ABSTRACT
The oxidative stress damage on cells is an example of roles in the pathogenesis of different degenerative diseases and the search of compounds that can slow this oxidation is continuous. The aim of this work was to obtain bioactive fractions from wild shrimp (Litopenaeus stylirostris) muscle, in order to evaluate their protective capacity and chemo-structurally characterize them. ABTS and DPPH, and FRAP assays suggested that bioactive fractions possess free radical-scavenging capacity, and reducing power, respectively. An inhibitory effect observed on AAPH-induced hemolysis and results from an H2O2-derived radicals-scavenging assay (retinoprotective) suggest that these fractions can exert their protective activity in human cells. UV–Vis, fluorescence, 13C-, 1H-NMR, and ESI-MS studies performed on the most bioactive fraction, suggests that their main components are eicosapentaenoic acid, dioctyl phthalate, and a possibly novel indolocarbazole alkaloid derivative. These results suggest that these compounds are good candidates to further investigations as possible chemoprotective agents.
RESUMEN
El daño por estrés oxidativo sufrido por las células es un ejemplo de roles en la patogénesis de diferentes enfermedades degenerativas, por lo que la búsqueda de compuestos que retrasen dicha oxidación es continua. Este trabajo tuvo por objetivo obtener fracciones bioactivas del músculo de camarón salvaje (Litopenaeus stylirostris), con la finalidad de evaluar su capacidad protectora y de realizar su caracterización quimioestructural. Los ensayos ABTS-DPPH y FRAP indicaron que las fracciones bioactivas poseen capacidad de eliminación de radicales libres y poder reductor, respectivamente. La hemólisis inducida por AAPH permite observar un efecto inhibitorio. Los resultados procedentes de un ensayo de eliminación de radicales derivados de H2O2 (retinoprotector), sugieren que estas fracciones pueden ejercer actividad protectora en células humanas. Los estudios de UV-Vis, fluorescencia, 13C-, 1H-NMR y ESI-MS aplicados a la fracción más bioactiva mostraron que sus componentes principales son ácido eicosapentaenoico, ftalato de dioctilo y un derivado de alcaloide de indolocarbazol posiblemente nuevo. Tales resultados insinúan que estos compuestos son buenos candidatos para que futuras investigaciones los evalúen como posibles agentes quimioprotectores.
Introduction
Whiteleg shrimp (Penaeus vannamei) is the most consumed species among crustaceans (53% of the total crustacean species produced in world aquaculture in 2016); however, currently wild shrimp (Litopenaeus stylirostris) is one of the most consumed among populations close to bays (FAO, Citation2018). Even though information about chemical composition and nutritional quality of L. stylirostris (about 19% protein, 2% lipids, 1.2% ashes, and 0.7% fiber) (Bing & Wang, Citation2006; Ramirez & Herrera, Citation1991) has been reported, still scarce studies exist on the health-promoting properties (e.g. antioxidants, antimutagens, antiproliferative, etc.) that shrimp biomolecules might have.
Natural antioxidants have received considerable interest from the food industry due to concerns about the safety of synthetic antioxidants. Marine natural products (MNP) have attracted the attention of scientists for their potential application in the food industry (Claeson & Bohlin, Citation1997; König & Wright, Citation1996; Newman, Cragg, & Snader, Citation2000). Until 1995, about 6500 MNP’s had been isolated; however, more than 19000 compounds have been reported up to date (Ciavatta et al., Citation2017). However, the marine environment has also been in the spotlight due to a wide range of biological activities associated with MNP and their potential therapeutic applications (Pawlik, Citation1993), among them are the antioxidants.
Among the sources of MNP with antioxidant properties are blue-green algae, which contain significant amounts of antioxidant carotenoids (Singh, Kate, & Banerjee, Citation2005), polysaccharides evaluated in vivo (De Almeida et al., Citation2011); Further, Belcastro, Marino, Russo, and Toscano (Citation2006) reported the isolation of molecules with antioxidant potential from a fungal species, while Shindo et al. (Citation2007) found bioactive molecules in marine bacterial species. However, higher marine organisms (cephalopods, fin-fish, crustaceans, etc.) may also be considered as a potential source of bioactive compounds; as an example, shrimp is a widely consumed seafood that has been studied for this purpose.
Various types of MNP in shrimp have been reported (da Silva et al., Citation2015; López-Saiz, Suárez-Jiménez, Plascencia-Jatomea, & Burgos-Hernández, Citation2013; Seymour, Li, & Morrissey, Citation1996; Sowmya & Sachindra, Citation2012). Among these types of bioactive compounds are peptides with ferrous ion chelating activity and radical scavenging activity (Sinthusamran, Benjakul, Kijroongrojana, Prodpran, & Kishimura, Citation2018), hydrolysates (Latorres, Rios, Saggiomo, Wasielesky, & Prentice-Hernandez, Citation2018), and caroteno-proteins (López-Saiz et al., Citation2014; Poonsin et al., Citation2018). Shrimp has also been reported as a source of astaxanthin, α-tocopherol, and polyunsaturated fatty acid (Gómez-Guillén, Montero, López-Caballero, Baccan, & Gómez-Estaca, Citation2018), all of them with chemical antioxidant capability as tested in vitro. However, questions on whether these in vitro antioxidant compounds have the capability of acting on protecting cells against oxidative stress-induced damages arise. Since many of the shrimp species-derived compounds with antioxidant activity as tested in vitro, are of lipid nature, the hypothesis of the present research work is that the antioxidant molecules present in L. stylirostris, yet to be fully characterized chemically, will be capable of showing chemoprotective activity in cellular models.
Based on the above, the aim of the present study was to obtain bioactive fractions from wild shrimp (Litopenaeus stylirostris) muscle, in order to evaluate their protective capacity, and identify the molecules responsible for this bioactivity.
Materials and methods
Testing species
Wild shrimp was obtained from Hermosillo coast (Kino bay), Sonora, Mexico (28°48ʹ37. 1”N 111°55ʹ43. 4”W) during the spring of 2018, transported in ice to the University of Sonora Seafood Laboratory, where was de-headed, peeled, and shrimp muscle were packed, and stored at −20°C until analysis.
