrup, T., & Müllertz, S. (1952). The fibrin plate method for estimating fibrinolytic activity. Archives of Biochemistry and Biophysics, 40(2), 346–351.
- Chang, C. T., Fan, M. H., Kuo, F. C., & Sung, H. Y. (2000). Potent fibrinolytic enzyme from a mutant of Bacillus subtilis IMR-NK1. Journal of Agricultural and Food Chemistry, 48(8), 3210–3216.
- Chothia, C., & Lesk, A. M. (1986). The relation between the divergence of sequence and structurein proteins. The EMBO Journal, 5(4), 823–826.
- Fujita, M., Nomura, K., Hong, K., Ito, Y., Asada, A., & Nishimuro, S. (1993). Purification and characterization of a strong fibrinolytic enzyme (nattokinase) in the vegetable cheese natto, a popular soybean fermented food in Japan. Biochemical and Biophysical Research Communications, 197(3), 1340–1347.
- Jeong, Y. K., Kim, J. H., Gal, S. W., Kim, J. E., Park, S. S., Chung, K. T., … Joo, W. H. (2004). Molecular cloning and characterization of the gene encoding a fibrinolytic enzyme from Bacillus subtilis strain A1. World Journal of Microbiology and Biotechnology, 20(7), 711–717.
- Kieliszek, M., & Lipinski, B. (2018). Pathophysiological significance of protein hydrophobic interactions: An emerging hypothesis. Medical Hypotheses, 110, 15–22.
- Ko, J. H., Yan, J. P., Zhu, L., & Qi, Y. P. (2004). Identification of two novel fibrinolytic enzymes from Bacillus subtilis QK02. Comparative Biochemistry and Physiology Part C: Toxicology & Pharmacology, 137(1), 65–74.
- Lipinski, B., & Pretorius, E. (2013). Iron-induced fibrin in cardiovascular disease. Current Neurovascular Research, 10(3), 269–274.
- Liu, J. G., Xing, J. M., Chang, T. S., & Liu, H. Z. (2006). Purification of nattokinase by reversemicelles extraction from fermentation broth: Effect of temperature and phase volumeratio. Bioprocess and Biosystems Engineering, 28(4), 267.
- Liu, X., Kopparapu, N. K., Shi, X., Deng, Y., Zheng, X., & Wu, J. (2015). Purification and biochemical characterization of a novel fibrinolytic enzyme from culture supernatant of Cordyceps militaris. Journal of Agricultural and Food Chemistry, 63(8), 2215–2224.
- Lu, M. H., Lin, D. Q., Wu, Y. C., Yun, J. X., Mei, L. H., & Yao, S. J. (2005). Separation of nattokinase from Bacillus subtilis fermentation broth by expanded bed adsorption with mixed-mode adsorbent. Biotechnology and Bioprocess Engineering, 10(2), 128.
- Ng, T. B. (1998). A review of research on the protein-bound polysaccharide (polysaccharopeptide, PSP) from the mushroom Coriolus versicolor (Basidiomycetes: Polyporaceae). GeneralPharmacology: the Vascular System, 30(1), 1–4.
- Peng, Y., Huang, Q., Zhang, R. H., & Zhang, Y. Z. (2003). Purification and characterization of a fibrinolytic enzyme produced by Bacillus amyloliquefaciens DC-4 screened from douchi, a traditional Chinese soybean food. Comparative Biochemistry and Physiology Part B: Biochemistry and Molecular Biology, 134(1), 45–52.
- Peng, Y., Yang, X., & Zhang, Y. (2005). Microbial fibrinolytic enzymes: An overview of source, production, properties, and thrombolytic activity in vivo. Applied Microbiology and Biotechnology, 69(2), 126.
- Seo, J. H., & Lee, S. P. (2004). Production of fibrinolytic enzyme from soybean grits fermented by Bacillus firmus NA-1. Journal of Medicinal Food, 7(4), 442–449.
- Singh, R., Kumar, M., Mittal, A., & Mehta, P. K. (2016). Microbial enzymes: Industrial progress in 21st century. 3 Biotech, 6(2), 174.
- Smith, P. E., Krohn, R. I., Hermanson, G. T., Mallia, A. K., Gartner, F. H., Provenzano, M., … Klenk, D. C. (1985). Measurement of protein using bicinchoninic acid. Analytical Biochemistry, 150(1), 76–85.
- Sumi, H., Hamada, H., Tsushima, H., Mihara, H., & Muraki, H. (1987). A novel fibrinolytic enzyme (nattokinase) in the vegetable cheese Natto; a typical and popular soybean food in the Japanese diet. Experientia, 43(10), 1110–1111.
- Wang, C., Du, M., Zheng, D., Kong, F., Zu, G., & Feng, Y. (2009). Purification and characterization of nattokinase from Bacillus subtilis natto B-12. Journal of Agricultural and Food Chemistry, 57(20), 9722–9729.
- Waśko, A., Kieliszek, M., & Targoński, Z. (2012). Purification and characterization of a proteinase from the probiotic Lactobacillus rhamnosus OXY. Preparative Biochemistry and Biotechnology, 42(5), 476–488.
- Yang, C., Xing, J., Guan, Y., & Liu, H. (2006). Superparamagnetic poly (methyl methacrylate) beads for nattokinase purification from fermentation broth. Applied Microbiology and Biotechnology, 72(3), 616.
- Yin, L. J., Lin, H. H., & Jiang, S. T. (2010). Bioproperties of potent nattokinase from Bacillus subtilis YJ1. Journal of Agricultural and Food Chemistry, 58(9), 5737–5742.