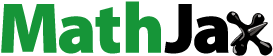
ABSTRACT
Peanut protein isolate was hydrolyzed by trypsin. The resulting peanut peptide fractions (PPF) were then divided into three molecular weight components by ultrafiltration (PPF1, MW >5 kDa; PPF2, 3 kDa < MW <5 kDa; PPF3, MW <3 kDa; and untreated PPF). Experiments showed that PPF3 had the best capacity for chelation with zinc. PPF3 and the PPF3–Zn chelate were characterized by Ultraviolet spectrophotometry, Fourier-transform infrared spectroscopy, X-ray diffraction, Scanning electron microscopy, Energy disperse spectroscopy, Fluorescence spectroscopy, and Thermogravimetric analysis. The results showed that the apparent characteristics and internal structures of PPF3 were changed by chelation of Zn2+. PPF3 contained sufficient metal-binding sites, with – COOH, – OH, and – NH2 potentially participating in coordination with Zn2+ to form the PPF3-Zn chelate. Furthermore, the results suggested that peanut peptides might chelate with zinc to act as an emerging carrier for zinc supplements.
RESUMEN
Para fines del presente estudio, el aislado de proteína de cacahuate fue hidrolizado empleando tripsina. Empleando ultrafiltración, se dividieron las fracciones peptídicas del cacahuate (PPF) en tres componentes de peso molecular. (PPF1, MW >5 kDa; PPF2, 3 kDa < MW <5 kDa; PPF3, MW <3 kDa; y PPF no tratado). Los experimentos constataron que el PPF3 tiene la mejor capacidad de quelación con el zinc. El PPF3 y el quelato de PPF3-Zn fueron caracterizados utilizando espectrofotometría ultravioleta, espectroscopia infrarroja por transformada de Fourier, difracción de rayos X, microscopía electrónica de barrido, espectroscopia de energía dispersa, espectroscopia de fluorescencia y análisis termogravimétrico. Los resultados dieron cuenta de que las características aparentes y las estructuras internas del PPF3 se modificaron a partir de la quelación del Zn2+. El PPF3 mostró suficientes sitios de unión de metales, con —COOH, —OH y —NH2, participando potencialmente para formar el quelato PPF3-Zn en coordinación con el Zn2+. Además, los resultados establecieron que los péptidos del cacahuate podrían quelatarse con el zinc para actuar como portadores emergentes de suplementos de zinc.
1. Introduction
Zinc, as one of the essential trace elements of the human body, plays an irreplaceable role in maintaining the normal life activities and physiological state of the human body. It is called “life element” and participates of hundreds of functional proteins and enzymes in human body (Bae et al., Citation2011; Depciuch et al., Citation2017). Its demand is small, but it is closely related to body health and disease. The growth and development of the human body, immune function, lipid metabolism, and other life activities cannot be separated from the role of zinc. In children, zinc deficiency can delay growth and development, while in adults, it can impair gonadal function, leading to neurological and immune dysfunction (Wang et al., Citation2012; Xu et al., Citation2017). Natural sources of zinc are extensive and abundant, but zinc absorption and metabolism by the human body is limited, such that not enough zinc is absorbed (C. Zhang et al., Citation2017). Most zinc supplement products on the market are inorganic salt additives, such as zinc sulfate. These products are unstable, which can cause irritation of the gastrointestinal tract (Akbar et al., Citation2013), making them unsuitable for long-term intake. Studies have shown that soluble organic compounds with low molecular weights, such as organic acids, amino acids, and peptides, can improve the bioavailability of zinc through chelation with zinc ions (Miquel & Farré, Citation2007). Zinc-chelating peptides can be prepared from many food proteins, such as sesame protein (Wang et al., Citation2012), milk protein (X. Wang et al., Citation2011), yak casein (Chen et al., Citation2013), and oyster protein (Gerbino et al., Citation2011). Their structures contain metal ligands, such as His, Cys, Asp, Glu, and Ser (Storcksdieck et al., Citation2007; Swain et al., Citation2002; Taylor et al., Citation1986; Udechukwu et al., Citation2016), which form soluble zinc chelates that enhance zinc absorption. Compared with inorganic zinc salts, zinc–peptide chelates are more readily absorbed by the gastrointestinal tract (Y. Zhang et al., Citation2013).