Sample preparation
A total sample of 1.2 kg of shrimp muscle was separated into 100 g – portions. Chloroform-soluble extracts were prepared according to López-Saiz et al. (Citation2014). This extract was dissolved in 30 mL chromatographic grade (Sigma-Aldrich, St. Louis, MO, USA) ethyl acetate and stirred in a magnetic starred (IKA C-MAG HS 4, IKA – Werke GmbH & Co. KG, Staufen, Germany) for 12 h at room temperature at 500 rpm. Subsequently, it was filtered under vacuum to remove the suspended particles.
Column chromatography
In order to perform the fractionation procedure, 15 g of silica gel 60 (0.040–0.063 mm, Merck KGaA, Darmstadt, Germany) was impregnated with the shrimp muscle-extract. As a stationary phase, silica gel of the same characteristics was used to pack a 4 cm (id) x 27 cm glass chromatography column with 1 L of chromatographic grade (Sigma-Aldrich, St. Louis, MO, USA) hexane. The mobile phase consisted of chromatographic grade hexane (Hx) and ethyl acetate (AcOEt) in different proportions; the polarity of the mobile phase was gradually increased until 100% chromatographic grade methanol was used. Eluents were obtained every 250 mL in glass bottles and their contents were monitored using TLC (Thin Layer Chromatography) testing plates coated with TLC Silica gel 60 F245 (Merck KGaA, Darmstadt, Germany) to allow observation of bands at 254 and 365nm UV light (TLC was eluted with the same solvent system with which the open column was eluted). Eluents showing similar contents were combined, evaporated under reduced pressure at 35°C, and dried under N2 stream (each combination represented one fraction).
Antioxidant activity
In order to evaluate the antioxidant activity of the bioactive fraction (using fraction concentrations of 20 mg/mL and downwards), chemical (ABTS, DPPH, and FRAP assays) and cellular (antihemolysis and intracellular ROS scavenging assays) assays were performed to assess the chemical capability of inhibiting oxidation and the capacity of reducing oxidative cellular damage, respectively.
ABTS assay [2, 2'-azino-bis (3-ethylbenzothiazoline-6-sulfonic acid)]
This assay was carried out according to Loarca-Piña, Mendoza, Ramos-Gómez, and Reynoso (Citation2010). A 20-µL aliquot of each fraction, previously dissolved in DMSO, was combined with 230 µL of the ABTS solution and was incubated for 30 min. Absorbance values were obtained at 730 nm (Benchmark Microplate Reader; Bio-Rad, Hercules, CA, USA). Positive control contained all the reaction reagents except the fractions.
DPPH assay (1, 1-diphenyl-2-picrylhydrazyl)
This test was performed according to Loarca-Piña et al. (Citation2010). A 20-µL aliquot of each fraction, previously dissolved in DMSO, was combined with 200 µL of the DPPH (150 µM) solution (prepared in 80% methanol), and was incubated for 30 min. Absorbance values were obtained at 520 nm (Benchmark Microplate Reader; Bio-Rad, Hercules, CA, USA).
Ferric reducing antioxidant power (FRAP) assay
FRAP assay was done according to Benzie and Strain (Citation1996) with some modifications (the technique was adapted to a microplate and our equipment, a scan was performed, and the region where it absorbs the most is 638 nm). First, stock solutions were prepared in acid condition, which included 300 mM sodium acetate buffer (pH 3.6), 20 mM FeCl3 · 6H2O, and 10 mM TPTZ (2, 4, 6-tripyridyl-s-triazine) in 40 mM HCl. These solutions were mixed in a 10:1:1 ratio to prepare the working solution. A 20-µL aliquot of the bioactive fraction was combined with 280 µL of the FRAP solution, placed into wells, and incubated for 30 min at room temperature. Absorbance values were obtained at 638 nm (Thermo Fisher Scientific Inc. Multiskan GO, NY, USA).
For all of the assays above, a Trolox curve was made and all of the results were expressed as µM TE/g testing fraction.
AAPH assay [2, 2'-azobis- (2-methylpropionamidine)]
The antihemolysis activity was determined as described by Hernández-Ruiz et al. (Citation2018). Human erythrocytes were washed three times with a phosphate buffered saline (PBS) solution at pH 7.4. A suspension of 2% human erythrocytes is prepared in PBS. In a test tube, 100 μL of the erythrocyte suspension, 100 μL of fraction (at different concentrations), and 100 μL of 40 mM AAPH were combined. Test tubes were incubated at 37°C under agitation (Labquake Tube Shaker, Thermo Fisher Scientific Inc., MA, USA) at 30 rpm at darkness for 3 h. After incubation, the reaction mixture was diluted with 1 ml of PBS and centrifuged at 2000 xg for 10 min (Thermo Biofuge Stratos, Thermo Fisher Scientific Inc., Mass, USA). The absorbance of the supernatant was measured at 540 nm (Thermo Fisher Scientific Inc. Multiskan GO, NY, USA).
MTT [3-(4, 5-Dimethylthiazol-2-yl)-2, 5-Diphenyltetrazolium Bromide assay on ARPE-19 cell line
The retinoprotective activity was determined as described by Liu et al. (Citation2017). Human retinal pigment epithelial (ARPE-19) cells line was cultured in Dulbecco’s modified Eagle’s medium (Sigma-Aldrich, St. Louis, MO, USA), supplemented with 10% heat-inactivated fetal bovine serum (FBS) (Corning, NY, USA), and grown at 37°C in an atmosphere of 5% CO2 (VWR 2325 Water-Jacketed CO2 Incubator, Pa, USA). This assay (MTT) was carried out using the Roche cell proliferation kit I (Cat. No. 11-465-007-001, Roche Diagnostics GmbH, Mannheim, Germany) according to the manufacturer instructions, and was used to i) to determine the H2O2 concentration to be applied in the study on protection against H2O2-induced oxidative cell damage activity and ii) in that on the protective activity against H2O2-induced cell injury.
