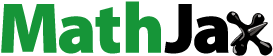
ABSTRACT
The aim of this study was to investigate the effect of fermentation parameters on heat-denatured soybean meal by Monascus purpureus 04093 on the soluble protein and polypeptide production and to evaluate the antioxidant activity and functional properties of the fermented products. The optimal fermentation parameters were obtained as follows: 11.25% soybean meal loaded in a 80 g/500 mL flask with 0.02 g/L Na2SO4 at 28°C and pH 6.5 for 96 h. The soluble protein and polypeptide yield reached 290.9 ± 3.4 mg/g and 106.6 ± 0.9 mg/g, respectively, which was 3.25 and 12.25 times that of the control, respectively. Moreover, the polysaccharide and amino acid contents, the antioxidant activities, the emulsifying properties and foaming capacities were significantly enhanced by Monascus fermentation (P < 0.05). The results indicated the viability of soybean meal in combination with Monascus for producing proteins with desirable characteristics as functional ingredients utilized in the food industry.
RESUMEN
El presente estudio se propuso investigar el efecto de los parámetros de fermentación de Monascus purpureus 04093 en la harina de soya desnaturalizada por calor y en la producción de proteínas y polipéptidos solubles, evaluando, a la vez, la actividad antioxidante y las propiedades funcionales de los productos fermentados. Los parámetros de fermentación óptimos de Monascus purpureus 04093 se obtuvieron en las siguientes condiciones: 11.25% de harina de soya colocada en un matraz de 80 g/500 ml con 0.02 g/L de Na2SO4, a 28°C y un pH de 6.5 durante 96 horas. Bajo estas condiciones, el rendimiento de proteína y polipéptido soluble fue de 290.9±3.4 mg/g y 106.6±0.9 mg/g, respectivamente, esto es, 3.25 y 12.25 veces mayor que el control, respectivamente. Además, los contenidos de polisacáridos y aminoácidos, las actividades antioxidantes, las propiedades emulsionantes y las capacidades de espumación mejoraron significativamente gracias a la fermentación de Monascus (P < 0.05). Los resultados dan cuenta de que la combinación de harina de soya con Monascus es adecuada para producir proteínas con características deseables para ser utilizadas como ingredientes funcionales en la industria alimentaria.
1. Introduction
Soybean meal is a by-product of soybean oil refining, which contains a large amount of protein (approximately 50%) and a balanced content of amino acids (Park, Chang, & Cho, Citation2019). Soybean meal has been widely used in the feed industry on account of its high nutritional value and low cost (Jacobsen et al., Citation2018). According to USDA Agricultural Projections to 2025, the world trade of soybean meal is projected to rise rapidly and increase by 20 percent (Lee, Tran, Hansen, & Ash, Citation2016). Nevertheless, the thermal treatment for the passivation of anti-nutritional factors and the desolventizing process in oil extraction resulted in highly denatured soybean proteins. Studies have reported that proteins were modified to achieve desirable properties by taking advantage of enzymatic techniques (Bah, Bekhit, McConnell, & Carne, Citation2016). Furthermore, microbe-proteases are widely used because they are cheaper in contrast with plant or animal proteases and exhibit benefits of enhanced functional properties of food proteins (de Oliveira et al., Citation2015). Hence, it is expected that heat-denatured soybean meal can be used for human consumption as a functional food if the nutritional values and functional properties were enhanced by food grade microorganisms.
The filamentous fungal genus Monascus belongs to the class Ascomycetes and family Monascaceae, which has been used to produce fermented products such as red kojic rice, red bean curd, and red rice liquor etc. in China, India and other oriental countries for centuries (Chen et al., Citation2015). There has been extensive research of the beneficial effects of Monascus-fermented products (Chen, Chang, & Pan, Citation2016). Monascus strains exhibit strong antioxidant activity through free radical scavenging (Dhale, Divakar, Kumar, & Vijayalakshmi, Citation2007). Furthermore, some studies have revealed that Monascus fermentation can be used as a potential method to further enhance the bioactivities of substrates and produce valuable compounds (Huang, Zhang, & Xue, Citation2017). Monascus is able to secrete abundant microbial proteolytic enzymes, which have the ability of disrupting large insoluble protein aggregates into smaller soluble proteins and polypeptides (Delavenne et al., Citation2015). In addition, the bioactive components in fermented soybean products exhibit antioxidant, antimicrobial, anti-hypertensive and anticancer activities (Sanjukta & Rai, Citation2016).
The functional properties of proteins are of great importance to their application in food, which can be modified by microbial fermentation. Molina Ortiz and Wagner (Citation2002) demonstrated that the hydrolysis of soy protein isolates results in enhancement of solubility and foam properties at acidic pH. Moreover, protein hydrolysis usually presents a positive effect on foaming capacity (Jamdar et al., Citation2010). Both Monascus and soybean meal have beneficial functions, making further investigation on the combination of Monascus and heat-denatured soybean meal crucial for increasing the production of soluble protein and polypeptide. The product yield is influenced by many factors, such as medium components, pH, temperature, fermentation time, etc. (Suraiya et al., Citation2018). The objective of this study was to develop an effective biotechnological strategy to produce heat-denatured soybean meal with more nutritional, functional and beneficial components by fermentation with Monascus. Hence, the fermentation parameters were optimized using single factor experiments for enhancing the production of soluble protein and polypeptide. Additionally, the amino acid and polysaccharide content, antioxidant activities and functional properties of heat-denatured soybean meal fermented by Monascus purpureus (M. purpureus) 04093 were also determined.