Peanut protein is an excellent edible protein. Among plant proteins, peanut protein is second only to soybean protein in terms of nutrition and quantity. Arachin and conarrachin comprise 90% of peanut protein, while albumin accounts for about 10%. Peanut protein is also rich in various minerals, trace elements, vitamins, and amino acids, allowing it to be easily digested and absorbed by the human body. Meanwhile, its effective availability rate can be up to 98%. Although peanut meal after oil extraction is often used as feed or directly as fertilizer, peanut protein has yet to be fully utilized. Peanut peptide is a mixture of peptides with different molecular weights formed by enzymatic hydrolysis or acid–base treatment of peanut protein. Peanut peptide contains various amino acids, with peptides composed of different amino acids playing different roles. Recent studies have shown that peanut short peptides have antioxidation, blood pressure-lowering, cholesterol-lowering, blood sugar-lowering, anticancer, antifatigue, and immune system-strengthening activities, among other physiological functions (Dam et al., Citation1956; Guo et al., Citation2015). However, their metal-chelation properties have rarely been studied. Europe, America, Japan, and other developed regions have wide-ranging peanut peptide markets, where peanut peptide research is in a world-leading position. The main products of this field are peptide drugs, reagents, active peptides as functional factors for low-antigen health foods, and food-grade polypeptides. The development of peanut peptide not only improves the utilization efficiency of peanut protein, but also helps solve the shortage of protein supply.
In this study, peanut peptide was prepared by enzymatic hydrolysis of peanut protein and then chelated with soluble zinc ions to obtain peanut peptide–zinc chelates. Ultraviolet (UV) spectrophotometry, Fourier-transform infrared (FTIR) spectroscopy, X-ray diffraction (XRD), Scanning electron microscopy (SEM), Energy disperse spectroscopy (EDS), Fluorescence spectroscopy, and Thermogravimetric (TG) analysis were used to characterize the peptides and peptide–zinc chelates. After the chelation happened between peptides and zinc ion, many spectral characteristics of peptide-zinc chelates had changed, which was the main basis for the identification of peptide-zinc chelates. Through the qualitative study of the chelates, not only could clear the composition, structure and production of the chelates, but also the chemical bond changes of the chelates could be understood, which provided the basis for the study of the chelating mechanisms of peanut peptides and zinc.
2. Materials and methods
2.1. Materials
Peanut (yuanza 9102, Henan Academy of Agricultural Sciences, China), trypsin (2.4 × 105 U/g, Novozymes), and ultrafiltration polyethersulfone membranes (3 and 5 kDa; Sartorius Stedim Biotech GmbH, Germany) were purchased. Other reagents used were of analytical grade.
2.2. Peanut protein isolate (PPI) preparation
The red skin was removed from selected peanut kernels, which were then pressed at a low temperature. The pressed peanuts were degreased by n-hexane and sifted with 40 mesh to obtain skim peanut meal powder. A defatted flour solution was prepared by mixing skim peanut meal powder and water in a 1:10 (w/v) ratio, and the solution pH was adjusted to 9.0. This slurry was then stirred for 2 h at 50°C, and centrifuged at 2415 g force for 10 min (SIGMA 3–18 K, SIGMA LABORZENTRIFUGEN GMBH, Germany). The supernatant obtained after centrifugation was collected and the pH was adjusted to 4.5, before allowing the protein precipitate to settle. The PPI was recovered by freeze-drying (LGJ-25E, Sihuan Freike Technology Development Co., Ltd., China)
2.3. Enzymatic preparation of peanut peptide fractions (PPF)
The PPI was added to deionized water to give a 7 wt% solution. This homogenate was denatured in a water bath at 90°C for 10 min. The homogenate was then adjusted to pH 6 using 1 M HCl and hydrolyzed by trypsin at an enzyme dosage of 5000 U/g of protein at 50°C for 2 h. Trypsin was inactivated by boiling for 10 min, and the sample was kept at room temperature. The mixture was then centrifuged at 2415 g force for 20 min. The hydrolysate supernatant was collected and freeze-dried for later use. This experiment was performed in triplicate.
2.4. Ultrafiltration
Ultrafiltration was used to separate the PPF solution. Ultrafiltration membranes with molecular weight cut-offs of 3 and 5 kDa were used as the separation medium to intercept large molecules and screen small molecules. Retentates PPF1, (MW > 5 kDa) PPF2, (3 kDa < MW < 5 kDa), and PPF3 (MW < 3 kDa) were freeze-dried for further use (Wang et al., Citation2012).
2.5. Evaluation of zinc-chelating capacity
2.5.1. Peptide–zinc chelates
Peptide–zinc chelates were prepared according to the modified method of Wang et al. (Hedberg et al., Citation2011). Lyophilized powders of untreated PPF, PPF1, PPF2, and PPF3 were added to deionized water (10 mg/mL). ZnSO4 solution (10 mg/mL) was then added at a ratio of 2:1 (v/v). The mixture was adjusted to pH 5.0 and stirred at 60°C for 1 h. The reaction solution was centrifuged at 3773 g force for 10 min to remove impurities. Three times the volume of anhydrous ethanol was added to the supernatant. After mixing thoroughly, the supernatant was centrifuged at 1849.g force for 15 min. The peptide–zinc chelates were then collected for lyophilization and stored at – 20°C.
2.5.2. Evaluation of zinc-chelating capacity by atomic absorption spectrometry (AAS)
The peptide–zinc chelate was digested in an acidic solution and analyzed by AAS (ICE-3000, Thermo Fisher Scientific Co., Ltd., Germany) according to the method of Yan et al (X. Yan et al., Citation2015).
