ABSTRACT
Polyphenol oxidase (PPO) is responsible for the enzymatic browning of fruits and vegetables, and thus has negative effects on the quality of food. In this study, extraction and purification of PPO from water yam was performed successfully, and the characteristics of PPO and inhibitors of enzyme activity were analysed. The results showed that PPO activity improved by 4.58-fold through the DEAE-Sepharose and Superdex G-75 purification technique, which reached electrophoretic purity. The enzyme molecular weight was estimated to be 32 kDa. The optimum pH and temperature values of PPO were 6.0 and 35°C, respectively, and it was not thermostable at higher temperatures. PPO had the highest substrate affinity towards catechol (Km = 27.82 mmol/L and Vmax = 1.464 △A/min). The most effective inhibitor was L-cysteine followed by ascorbic acid and sodium sulfite.
RESUMEN
La polifenol oxidasa (PPO) es responsable del pardeamiento enzimático de frutas y verduras; por lo tanto, tiene efectos negativos en la calidad de los alimentos. En el presente estudio se extrajo y se purificó con éxito la PPO proveniente del ñame de agua, y se analizaron sus características y los inhibidores de la actividad enzimática. En este sentido, los resultados indicaron que la actividad de la PPO mejoró 4.58 veces al emplear la técnica de purificación DEAE-Sepharose y Superdex G-75, que alcanzó la pureza electroforética. El peso molecular de la enzima se estimó en 32 kDa. Los valores óptimos de pH y temperatura de la PPO fueron 6.0 y 35°C, respectivamente, constatándose que no era termoestable a temperaturas más altas. La PPO mostró mayor afinidad de sustrato hacia el catecol (Km = 27.82 mmol/L y Vmax = 1.464 △A/min). Su inhibidor más efectivo fue la L-cisteína, seguida por el ácido ascórbico y el sulfito de sodio.
1. Introduction
Water yam (Dioscorea alata), a plant resource used both as medicine and food (Liu et al., Citation2012), has already been recognized as a promising source of food nutrients and attracts particular interest in the food industry. As one of the most economically significant tubers of Dioscorea alata, water yam, is widely distributed in tropical and subtropical zones and is consumed worldwide. It is characterized by rich amounts of mucin, micronutrients, phenolic compounds, saponin, vitamins and other functional components (Fang et al., Citation2011), which are considered to have positive effects on health. Fresh-cut technology of fruits and vegetables is the development trend of food processing at present. The development of enzymatic browning on the cut surface induced by mechanical operations is an undesirable phenomenon leading to changes in the appearance and sensory properties of food (Lasekan, Khatib, Juhari, Patiram, & Lasekan, Citation2013; Pristijino, Wills, & Golding, Citation2006). For example, when yam is sold, for the purpose of convenience, cleanliness and rapidity, fresh yam will be selected, cleaned, peeled and cut for consumer consumption (Luo, Wang, Jiang, & Xu, Citation2015). However, yam has a relatively short storage period and is prone to enzymatic browning during processing, which causes changes in colour, flavour and texture, and decreases the commercial value of yams (Saltveit, Citation2000). Therefore, browning has become an urgent problem to tackle in the processing of fresh-cut yams (Teoh, Lasekan, Adzahan, & Hashim, Citation2016a).
Figure 1. Flow chart for the extraction, separation and product preparation of polyphenol oxidase (PPO) from water yam.
Figura 1. Diagrama de flujo para la extracción, separación y preparación del producto de polifenol oxidasa (PPO) a partir del ñame de agua.
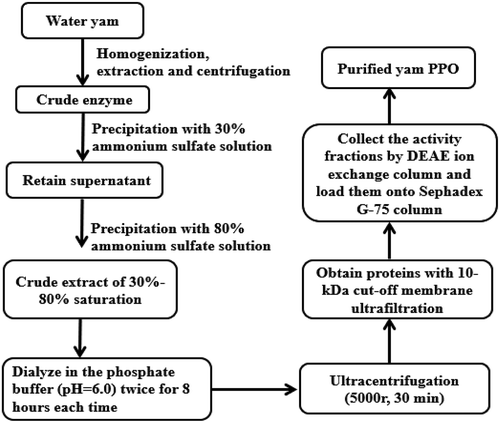
Polyphenol oxidase (PPO) is a general natural multi-copper oxidoreductase and plays significant roles in the metabolic processes of many fruits and vegetables (Mayer, Citation2006). Many studies have shown that enzymatic browning is an undesirable phenomenon during storage and processing of plants food and is mainly initiated by the action of PPO (Muñoz-Pina et al., Citation2018). PPO is released from the cytoplasm when the yam is damaged by inappropriate handling, and it catalyses polyphenols into quinones under aerobic conditions. These quinone compounds can polymerize and/or interact with amino acids and proteins to produce a complex brown pigment (Yoruk & Marshall, Citation2003). Furthermore, enzymatic browning not only affects organoleptic acceptance but also lowers the nutritional value of the food, which directly reduces the quality and marketability of the products. Therefore, enzymatic browning is believed to be one of the great challenges for vegetable and fruit industries (Anaya-Esparza et al., Citation2017; Sikora & Świeca, Citation2018). Several techniques including removing oxygen, heating, and high pressure have been regarded as useful measures to prevent enzymatic browning (Fang, Han, & Ni, Citation2017; Gavirangappa, Harshini, Arugakeerthy, Kulathooran, & Sr, Citation2018; Tinello & Lante, Citation2018). Moreover, the addition of inhibitors, which are non-toxic and highly active at low concentrations, is also an effective way to inhibit the browning reaction (Moon et al., Citation2018). Many common and effective inhibitors of PPO (Holzwarth, Wittig, Carle, & Kammerer, Citation2013) such as ascorbic acid, citric acid, sodium sulfite, phytic acid, sodium chloride and other compounds that have thiol groups (L-cysteine, glutathione) have been reported. More recently, the characterization of PPO has been studied in various plants food such as mung bean sprouts (Sikora & Świeca, Citation2018), bayberry juice (Cao, Cai, Wang, & Zheng, Citation2017), acai fruit (Jesus, Leite, & Cristianini, Citation2018), blueberry (Siddiq & Dolan, Citation2017), tea leaf (Teng et al., Citation2017), soursop nectar (Anaya-Esparza et al., Citation2017), cape gooseberry (Bravo & Osorio, Citation2016). However, few detailed studies have reported on the purification and characterization of water yam PPO.