Briefly, 1 × 104 cells/well were suspended in 100 µL on DMEM at 10% of SFB; they were seeded in each well of a flat 96-well microtitration plate. After incubation time, the mixture containing 100 μL of culture medium at 10% of SFB was combined with 100 μg/mL of each fraction in diluted (0.5% v/v) DMSO (4 and 24 h before H2O2 stimuli). Later, wells were washed two times with 100 μL PBS and 100 μL of 10 mM H2O2 in culture medium was added, and the mixture was incubated for 30 min. Negative control cell cultures were incubated only in medium (containing DMSO 0.5% v/v) and positive control cell cultures were incubated only in 10 mM H2O2 in the culture medium.
Phalloidin–tetramethylrhodamine B isothiocyanate and 4′, 6-Diamidino-2 phenyindole dilactate fluorescence cell staining on ARPE-19 cell line
In order to observe the effect of the bioactive fraction against H2O2-induced cell injury on the tested cell line, internal cell morphological/structural aspects were observed following cell-staining procedures after the addition of fractions (Wankun et al., Citation2011). Cells were seeded onto 96-well microtitration plates, incubated for a period of 24 h at 37°C in an atmosphere of 5% CO2 (VWR 2325 Water-Jacketed CO2 Incubator, Pa, USA), and treated with bioactive fraction and H2O2. Cells were fixed with 3.7% formaldehyde in PBS per 15 min and further permeabilized with 0.2% Triton X-100 in PBS for 15 min. Then, cells were stained either with phalloidin–tetramethylrhodamine B isothiocyanate (Phalloidin) (Sigma-Aldrich, MFCD00278840) or 4′, 6-diamidino-2-phenyindole, dilactate (DAPI) (Sigma-Aldrich, D9564), in order to visualize cell structural issues such as F-actin microtubules or nuclear DNA, respectively. In order to performed observations, the microtitration plates were mounted on an inverted epifluorescence microscope (Leica DMi8, Leica-Microsystems, Wetzlar, Germany) equipped with fluorescence filters (546/10 RHOD excitation filter and emission 585/40, 350/50 DAPI excitation filter and 460/40 emission, and 480/40 excitation FITC filter and 527/30 emission), a cooled monochromatic DFC 450C camera (Leica Microsystems, Wetzlar, Germany) and fluorescence overlay software (LAS AF version 3.1.0, Leica Microsystems CMS GmbH, Mannheim, Germany).
Intracellular ROS scavenging assay on ARPE-19 cell line
Measurements of intracellular ROS levels in ARPE-19 cells were made using 2′,7′-dichlorodihydrofluorescein diacetate (DCFH-DA) as described by Halliwell and Whiteman (Citation2004), who reported it as a reliable method for studying intracellular ROS. Cell samples were incubated in the presence of 10 μM DCFH-DA at 37°C for 30 min (VWR 2325 Water-Jacketed CO2 Incubator, Pa, USA) and washed two times with PBS. The intracellular fluorescence was assayed by excitation at 498 nm and emission 530 nm to monitor intracellular ROS (FLUOstar Omega, BMG Labtech Inc., Ortenberg, Germany). Also, for microscopical qualitatively measuring of the effect of the bioactive fraction on H2O2-induced intracellular ROS production, cells were labeled with DCFH-DA under an inverted fluorescence microscope (Leica DMi8, Leica Microsystems GmbH, Wetzlar, Germany) equipped with fluorescence filters (with same characteristics as those described above) to performed observations.
Ultraviolet–visible (UV-Vis) and fluorescence spectroscopies
The bioactive sample was solubilized in chromatographic-grade methanol and analyzed on an Agilent 8453 diode array spectrophotometer (Agilent Technologies, Inc, Santa Clara, CA, USA) for the UV–Vis, and in a Perkin Elmer LS-50B Luminescence Spectrometer (PerkinElmer, Inc., Waltham, MA, USA) in case of fluorescence spectroscopy. In both determinations, a wavelength-range from 200 to 700 nm (at 25°C) and 1 cm quartz cuvette were used.
Carbon and proton nuclear magnetic resonance (13C-NMR and 1H-NMR)
Measurements were analyzed using Bruker NMR spectrometry equipment operated at 400 MHz (Bruker, Billerica, MA, USA). Each fraction was dissolved in 500 µL CDCl3 (Sigma-Aldrich, Saint Louis, MI, USA), using tetramethylsilane as internal standard, and the resulting mixture was placed into a 5 mm diameter ultra-precision NMR sample tubes at 23°C. Carbon was measured at 100 and proton at 400 MHz.
Electrospray ionization tandem mass spectrometry (ESI/MS)
The ESI/MS was performed on Agilent 6130 Quadrupole LC/MS (Agilent Technologies, Inc, Santa Clara, CA, USA). The instrument was operated in the negative [M-H] – and positive [M + H] + ion mode. The sample was performed in methanol acetonitrile 50:50, at 300°C.
Statistical analysis
Data were analyzed using an analysis of variance (ANOVA) with Tukey multiple comparison test (Tukey’s post hoc test), at a 95% confidence interval, and the level of significance of P ≤ 0.05 (SPSS). In order to calculate IC50 values (half-maximal inhibitory concentration) for biologically active fractions, a Probit analysis was performed using the Number Cruncher Statistical Software (NCSS, LLC., Kaysville, Utah, USA), version 2001, NCSS Statistical Software, U.S.A. All data were presented as the mean value with their indicated standard deviation (mean ± S.D.).
Results and discussion
Shrimp extraction, fractionation, and yield
Open column chromatography of the extract from shrimp resulted in a total of 18 fractions, A – R, having each different yields (these results of yield in mg are shown in the supplementary material). Fractions with the highest antioxidant potential were screened using both, chemical, and cellular assays.