To determine the total amount of Zn2+, the peanut peptide-chelated zinc solution was filtered through a 0.45 μm membrane. A 5 mL aliquot of the filtrate was made up to 50 mL with deionized water. The total amount of Zn2+ in the solution was then measured by AAS.
To determine the amount of chelating Zn2+, a 5 mL aliquot of the filtrate from the above steps was taken, mixed with anhydrous ethanol (15 mL), and centrifuged (1849. g force, 15 min). The resulting precipitate was made up to a volume of 50 mL, and the amount of chelating Zn2+ was assayed by AAS.
The zinc-chelating ability was calculated using the following equation:
where M0 and M1 are the total zinc and the chelating zinc contents, respectively.
2.6. UV spectrophotometry
Peanut peptide and zinc–peptide chelate samples were prepared as 0.1 mg/mL solutions, and UV visible spectrophotometry (TU-1901 spectrophotometer, Beijing General Instrument Co., Ltd., China) was performed in the wavelength range of 190–400 nm. A quartz tube with a path length of 1.0 cm was used for testing.
2.7. FTIR spectroscopy
Peanut peptide or zinc–peptide chelate (2 mg) was uniformly mixed with KBr (200 mg) in an agate mortar under infrared light (Hajji et al., Citation2015). The ground powder was placed in a tablet mould and pressurized at about 60 MPa for 5 min. After removing the pressure, a transparent film was obtained, which was placed in the infrared spectrometer for analysis (FTIR TENSOR 27, Brooklyn Co., Ltd., Germany). The number of scans was 32 and the scanning range was 400–4000 cm–1 to improve the signal-to-noise ratio (Oliveira et al., Citation2014). Spectra were processed using Omnic 8.0 software.
2.8. XRD analysis
A small amount of peptide or zinc–peptide chelate was ground into a uniform powder with a particle diameter of less than 10 μm using a mortar. The sample was placed in a sample tank, carefully pressed into a flat specimen, and then analyzed by X-ray diffraction (MiniFlex 600, Rigaku Co., Ltd., Japan). The equipment parameters were as follows: Light source, copper target tube radioactive source (λ = 0.154 nm); acceleration voltage, 40 kV; acceleration current, 40 mA; scanning range (2θ), 5–90°; scanning speed, 0.02°/0.2 s.
2.9. SEM and EDS analyses
For SEM analysis, peptide or zinc–peptide chelate samples were pasted on an aluminum sample table using double-sided adhesive, and then sprayed with a thin layer of gold film. The microscopic sample morphology was observed and photographed by SEM (Quanta 250 FEG, FEI Co., Ltd., China) at 10,000× magnification. For EDS analysis, peptide or zinc–peptide chelate samples were pasted on an aluminum sample table with double-sided adhesive and subjected to microregion detection. The weight percentage and atomic percentage contents of the major elements in the microregion were measured by EDS, which was repeated three times. The EDS instrument parameters were as follows: Voltage, 10 kV; electron beam, 6.0; operating distance, 10.0 mm (Zhao et al., Citation2015).
2.10. Fluorescence spectroscopy
An appropriate amount of peptide or zinc–peptide chelate was used to prepare a sample solution (0.5 mg/mL). Fluorescence absorption was measured using a fluorescence spectrophotometer (G9800A, Agilent Technology Co., Ltd., Germany) with an excitation wavelength of 272 nm and emission wavelength of 290–500 nm, using a slit width of 5 nm for excitation and emission, and a quartz tube with a path length of 1.0 cm.
2.11. TG analysis
Powder samples of peptide or zinc peptide chelate (5 mg) were placed into an aluminum crucible, with an empty crucible used as the reference. The protective gas was nitrogen, the heating rate was 30°C/min, and the temperature range was 25–1000°C (STA 449F3 system, NETZSCH Instrument Manufacturing Co., Ltd., Germany).
2.12. Statistical analysis
All data were obtained from experiments repeated three times, with the results expressed as means ± standard deviation. SPSS 16.0 software was used for data significance analysis, and Origin 8.5 software was used for drawing.
3. Results and discussion
3.1. Zinc-chelating capacity of different peptide components
After ultrafiltration, the three peptide fractions (PPF1–3) with different molecular weight compositions were evaluated by AAS to investigate the zinc-chelating rate. As shown in , PPF2 had the lowest rate of zinc chelation, while PPF3 had the highest (p < .05). This was due to changes in the quantity and type of amino acids in the different peptide components after ultrafiltration. Differences in the number of zinc-binding sites also contributed to these results. As PPF3 chelated with zinc most readily, PPF3 and PPF3-Zn were selected for further analysis.
Table 1. Rate of peptide–zinc chelation for different peptide components.
Tabla 1. Tasa de quelación péptido-zinc para diferentes componentes peptídicos.
