ABSTRACT
Bacillus coagulans BC198 and Lactobacillus paracasei S38 in combination reduce body fat accumulation and modulate gut microbiota. This study investigated the effects of two single strains (Bacillus coagulans BC198 and Lactobacillus paracasei S38) at a level of 5 × 109 CFU and a mixture of these two strains (1 x 108 CFU) on body fat accumulation and gut microbiota in rats. In contrast to high-fat control, L. paracasei S38 intakes resulted in significant (p < .05) reductions in total visceral fat (−15%) and liver lipids (−19%). The supplementation of the multi-strain mixture at a relatively lower dose was more efficient (p < .05) in reducing feed efficiency (−17%), total visceral fat (−30%), and liver lipids (−32%). A higher concentration of total short-chain fatty acids and a higher proportion of Bacteroidetes were also observed in the fecal samples of the multi-strain group. Consumption of multiple strains of B. coagulans BC198 and L. paracasei S38 appeared to show greater efficacy than single strains even at a relatively low dose.
RESUMEN
El presente estudio se propuso investigar los efectos de dos cepas individuales (Bacillus coagulans BC198 y Lactobacillus paracasei S38) a un nivel de 5 × 109 UFC y de una mezcla de estas dos cepas (1 x 108 UFC) en la acumulación de grasa corporal y la microbiota intestinal de las ratas. En contraste con el control de grasas, la ingesta de L. paracasei S38 provocó reducciones significativas (p < .05) de la grasa visceral total (−15%) y los lípidos hepáticos (−19%). La suplementación con la mezcla de múltiples cepas a una dosis relativamente más baja fue más efectiva (p < .05) para reducir la eficiencia de la alimentación (−17%), la grasa visceral total (−30%) y los lípidos hepáticos (−32%). Asimismo, se observó mayor concentración de ácidos grasos totales de cadena corta (SCFA) y mayor proporción de Bacteroidetes en las muestras fecales del grupo que consumió cepas múltiples. En conclusión, el consumo de cepas múltiples de B. coagulans BC198 y L. paracasei S38 parece tener mayor eficacia que las cepas individuales, incluso a una dosis relativamente baja, para reducir la acumulación de grasa corporal y modular la microbiota intestinal.
1. Introduction
Overweight and obesity have been defined as abnormal or excessive fat accumulation that poses a risk to public health. It is in general caused by an energy imbalance between calories consumed and calories expended, and is among the most significant contributors to ill health.
Modification of gut microbiota composition was a factor in inducing or suppressing obesity (Dahiya et al., Citation2017). Previous studies have indicated that obesity has an association with gut microbiota (Ley et al., Citation2006). Dysbiosis of intestinal microbiota might predispose to higher risks of colorectal cancer, type 2 diabetes, and cardiovascular disease (Shreiner et al., Citation2015).
Probiotic supplementation could increase the levels of fecal short-chain fatty acids (SCFAs), help modulate gut microbiome in a positive way, and have been regarded as important assets in the management of obesity (Dahiya et al., Citation2017). The increasing level of SCFAs might elevate release of the hormone glucagon-like peptide-1 (GLP-1), subsequently resulting in decreased food consumption and body weight gain (Yadav et al., Citation2013). Long-term ingestion of Bacillus coagulans and Lactobacillus paracasei could regulate gut microbes and modulate triglyceride deposition into adipocytes (Aminlari et al., Citation2018). Bacillus coagulans was also found capable of exerting an antagonistic effect on intestinal coliform contributing to the displacement of a considerable population of coliforms in gut (Haldar & Gandhi, Citation2016). L. paracasei ST11 may affect autonomic nerve to enhance lipolysis and reduce body weight in rats (Tanida et al., Citation2008). L. paracasei subsp. paracasei F19 could act as a regulator of triglyceride deposition via modulating gut microbes (Aronsson et al., Citation2010). Another study also indicated that L. paracasei NFRI 7415 may improve intestinal microflora and immune function in humans (Komatsuzaki et al., Citation2017).