Therefore, the aim of this study was to develop an improved way to extract and purify PPO from water yam and to explore its enzyme characteristics and the effectiveness of different inhibitors, which could provide a suitable method to reduce browning.
2. Materials and methods
2.1. Materials
Water yams were purchased from the market (Yantai, Shandong Province, China). The water yam tubers were washed adequately and stored at 4°C. Triton X-100, polyethylene glycol 6000 (PEG 6000), catechol, sodium dihydrogen phosphate, sodium hydrogen phosphate, polyvinyl-polypyrrolidone (PVPP), ammonium sulfate, bovine serum albumin (BSA), inhibitors (L-cysteine, phytic acid, ascorbic acid, citric acid, sodium bisulfate, protein markers, sodium chloride) were purchased from Solarbio (Qingdao, China). DEAE Sepharose Fast Flow, Superdex G-75, ÄKTAprime plus were obtained from GE Healthcare. An Agilent 8453E UV-Vis spectrometer (Agilent Technologies GmbH, Karlsruhe, Germany) and refrigerated centrifuge were used for our experiments. All chemical reagents were analytically pure.
2.2. Extraction and purification of PPO
2.2.1. Crude enzyme extraction
The extraction, separation and product preparation of PPO from water yam was shown in . Enzyme extraction was conducted according to Zhou’s method (Zhou, Xiao, Meng, & Liu, Citation2018) with some modifications. The peeled water yam samples (500 g) were frozen for 24 hour and then washed, cut and homogenized for 3 min in a waring blender with 500 mL phosphate buffer (0.1 mol/L, pH 6.0), which contained 0.5% Triton X-100, 10 mg PVPP and 0.34% PEG6000. After extraction for 2 hours in an ice bath, the mixture was filtered and then centrifuged at 4000 g for 20 min at 4°C. The resulting supernatant was fractionated by the ammonium sulfate precipitation method with a 10% concentration increase at each step. The crude extract was 30%-80% saturated as determined by PPO specific activity analysis. The precipitated fractions were re-solubilized in phosphate buffer, and dialyzed (deionized water, 4°C refrigerator) for 24 hour and ultrafiltered through a 10-kDa cut-off membrane. The PPO ultrafiltrate was collected for subsequent purification experiments.
2.2.2. Ion-exchange chromatography using deae-sepharose fast flow
The purification of PPO was based on the method of Liu, Zhao, Wen, and Ni (Citation2015) with modifications. The DEAE-Sepharose Fast Flow column (XK 16/10 column) was equilibrated with 60 mL phosphate buffer (pH 6.0, 0.05 mol/L) and a linear gradient of NaCl from 0.1 to 1.0 mol/L at a flow rate of 1.0 mL/min. 5.0 mL per fraction was collected using a fraction collector tube. The protein and PPO activities in each tube were measured. Each active fraction was pooled and separated through ultrafiltration membranes (10 kDa cut-off).
2.2.3. Gel-filtration chromatography using superdex G-75
The final step of the purification was conducted using the Superdex G-75 column (XK 26/600) chromatography Äkta Purifier system (software Unicorn version 7.0, GE Healthcare Life Science, Germany). The enzyme solution obtained by DEAE-Sepharose was poured into the column pre-equilibrated with 0.1 mol/L phosphate buffer and eluted with the same buffer containing 0.15 mol/L NaCl at a flow rate of 2.0 mL/min. The fractions collected were 5 mL/tube. The collected fractions were separated by an ultrafiltration membrane (10 kDa) and stored at −20°C for further use.
2.3. Polyacrylamide gel electrophoresis
The molecular weight of the purified PPO from yam was determined by SDS-PAGE using Tanon EPS300 electrophoresis with a 5% stacking gel and a 12% separating gel (Solarbio P1200). The protein sample solution was heated for 5 min at 100°C in a bath water with a 4× SDS-PAGE loading buffer (Solarbio P1015, with DTT). The gels were run at a constant voltage of 80 V for approximately 40 min in the stacking gel and at constant voltage of 100 V for approximately 70 min in the separating gel. The protein bands were visualised by the Coomassie Blue staining technique and molecular weight was estimated by a commercial kit containing nine proteins of known molecular weight (10, 17, 26, 34, 43, 55, 72, 95, 130 and 180 kDa).
2.4. Substrate specificity and enzyme kinetics
The scanning range of the PPO catalysed reaction product was from 300 to 900 nm to determine the optimum wavelength. The substrate specificity of the PPO was determined by measuring its activity towards catechol, resorcinol, phloroglucinol, pyrogallic acid at various concentrations of 2–22 mg/mL, respectively. The Michaelis-Menten constant (Km) and maximum reaction velocity (Vmax) were determined for four substrates by plotting 1/activity (1/ν) versus 1/substrate (1/[S]) according to the method of Lineweaver and Burk (Lineweaver & Burk, Citation1934). The optimum substrate and concentration obtained were used in all our research.