2. Materials and methods
2.1. Microorganism and culture media
M. purpureus 04093 was originally screened from mutated strains of M. purpureus 3.5833 by ultraviolet light, was obtained from the China General Microbiological Culture Collection Center (CGMCC), and was preserved in the College of Food Science, Northeast Agricultural University, China. The seed media contained 10 g/L glucose, 10 g/L peptone, 5 g/L yeast extract at pH 6.0–6.2. The pre-inoculum was prepared by growing M. purpureus 04093 in the seed media at 28°C in a rotary shaker (5.43 × g) for 24 h. All other biochemicals were of analytical grade.
2.2. Fermentation of heat-denatured soybean meal
The heat-denatured soybean meal was provided by Yanglin Soybean Inc. (Heilongjiang, China). Initially, soybean meal was crushed with a grinding particle size of 80 mesh. A mixture containing heat-denatured soybean meal (10%, w/w) and distilled water was prepared in 500 mL flasks (initial pH 6.5). The media were inoculated (approximately 107 CFU/mL) as 10% (v/w) after being autoclaved at 121°C for 20 min, and then were shaken vigorously at 5.43 × g for 120 h at 28°C. The culture was then centrifuged (Allegra X-30R, Beckman Coulter, Inc., USA) at 7000 × g at 4°C for 15 min. The supernatant was freeze-dried using Alpha 1–2 LD plus vacuum freeze-drying machine (Martin christ, Germany) and was used for further study.
2.3. Determination of soluble protein and polypeptide content
The soluble protein (SP) and polypeptide (PP) content were determined by the biuret method (Cotton, Delobbe, & Gulbis, Citation1997). For SP content, a 1 mL sample solution was added to 4 mL biuret reagent, the mixture was allowed to sit at 25°C for 20 min, and then the absorbance at 540 nm was determined by a Shimadzu UV mini-1240 spectrophotometer (Shimadzu Co., Kyoto, Japan). For PP content, a 1 mL of supernatant was placed in 1 mL 10% trichloroacetic acid (TCA), the mixture was incubated at 25°C for 30 min, and then centrifuged at 5000 × g for 10 min. Supernatants were collected and filtered using fiber syringe filters (0.45 μm, Takara, Dalian, China). The concentration of SP or PP was calculated according to the standard curve made using casein as the standard. The content of SP (or PP) in heat-denatured soybean meal was calculated from the following equation:
where N was the concentration of SP or PP in supernatant, V was the volume of distilled water, and m was the total mass of the soybean meal.
2.4. Optimization of fermentation parameters for SP and PP production
To investigate the effects of different culture conditions and mediums on the production of SP and PP, the process parameters and levels were optimized by single-factor experiments. The fermentation time for Monascus growth and production of SP and PP were monitored every 24 h for a incubation period of 120 h. Various content of soybean meal in the range of 6.25, 7.50, 8.75, 10.00, 11.25, 12.50, and 13.75% were employed to enhance the SP and PP production. The effect of initial pH on SP and PP production by Monascus 04093 was determined at pH 4.5, 5.0, 5.5, 6.0, 6.5 and 7.0 (adjusted with lactic acid and NaOH). Moreover, the media capacity of 48, 64, 80, 96, 112, and 128 g/500 mL flask was also evaluated. Fermentation was carried out at various temperatures including 26, 28, 30 and 32°C. Various inorganic salts such as CaCl2, MnSO4, ZnSO4, MgSO4, K2SO4, Na2SO4, and FeSO4 were also evaluated at a concentration of 0.01, 0.02 and 0.05 mol/L.
2.5. Determination of amino acid and polysaccharide content
The method of Falade and Adewusi (Citation2013) was used to determine the amino acid content. Briefly, the freeze-dried samples mentioned in Section 2.2 were mixed with 6 mol/L HCl (tryptophan with NaOH), sealed under vacuum and hydrolyzed at 110°C for 24 h. The type and amounts of the amino acids were analyzed by an automatic amino acid analyzer (L-8900, HITACHI, Japan). The content of polysaccharide was measured using the phenol-sulfuric acid method (Nazeam, Gad, Esmat, El-Hefnawy, & Singab, Citation2017). Samples were dissolved in 80% (v/v) ethanol, and stored overnight at 4°C. The precipitate was collected by centrifugation at 10000 × g for 15 min, and then dried at 60°C for 2 h and redissolved in distilled water. Sample solution (1 mL), phenol solution (1 mL) and concentrated sulfuric acid (5 mL) were added to screw cap tubes, the mixture was shaken vigorously, and then incubated at 25°C for 10 min. The absorbance was measured at 490 nm; anhydrous glucose was used as the standard.
2.6. Antioxidant activities of heat-denatured soybean meal
2.6.1. Ferric reducing antioxidant power assay
Ferric reducing antioxidant power (FRAP) was measured using a total antioxidant capacity assay kit (Beyotime Institute of Biotechnology, Shanghai, China) with the FRAP method, as described by Sun et al. (Citation2014). A working solution was prepared with TPTZ (2,4,6-tripyridyl-s-triazine) diluent, TPTZ solution, and detective buffer in a ratio of 10:1:1 (v/v) and incubated at 37°C. The sample solution (2.0 mg/mL, 5 μL) was mixed with 180 μL FRAP working solution. After incubation at 37°C for 4 min, the absorbance of the mixture was measured at 593 nm. The standard curve was determined using a FeSO4 standard solution with concentrations of 0.15, 0.3, 0.6, 0.9, 1.2, 1.5 mM. The antioxidant capacity was calculated using the standard curve and the following equation; PBS was used as a control.
where C1 was the concentration of FeSO4 solution when the absorbance of the sample was equal to that of FeSO4 solution, C2 was the concentration of the sample (2.0 mg/mL).