3.2. UV spectroscopy
The structure characteristics of substances can be analyzed by UV spectrophotometry. Differences in the samples after chelation can be analyzed from changes in the dislocation and strength of UV absorption spectra (Hedberg et al., Citation2011). As shown in , the absorbance of PPF3-Zn decreased significantly compared with that of PPF3. The peanut peptide showed significant absorptions at 207 and 279 nm (Z. Yan et al., Citation2016). After chelation with zinc, the absorption peaks moved to 205 and 274 nm, respectively, with that at 274 nm decreased significantly. This might be due to chiral changes in the chromospheres and auxochromes involving C = O, COOH, – OH, and – NH2 when PPF3 binded with zinc ions (Armas et al., Citation2006). The maximum absorption peak of peanut peptide was at about 207 nm, which was attributed to the n→π* electron transition of the peptide carbonyl (C = O) bond. When the peptide chelated with a zinc ion, Zn2+ formed a coordination bond with N and O in the peptide, which affected the n→π* electron transition of the peptide carbonyl (C = O) bond, causing a blueshift (Blindauer et al., Citation2009). Furthermore, after chelating with a zinc ion, the weak absorption peak of PPF3 at 279 nm, attributed to the π→π* electron transition of the ligand (N–C–O), shifted to 274 nm (Huang et al., Citation2015). These phenomena indicated that chelation between PPF3 and Zn2+ occurred through the metal-binding sites.
3.3. FTIR spectroscopy
FTIR spectra can be used to analyze the composition of substances and detect their functional groups, such as C–O, N–H, and O–H (Qian et al., Citation2009). The infrared spectra of PPF3 and PPF3-Zn in the wavenumber range of 400–4000 cm–1 are shown in . The absorption peak position and absorption intensity of these two substances were different (Warren et al., Citation2015). A characteristic infrared spectrum band of PPF3 was observed at 3292.75 cm–1, which was attributed to the N–H stretching vibration band of the peptide group. In contrast, the same absorption peak of PPF3-Zn was observed at 3451.96 cm–1 and was slightly wider than that of PPF3. Furthermore, the peak showed significantly reduced transmittance, which might be due to the presence of Zn instead of H (Liao et al., Citation2016). The PPF3 absorption peak at 2962.13 cm–1 was shifted to 2946.05 cm–1 after chelating with zinc, and corresponded to the alkyl stretching vibration zone (Muyonga et al., Citation2004). The PPF3 absorption peak at 1666.20 cm–1 was shifted to 1658.48 cm–1 after chelation, and was attributed to a – C = O stretching vibration or – COO – antisymmetric vibration. Finally, the PPF3 absorption peak at 1029.80 cm–1 was shifted to 1110.80 cm–1 in the coordinated peanut peptide, and indicated a – C = O vibration or – CNC – stretching vibration. The above results showed that changes in the infrared spectrum mainly occurred in the amide band region and the vibrational band attributed to – CNC – or – C = O bonds, indicating that bonding occurred between zinc ions and amino or carboxyl groups on the amino acid residues (Wang et al., Citation2014).
3.4. XRD analysis
XRD can be used to determine the spatial structure of chelates. When X-rays of certain wavelengths are irradiated onto the crystalline substance, they will be scattered by atoms or ions encountered in a regular arrangement in the crystal. The phase of the scattered X-ray will be strengthened in some directions, showing the unique diffraction phenomenon corresponding to the crystal structure (Ahmad et al., Citation2012). To understand the change in peptide structure after chelation with zinc ions, XRD was used to analyze changes between PPF3 and PPF3-Zn.
The diffraction patterns of the two samples are shown in . The XRD pattern of PPF3-Zn was quite different from that of PPF3. PPF3 was complex, its XRD pattern indicating that it was an amorphous material (Jin et al., Citation2011). No sharp XRD diffraction peak was observed, but an absorption was present at 2θ = 20.34°. Furthermore, the absorption peak background of PPF3 was larger. The absorption peak at 20.34° had clearly disappeared from the XRD pattern of PPF3-Zn, but other strong absorption peaks appeared. These diffraction peaks were high and sharp, with a small background. PPF3-Zn also showed a few weak dispersive diffraction peaks with a better crystal shape. These phenomena indicated that the structure of PPF3 changed greatly after chelation with zinc ions, producing a mixture of crystalline and amorphous structures with a significant increase in crystallinity (Jin et al., Citation2011).
3.5. SEM and EDS analyses
As an advanced modern analytical technique, SEM has a resolution of several nanometers, which allows tiny and even undetectable substances to be magnified several thousand-fold. Therefore, SEM is often used for morphological observation and quantitative analysis of element composition (Nune et al., Citation2016).
SEM was used to observe the microscopic surface structure of PPF3 and PPF3-Zn, with the results shown in ,). The surface of PPF3 showed a flake structure, while that of PPF3-Zn showed a block structure. PPF3 had a low molecular weight, resulting in a more uniform, fine, smooth, and flat organization. In contrast, PPF3-Zn had a rough surface with many small surface particles, which might be zinc crystals. In addition to ion binding and coordination binding, chelation could also be concluded to have some adsorption effects (Li et al., Citation2019).