2.5. Measurement of PPO activity
The enzymatic activity was assayed according to the method of Zhou (Zhou et al., Citation2018), which was determined by measuring the change in absorbance at 408 nm. The standard reaction consisted of 0.3 mL of PPO solution and 2.7 mL of 14 mg/mL catechol in 0.1 mol/L phosphate buffer (pH 6.0) and the blank sample only contained 3 mL of substrate solution in the same buffer. A unit of PPO activity was defined as the change in absorbance of 0.001 per minute.
2.6. Determination of proteins
Protein content in the PPO extract was determined according to the Bradford procedure using bovine serum albumin (BSA) as a standard reagent (Zor & Selinger, Citation1996). Standard curves were prepared with different volume BSA reagents (0, 0.1 mL, 0.3 mL, 0.5 mL, 0.7 mL and 0.9 mL) and 5 mL dyes, the reaction lasted for 2 minutes and then determination the wavelength.
2.7. Characterization of PPO
2.7.1. Effects of pH on PPO activity
The optimum pH of the purified PPO was determined in 0.1 mol/L phosphate buffer (pH 5.0–7.5) with 14 mg/mL catechol as the substrate. PPO activity was calculated using an Agilent 8453E UV-Vis spectrometer (Agilent Technologies GmbH, Karlsruhe, Germany) and loading a thermostated cell holder with magnetic stirring. The optimum pH of yam PPO obtained was used in all our research.
2.7.2. Effects of temperature on PPO activity
The optimum temperature was designed to range from −18°C to 80°C, The samples at −18 and 0°C were recovered to room temperature before measurements. After balance of the reaction mixture at the testing temperature, PPO was added, then the PPO activity was calculated in the form of percent residual activity.
2.7.3. Thermal inactivation of PPO
Water yam PPO (0.3 mL) in a 5 mL test tube was heated in a water bath and maintained at 60, 70, 80, 90°C. After heating for a designated time (1, 2, 3, 5, 10, 15, 20 and 30 min), the test tubes were immediately put in an ice bath and assayed. The results were also reported in the form of percent residual PPO activity.
2.7.4. Inhibition mechanism of PPO
The inhibitory effects of various inhibitors (L-cysteine, NaCl, NaHSO3, ascorbic acid, citric acid and phytic acid) at a range of concentrations on PPO enzyme activity were tested using 14 mg/mL catechol as the substrate: L-cysteine (0.1–1.2 mg/mL); NaCl (2.5–12.5 mg/mL); NaHSO3 (0.2–1.0 mg/mL); ascorbic acid (0.1–1.0 mg/mL); citric acid (1.0–10.0 mg/mL); phytic acid (0.5–20 mg/mL). Different concentrations of inhibitors were added to the reaction mixture, which contained 0.3 mL inhibitor, 0.3 mL PPO extract and 2.7 mL of 14 mg/mL catechol. Every treatment included a blank sample which contained the substrate and inhibitors without the enzyme solution.
2.8. Statistical analysis
The entire study was replicated two times, and triplicate samples were evaluated for significance (P < .05) between means using the least significant difference (LSD) method. Data were analysed using the General Linear Models procedure of the Statistix 8.1 software package (Analytical Software, St. Paul, MN). Analysis of variance (ANOVA) was performed to determine the significance of the main effects.
3. Results and discussion
3.1. Isolation and purification
The results of purification of PPO from water yam are shown in . Yam PPO was successfully purified to 4.58 purification fold with 3.54 U/100 U total recovery. As can be observed, the specific activity gradually increased to 14292.81 U/mg protein from an initial value of 3120.70 U/mg protein, but the protein content declined after subsequent steps of purification. This could be due to the collection of fractions only at each peak of purification. The overall degree of purification was low, compared with partial purification of Whangkeumbae pear PPO for which 32.93 purification fold was achieved by the same method (Zhou et al., Citation2018). The PPO from the flower buds of Lonicera japonica Thunb was purified 30.76 times with 33.8% recovery after ammonium sulfate and DEAE-cellulose (Liu, Liu, & Wang, Citation2013) while a 6.5 purification fold with 11% recovery was observed for Chinese Parsley PPO (Lin, Ng, & Chen, Citation2016). From the data, it can be concluded that the purification fold and the yield are related to the extraction method, the type of raw materials, and the purification method.
Table 1. Isolation and purification of PPO from yam.
Tabla 1. Aislamiento y purificación de la PPO obtenida del ñame de agua.
Four protein peaks were obtained with an NaCl elution gradient by using the DEAE-Sepharose Fast Flow column ()). The middle two peaks showed the highest yam PPO activity among the four protein peaks. It revealed that the fractions in tubes 10–47, especially tube 17, had the high enzyme activity; the active fractions were collected to determine the degree of PPO purity. The result of the PPO purified using Superdex G-75 filtration is shown in ). The absorbance of the first and last peaks showed no activity at 280 nm, while the middle peak, especially the second peak (tubes 32–61) showed high activity. The pure PPO eluted in the Superdex G-75 separations was used in later experiments.
Figure 2. (a) DEAE-Sepharose fast flow column (absorbance at 280 nm and enzyme activity) elution profiles of yam polyphenol oxidase (PPO). (b) Superdex G-75 filtration fast flow column (absorbance at 280 nm and enzyme activity) elution profiles of yam PPO. (c) Denaturing SDS-PAGE of purified PPO from yam. (Lane 1: Molecular weight marker; Lane 2: Denaturing SDS-PAGE of DEAE enzyme eluate; Lane 3: Denaturing SDS-PAGE of Superdex G-75 enzyme eluate.).