Most research on the efficacy of probiotics usually utilized single strains. Based on a limited number of studies, a combination of different probiotics might be more beneficial than single-strains (Chapman et al., Citation2011; Yoo et al., Citation2013). However, no conclusive remark on whether it was attributed to synergistic interactions between strains or actually a consequence of the higher dose of strains used in those studies have yet been clarified.
The purpose of this experiment was to compare the abilities of L. paracasei S38, B. coagulans BC198, and their combination (at a ratio of 1:1, w/w) at a relatively lower dose on reducing the body fat accumulation of rat fed high-fat diets. More specifically, the amounts of visceral fat pads, liver lipid, fecal fat, and fecal SCFAs as well as gut microbiota were evaluated. The changes in gut microbiota in rats fed with single- and multi-strain probiotics were also discussed.
2. Materials and methods
2.1. Animal and experimental design
A total of 30 Sprague-Dawley male rats (8 weeks old) were purchased from the animal department of BioLASCO, Taipei, Taiwan. The animal study protocol was approved by the Animal Care and Use Committee of National Chung Hsing University and the laboratory animals were handled in accordance with the institutional ethical guideline. The rats were individually housed in stainless steel screen-bottomed cages and placed in an animal room at 22 ± 1°C with a12:12 h light/dark cycle.
n the present study, a pure strain of B. coagulans 198 (1 × 1010 CFU/g) and L. paracasei S38 (1 × 1010 CFU/g) were isolated from green malt and feces samples of healthy adults, respectively. All bacterial strain powders were obtained from Syngen Biotech Co., Ltd. (Taiwan). After acclimation for 1 week, the animals weighing 352.80–402.40 g were divided into five weight classes of 6 animals each. The rats in each weight class were randomly assigned to one of the five groups, including one normal control group (NC) and four high-fat diet groups. As shown in , high-fat diet consisted of powdered chow, sweetened condensed milk, soybean oil, and lard. The four high-fat diet groups included one high-fat (HF) control and three sample groups, namely, BC198, S38, and BC198S38. The BC198 and S38 groups were given B. coagulans BC198 (5 × 109 CFU) and L. paracasei S38 (5 × 109 CFU), respectively, at a level of 1 g/kg bw. The BC198S38 group was supplemented with a mixture of B. coagulans BC198 and L. paracasei S38 (at 1:1 ratio, a total of 1 × 108 CFU) at a level of 0.02 g/kg bw. A relatively lower dose of multi-strain mixture was used in this experiment. All probiotic samples were suspended in 1 mL of water, and were given to the corresponding sample group daily.
Table 1. Composition of experimental diets.
Tabla 1. Composición de las dietas experimentales
Food intakes and body weights were recorded daily. Feces were collected during the last 3 days and stored at ‐20°C until analyzed. At the end of experimental periods, animals fasted for 12 h were then euthanized by carbon dioxide. After laparotomy, visceral fat pads were excised, blot dried, and weighed.
2.2. Measurement of feed efficiency
Feed efficiency for each animal group within the experimental period was determined by the following formula:
Feed efficiency = body weight change (g)/food consumption (g) × 100%
2.3. Measurement of total Visceral fat
Based on the methods of Chu et al. (Citation2014), total visceral fat, which was defined as the sum of the perirenal, mesenteric, and epididymal fat depots, were collected and weighted:
Total visceral fat % = total visceral fat (g)/final body weight (g) × 100%
2.4. Determination of liver lipids
According to the methods described by Folch et al. (Citation1957) with slight modifications, total lipids in the lyophilized liver sample were extracted with a hexane:methanol (2:1, v/v) mixture. Liver total lipids were quantified gravimetrically after desolventization.
2.5. Determination of fecal fats and moisture
Based on the methods described by Folch et al. (Citation1957), crude fats in the dried fecal samples were extracted with a hexane:methanol (2:1, v/v) mixture and were quantified gravimetrically. Fecal moisture content was measured by drying the fecal samples on foil trays to a constant weight in an air-oven at the temperature of 105°C.