Chemically (ABTS, DPPH, and FRAP) assayed antioxidant activity
An antioxidant has the capability to, at low concentrations, significantly prevent the oxidation of biomolecules, such as proteins, lipids, carbohydrates, and DNA. In the present study, the antioxidant activity of fractions was calculated using standard curves for ABTS, DPPH, and FRAP assays correlations values (R2) of 0.9986, 0.8712, and 0.8182 for ABTS, DPPH, respectively.
As shown in , among all of the fractions tested, M produced the significantly (P ≤ 0.05) highest μM TE/g values (21.4 ± 2.4, 25.2 ± 1.5, and 166.8 ± 7.2, for ABTS, DPPH, and FRAP, respectively).
Table 1. Indicators of antioxidant activity and half-maximal inhibitory concentration (IC50) of antihemolysis activity of fractions obtained from wild shrimp (Litopenaeus stylirostris) muscle.
Tabla 1. Indicadores de actividad antioxidante y concentración inhibitoria 50% (IC50) de la actividad antihemólisis de las fracciones obtenidas del músculo del camarón salvaje (Litopenaeus stylirostris)
Antioxidant indicator assays determine the capability of a compound (or group of compounds) to interact with a free neutral radical with a single electron not paired in one of its orbitals (McMurry, Citation2012). Free radicals are unstable and reactive and, due to their need to achieve stability by electron scavenging, they can initiate chain reactions that destroy cell’s chemical structures, such as membranes, lipids, proteins, and among others (Avello & Suwalsky, Citation2006). Based on the above, molecules present in the fraction M might have the capacity to donate protons and electrons (HAT and SET systems), therefore, to act as an antioxidant in a cellular model.
Antihemolysis activity
Fractions were also tested on their capacity of inhibiting free-radical-initiated peroxidation, which mediated the hemolysis of human erythrocyte induced by a water-soluble initiator, AAPH. The hemolytic activity of all fractions was screened against human erythrocytes and tested at different fractions. Erythrocytes are susceptible to peroxidation due to the accumulation of hemoglobin and polyunsaturated fatty acids (Babu, Shylesh, & Padikkala, Citation2001). shows that fractions M, L, and E (minimum IC50 values of 213 ± 82, 308 ± 71, and 315 ± 24 μg/mL, respectively) show the higher inhibition of AAPH radicals, which suggests they may contain primary antioxidants that have antihemolysis potential. Under oxidative stress, lipid peroxidation caused by peroxyl radicals may promote loss of erythrocyte membrane integrity; when AAPH enters the cytosol, a ROS chain reaction may occur due to radicals generated by AAPH decomposition (Zheng et al., Citation2016). Based on the above, components of fraction M may prevent AAPH-induced erythrocyte hemolysis, phenomenon that can be studied by determining their capability of deactivating peroxyl radicals through SET and HAT systems.
Results from the antihemolysis study, the activity of fraction M is significantly (P ≤ 0.05) different from that obtained for the antioxidant control gallic acid (GA) (IC50 of 49 μg/mL). An issue to be highlighted is that, gallic acid is a hydrophilic molecule, therefore, its antioxidant action takes place in the cellular plasma (as an extracellular protector in blood), while the fractions from shrimp are lipophilic; therefore, their action may be more associated to membrane cell protection; and even, they might enter into the cell and also provide intracellular protection (Nimse & Pal, Citation2015; Sies, Citation1997); for this reason, a retinoprotective study was pertinent; these results are presented below.
Retinoprotective against H2O2-induced oxidative cell damage activity
Cellular ROS mainly include H2O2 which easily penetrate cell membranes and cause damages to cells (Akhtar, Ahamed, Alhadlaq, & Alshamsan, Citation2017). Once inside the cell, H2O2 can form the hydroxyl radical causing cell damage/injury to structural proteins, enzymes, phospholipid membrane, and DNA (Liochev & Fridovich, Citation1994).
As shown in ), low concentrations of H2O2 did not diminish ARPE-19 cellular viability, possibly due to pathways of oxidative stress regulation. However, H2O2 concentration is high, ROS exceeds cellular regulation capacity and damage becomes possible.
Figure 1. Cell viability of ARPE-19 cells exposed to a) different concentrations of H2O2 (0.06 to 20 mM) for 30 min, and b) to different concentrations of fraction M (fM) (12.5 to 400 μg/mL) for 24 h. Values represent means from three determinations. Control cell cultures were incubated with DMSO as the vehicle solvent at a maximum concentration of 0.5% and represent 100% viability.
Figura 1. Viabilidad celular de las células ARPE-19 expuestas a: a) diferentes concentraciones de H2O2 (0.06 a 20 mM) durante 30 min y b) diferentes concentraciones de fracción M (fM) (12.5 a 400 μg/mL) durante 24 horas. Los valores representan las medias de tres determinaciones. Los cultivos celulares de control se incubaron con DMSO como disolvente vehículo a una concentración máxima de 0.5% y representan una viabilidad de 100%
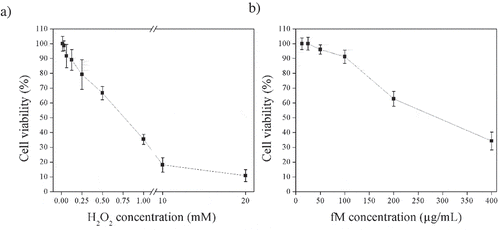
In order to know whether the bioactive fractions (BF) protect cells from H2O2-induced damage, cultivated cells were pre-treated with BF and then exposed to H2O2. As shown in , 10 mM H2O2 caused 80–85% loss of cellular viability compared to control (untreated) cells, but no significant (P ≤ 0.05) cytotoxicity was observed in the presence of 100 μg/mL BF alone. On the other hand, when cells were pre-treated with BF and then exposed to H2O2, cell survival rate remarkably increased. The effect of fraction M (fM) outstand from the rest of the fractions when incubated for 24 h (viability of 75%), strongly suggesting that fM has the capacity of protecting cells against H2O2. Also, as shown in ), cellular viability lower than 90% was only observed only when fM concentration was 100 μg/mL. This suggest that compounds present in fM might act as chemo-protectors at that concentration and not higher. It is important to mention that the effect of protection against oxidative stress caused by H2O2 does not always shows a linear behavior, therefore, it is necessary to carry out further research on this regard (Halliwell, Citation2012; Noguera-Artiaga et al., Citation2019).