Figura 2. (a) Perfiles de elución de DEAE-Sepharose Fast Flow (absorbancia a 280 nm y actividad enzimática) de la polifenol oxidasa (PPO) obtenida del ñame de agua. (b) Perfiles de elución de la columna Fast Flow de filtración Superdex G-75 (absorbancia a 280 nm y actividad de la enzima) de la PPO obtenida del ñame de agua. (c) Desnaturalización de la PPO purificada a partir de ñame de agua mediante la técnica de SDS-PAG. (Carril 1: Marcador de peso molecular; Carril 2: Desnaturalización del eluato enzimático DEAE mediante la técnica SDS-PAGE; Carril 3: SDS-PAGE Desnaturalización del eluato enzimático Superdex G-75 mediante la técnica SDS-PAGE).
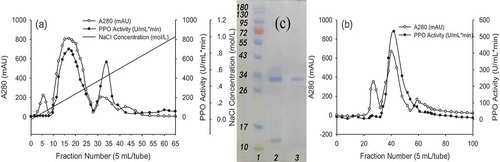
The molecular weight of the purified PPO was confirmed by SDS-PAGE. As shown in ), Lane 2 shows that collected solutions clearly included two protein bands, which indicating that the target PPO had not yet been completely purified. Therefore, the PPO was further purified using the Sephadex G-75 column. The result revealed that only a single major protein band was detected in Lane 3 and it confirmed that taro PPO is a monomeric protein with an estimated molecular weight of 32 kDa, which reached electrophoretic purity and confirmed the effectiveness of the purification procedure through Sephadex G-75 for water yam PPO. The result was similar with that of Chestnut kernel PPO (32.5 kDa) (Gong, Li, Liu, Cheng, & Wang, Citation2015) and Gooseberry PPO (31 kDa) (Bravo & Osorio, Citation2016), but lower than that of Solanum lycocarpum fruit PPO (47 and 68 kDa) (Batista, Batista, Alves, & Fernandes, Citation2014), buriti fruit PPO (65 kDa) (Carvalho & Orlanda, Citation2017), and Bacillus sp PPO (120 kDa) (Zeynep & Mohamed, Citation2013), respectively.
3.2. Parameters for measurement of PPO
As shown in ), wavelengths from 300 to 900 nm were scanned, and the result showed that the strongest wavelength of absorbance was 408 nm, which reached the maximum absorbance value of 1.065. Similarly, PPO activity from blueberry was at 420 nm (Siddiq & Dolan, Citation2017; Terefe, Delon, Buckow, & Versteeg, Citation2015). The highest wavelength of absorbance at 410 nm was automatically assayed for PPO from Fuji apple (Fang, Zhao, Gan, & Ni, Citation2015) and at 412 nm was determined for Whangkeumbae pear (Zhou et al., Citation2018).
Figure 3. Parameters for measurement of polyphenol oxidase (PPO). (a) Scanning at wavelengths of 300–900 nm; (b) the substrate specificity profile; (c) Lineweaver-Burk plot of yam PPO activity measured with different substrates at various concentrations.
Figura 3. Parámetros para la medición de la polifenol oxidasa (PPO). (a) Exploración en longitudes de onda de 300–900 nm; (b) perfil de especificidad del sustrato; (c) Diagrama de Lineweaver-Burk de la actividad de la PPO del ñame de agua medida con diferentes sustratos en diversas concentraciones.
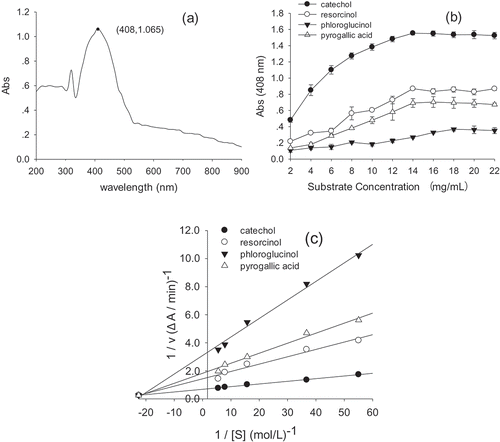
Phenolics are crucial substrates of PPO; diphenol and triphenol compounds were selected to measure yam PPO substrate specificity. As shown in ), yam PPO showed substrate preference for diphenols, with most activity detected with catechol, followed by resorcinol. In contrast, the PPO had low activity towards triphenols such as phloroglucinol and pyrogallic acid. Similar results were reported by Zaini, Osman, Hamid, and Ebrahimpour (Citation2013), which showed extreme preference towards catechol. We can also confirm that increasing the concentration of phenolics led to a linear increase in the absorbance value, reaching maximum value at 14 mg/mL for catechol. The magnitude of the Km reflects the affinity of the enzyme for the substrate, and the substrate with the smallest Km is the “natural” optimum substrate. Km and Vmax were calculated using a Lineweaver-Burk plot with different substrates at various concentrations ()). As the best substrate, the values of Km and Vmax for catechol were 27.82 mmol/L and 1.464 △A/min, which indicated higher affinity than the affinities of resorcinol, phloroglucinol, and pyrogallic acid. The results of this study are in agreement with other studies, which reported that the Km and Vmax values of PPO for Solanum lycocarpum were 6.47 mmol/L and 3.42 U/(mL·min) for catechol, 0.15 mmol/L and 3 U/(mL·min) for 4-methyl catechol, and 20.11 mmol/L and 3.9 U/(mL·min) for pyrogallol (Batista et al., Citation2014).