Figure 4. (A,B) SEM photographs (10,000×) of (A) PPF3 and (B) PPF3-Zn. (C,D) EDS images of (C) PPF3 and (D) PPF3-Zn.
Figura 4. (A,B) Fotografías SEM (10,000×) de (A) PPF3 y (B) PPF3-Zn. (C,D) Imágenes EDS de (C) PPF3 y (D) PPF3-Zn.SEM: Microscopio electrónico de barrido, EDS: Espectroscopia de energía dispersa, PPF3: Péptido del cacahuate con MW < 3 kDa, PPF3-Zn: Quelato del péptido del cacahuate con MW < 3 kDa y Zn2+, MW: Peso molecular.
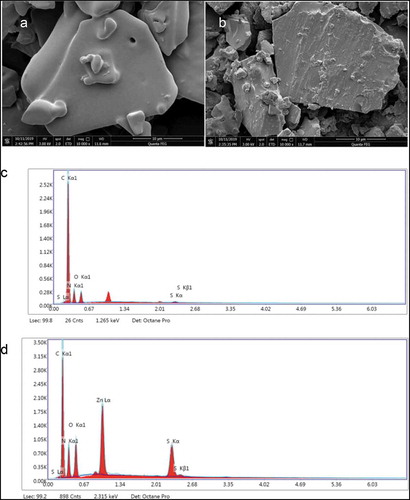
The elemental compositions of PPF3 and PPF3-Zn were analyzed by EDS. The EDS spectra were shown in ,), and the analysis results are shown in . PPF3 was found to contain C, N, O, and S, but did not contain Zn. In addition to these elements, PPF3-Zn also contained Zn, which confirmed the presence of Zn in the peptide–zinc chelate. Quantitative elemental analysis showed that the percentage weight of zinc in PPF3-Zn was 17.47%. The samples were obtained by precipitation in ethanol, which ruled out the effect of free zinc. The EDS results showed that a large number of Zn2+ ions and peanut peptides were chelated in the reaction.
Table 2. EDS analysis of PPF3 and PPF3-Zn.
Tabla 2. Análisis EDS de PPF3 y PPF3-Zn.
3.6. Fluorescence spectroscopy
Fluorescence spectroscopy is an effective method to analyze protein molecular conformation (S. Y. Wang et al., Citation2012). By selecting appropriate excitation and emission spectra, conformational changes in protein molecules can be determined according to spectral curves. Tryptophan (Trp), tyrosine (Tyr), and phenylalanine (Phe) in proteins have different fluorescence excitation and emission spectra with different side-chain chromogenic groups (Zhao et al., Citation2014). When the peptide molecules chelate with some metal ions, the structure of some peptide fluorescent groups might be changed, which alters the fluorescence characteristics of the peptide.
Differences in the fluorescence spectra of PPF3 and PPF3-Zn are shown in . After adding Zn2+, the fluorescence peak generated by Trp was redshifted from 353.03 to 356.96 nm, and the fluorescence peak generated by Tyr also changed slightly. The fluorescence intensity of PPF3-Zn was significantly lower than that of PPF3 (Makowska et al., Citation2016), which indicated that binding with zinc ions led to folding and fluorescence quenching of PPF3. Meanwhile, PPF3 reacted with Zn2+ to establish new chromogenic group structures, which led to changes in the energy of the excited state (Uppal et al., Citation2008; Wu et al., Citation2012). The spectra showed that chelation might be caused by the participation of NH2 groups in the reaction, or the interaction of side-chain residues.
3.7. TG analysis
In TG analysis, the mass of a substance is measured at different temperatures to determine changes in its chemical structure. TG analysis mainly uses the relationship between temperature and the physical properties of chelates to determine their geometric configuration. Different metal–peptide chelates have fixed melting points, and the thermal decomposition of chelates is usually divided into two steps. If these two characteristics are observed, then the formation of metal peptide chelates is proven (Predieri et al., Citation2003).
The TG curves of PPF3 and PPF3-Zn are shown in . In both cases, the sample mass decreased with increasing temperature. The curves were mainly divided into two steps, water loss before 105°C, and the gradual decomposition of organic components at temperatures higher than 200°C. The weight changes of PPF3 and PPF3-Zn in TG analysis showed similar water contents of about 6–9%. The temperature required to obtain the lowest mass fraction with no further change was 927°C for PPF3-Zn, which was significantly higher than that of PPF3 (780°C). This indicated that intermolecular and intramolecular forces between the peptides and zinc ion were increased after chelation. Therefore, the temperature required to break down molecular forces was higher. The results showed that the ash content of PPF3-Zn was higher than that of PPF owing to the presence of zinc.