Figure 2. Cell viability of H2O2-induced damage ARPE-19 cells, previously exposed to 100 μg/mL of different fractions from wild shrimp (Litopenaeus stylirostris) muscle after 4 and 24 h. Values represent means from three determinations. Different letters among bars mean significant differences (P ≤ 0.05); Tukey´s least significant difference test. Fractions were obtained from open column chromatography.
Figura 2. Viabilidad celular de células ARPE-19 con daño inducido por H2O2, previamente expuestas a 100 μg/ml de diferentes fracciones del músculo del camarón salvaje (Litopenaeus stylirostris) después de 4 y 24 horas. Los valores representan las medias de tres determinaciones. Las distintas letras entre barras significan que existen diferencias significativas (P ≤ 0.05); prueba de Diferencia Mínima Significativa de Tukey. Las fracciones se obtuvieron mediante cromatografía en columna abierta
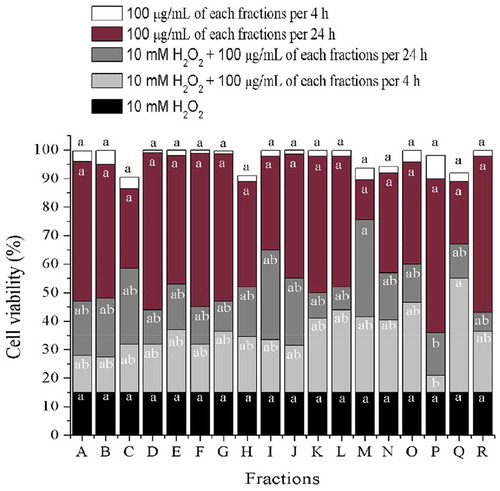
Retinoprotective effect of fM against H2O2-induced cell injury activity
Cell damage/death is initiated when organelles are injured and, knowing which are either affected or protected, may contribute to know the ways by which a molecule exerts its antioxidant activity. shows actin and chromatin morphology in cells stained with phalloidin and DAPI, either in the presence or absence of 250 μM H2O2; this made it possible to visualize the existence (or not) of cellular injury.
Figure 3. Effect of H2O2 on structural cellular aspects of ARPE-19 human cell line, and the retinoprotective effect of fraction M (fM) against H2O2-induced cell injury. Cells actin-microfilament cytoskeleton (red-colored) and nuclear DNA (blue-colored), after staining with phalloidin and DAPI, respectively. Observations were made at 20x. Control cells were incubated with DMSO as the vehicle solvent at a maximum concentration of 0.5% and represent no morphologic changes.
Figura 3. Efecto de H2O2 sobre los aspectos celulares estructurales de la línea celular humana ARPE-19 y efecto retinoprotector de la fracción M (fM) contra la lesión celular inducida por H2O2. Citoesqueleto de actina y microfilamento de células (de color rojo), y ADN nuclear (de color azul), después de su tinción con faloidina y DAPI, respectivamente. Las observaciones se hicieron a 20x. Las células control se incubaron con DMSO como disolvente vehículo a una concentración máxima de 0.5% y no representan cambios morfológicos
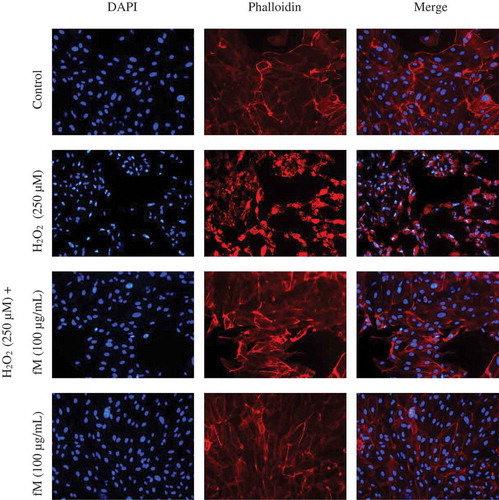
Cells treated with H2O2 showed evidence of changes in their structure associated with actin microfilaments de-polymerization, volume reduction (cellular shrinkage), membrane blebbing, and damage to phospholipids membrane. In addition, intense fluorescence suggests severe chromatin damage, and pyknotic and fragmented nuclei. However, actin and DNA structure in cells exposed to H2O2 but pretreated with fM were similar to the control. These results suggest that fM possesses the capability to attenuate H2O2-induced cellular injury.
Fraction M (fM) inhibits H2O2-induced intracellular ROS generation
Kietzmann (Citation2010) described oxidative stress as a pathological state of excessive ROS production; additionally, H2O2 may induce cellular apoptosis at harmful concentrations (Li et al., Citation2013). Based on the above, the capability of fM of intracellularly inhibit H2O2-induced damage in ARPE-19 cells was investigated. The effect of fM on intracellular ROS levels was determined using the DCFH-DA assay. Briefly, in this assay, non-fluorescent DCFH-DA is enzymatically transformed to non-fluorescent DCFH, which oxidized by intracellular ROS results in fluorescent DCF which stains the cells (Gomes, Fernandes, & Lima, Citation2005).
As shown in ), fluorescence intensity increases in cells with H2O2 concentration suggesting an increasing intracellular ROS concentration. On the other hand, ) shows that fM did not significantly produce ROS in this model. When cells, previously treated with fM at different concentrations, were exposed to 250 μM H2O2, the fluorescence intensity significantly (P ≤ 0.05) decreased as fM concentration increased ()), suggesting that components of fM may protect the cell, possibly by directly blocking ROS. Microscopic visualization subjected to the experimental procedures described above was consisting of fluorescence intensity measurements ()).