3.3. pH optimum, temperature optimum and thermostability
Enzymes are extremely sensitive to pH, which would affect PPO’s surface charge, its solubility, conformation and binding-ability with different substrates or inhibitors. The effect of pH on yam PPO activity was determined by using 14 mg/mL catechol as substrate at different pH conditions ranging from 5.0 to 7.5 at 35°C ()). As observed, the optimum pH value was 6.0, which was similar to Iranian medlar fruit using catechol as substrate (Yolmeh & Sadeghi Mahoonak, Citation2016). It was reported that the optimum pH of borage PPO enzyme was 5.0 for 4-methylcatechol, 5.5 for caffeic acid and 7.5 for catechol and pyrogallol (Alici & Arabaci, Citation2016). The optimum pH of cape gooseberry PPO was found to be 5.5 for catechol and 5.0 for chlorogenic acid (Bravo et al., Citation2016). Additionally, the optimum pH of the PPO enzyme from buriti pulp and tea leaf were determined to be pH 7.0 and 8.0 (Carvalho et al., Citation2017; Teng et al., Citation2017). Obviously, the optimal pH for PPO activity varies from approximately 5.0 to 8.0 depending on plant sources, the extraction methods, substrates and enzyme localization. An appropriate pH value is very important for PPO to achieve maximum activity. Perhaps avoiding the optimum pH value is an effective way to prevent browning during food processing.
Figure 4. Effect of pH value (a) on activity of yam polyphenol oxidase (PPO) at 35°C. Effect of temperature on PPO activity (b) and thermostability (c).
Figura 4. Efecto del valor del pH (a) sobre la actividad de la polifenol oxidasa (PPO) del ñame de agua a 35°C. Efecto de la temperatura sobre la actividad de la PPO (b) y la termoestabilidad (c).
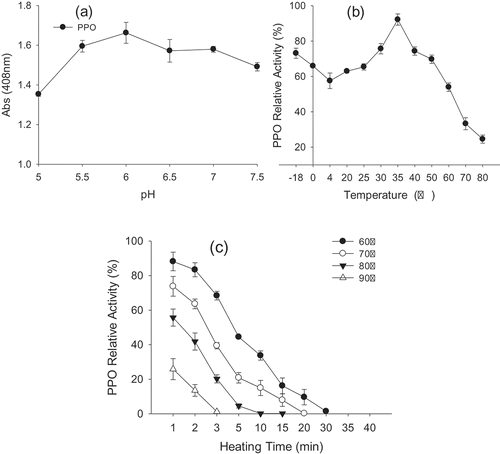
To investigate the optimal temperature stability of PPO, the crude yam extracts were set to temperatures from −18°C-80°C. The samples at −18 and 0°C were recovered to room temperature before measurements. The optimum temperature for yam PPO is 35°C ()). Between −18°C and 35°C, the activity of PPO decreased first and then increased gradually from 4°C to 35°C. Above 35°C, PPO activity declined sharply, the remaining enzyme activity was only 24% at 80°C. This result suggested that temperatures at 4°C or above 60°C were beneficial for inhibiting enzymatic browning of yams during transportation and processing. To evaluate thermostability, PPO activity was measured by incubating the enzyme from 60 to 90°C for 30 min. As seen, the enzyme activities decreased with increase in temperature and incubation time ()). The enzyme retained 44.48%, 20.85%, 4.58% and 0% of its original activity after 5 min of incubation at 60, 70, 80 and 90°C, respectively. Furthermore, PPO was completely inactivated under incubation at 60°C for 30 min, 70°C for 20 min, 80°C for 15 min or 90°C for 3 min.
This result could be due to the increase in temperature enhances the kinetic energy and leads to the reaction occurring rapidly. In agreement with other literature, PPO sources and substrates have different optimum and unstable temperatures. For example, the optimum temperature was 30°C for Desert truffle using catechol as a substrate (Hicham, Christophe, Abdelhafid, &Thibaud, Citation2013), and 40°C for Honeydew peach using catechol as substrate (Liu, Cao, Yang, & Qi, Citation2015). The thermostability of yam PPO was similar to the results of apricot PPO, which loses more than 90% of its activity after incubation at 70°C for 10 min (Derardja, Pretzler, Kampatsikas, Barkat, & Rompel, Citation2011). By contrast, Chinese parsley PPO still had 15% of its activity after incubation at 90°C for 40 min (Lin et al., Citation2016).