2.6.2. ABTS+ radical scavenging activity assay
The 2,2ʹ-azino-bis-3-ethylbenzthiazoline-6-sulphonic acid (ABTS+) radical scavenging activity was determined using a total antioxidant capacity assay kit (Beyotime Institute of Biotechnology, Shanghai, China) according to Zheng, Zhao, Dong, Su, and Zhao (Citation2016), with some modifications. The ABTS+ solution was diluted with 10 mM phosphate buffered saline (PBS, pH 7.0) to reach an absorbance of 0.70 ± 0.05 at 734 nm. The sample solution was obtained by adding 10 μL of antioxidant compounds (1.0 mg/mL) to 200 μL of the ABTS+ solution and was then mixed gently at 25°C. The absorbance of the mixture was measured at 734 nm after incubation in the dark at 25°C for 5 min. The sample was replaced by an equivalent volume of PBS and was used as a control. Ten millimolar 6-hydroxy-2,5,7,8-tetramethylchroman2-carboxylic acid (Trolox) solution was diluted with PBS to 0.05, 0.10, 0.15, 0.20, 0.25 mM to obtain the standard curve. The ABTS+ radical scavenging activity was calculated as follows:
where A1 and A2 were the absorbance of the control group and sample, respectively. C1 was the concentration of Trolox when the ABTS+ inhibition rate of the sample was equal to the ABTS+ inhibition rate of Trolox, C2 was the concentration of the sample, 1.0 mg/mL.
2.6.3. Superoxide anion (O2‒) radical scavenging activity assay
The O2‒ radical scavenging activity was evaluated using an inhibition and produce superoxide anion assay kit (Jiancheng Institute of Bioengineering, Nanjing, China). Sample was incubated at 37°C for 40 min, then PBS was added, and the absorbance of the mixture at 550 nm was measured after incubation at 25°C for 10 min. The superoxide anion radical scavenging activity was calculated as follows:
where A0, A1 and A2 were the absorbance values of the vitamin C standard, control group and the sample, respectively. C1 was the concentration of the vitamin C standard (0.15 mg/mL), C2 represented the concentration of the sample to be tested (g/L).
2.7. Functional properties of heat-denatured soybean meal
2.7.1. Solubility
The solubility of total protein was determined by the Noman et al. (Citation2018) method. Samples were added to buffer solutions with pH values of 4.00, 6.86 and 9.18 to prepare solutions (1%, w/v), followed by incubation at 25°C for 30 min. Then, the dispersions were centrifuged at 10,000 × g for 30 min. The solubility was calculated using the following equation:
where m1 was grams of total protein in the supernatant, and m2 was 100 g of total protein in the original sample.
2.7.2. Emulsifying properties
The emulsion activity index (EAI) and emulsion stability index (ESI) were determined (Noman et al., Citation2018). Nine milliliters of 1% (w/v) sample dispersion in phosphate butter (pH 7.0) together with 1 mL soybean oil were homogenized at 7000 × g for 2 min. Emulsions (10 μL) were pipetted from the bottom of the homogenized emulsion, and diluted with 5 mL of 0.1% (w/v) sodium dodecyl sulfate (SDS). The absorbance of the emulsions was measured immediately at 500 nm at 0 and 30 min, and 0.1% SDS solution was used as a control. The EAI and ESI were calculated by the following equations:
where A500 was the absorbance of the sample at 500 nm, N was the dilution factor, φ was the volumn fraction of oil in the emulsion (0.25), L was the optical path (1 cm), and C was the concentration of protein (g/mL).
where A0 was the absorbance of the diluted emulsions after homogenization at 500 nm, A30 was the absorbance of the diluted emulsions at 500 nm at 30 min, and ΔT was 30 min.
2.7.3. Foaming properties
The foaming capacity (FC) and foaming stability (FS) were determined according to a slightly modified version of the method described by Kunarayakul, Thaiphanit, Anprung, and Suppavorasatit (Citation2018). The samples were dispersed in phosphate butter (pH 7.0) at 25°C to prepare a 1.0% (w/v) test solution and 20 mL of the test solution was stirred vigorously at 10,000 × g for 1 min. The volume was recorded immediately after vigorous stirring and again at 30 min after stirring. The FC and FS were calculated as follows:
where V0 represented the volume of the mixture before stirring, V1 was the volume of foam immediately after vigorous stirring, and V30 was the volume of foam after 30 min.
2.8. Statistical analysis
All determinations were performed in triplicate and the results are expressed as mean ± deviation (SD). The statistical significance of the data was determined by one-way analysis of variance (ANOVA) using SPSS 19.0 software. Differences with P < 0.05 were considered statistically significant.
3. Results and discussion
3.1. Effects of fermentation time on biomass, SP and PP content
The influence of fermentation time on the biomass, SP and PP content in the heat-denatured soybean meal by M. purpureus 04093 are shown in . M. purpureus 04093 was in logarithmic phase from 0 to 24 h and reached stationary phase within 48–72 h. The PP content increased with fermentation time from 0 to 96 h, and remained unchanged from 96 to 120 h. In contrast, the SP content in soybean meal decreased at first, then increased with increasing fermentation time. The highest SP content was observed at 96 h (266.7 ± 10.1 mg/g), while the PP content reached a maximum value when the fermentation time was between 96–120 h. This was probably due to the changes in viable cell number and metabolic activities. Moreover, the optimum growth of microorganisms was not often in accordance with the amounts of fermentation products. The influence of fermentation on SP and PP production could be attributed to the integration of numerous factors. Several studies suggested that fermented process is believed to improve nutritional qualities compared to original unfermented soy-based materials (Seo & Cho, Citation2016). Here, the content of SP was taken as the main index, therefore, 96 h was chosen as the optimal fermentation time for further study.