Figure 4. Retinoprotective effect of fraction M (fM) against H2O2-induced intracellular ROS production in ARPE-19 cells. a) Intracellular ROS production in cells exposed to different concentrations of H2O2. b) Intracellular ROS production in cells treated with different concentrations of fM during 24 h. c) Intracellular ROS production in cells treated with and without 250 μM H2O2 and fM at different concentrations per 24 h, (-) stimulus not used, (+) stimulus used. d) Microscopic visualization of the effect of fM on H2O2-induced intracellular ROS production. Images were selected as representative data from three independent experiments (at 20x). Control: not stimulated cells. Each value represents the mean ± SD of three independent experiments (P ≤ 0.05); Tukey´s least significant difference test.
Figura 4. Efecto retinoprotector de la fracción M (fM) contra la producción de ROS intracelular inducida por H2O2 en células ARPE-19. a) Producción intracelular de ROS en células expuestas a diferentes concentraciones de H2O2. b) Producción intracelular de ROS en células tratadas con diferente concentración de fM durante 24 horas. c) Producción intracelular de ROS en células tratadas con y sin H2O2 250 μM y fM a diferentes concentraciones por 24 horas; (-) estímulo no utilizado, (+) estímulo utilizado. d) Visualización microscópica del efecto de fM sobre la producción de ROS intracelular inducida por H2O2. Las imágenes se seleccionaron como datos representativos de tres experimentos independientes (a 20x). Control: células no estimuladas. Cada valor representa la media ± DE obtenida en tres experimentos independientes (P ≤ 0.05); prueba de diferencia menos significativa de Tukey
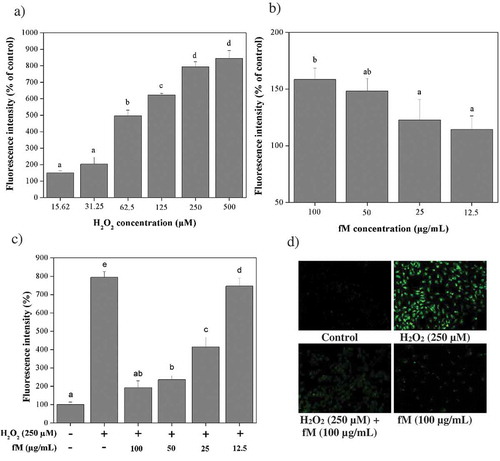
Characterization of fM
According to the results, fM was the most chemoprotective fraction, therefore, it was selected for chemical/structural characterization. This information is important for the establishment of association between structural aspects and bioactivity.
The UV–Vis analysis (Supplementary material) fM has maximum of absorption at 237 and 273 nm with absorbance values of 3.78, 1.25, which are attributed to π→π* transitions associated to the presence of aromatic compounds; bands with a very low absorbance values 302, 322, and 350 nm are attributed to n→π* transition which is produced by aromatics rings having atoms with available electrons such as oxygen and nitrogen. Fluorescence analyses (Supplementary material) showed that components of fM had emissions at λ = 352 nm and 515 nm when they were excited at λ = 315 and 380 nm, respectively.
shows 13C- and 1H-NMR signals suggesting that fM are composed of several compounds. Eicosapentaenoic acid (EPA) was found as a majoritarian component, also its signals are in agreement with Tyl, Brecker, and Wagner (Citation2008). Likewise, characteristic signals of both, patterns of 1, 4 di-substituted aromatic ring and coupling pattern of the protons of the aromatic ring, signals at δ = 7.71 ppm (dd, J = 5.7, 3.3 Hz) and 7.53 ppm (dd, J = 5.7, 3.3 Hz), suggested the presence of dioctyl phthalate (DOP). However, the amount of this compound was too small that no 13C-NMR signals could be determined. Similar results have been reported (Cruz-Ramírez et al., Citation2015; García-Romo et al., Citation2018; López-Saiz et al., Citation2014) also suggesting the presence of DOP.
Table 2. 13C- and 1H-NMR analyses of fraction M dissolved in CDCl3.
Tabla 2. Análisis de 13C y 1H-RMN de la fracción M disuelta en CDCl3.
Finally, 1H-NMR signals at δ = 9.77 ppm (s) corresponding to alcohol groups, δ = 7.52 (d), 7.32 (d) and 7.13 (dd) ppm corresponding to protons belonging to aromatic rings with a substitution, signals at δ = 3.7–3.4 (m) ppm associated to aliphatic protons and signals at δ = 1.14 (d) ppm related to methyl protons, as well as 13C-NMR signals at 116.66, 110.89, and 109.95 associated to carbons belonging to aromatic rings and signals at 71.10, 65.45, and 56.27 associated to carbons belong to aliphatic chains, were obtained. Also, these results indicate that the molecular weight of this molecule is 540.20 m/z; this molecular weight in conjunction with these signals mentioned above, corresponds to a new indolocarbazole alkaloid derivative, with a mass of 543 Da and a chemical formula C28H25N5O7. In order to confirm the presence of this compound, characterization by mass technique ESI in negative mode was performed (Supplementary material).
However, in agreement to evidence showed, the compounds responsible for shrimp muscle lipid extraction for anti-oxidant, anti-hemolytic, and retinoprotective activities are a least three compounds, EPA, a new indolocarbazole alkaloid derivative and DOP.
Conclusions
Based on the results obtained, the fM isolated from the muscle of wild shrimp (Litopeaneus stylirostris) contains antioxidants compounds indeed capable of exerting a chemoprotective effect on human cell lines. A possible novel indolocarbazole type of compound possibly is, in great part, responsible for this activity; however, further investigation is necessary for both, complete biological characterization and full chemical/structural elucidation.
Supplemental Material
Download MS Word (892.2 KB)Disclosure statement
No potential conflict of interest was reported by the authors.