4. Conclusion
In this study, zinc-chelating peptide was obtained from peanut protein by enzymatic hydrolysis. The results showed that peptide fraction PPF3 (<3 kDa) had the highest zinc chelation rate after ultrafiltration. Zinc caused peanut peptide folding, which resulted in microstructure differences between PPF3-Zn and PPF3. Furthermore, the formation of zinc–peptide chelates depended on the coordination of Zn2+ with hydroxyl oxygen, carboxyl oxygen, and amino nitrogen atoms, which are present in the peptide bond. The peptide–zinc chelates exhibited the dual effects of peptide and zinc. This study not only provides a feasible method for using peanut protein peptide as a new carrier for zinc supplements, but also provides a novel direction for peanut nutrition research. However, the peptide sequences with Zn-chelating capacities need to be characterized to analyze the relationship between their molecular structures and chelating ability. Furthermore, the gastrointestinal and in vivo absorption mechanism of PPF3-Zn must be studied.
Author’s contributions
Li Chen (30%), Bu Guanhao (30%), Chen Fusheng (30%), Li Tanghao (10%).
Disclosure statement
The authors declare there are no competing financial interests.
Additional information
Funding
References
- Ahmad, N., Sharma, S., Alam, M. K., Singh, V. N., Shamsi, S. F., Mehta, B. R., & Fatma, A. (2012). Rapid synthesis of silver nanoparticles using dried medicinal plant of Basil. Colloids and Surfaces B-Biointerfaces, 81(1), 81–86. https://doi.org/10.1016/j.colsurfb.2010.06.029
- Akbar, B., Niloufar, N., Abolfazl, M., Lofollah, S., Ali, K. Q., & Soheyla, V. (2013). Evaluation and comparison of zinc absorption level from 2-alkyle 3-hydroxy pyranon-zinc complexes and zinc sulfate in rat in vivo. Advanced Biomedical Research, 2(1), 77. https://doi.org/10.4103/2277-9175.116432
- Armas, A., Sonois, V., Mothes, E., Mazarguil, H., & Faller, P. (2006). Zinc(II) binds to the neuroprotective peptide humanin. Journal of Inorganic Biochemistry, 100(10), 1672–1678. https://doi.org/10.1016/j.jinorgbio.2006.06.002
- Bae, I. Y., Kim, H. Y., Lee, S., & Lee, H. G. (2011). Effect of the degree of oxidation on the physicochemical and biological properties of Grifola frondosa polysaccharides. Carbohydrate Polymers, 83(3), 1298–1302. https://doi.org/10.1016/j.carbpol.2010.09.037
- Blindauer, C. A., Harvey, I., Bunyan, K. E., Stewart, A. J., Sleep, D., Harrison, D. J., Berezenko, S., & Sadler, P. (2009). Structure, properties, and engineering of the major zinc binding site on human albumin. Journal of Biological Chemistry, 284(34), 23116–23124. https://doi.org/10.1074/jbc.M109.003459
- Chen, D., Liu, Z., Huang, W., Zhao, Y., Dong, S., & Zeng, M. (2013). Purification and characterisation of a zinc-binding peptide from oyster protein hydrolysate. Journal of Functional Foods, 5(2), 689–697. https://doi.org/10.1016/j.jff.2013.01.012
- Dam, H., Kristensen, G., Nielsen, G. K., Prange, I., & Sondergaard, E. (1956). Influence of varied levels of peanut oil and cholesterol on cholesterol and polyenoic acids in tissues of the chicks. Acta physiologica, 36(4), 319–328. https://doi.org/10.1111/j.1748-1716.1956.tb01328.x
- Depciuch, J., Sowa-Kućma, M., Nowak, G., Szewczyk, B., Doboszewska, U., & Parlinska-Wojtan, M. (2017). The role of zinc deficiency-induced changes in the phospholipid-protein balance of blood serum in animal depression model by Raman, FTIR and UV–vis spectroscopy. Biomedicine & Pharmacotherapy, 89(2017), 549–558. https://doi.org/10.1016/j.biopha.2017.01.180
- Gerbino, E., Mobili, P., Tymczyszyn, E., Fausto, R., & Gómez-Zavaglia, A. (2011). FTIR spectroscopy structural analysis of the interaction between Lactobacillus kefir S-layers and metal ions. Journal of Molecular Structure, 987(1–3), 186–192. https://doi.org/10.1016/j.molstruc.2010.12.012
- Guo, P., Qi, Y., Zhu, C., & Wang, Q. (2015). Purification and identification of antioxidant peptides from Chinese cherry (Prunus pseudocerasusLindl.) seeds. Journal of Functional Foods, 19(2015), 394–403. https://doi.org/10.1016/j.jff.2015.09.003