Figure 1. Effects of fermentation time on biomass, SP and PP content. SP = soluble protein and PP = polypeptide. Different letters (a-f) indicate significant differences (P < 0.05).
Figura 1. Efectos del tiempo de fermentación sobre la biomasa con contenido de SP y PP. SP = proteína soluble y PP = polipéptido. Las letras diferentes (a-f) indican la presencia de diferencias significativas (P < 0.05).
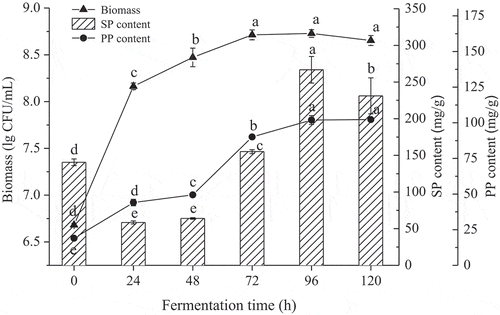
3.2. Effects of fermentation parameters on SP and PP content
) shows that the content of SP and PP had a significant increasing trend with the increase in soybean meal content. Interestingly, the production of PP showed a slight fluctuation when the amount of soybean meal was between 7.50% and 11.25%. Furthermore, both SP and PP had the highest yield when the amount of soybean meal was 11.25%. However, when the amount of soybean meal was above 11.25%, the SP and PP production declined sharply (P < 0.05). This could be explained based on the higher amount of soybean meal in the vessel and the lower moisture content, which would have negatively affected the thermal transmission and oxygen supply (Babitha, Soccol, & Pandey, Citation2007). These results are in agreement with Gervais and Molin (Citation2003) who reported moisure content plays an important role in promoting the oxygen transfer for the growth of microorganisms and improving the heat dissipation capacity. The results indicated that 11.25% soybean meal was the appropriate amount for M. purpureus 04093 to produce SP.
Figure 2. Effects of soybean meal content, initial pH, media capacity and temperature on SP and PP content. SP = soluble protein and PP = polypeptide. Different letters (a-f) indicate significant differences (P < 0.05).
Figura 2. Efectos del contenido de harina de soya, pH inicial, capacidad de medios y temperatura sobre el contenido de SP y PP. SP = proteína soluble y PP = polipéptido. Las letras diferentes (a-f) indican la presencia de diferencias significativas (P < 0.05).
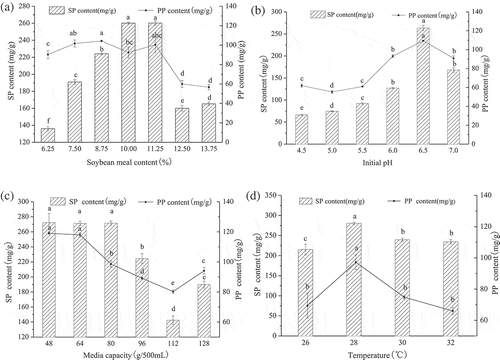
On the whole, the SP and PP production increased with an increase in pH up to 6.5, whereas a further increase in the pH decreased SP and PP production ()). The maximum SP and PP production were simultaneously observed at pH 6.5 with a yield of 263.6 ± 6.2 mg/g and 109.4 ± 0.7 mg/g, respectively. The SP and PP production was rather low when the initial pH was between 4.5 and 5.5. Several studies have shown that the optimum pH range for Monascus growth was 3.5–5, however, the lower initial pH may cause the charge of the protein to be neutralized and reduce the binding capacity between protein and water molecules (Kester & Richardson, Citation1984). It is well known that pH influences the transport of substrate components such as nitrogen sources, metabolic pathways and the accumulation of metabolites (Singh, Goel, Singh, Kumar Pathak, & Kaushik, Citation2015). Hence, it was concluded that pH 6.5 was suitable for the production of SP and PP.
It was observed that there were no significant differences in SP and PP production between 48 and 64 g/500 mL media ()). When the media capacity was over 80 g/500 mL, the SP and PP production first decreased and then showed a slight upward trend. In general, the optimal volume of the culture medium for shaking filamentous microorganisms is 50–100 mL/500 mL flask, which is 10-20% of the volume of the container used. Our study suggested that the relatively low media capacity was more conducive to the production of SP and PP; this might be related to the possibility that a higher capacity had an adverse effect on heat exchange. Moreover, Monascus is an aerobic microorganism, so a higher media capacity may result in insufficient oxygen supply (Babitha et al., Citation2007). Taking into account the above data, 80 g/500 mL was selected as the optimal media capacity for further study and to turn the denatured protein into soluble protein to the utmost extent.
All metabolic reactions of microorganisms are catalyzed by enzymes and the rate of the catalytic reaction depends on the temperature. As shown in ), the trend of SP content in soybean meal was similar to the content of PP; the increase in temperature from 26 to 28°C resulted in significant enhancements of SP and PP production, which reached a maximum value at 28°C. This phenomenon may be due to the impact of temperature on the growth of Monascus and the generation of microbial protease (Xu, Jiang, Li, & Wang, Citation2011). Nevertheless, there was an obvious decrease when the fermentation temperature was over 28°C, which may be attributed to the fact that the microorganism metabolism was destroyed to a certain extent. Moreover, there were no statistically significant differences in the yield of SP and PP between 30 and 32°C (P > 0.05). Hence, subsequent experiments were conducted using 28°C as the optimum fermentation temperature.