Supplementary material
Supplemental data for this article can be accessed Publisher’s website
Additional information
Funding
References
- Akhtar, M. J., Ahamed, M., Alhadlaq, H. A., & Alshamsan, A. (2017). Mechanism of ROS scavenging and antioxidant signalling by redox metallic and fullerene nanomaterials: Potential implications in ROS associated degenerative disorders. Biochimica Et Biophysica Acta (BBA) - General Subjects, 1861, 802–813. doi:10.1016/j.bbagen.2017.01.018
- Avello, M., & Suwalsky, M. (2006). Radicales libres, antioxidantes naturales y mecanismos de protección. Atenea (Concepción), 2006, 161–172. ISSN 0718-0462
- Babu, B. H., Shylesh, B. S., & Padikkala, J. (2001). Antioxidant and hepatoprotective effect of Acanthus ilicifolius. Fitoterapia, 72, 272–277. doi:10.1016/S0367-326X(00)00300-2
- Belcastro, M., Marino, T., Russo, N., & Toscano, M. (2006). Structural and electronic characterization of antioxidants from marine organisms. Theoretical Chemistry Accounts, 115, 361–369. doi:10.1007/s00214-006-0077-5
- Benzie, I. F. F., & Strain, J. J. (1996). The ferric reducing ability of plasma (FRAP) as a measure of “antioxidant power”: The FRAP assay. Analytical Biochemistry, 239, 70–76. doi:10.1006/abio.1996.0292
- Bing, X., & Wang, J. (2006). A comparative study of nutritional quality in the muscle of Penaeus stylirostris and Penaeus vannamei in the cultured-pond. Acta Hydrobiologica Sinica, 30, 458.
- Ciavatta, M. L., Lefranc, F., Carbone, M., Mollo, E., Gavagnin, M., Betancourt, T., … Kiss, R. (2017). Marine mollusk-derived agents with antiproliferative activity as promising anticancer agents to overcome chemotherapy resistance. Medicinal Research Reviews, 37, 702–801.
- Claeson, P., & Bohlin, L. (1997). Some aspects of bioassay methods in natural-product research aimed at drug lead discovery. Trends in Biotechnology, 15, 245–248. doi:10.1016/S0167-7799(97)01051-2
- Cruz-Ramírez, S., López-Saiz, C., Plascencia-Jatomea, M., Machi-Lara, L., Rocha-Alonzo, F., Márquez-Ríos, E., & Burgos-Hernández, A. (2015). Isolation and identification of an antimutagenic phthalate derivative compound from octopus (Paraoctopus limaculatus). Tropical Journal of Pharmaceutical Research, 14, 1257. doi:10.4314/tjpr.v14i7.19
- da Silva, F. O., Tramonte, V. L. C. G., Parisenti, J., Lima-Garcia, J. F., Maraschin, M., & da Silva, E. L. (2015). Litopenaeus vannamei muscle carotenoids versus astaxanthin: A comparison of antioxidant activity and in vitro protective effects against lipid peroxidation. Food Bioscience, 9, 12–19. doi:10.1016/j.fbio.2014.11.001
- De Almeida, C. L. F., Falcão, D. S., Lima, D. M., Gedson, R., Montenegro, D. A., Lira, N. S., … Barbosa-Filho, J. M. (2011). Bioactivities from marine algae of the genus gracilaria. International Journal of Molecular Sciences, 12, 4550–4573. doi:10.3390/ijms12074550
- FAO. (2018). The State of World Fisheries and Aquaculture 2018 - Meeting the sustainable development goals. Rome. Licence: CC BY-NC-SA 3.0 IGO. ISBN 978-92-5-130562-1
- García-Romo, J. S., Yépiz-Gómez, M. S., Plascencia-Jatomea, M., Ortega, H. D. C. S., Burgos-Hernández, A., León, J. R. G. D., … Borboa-Flores, J. (2018). Compounds with in vitro antibacterial activity from hydrosol of Lippia palmeri and morphometric changes on listeria monocytogenes. Biotecnia, 20, 35–42.
- Gomes, A., Fernandes, E., & Lima, J. L. F. C. (2005). Fluorescence probes used for detection of reactive oxygen species. Journal of Biochemical and Biophysical Methods, 65, 45–80. doi:10.1016/j.jbbm.2005.10.003
- Gómez-Guillén, M. C., Montero, P., López-Caballero, M. E., Baccan, G. C., & Gómez-Estaca, J. (2018). Bioactive and technological functionality of a lipid extract from shrimp (L. vannamei) cephalothorax. LWT - Food Science and Technology, 89, 704–711. doi:10.1016/j.lwt.2017.11.052
- Halliwell, B. (2012). Free radicals and antioxidants: Updating a personal view. Nutrition Reviews, 70.5, 257–265. doi:10.1111/j.1753-4887.2012.00476.x
- Halliwell, B., & Whiteman, M. (2004). Measuring reactive species and oxidative damage in vivo and in cell culture: How should you do it and what do the results mean? British Journal of Pharmacology, 142, 231–255.
- Hernández-Ruiz, K. L., Ruiz-Cruz, S., Cira-Chávez, L. A., Gassos-Ortega, L. E., de Jesús Ornelas-paz, J., Del-Toro-Sánchez, C. L., … Rodríguez-Félix, F. (2018). Evaluation of antioxidant capacity, protective effect on human erythrocytes and phenolic compound identification in two varieties of plum fruit (Spondias spp.) by UPLC-MS. Molecules, 23, 3200. doi:10.3390/molecules23123200
- Kietzmann, T. (2010). Intracellular redox compartments: Mechanisms and significances. Antioxidants & Redox Signaling, 13, 395–398. doi:10.1089/ars.2009.3001
- König, G., & Wright, A. (1996). Marine natural products research: Current directions and future potential. Planta Medica, 62, 193–211. doi:10.1055/s-2006-957861
- Latorres, J. M., Rios, D. G., Saggiomo, G., Wasielesky, W., & Prentice-Hernandez, C. (2018). Functional and antioxidant properties of protein hydrolysates obtained from white shrimp (Litopenaeus vannamei). Journal of Food Science and Technology, 55, 721–729. doi:10.1007/s13197-017-2983-z
- Li, Z., Dong, X., Liu, H., Chen, X., Shi, H., Fan, Y., … Zhang, X. (2013). Astaxanthin protects ARPE-19 cells from oxidative stress via upregulation of Nrf2-regulated phase II enzymes through activation of PI3K/Akt. Molecular Vision, 19, 1656–1666.