3.4. Effect of inhibitors on yam PPO activity
The inhibition of enzymatic browning has been the subject of continuing concern among researchers and the food industry. Several compounds have been investigated for their effectiveness in preventing enzymatic browning in crude extracts of fruit and vegetables (Lim & Chen, Citation2018; Yılmaz & Elmacı, Citation2018). Similarly, in this experiment, six inhibitors were tested for their inhibitory effect against yam PPO (). As can be observed, with the increases in inhibitor concentration, the absorption value decreased in the same reaction time. L-cysteine, a thiol compound, can react with o-quinones to form a stable colourless product, which interferes with browning of enzymes. Therefore, the most effective inhibitor was L-cysteine with only 0.050 absorbance value retained when the concentration was its 1.2 mg/mL ()). Our results are close to those of Liu et al. (Citation2015), who reported that even at low concentrations of 0.1 mM, 75% or more effective inhibitory action occurred. In comparison with L-cysteine, ascorbic acid exhibited a slightly weaker inhibitory action but was better than other reagents. The absorption value decreased greatly when the concentration increased from 0.1 mg/mL to 1.0 mg/mL and the value was lowest at high concentration ()). This is probably because ascorbic acid can act as a reducing agent for quinones, which can interfere with quinones forming phenols and reduce the production of brown pigment (Batista et al., Citation2014). Similarly, Yam slices treated with the ascorbic acid and assisted UV-C dosage of 6.84 kJ m−2 showed significantly low PPO activities (Teoh et al., Citation2016a) and UV-C treated potato slices at 13.68 kJ m−2 dosage level showed significantly higher PPO activities (Teoh, Lasekan, Adzahan, & Hashim, Citation2016b). L-cysteine and ascorbic acid also gave rise to an inhibitory effect on edible yam enzymes as observed by Fujita et al. (Citation2006). Ascorbic acid is also an effective inhibitor for borage (Trachystemon orientalis L.) PPO enzyme (Alici & Arabacia, Citation2016). Our data indicate that sodium bisulfite also has some inhibitory effect on enzymatic browning ()). In summary, the most promising inhibitor was L-cysteine followed by ascorbic acid and sodium sulfite. The inhibitory effect of citric acid ()) and phytic acid ()) were similar to sodium chloride ()) by contrast based on the colour of the results. Therefore, these experiments evaluated the inhibitory effect of different inhibitors on PPO activity and provide the theoretical basis for industrial production.
Figure 5. Effect of different inhibitors on yam polyphenol oxidase (PPO) activity. (a) sodium chloride (NaCl); (b) Sodium bisulfite (NaHSO3); (c) ascorbic acid; (d) citric acid; (e) L-cysteine; (f) phytic acid (pH 6.0; room temperature; catechol concentration, 14 mg/mL); the experimental results for 30 s are pictured above.
Figura 5. Efecto de diferentes inhibidores sobre la actividad de la polifenol oxidasa (PPO) obtenida del ñame de agua. (a) cloruro de sodio (NaCl); (b) bisulfito de sodio (NaHSO3); (c) ácido ascórbico; (d) ácido cítrico; (e) L-cisteína; (f) ácido fítico (pH 6.0; temperatura ambiente; concentración de catecol, 14 mg/ml); los resultados experimentales de 30 s se muestran arriba.
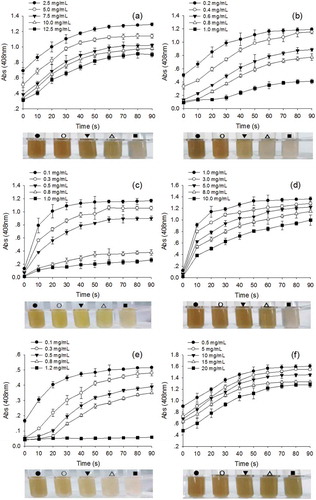
4. Conclusions
PPO was successfully extracted and purified using an extraction buffer, ammonium sulfate precipitation, dialysis, ultrafiltration, DEAE weak anion Fast Flow column and gel-filtration chromatography. Yam PPO was purified to 4.58 purification fold with 3.54 U/100 U total recovery. PPO showed the highest affinity to catechol among all the substrates, and the enzyme molecular weight was estimated to be 32 kDa. The values of Km and Vmax for catechol were 27.82 mmol/L and 1.464 △A/min, respectively. The optimal pH and temperature value for the enzyme with catechol as a substrate were 6.0 and 35°C, respectively. Yam PPO enzyme was not thermostable at higher temperatures, and the most promising inhibitors were L-cysteine followed by ascorbic acid and sodium bisulfite. From these studies, it can be concluded that the treatment of vegetables with a single factor or combination of factors could effectively reduce the effect of browning, thus maintaining the quality of vegetables during processing.
Disclosure statement
No potential conflict of interest was reported by the authors.
Additional information
Funding
References
- Alici, E. H., & Arabaci, G. (2016). Purification of polyphenol oxidase from borage (Trachystemon orientalis L.) by using three-phase partitioning and investigation of kinetic properties. International Journal of Biological Macromolecules, 93(Pt A), 1051–1056. doi:10.1016/j.ijbiomac.2016.09.070
- Anaya-Esparza, L. M., Velázquez-Estrada, R. M., Sayago-Ayerdi, S. G., Sánchez-Burgos, J. A., Ramírez-Mares, M. V., García-Magaña, M. D. L., & Montalvo-González, E. (2017). Effect of thermosonication on polyphenol oxidase inactivation and quality parameters of soursopnectar. LWT - Food Science and Technology, 75, 545–551. doi:10.1016/j.lwt.2016.10.002
- Batista, K. A., Batista, G. L. A., Alves, G. L., & Fernandes, K. F. (2014). Extraction, partial purification and characterization of polyphenol oxidase from Solanum lycocarpum fruits. Journal of Molecular Catalysis B Enzymatic, 102(4), 211–217. doi:10.1016/j.molcatb.2014.02.017
- Bravo, K., & Osorio, E. (2016). Characterization of polyphenol oxidase from Cape gooseberry (Physalis peruviana L.) fruit. Food Chemistry, 197(Pt A), 185–190. doi:10.1016/j.foodchem.2015.10.126
- Cao, X., Cai, C., Wang, Y., & Zheng, X. (2017). The inactivation kinetics of polyphenol oxidase and peroxidase in bayberry juice during thermal and ultrasound treatments. Innovative Food Science & Emerging Technologies, 45, 169–178. doi:10.1016/j.ifset.2017.09.018
- Carvalho, J. D. O., & Orlanda, J. F. F. (2017). Heat stability and effect of pH on enzyme activity of polyphenol oxidase in buriti (Mauritia flexuosa Linnaeus f.) fruit extract. Food Chemistry, 233, 159–163. doi:10.1016/j.foodchem.2017.04.101
- Derardja, A. E., Pretzler, M., Kampatsikas, I., Barkat, M., & Rompel, A. (2011). Purification and characterization of latent polyphenol oxidase from apricot (Prunus armeniaca L). Journal of Agricultural and Food Chemistry, 88(1), 105–110. doi:10.1016/0305-0491(87)90087-3
- Fang, L., Han, Q., & Ni, Y. (2017). Comparison of biochemical properties and thermal inactivation of membrane‐bound polyphenol oxidase from three apple cultivars (Malus domestica Borkh). International Journal of Food Science & Technology, 53(4), 1005–1012. doi:10.1111/ijfs.13676
- Fang, L., Zhao, J. H., Gan, Z. L., & Ni, Y. Y. (2015). Comparison of membrane-bound and soluble polyphenol oxidasein Fuji apple (Malus domesticaBorkh. cv. Red Fuji). Food Chemistry, 173, 86–91. doi: 10.1111/ijfs.13676.