3.3. Effects of inorganic salts on SP and PP content
As seen in ,b), most of the inorganic salts significantly inhibited SP and PP production at both higher and lower concentrations compared with the control (P < 0.05). Among the inorganic salts, the highest SP and PP production were simultaneously observed in medium supplemented with 0.02 mol/L Na2SO4, reaching 302.7 ± 3.9 mg/g and 106.7 ± 2.3 mg/g, respectively. Meanwhile, 0.01 mol/L Na2SO4 significantly improved the yield of SP in comparison with the control, and 0.02 mol/L K2SO4 exhibited little influence on the yield of PP in comparison with the control. Metal ions have been reported to be very influential factor to enzymes in biological systems, and the enzyme reactions are controlled by modifying the electron transfer reactions of enzyme and the substrate (Williams, Citation1953). The addition of Na+ and K+ may be the key reason why the yield of SP and PP were enhanced, due to the activation of M. purpureus 04093, but the mechanism needs further study.
Figure 3. Effects of different inorganic salts on the production of SP and PP. SP = soluble protein and PP = polypeptide. Different lower-case letters (a–g) indicate significant differences (P < 0.05) between different kinds of inorganic salts; Different upper-case letters (A–C) indicate significant differences (P < 0.05) between different concentrations of inorganic salts.
Figura 3. Efectos de diferentes sales inorgánicas en la producción de SP y PP. SP = proteína soluble y PP = polipéptido. Las distintas letras minúsculas (a–g) indican la presencia de diferencias significativas (P < 0.05) entre diferentes tipos de sales inorgánicas; las distintas letras mayúsculas (A–C) indican la presencia de diferencias significativas (P < 0.05) entre diferentes concentraciones de sales inorgánicas.
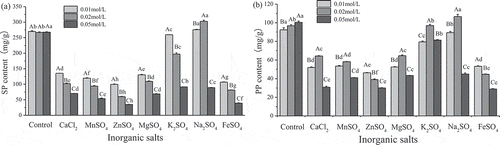
3.4. Verification experiment using the optimized parameters
When the optimized fermentation parameters were used, fermentation of heat-denatured soybean meal by M. purpureus 04093 significantly increased the SP and PP production at a yield of 290.9 ± 3.4 mg/g and 106.6 ± 0.9 mg/g, respectively (). This production was 3.25 and 12.25 times than that of control, respectively, indicating that the utilization of protein in the heat-denatured soybean meal was remarkably improved (P < 0.05). The optimal fermentation conditions for SP production were obtained by one-factor-at-a-time design as follows: 11.25% soybean meal loaded into an 80/500 mL flask with 0.02 g/L Na2SO4 at 28°C and pH 6.5 for 96 h.
Table 1. SP and PP production before and after fermentation by M. purpureus 04093 (expressed as milligrams of SP and PP production per grams of heat-denatured soybean meal) (mg/g).
Tabla 1. Producción de SP y PP antes y después de la fermentación de M. purpureus 04093 (expresada como miligramos de producción de SP y PP por gramos de harina de soya desnaturalizada por calor) (mg/g) .
3.5. Amino acid and polysaccharide analysis
The total amino acid content from heat-denatured soybean meal fermented by M. purpureus 04093 was increased significantly (P < 0.05) by 9.63% compared with that of the control (). Concerning the non-essential amino acids, glutamic acid, aspartic acid, proline and glycine content greatly increased. Concerning the essential amino acids, lysine exhibited the highest content (3.09%), followed by leucine and isoleucine. The results of our study with regard to amino acid content are similar to those of Song, Frias, Martinez-Villaluenga, Vidal-Valdeverde, and Gonzalez de Mejia (Citation2008). Additionally, the antioxidant activity of the peptides extracted from the protein hydrolysates hinge on the amino acid sequence of the peptides (Matsui et al., Citation2018). The amino acids in the fermented products are closer to the standard amino acid content recommended by FAO/WHO/UNO (Citation2007). The change of amino acid content probably because the degradation of the crude protein in heat-denatured soybean meal by protease produced by Monascus during fermentation (Dai et al., Citation2017). With regards to levels of polysaccharides, the fermented product (45.8 ± 0.9 mg/g) was significantly higher (P < 0.05) than that of the control (95.4 ± 1.2 mg/g). These results may be attributed to a change in the structure of carbohydrate components during fermentation by Monascus (Lee, Hsu, Liao, & Pan, Citation2012). It has been demonstrated that the polysaccharide components in Monascus-fermented products might be responsible for the effective antioxidant properties (Tseng et al., Citation2012).
Table 2. Content of amino acid from heat-denatured soybean meal fermented by M. purpureus 04093 (Unit of %).
Tabla 2. Contenido de aminoácidos en la harina de soya desnaturalizada por calor y fermentada por M. purpureus 04093 (unidad de %).
3.6. Antioxidant activities of fermented heat-denatured soybean meal
It is widely known that different compounds with antioxidant activities have different mechanisms to cope with oxidation, hence, it is difficult to completely evaluate the antioxidant capacity of complex samples by only one method (Huang et al., Citation2017). Therefore, several methods for the evaluation of antioxidant activity were adopted (). The ABTS+ radical scavenging activity showed an increasing trend with an increase in fermentation time. FRAP first increased and then decreased on the whole, and a similar trend was observed in the O2‒ radical scavenging activity. FRAP and ABTS+ radical scavenging activities reached maximum values of 0.19 ± 0.01 mmol/g at 24 h and 0.22 ± 0.01 mmol/g at 120 h, respectively, which were 2.62 and 3.30 times higher than that of the control, respectively. Previous studies have reported that ABTS+ radical scavenging activity was obviously higher in the Monascus fermented soybean milk than in the untreated control soybean milk (Lim et al., Citation2010). Furthermore, the O2‒ radical scavenging activity showed a remarkable increase until 24 h with a value of 6.74 ± 0.30 U/g, and was thereafter inhibited.