- Liochev, S. I., & Fridovich, I. (1994). The role of O2.− in the production of HO.: In vitro and in vivo. Free Radical Biology and Medicine, 16, 29–33. doi:10.1016/0891-5849(94)90239-9
- Liu, H., Liu, W., Zhou, X., Long, C., Kuang, X., Hu, J., … Fan, Y. (2017). Protective effect of lutein on ARPE-19 cells upon H2O2-induced G2/M arrest. Molecular Medicine Reports, 16, 2069–2074. doi:10.3892/mmr.2017.6838
- Loarca-Piña, G., Mendoza, S., Ramos-Gómez, M., & Reynoso, R. (2010). Antioxidant, antimutagenic, and antidiabetic activities of edible leaves from Cnidoscolus chayamansa Mc. Vaugh. Journal of Food Science, 75, H68–H72. doi:10.1111/jfds.2010.75.issue-2
- López-Saiz, C.-M., Suárez-Jiménez, G.-M., Plascencia-Jatomea, M., & Burgos-Hernández, A. (2013). Shrimp lipids: A source of cancer chemopreventive compounds. Marine Drugs, 11, 3926–3950. doi:10.3390/md11103926
- López-Saiz, C.-M., Velázquez, C., Hernández, J., Cinco-Moroyoqui, F.-J., Plascencia-Jatomea, M., Robles-Sánchez, M., … Burgos-Hernández, A. (2014). Isolation and structural elucidation of antiproliferative compounds of lipidic fractions from white shrimp muscle (Litopenaeus vannamei). International Journal of Molecular Sciences, 15, 23555–23570. doi:10.3390/ijms151223555
- McMurry, J. (2012). Quimica organica (8 ed.). Mexico, D.F.: CENGAGE Learning.
- Newman, D. J., Cragg, G. M., & Snader, K. M. (2000). The influence of natural products upon drug discovery (Antiquity to late 1999). Natural Product Reports, 17, 215–234. doi:10.1039/a902202c
- Nimse, S. B., & Pal, D. (2015). Free radicals, natural antioxidants, and their reaction mechanisms. RSC Advances, 5, 27986–28006. doi:10.1039/C4RA13315C
- Noguera-Artiaga, L., García-Romo, J. S., Rosas-Burgos, E. C., Cinco-Moroyoqui, F. J., Vidal-Quintanar, R. L., Carbonell-Barrachina, Á. A., & Burgos-Hernández, A. (2019). Antioxidant, Antimutagenic and Cytoprotective Properties of Hydrosos Pistachio Nuts. Molecules, 24, 4362. doi:10.3390/molecules24234362
- Pawlik, J. R. (1993). Marine invertebrate chemical defenses. Paper Presented at the Chemical Reviews, 93, 1911–1922.
- Poonsin, T., Simpson, B. K., Benjakul, S., Visessanguan, W., Yoshida, A., & Klomklao, S. (2018). Carotenoprotein from pacific white shrimp (Litopenaeus vannamei) shells extracted using trypsin from albacore tuna (Thunnus alalunga) spleen: Antioxidant activity and its potential in model systems. Journal of Food Biochemistry, 42, e12462. doi:10.1111/jfbc.2018.42.issue-2
- Ramirez, A. D., & Herrera, C. (1991). Chemical composition and yield of giant prawns (Macrobrachium rosembergii) and white shrimps (Penaeus sp.) and production of meal from waste materials. Ingenieria Y Ciencia Química, 13, 15–19.
- Seymour, T. A., Li, S. J., & Morrissey, M. T. (1996). Characterization of a natural antioxidant from shrimp shell waste. Journal of Agricultural and Food Chemistry, 44, 682–685. doi:10.1021/jf950597f
- Shindo, K., Kikuta, K., Suzuki, A., Katsuta, A., Kasai, H., Yasumoto-Hirose, M., … Takaichi, S. (2007). Rare carotenoids, (3R)-saproxanthin and (3R,2′S)-myxol, isolated from novel marine bacteria (Flavobacteriaceae) and their antioxidative activities. Applied Microbiology and Biotechnology, 74, 1350–1357. doi:10.1007/s00253-006-0774-y
- Sies, H. (1997). Oxidative stress: Oxidants and antioxidants. Experimental Physiology. Translation and Integration, 82, 291–295.
- Singh, S., Kate, B. N., & Banerjee, U. C. (2005). Bioactive compounds from cyanobacteria and microalgae: An overview. Critical Reviews in Biotechnology, 25, 73–95. doi:10.1080/07388550500248498
- Sinthusamran, S., Benjakul, S., Kijroongrojana, K., Prodpran, T., & Kishimura, H. (2018). Protein hydrolysates from pacific white shrimp cephalothorax manufactured with different processes: Compositions, characteristics and antioxidative activity. Waste and Biomass Valorization, 1, 1–14.
- Sowmya, R., & Sachindra, N. M. (2012). Evaluation of antioxidant activity of carotenoid extract from shrimp processing byproducts by in vitro assays and in membrane model system. Food Chemistry, 134, 308–314. doi:10.1016/j.foodchem.2012.02.147
- Tyl, C. E., Brecker, L., & Wagner, K. H. (2008). 1H NMR spectroscopy as tool to follow changes in the fatty acids of fish oils. European Journal of Lipid Science and Technology, 110, 141–148. doi:10.1002/ejlt.200700150
- Wankun, X., Wenzhen, Y., Min, Z., Weiyan, Z., Huan, C., Wei, D., … Xiaoxin, L. (2011). Protective effect of paeoniflorin against oxidative stress in human retinal pigment epithelium in vitro. Molecular Vision, 17, 3512.
- Zheng, L., Zhao, X., Zhang, P., Chen, C., Liu, S., Huang, R., … Zhang, Y. (2016). Hemocyanin from shrimp Litopenaeus vannamei has antiproliferative effect against hela cell in vitro. PloS One, 11, e0151801. doi:10.1371/journal.pone.0151801