- Fang, Z., Wu, D., Dong, Y., Ye, X., Liu, D., & Chen, J. (2011). Phenolic compounds in Chinese purple yam and changes during vacuum frying. Food Chemistry, 128(4), 943–948. doi:10.1016/j.foodchem.2011.03.123
- Fujita, S., Han, Y. Z., Kouno, C., Matsuo, T., Yamashita, M., Haraguchi, Y., … Yang, C. P. (2006). Purification and characterization of polyphenol oxidase from edible yam (Dioscorea opposita Thunb). Food Science & Technology Research, 12(3), 235–239. doi:10.3136/fstr.12.235
- Gavirangappa, H., Harshini, M., Arugakeerthy, C., Kulathooran, R., & Sr, R. K. S. M. (2018). Effect of adsorbent and acidulants on enzymatic browning of sugarcane juice. Journal of Food Science and Technology, 55(10), 4356–4362. doi:10.1007/s13197-018-3350-4
- Gong, Z., Li, D., Liu, C., Cheng, A., & Wang, W. (2015). Partial purification and characterization of polyphenol oxidase and peroxidase from chestnut kernel. LWT-Food Science and Technology, 60(2), 1095–1099. doi:10.1016/j.lwt.2014.10.012
- Hicham, G., Christophe, D., Abdelhafid, B., & Thibaud, C. (2013). First extraction of polyphenol oxidase from edible desert truffle (Terfezia leonis Tul.) and its thermal behavior. European Food Research & Technology, 237(5), 721–729. doi:10.1007/s00217-013-2040-8
- Holzwarth, M., Wittig, J., Carle, R., & Kammerer, D. R. (2013). Influence of putative polyphenoloxidase (PPO) inhibitors on strawberry (Fragaria x ananassa Duch.) PPO, anthocyanin and color stability of stored purées. LWT - Food Science and Technology, 52(2), 116–122. doi:10.1016/j.lwt.2012.10.025
- Jesus, A., Leite, T. S., & Cristianini, M. (2018). High isostatic pressure and thermal processing of açaí fruit (Euterpe oleracea Martius): Effect on pulp color and inactivation of peroxidase and polyphenol oxidase. Food Research International, 105, 853–862. doi:10.1016/j.foodres.2017.12.013
- Lasekan, O., Khatib, A., Juhari, H., Patiram, P., & Lasekan, A. (2013). Headspace solidphase micro extraction gas chromatography-mass spectrometry determination of volatile compounds in different varieties of African star apple fruit. Food Chemistry, 141, 2089–2097. doi:10.1016/j.foodchem.2013.10.089
- Lim, W. Y., & Chen, W. W. (2018). Inhibitory effect of chemical and natural anti-browning agents on polyphenol oxidase from ginger (Zingiber officinale Roscoe). Journal of Food Science & Technology, 55(8), 3001–3007. doi:10.1007/s13197-018-3218-7
- Lin, H., Ng, A. W. R., & Chen, W. W. (2016). Partial purification and characterization of polyphenol oxidase from Chinese parsley (Coriandrum sativum). Food Science & Biotechnology, 25(1), 91–96. doi:10.1007/s10068-016-0103-x
- Lineweaver, H., & Burk, D. (1934). The determination of enzyme dissociation constant. Journal of the American Chemical Society, 56(3), 658–666. doi:10.1021/ja01318a036
- Liu, F., Liu, N., & Wang, X. (2013). Purification and partial characterization of peroxidase from the flower buds of Lonicera japonica Thunb. Distributed by University of Washington Press., 138(1), 478–483. doi:10.1016/j.foodchem.2012.10.103
- Liu, F., Zhao, J. H., Wen, X., & Ni, Y. Y. (2015). Purification and structural analysis of membrane-bound polyphenol oxidase from Fuji apple. Food Chemistry, 183, 72–77. doi:10.1016/j.foodchem.2015.03.027
- Liu, L., Cao, S., Yang, H., & Qi, X. (2015). Pectin plays an important role on the kinetics properties of polyphenol oxidase from honeydew peach. Food Chemistry, 168, 14–20. doi:10.1016/j.foodchem.2014.07.064
- Liu, S. F., Chang, S. Y., Lee, T. C., Chuang, L. Y., Guh, J. Y., Hung, C. Y., … Hsieh, P. F. (2012). Dioscorea alata attenuates renal interstitial cellular fibrosis by regulating Smad- and epithelial-mesenchymal transition signaling pathways. PloS One, 7(11), 1–8. doi:10.1371/journal.pone.0047482
- Luo, Z., Wang, Y., Jiang, L., & Xu, X. (2015). Effect of nano-CaCO3-LDPE packaging on quality and browning of fresh-cut yam. LWT - Food Science and Technology, 60(2), 1155–1161. doi:10.1016/j.lwt.2014.09.021
- Mayer, A. M. (2006). Polyphenol oxidases in plants and fungi: Going places? A review. Phytochemistry, 67(21), 2318–2331. doi:10.1016/j.phytochem.2006.08.006