- Hajji, L., Boukir, A., Assouik, J., Lakhiari, H., Kerbal, A., Doumenq, P., Mille, G., & Carvalho, M. L. D. (2015). Conservation of Moroccan manuscript papers aged 150, 200 and 800years. Analysis by infrared spectroscopy (ATR-FTIR), X-ray diffraction (XRD), and scanning electron microscopy energy dispersive spectrometry (SEM–EDS). Spectrochimica Acta Part A: Molecular and Biomolecular Spectroscopy, 136(2015), 1038–1046. https://doi.org/10.1016/j.saa.2014.09.127
- Hedberg, Y., Herting, G., & Wallinder, I. O. (2011). Risks of using membrane filtration for trace metal analysis and assessing the dissolved metal fraction of aqueous media – A study on zinc, copper and nickel. Environmental Pollution, 159(5), 1144–1150. https://doi.org/10.1016/j.envpol.2011.02.014
- Huang, S. L., Zhao, L. N., Cai, X., Wang, S. Y., Huang, Y. F., Hong, J., & Rao, P. F. (2015). Purification and characterisation of a glutamic acid-containing peptide with calcium-binding capacity from whey protein hydrolysate. Journal of Dairy Research, 82(1), 29–35. https://doi.org/10.1017/s0022029914000715
- Jin, Y. G., Fu, W. W., & Ma, M. H. (2011). Preparation and structure characterization of soluble bone collagen peptide chelating calcium. African Journal of Biotechnology, 10(50), 11. https://doi.org/10.5897/ajb10.1923
- Li, B., He, H., Shi, W., & Hou, T. (2019). Effect of duck egg white peptide-ferrous chelate on iron bioavailability in vivo and structure characterization. Journal of the Science of Food and Agriculture, 99(4), 1834–1841. https://doi.org/10.1002/jsfa.9377
- Liao, W., Lai, T., Chen, L., Fu, J., Sreenivasan, S. T., Yu, Z., & Ren, J. (2016). Synthesis and characterization of a walnut peptides–zinc complex and its antiproliferative activity against human breast carcinoma cells through the induction of apoptosis. Journal of Agricultural & Food Chemistry, 104(7), 849–859. https://doi.org/10.1021/acs.jafc.5b04924
- Makowska, J., Zamojc, K., Wyrzykowski, D., Uber, D., Wierzbicka, M., Wiczk, W., & Chmurzynski, L. (2016). Binding of Cu(II) ions to peptides studied by fluorescence spectroscopy and isothermal titration calorimetry. Spectrochimica Acta Part A: Molecular and Biomolecular Spectroscopy, 153(2016), 451–456. https://doi.org/10.1016/j.saa.2015.08.016
- Miquel, E., & Farré, R. (2007). Effects and future trends of casein phosphopeptides on zinc bioavailability. Trends in Food Science & Technology, 18(3), 139–143. https://doi.org/10.1016/j.tifs.2006.11.004
- Muyonga, J. H., Cole, C. G. B., & Duodu, K. G. (2004). Characterisation of acid soluble collagen from skins of young and adult Nile perch (Lates niloticus). Food Chemistry, 85(1), 81–89. https://doi.org/10.1016/j.foodchem.2003.06.006
- Nune, M., Krishnan, U. M., & Sethuraman, S. (2016). PLGA nanofibers blended with designer self-assembling peptides for peripheral neural regeneration. Materials Science and Engineering C-Materials for Biological Applications, 62(2016), 329–337. https://doi.org/10.1016/j.msec.2016.01.057
- Oliveira, R. C., Hammer, P., Guibal, E., Taulemesse, J. M., & Garcia, O. (2014). Characterization of metal–biomass interactions in the lanthanum(III) biosorption on Sargassum sp. using SEM/EDX, FTIR, and XPS: Preliminary studies. Chemical Engineering Journal, 239(2014), 381–391. https://doi.org/10.1016/j.cej.2013.11.042
- Predieri, G., Tegoni, M., Cinti, E., Leonardi, G., & Ferruzza, S. (2003). Metal chelates of 2-hydroxy-4-methylthiobutanoic acid in animal feeding: Preliminary investigations on stability and bioavailability. Journal of Inorganic Biochemistry, 95(2–3), 221–224. https://doi.org/10.1016/s0162-0134(03)00067-9
- Qian, J. Y., Chen, W., Zhang, W. M., & Zhang, H. (2009). Adulteration identification of some fungal polysaccharides with SEM, XRD, IR and optical rotation: A primary approach. Carbohydrate Polymers, 78(3), 620–625. https://doi.org/10.1016/j.carbpol.2009.05.025
- Storcksdieck, S., Bonsmann, G., & Hurrell, R. F. (2007). Iron‐binding properties, amino acid composition, and structure of muscle tissue peptides from in vitro digestion of different meat sources. Journal of Food Science, 72(1), S019–S029. https://doi.org/10.1111/j.1750-3841.2006.00229.x
- Swain, J. H., Tabatabai, L. B., & Reddy, M. B. (2002). Histidine content of low-molecular-weight beef proteins influences nonheme iron bioavailability in Caco-2 cells. The Journal of Nutrition, 132(2), 245–251. https://doi.org/10.1093/jn/132.2.245