Figure 4. Antioxidant capacity of products from heat-denatured soybean meal fermented by M. purpureus 04093. Different letters (a-f) indicate significant differences (P < 0.05).
Figura 4. Capacidad antioxidante de productos de harina de soya desnaturalizada por calor y fermentada mediante M. purpureus 04093. Las distintas letras (a-f) indican la presencia de diferencias significativas (P < 0.05).
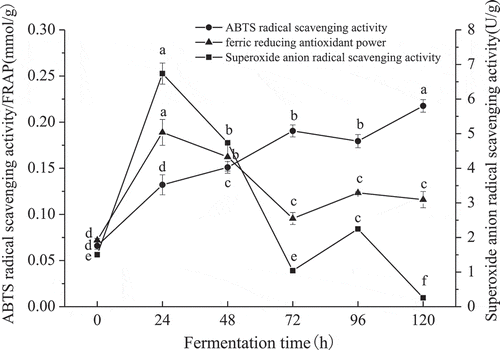
3.7. Solubility, emulsifying properties and foaming properties
The solubility’s of the fermented samples and the unfermented control gradually increased with increasing pH (). The solubility’s of Monascus fermented products were higher than 95% at pH 9.18. The solubility of fermented products was significantly higher than that of the control (P < 0.05), which may be due to a reduction in the chain length and molecular weight of soybean peptides. Moreover, the hydrolysis of protein could have exposed the dissociable amino and carboxyl groups, which increased the interactions of the hydrophilic amino acids with water molecules and thereby increased the hydrophilicity of the fermented products (Lamsal, Jung, & Johnson, Citation2007).
Table 3. Solubility of products from the heat-denatured soybean meal fermented by M. purpureus 04093 (Unit of %).
Tabla 3. Solubilidad de productos de la harina de soya desnaturalizada por calor y fermentada por M. purpureus 04093 (unidad de %).
shows that the fermented product exhibited significantly higher EAI and ESI when compared to the control (P < 0.05). Wang et al. (Citation2008) reported that the extent and mode of protein aggregation of unfolded proteins may affect emulsifying properties. Previous research indicated that peptides with low molecular weight contribute to higher emulsifying properties since the hydrolyzate can migrate rapidly to the water-oil interface prior to its adsorption (Popineau, Huchet, Larré, & Bérot, Citation2002). The transfer and rearrangement of amino acids during fermentation by Monascus resulted in the enhancement of electrostatic interactions among peptides. Furthermore, molecular flexibility and steric stabilization of proteins are also important elements that influence the stability of emulsions (Withana-Gamage & Wanasundara, Citation2012).
Table 4. Emulsifying and foaming properties of products from the heat-denatured soybean meal fermented by M. purpureus 04093.
Tabla 4. Propiedades emulsionantes y espumantes de productos de la harina de soya desnaturalizada por calor y fermentada por M. purpureus 04093.
The fermented product exhibited significantly higher FC than that of the control and had better FS compared with the unfermented control (P < 0.05). Molina Ortiz and Wagner (Citation2002) showed that a decrease in the particle size of soybean protein, as well as the high surface aromatic hydrophobicity in hydrolysates are associated with an improvement in foaming properties. Moreover, it has been reported that the behaviour of proteins at air/water interfaces is of great importance in foam systems (Radha, Ramesh Kumar, & Prakash, Citation2008). Additionally, a considerable number of flexible peptides are produced during Monascus fermentation, which results in the reduction of disulfide bonds in glycinin, thereby improving the properties of interfacial films (Kim & Kinsella, Citation1987).
4. Conclusions
The optimal fermentation parameters were obtained as follows: 11.25% soybean meal loaded in an 80 g/500 mL flask with 0.02 g/L Na2SO4 at 28°C and pH 6.5 for 96 h. The yield of SP and PP reached 290.9 ± 3.4 mg/g and 106.6 ± 0.9 mg/g, respectively, which were 3.25 and 12.25 times that of the control, respectively. Monascus fermentation resulted in greater amino acid and polysaccharide content, and higher antioxidant properties than that of the control. Moreover, the solubility, emulsifying properties and foaming properties were markedly improved (P < 0.05). These results indicated that heat-denatured soybean meal fermented by M. purpureus 04093 had desirable nutritional value and functional properties, and had great potential to be developed as novel functional ingredients and dietary supplements for food applications.
Disclosure statement
No potential conflict of interest was reported by the authors.