- Moon, K. M., Lee, B., Cho, W. K., Lee, B. S., Kim, C. Y., & Ma, J. Y. (2018). Swertiajaponin as an anti-browning and antioxidant flavonoid. Food Chemistry, 252, 207–214. doi:10.1016/j.foodchem.2018.01.053
- Muñoz-Pina, S., Ros-Lis, J. V., Argüelles, Á., Coll, C., Martínez-Máñez, R., & Andrés, A. (2018). Full inhibition of enzymatic browning in the presence of thiol-functionalised silica nanomaterial. Food Chemistry, 241, 199–205. doi:10.1016/j.foodchem.2017.08.059
- Pristijino, P., Wills, R. B. H., & Golding, J. B. (2006). Inhibition of browning on the surface of apple slices by short term exposure to nitric oxide (NO) gas. Postharvest Biology and Technology, 42, 256–259. doi:10.1016/j.postharvbio.2006.07.006
- Saltveit, M. E. (2000). Wound induced changes in phenolic metabolism and tissue browning are altered by heat shock. Postharvest Biology and Technology, 21(1), 61–69. doi:10.1016/S0925-5214(00)00165-4
- Siddiq, M., & Dolan, K. D. (2017). Characterization of polyphenol oxidase from blueberry (Vaccinium corymbosum L.). Food Chemistry, 218, 216–220. doi:10.1016/j.foodchem.2016.09.061
- Sikora, M., & Świeca, M. (2018). Effect of ascorbic acid postharvest treatment on enzymatic browning, phenolics and antioxidant capacity of stored mung bean sprouts. Food Chemistry, 239, 1160–1166. doi:10.1016/j.foodchem.2017.07.067
- Teng, J., Gong, Z., Deng, Y., Chen, L., Li, Q., Shao, Y., … Xiao, W. (2017). Purification, characterization and enzymatic synthesis of theaflavins of polyphenol oxidase isozymes from tea leaf (Camellia sinensis). LWT - Food Science and Technology, 84, 263–270. doi:10.1016/j.lwt.2017.05.065
- Teoh, L. S., Lasekan, O., Adzahan, N. M., & Hashim, N. (2016a). The effect of combinations of uv-c exposure with ascorbate and calcium chloride dips on the enzymatic activities and total phenolic content of minimally processed yam slices. Postharvest Biology & Technology, 120, 138–144. doi:10.1016/j.postharvbio.2016.06.008
- Teoh, L. S., Lasekan, O., Adzahan, N. M., & Hashim, N. (2016b). The effect of ultraviolet treatment on enzymatic activity and total phenolic content of minimally processed potato slices. Journal of Food Science and Technology, 53(7), 3035–3042. doi:10.1007/s13197-016-2275-z
- Terefe, N. S., Delon, A., Buckow, R., & Versteeg, C. (2015). Blueberry polyphenol oxidase: Characterization and the kinetics of thermal and high pressure activation and inactivation. Food Chemistry, 188, 193–200. doi:10.1016/j.foodchem.2015.04.040
- Tinello, F., & Lante, A. (2018). Recent advances in controlling polyphenol oxidase activity of fruit and vegetable products. Innovative Food Science & Emerging Technologies, 50, 73–83. doi:10.1016/j.ifset.2018.10.008
- Yılmaz, L., & Elmacı, Y. (2018). Polyphenol oxidase enzyme and inactivation methods. Turkish Journal of Agriculture-Food Science and Technology, 6, 333–345. doi:10.24925/turjaf.v6i3.333–345.1727
- Yolmeh, M., & Sadeghi Mahoonak, A. (2016). Characterization of polyphenol oxidase and peroxidase from Iranian medlar (Mespilus germanica L.) fruit. Journal of Agricultural Science and Technology, 18(5), 1187–1195.
- Yoruk, R., & Marshall, M. R. (2003). Physicochemical properties and function of plant polyphenol oxidase: A review. Journal of Food Biochemistry, 27(5), 361–422. doi:10.1111/j.1745-4514.2003.tb00289.x
- Zaini, N. A., Osman, A., Hamid, A. A., Ebrahimpour, A., & Saari, N. (2013). Purification and characterization of membrane-bound polyphenoloxidase (mPPO) from Snake fruit [Salacca zalacca (Gaertn.) Voss]. Food Chemistry, 136(2), 407–414. doi:10.1016/j.foodchem.2012.08.034
- Zeynep, M., & Mohamed, A. (2013). A new thermophilic polyphenol oxidase from bacillus sp.: Partial purification and biochemical characterization. Journal of Internal Medicine, 267(4), 11–20. doi:10.1111/j.1365-2796.2009.02169.x
- Zhou, X. R., Xiao, Y. J., Meng, X. H., & Liu, B. J. (2018). Full inhibition of Whangkeumbae pear polyphenol oxidase enzymatic browning reaction by l-cysteine. Food Chemistry, 266, 1–8. doi:10.1016/j.foodchem.2018.05.086
- Zor, T., & Selinger, Z. (1996). Linearization of the Bradford protein assay increases its sensitivity: Theoretical and experimental studies. Analytical Biochemistry, 236(2), 302–308. doi:10.1006/abio.1996.0171