- Taylor, P. G., Martínez-Torres, C., Romano, E. L., & Layrisse, M. (1986). The effect of cysteine-containing peptides released during meat digestion on iron absorption in humans. The American Journal of Clinical Nutrition, 3(1), 68–71. https://doi.org/10.1093/ajcn/43.1.68
- Udechukwu, M. C., Collins, S. A., & Udenigwe, C. C. (2016). Prospects of enhancing dietary zinc bioavailability with food-derived zinc-chelating peptides. Food & Function, 7(10), 4137. https://doi.org/10.1039/c6fo00706f
- Uppal, R., Lakshmi, K. V., & Valentine, A. M. (2008). Isolation and characterization of the iron-binding properties of a primitive monolobal transferrin from Ciona intestinalis[J]. JBIC Journal of Biological Inorganic Chemistry, 13(6), 873–885. https://doi.org/10.1007/s00775-008-0375-6
- Wang, C., Li, B., & Ao, J. (2012). Separation and identification of zinc-chelating peptides from sesame protein hydrolysate using IMAC-Zn2⁺ and LC-MS/MS. Food Chemistry, 134(2), 1231–1238. https://doi.org/10.1016/j.foodchem.2012.02.204
- Wang, C., Wang, C., Li, B., & Li, H. (2014). Zn(II) chelating with peptides found in sesame protein hydrolysates: Identification of the binding sites of complexes. Food Chemistry, 165(2014), 594–602. https://doi.org/10.1016/j.foodchem.2014.05.146
- Wang, S. Y., Zhu, B. B., Li, D. Z., Fu, X. Z., & Shi, L. (2012). Preparation and characterization of TIO2/SPI composite film. Materials Letters, 83(none), 42–45. https://doi.org/10.1016/j.matlet.2012.05.104
- Wang, X., Zhou, J., Tong, P. S., & Mao, X. Y. (2011). Zinc-binding capacity of yak casein hydrolysate and the zinc-releasing characteristics of casein hydrolysate-zinc complexes. Journal of Dairy Science, 94(6), 2731–2740. https://doi.org/10.3168/jds.2010-3900
- Warren, F. J., Gidley, M. J., & Flanagan, B. M. (2015). Infrared spectroscopy as a tool to characterise starch ordered structure- a joint FTIR-ATR, NMR, XRD and DSC study. Carbohydrate Polymers, 139(2015), 35–42. https://doi.org/10.1016/j.carbpol.2015.11.066
- Wu, H., Liu, Z., Zhao, Y., & Zeng, M. (2012). Enzymatic preparation and characterization of iron-chelating peptides from anchovy (Engraulis japonicus) muscle protein. Food Research International, 48(2), 435–441. https://doi.org/10.1016/j.foodres.2012.04.013
- Xu, N., Ren, Z., Zhang, J., Song, X., Gao, Z., Jing, H., Li, S., Wang, S., & Jia, L. (2017). Antioxidant and anti-hyperlipidemic effects of mycelia zinc polysaccharides by Pleurotus eryngii var. tuoliensis. International Journal of Biological Macromolecules, 95(2017), 204–214. https://doi.org/10.1016/j.ijbiomac.2016.11.060
- Yan, X., Ye, R., & Chen, Y. (2015). Blasting extrusion processing: The increase of soluble dietary fiber content and extraction of soluble-fiber polysaccharides from wheat bran. Food Chemistry, 180(2015), 106–115. https://doi.org/10.1016/j.foodchem.2015.01.127
- Yan, Z., Wen, X., Kang, Y., & Chu, W. (2016). Intermolecular interactions of α-amino acids and glycyl dipeptides with the drug domiphen bromide in aqueous solutions analyzed by volumetric and UV–vis spectroscopy methods. The Journal of Chemical Thermodynamics, 101(2016), 300–307. https://doi.org/10.1016/j.jct.2016.06.018
- Zhang, C., Gao, Z., Hu, C., Zhang, J., Sun, X., Rong, C., & Jia, L. (2017). Antioxidant, antibacterial and anti-aging activities of intracellular zinc polysaccharides from Grifola frondosa SH-05. International Journal of Biological Macromolecules, 95(2017), 778–787. https://doi.org/10.1016/j.ijbiomac.2016.12.003
- Zhang, Y., Liu, J., Lu, X., Zhang, H., Wang, L., Guo, X., Qi, X., & Qian, H. (2013). Isolation and identification of an antioxidant peptide prepared from fermented peanut meal using fermentation. International Journal of Food Properties, 17(6), 1237–1253. https://doi.org/10.1080/10942912.2012.675605
- Zhao, L., Cai, X., Huang, S., Wang, S., Huang, Y., Hong, J., & Rao, P. (2015). Isolation and identification of a whey protein-sourced calcium-binding tripeptide Tyr-Asp-Thr. International Dairy Journal, 40(2015), 16–23. https://doi.org/10.1016/j.idairyj.2014.08.013
- Zhao, L., Huang, S., Cai, X., Hong, J., & Wang, S. (2014). A specific peptide with calcium chelating capacity isolated from whey protein hydrolysate. Journal of Functional Foods, 10(2014), 46–53. https://doi.org/10.1016/j.jff.2014.05.013