Additional information
Funding
References
- Babitha, S., Soccol, C. R., & Pandey, A. (2007). Solid-state fermentation for the production of Monascus pigments from jackfruit seed. Bioresource Technology, 98(8), 1554–1560. doi:10.1016/j.biortech.2006.06.005
- Bah, C. S., Bekhit, A. E. D. A., McConnell, M. A., & Carne, A. (2016). Generation of bioactive peptide hydrolysates from cattle plasma using plant and fungal proteases. Food Chemistry, 213, 98–107. doi:10.1016/j.foodchem.2016.06.065
- Chen, C. L., Chang, K. Y., & Pan, T. M. (2016). Monascus purpureus NTU 568 fermented product improves memory and learning ability in rats with aluminium-induced Alzheimer’s disease. Journal of Functional Foods, 21, 167–177. doi:10.1016/j.jff.2015.12.017
- Chen, W., He, Y., Zhou, Y., Shao, Y., Feng, Y., Li, M., & Chen, F. (2015). Edible filamentous fungi from the species Monascus: Early traditional fermentations, modern molecular biology, and future genomics. Comprehensive Reviews in Food Science and Food Safety, 14(5), 555–567. doi:10.1111/1541-4337.12145
- Cotton, F., Delobbe, E., & Gulbis, B. (1997). Assessment of a biuret method without concentration step for total protein determination in cerebrospinal fluid. Clinical Biochemistry, 30(4), 313–314. doi:10.1016/S0009-9120(97)00038-6
- Dai, C., Ma, H., He, R., Huang, L., Zhu, S., Ding, Q., & Luo, L. (2017). Improvement of nutritional value and bioactivity of soybean meal by solid-state fermentation with Bacillus subtilis. LWT - Food Science and Technology, 86, 1–7. doi:10.1016/j.lwt.2017.07.041
- de Oliveira, C. F., Correa, A. P. F., Coletto, D., Daroit, D. J., Cladera-Olivera, F., & Brandelli, A. (2015). Soy protein hydrolysis with microbial protease to improve antioxidant and functional properties. Journal of Food Science and Technology, 52(5), 2668–2678. doi:10.1007/s13197-014-1317-7
- Delavenne, E., Cliquet, S., Trunet, C., Barbier, G., Mounier, J., & Le Blay, G. (2015). Characterization of the antifungal activity of Lactobacillus harbinensis K.V9.3.1Np and Lactobacillus rhamnosus K.C8.3.1I in yogurt. Food Microbiology, 45(Pt A), 10–17. doi:10.1016/j.fm.2014.04.017
- Dhale, M. A., Divakar, S., Kumar, S. U., & Vijayalakshmi, G. (2007). Isolation and characterization of dihydromonacolin-MV from Monascus purpureus for antioxidant properties. Applied Microbiology and Biotechnology, 73(5), 1197–1202. doi:10.1007/s00253-006-0578-0
- Falade, O. S., & Adewusi, S. R. A. (2013). Comparative analysis of some essential amino acids and available lysine in Acacia colei and A. tumida seeds using chemical methods and an amino acid analyzer. Journal of AOAC International, 96(1), 102–109. doi:10.5740/jaoacint.11-551
- FAO/WHO/UNO. (2007). Protein and amino acid requirements in human nutrition. World Health Organization Technical Report Series, 935, 1–265.
- Gervais, P., & Molin, P. (2003). The role of water in solid-state fermentation. Biochemical Engineering Journal, 13(2–3), 85–101. doi:10.1016/s1369-703x(02)00122-5
- Huang, Q., Zhang, H., & Xue, D. (2017). Enhancement of antioxidant activity of Radix Puerariae and red yeast rice by mixed fermentation with Monascus purpureus. Food Chemistry, 226, 89–94. doi:10.1016/j.foodchem.2017.01.021
- Jacobsen, H. J., Kousoulaki, K., Sandberg, A.-S., Carlsson, N.-G., Ahlstrøm, Ø., & Oterhals, Å. (2018). Enzyme pre-treatment of soybean meal: Effects on non-starch carbohydrates, protein, phytic acid, and saponin biotransformation and digestibility in mink (Neovison vison). Animal Feed Science and Technology, 236, 1–13. doi:10.1016/j.anifeedsci.2017.11.017
- Jamdar, S. N., Rajalakshmi, V., Pednekar, M. D., Juan, F., Yardi, V., & Sharma, A. (2010). Influence of degree of hydrolysis on functional properties, antioxidant activity and ACE inhibitory activity of peanut protein hydrolysate. Food Chemistry, 121(1), 178–184. doi:10.1016/j.foodchem.2009.12.027
- Kester, J. J., & Richardson, T. (1984). Modification of whey proteins to improve functionality. Journal of Dairy Science, 67(11), 2757–2774. doi:10.3168/jds.S0022-0302(84)81633-1
- Kim, S. H., & Kinsella, J. E. (1987). Surface active properties of food proteins: Effects of reduction of disulfide bonds on film properties and foam stability of glycinin. Journal of Food Science, 52(1), 128–131. doi:10.1111/jfds.1987.52.issue-1
- Kunarayakul, S., Thaiphanit, S., Anprung, P., & Suppavorasatit, I. (2018). Optimization of coconut protein deamidation using protein-glutaminase and its effect on solubility, emulsification, and foaming properties of the proteins. Food Hydrocolloids, 79, 197–207. doi:10.1016/j.foodhyd.2017.12.031
- Lamsal, B. P., Jung, S., & Johnson, L. A. (2007). Rheological properties of soy protein hydrolysates obtained from limited enzymatic hydrolysis. LWT - Food Science and Technology, 40(7), 1215–1223. doi:10.1016/j.lwt.2006.08.021
- Lee, B. H., Hsu, W. H., Liao, T. H., & Pan, T. M. (2012). Inhibition of leukemia proliferation by a novel polysaccharide identified from Monascus-fermented dioscorea via inducing differentiation. Food & Function, 3(7), 758–764. doi:10.1039/c2fo30026e
- Lee, T., Tran, A., Hansen, J., & Ash, M. (2016). Major factors affecting global soybean and products trade projections. Amber Waves, (4), 22–34.
- Lim, J. Y., Kim, J. J., Lee, D. S., Kim, G. H., Shim, J. Y., Lee, I., & Imm, J. Y. (2010). Physicochemical characteristics and production of whole soymilk from Monascus fermented soybeans. Food Chemistry, 120(1), 255–260. doi:10.1016/j.foodchem.2009.10.017
- Matsui, R., Honda, R., Kanome, M., Hagiwara, A., Matsuda, Y., Togitani, T., & Terashima, M. (2018). Designing antioxidant peptides based on the antioxidant properties of the amino acid side-chains. Food Chemistry, 245, 750–755. doi:10.1016/j.foodchem.2017.11.119
- Molina Ortiz, S. E., & Wagner, J. R. (2002). Hydrolysates of native and modified soy protein isolatesstructural characteristics, solubility and foaming properties. Food Research International, 35(6), 511–518. doi:10.1016/S0963-9969(01)00149-1
- Nazeam, J. A., Gad, H. A., Esmat, A., El-Hefnawy, H. M., & Singab, A. B. (2017). Aloe arborescens polysaccharides: In vitro immunomodulation and potential cytotoxic activity. Journal of Medicinal Food, 20(5), 491–501. doi:10.1089/jmf.2016.0148
- Noman, A., Xu, Y., Al-Bukhaiti, W. Q., Abed, S. M., Ali, A. H., Ramadhan, A. H., & Xia, W. (2018). Influence of enzymatic hydrolysis conditions on the degree of hydrolysis and functional properties of protein hydrolysate obtained from Chinese sturgeon (Acipenser sinensis) by using papain enzyme. Process Biochemistry, 67, 19–28. doi:10.1016/j.procbio.2018.01.009
- Park, S., Chang, K. H., & Cho, S. J. (2019). Enhanced emulsifying and calcium-binding properties of fermented soybean meal. Biotechnology and Bioprocess Engineering, 24(1), 183–190. doi:10.1007/s12257-018-0237-1
- Popineau, Y., Huchet, B., Larré, C., & Bérot, S. (2002). Foaming and emulsifying properties of fractions of gluten peptides obtained by limited enzymatic hydrolysis and ultrafiltration. Journal of Cereal Science, 35(3), 327–335. doi:10.1006/jcrs.2001.0437
- Radha, C., Ramesh Kumar, P., & Prakash, V. (2008). Enzymatic modification as a tool to improve the functional properties of heat-processed soy flour. Journal of the Science of Food and Agriculture, 88(2), 336–343. doi:10.1002/jsfa.3094
- Sanjukta, S., & Rai, A. K. (2016). Production of bioactive peptides during soybean fermentation and their potential health benefits. Trends in Food Science & Technology, 50, 1–10. doi:10.1016/j.tifs.2016.01.010
- Seo, S. H., & Cho, S. J. (2016). Changes in allergenic and antinutritional protein profiles of soybean meal during solid-state fermentation with Bacillus subtilis. LWT - Food Science and Technology, 70, 208–212. doi:10.1016/j.lwt.2016.02.035
- Singh, N., Goel, G., Singh, N., Kumar Pathak, B., & Kaushik, D. (2015). Modeling the red pigment production by Monascus purpureus MTCC 369 by artificial neural network using rice water based medium. Food Bioscience, 11, 17–22. doi:10.1016/j.fbio.2015.04.001
- Song, Y. S., Frias, J., Martinez-Villaluenga, C., Vidal-Valdeverde, C., & Gonzalez de Mejia, E. (2008). Immunoreactivity reduction of soybean meal by fermentation, effect on amino acid composition and antigenicity of commercial soy products. Food Chemistry, 108(2), 571–581. doi:10.1016/j.foodchem.2007.11.013
- Sun, L. L., Gao, W., Zhang, M. M., Li, C., Wang, A. G., Su, Y. L., & Ji, T. F. (2014). Composition and antioxidant activity of the anthocyanins of the fruit of berberis heteropoda schrenk. Molecules, 19(11), 19078–19096. doi:10.3390/molecules191119078
- Suraiya, S., Kim, J. H., Tak, J. Y., Siddique, M. P., Young, C. J., Kim, J. K., & Kong, I.-S. (2018). Influences of fermentation parameters on lovastatin production by Monascus purpureus using Saccharina japonica as solid fermented substrate. LWT - Food Science and Technology, 92, 1–9. doi:10.1016/j.lwt.2018.02.013
- Tseng, K. C., Fang, T. J., Chiang, S. S., Liu, C. F., Wu, C. L., & Pan, T. M. (2012). Immunomodulatory activities and antioxidant properties of polysaccharides from Monascus-fermented products in vitro. Journal of the Science of Food and Agriculture, 92(7), 1483–1489. doi:10.1002/jsfa.4731
- Wang, X. S., Tang, C. H., Li, B. S., Yang, X. Q., Li, L., & Ma, C. Y. (2008). Effects of high-pressure treatment on some physicochemical and functional properties of soy protein isolates. Food Hydrocolloids, 22(4), 560–567. doi:10.1016/j.foodhyd.2007.01.027
- Williams, R. J. P. (1953). Metal ions in biological systems. Biological Reviews, 28(4), 381–412. doi:10.1111/brv.1953.28.issue-4
- Withana-Gamage, T. S., & Wanasundara, J. P. D. (2012). Molecular modelling for investigating structure–Function relationships of soy glycinin. Trends in Food Science & Technology, 28(2), 153–167. doi:10.1016/j.tifs.2012.06.014
- Xu, J., Jiang, L. Z., Li, Y., & Wang, J. L. (2011). Study on the antioxidative activity of enzymatic hydrolysates of defatted soybean meal. Applied Mechanics and Materials, 140, 459–464. doi:10.4028/www.scientific.net/AMM.140
- Zheng, L., Zhao, Y., Dong, H., Su, G., & Zhao, M. (2016). Structure–Activity relationship of antioxidant dipeptides: Dominant role of Tyr, Trp, Cys and Met residues. Journal of Functional Foods, 21, 485–496. doi:10.1016/j.jff.2015.12.003