2.6. Analysis of gut microbiota
Fresh fecal samples collected on the last day of the experimental period were analyzed for the gut microbiome. The gut microbiome content was analyzed with the 16S rRNA gene sequencing and bioinformatics analysis. More specifically, bacterial DNA was extracted from fresh fecal samples using the CatchGeneTM Stool DNA kit (CatchGene Co., Ltd, Taiwan) following the manufacturer’s recommendations. After sequencing the amplicon with 300bp paired-end raw reads, the entire paired-end reads were assembled using FLASH v.1.2.7 (Magoc & Salzberg, Citation2011). Demultiplexing was performed based on barcode identification. Low-quality reads (Q < 20) were discarded using the QIIME (Version 1.9.1, University of Colorado, Boulder, CO, USA) software package. Effective tags obtained from chimera detection using UCHIME were filtered from the data set. The sequences were analyzed and clustered into 97% identity operational taxonomic units (OTUs) using the UPARSE function in the USEARCH v.7 pipeline (Edgar, Citation2013).
Taxonomy classification of representative sequences was determined by employing the RDP classifier (v.2.2) algorithm (Wong et al., Citation2016). Multiple sequence alignment was conducted using the PyNAST software (v.1.2) to analyze the sequence similarities among different OTUs (Ley et al., Citation2005). Principal coordinate analysis (PCoA) was performed to obtain principal coordinates for visualization of patterns and multidimensional data. Linear discriminant analysis (LDA) effect size (LEfSe) was used to identify the statistically significant bacterial taxa between groups. The non-parametric Kruskal-Wallis test and Wilcoxon rank-sum test were applied in the LEfSe analysis to detect differentially abundant taxa among various groups. Threshold on the logarithmic LDA score for discriminative features was set to 4.0.
2.7. Determination of SCFAs
According to the methods of Kao et al. (Citation2018), fresh fecal samples were homogenized with cold saline (0.9%, w/v) at a ratio of 1:10 (w/v). Fecal homogenates were centrifuged at 1006 g for 10 min, 2 mL of the supernatant was acidified with 10 μL of internal standard (isocaproic acid) and 20 μL of 50% (w/v) sulfuric acid. After extracting with diethyl ether, the SCFAs in the ether layer (1 μL) was analyzed by a column (Agilent J and W HP-INNO Wax GC Column, 30 m, 0.25 mm. 0.25 μm). Helium gas was supplied as the carrier in a constant flow mode of 7 mL/min. The conditions were as follows: the initial oven temperature was set at 80°C for 1 min and raised until 140°C at a rate of 20°C/min, then maintained at 140°C for another 1 min and raised again to 220°C at a rate of 20°C/min, and lastly held at 220°C for 2 mins; the temperature of the injector and detector were 140°C and 250°C, respectively.
2.8. Statistical analysis
All results (means ± standard deviation) were analyzed by one-way ANOVA using the SPSS statistics program (version 20.0; SPSS, Armonk, NY, USA). The value of p < .05 was regarded as statistically significant.
3. Results and discussion
3.1. Body weight gain, food intake, and feed efficiency
After a 12-week experimental period, shows that the HF control group (286.5 g) presented a markedly (p < .05) higher weight gain than the NC group (190.8 g), supporting that the high-fat experimental diet () could effectively induce obesity. As compared to the HF control group, no apparent changes in weight gain were noted in both BC198 and S38 groups, excepting that a significant (p < .05) decline in the body weight gain (−24%) was observed in the BC198S38 group (217.9 g). The results denoted that the consumption of the mixture of B. coagulans BC198 and L. paracasei S38 was capable of conferring a body weight reduction more effectively.
Table 2. Summary of body weights, total food intake, total calorie intakes, and feed efficiency ratio among different groups.
Tabla 2. Resumen del peso corporal, la ingesta total de alimentos, la ingesta total de calorías y la relación de eficiencia alimentaria en los diferentes grupos
The total food intake in the NC group (2126.2 g) was significantly (p < .05) higher than those of the HF groups (1694.3–1869.9 g). However, significantly (p < .05) higher amounts of total calorie intakes (7285.6–8040.6 g) were observed in the HF groups relative to the NC group (7122.8 g). Ingestion of high-fat diet might probably facilitate the development of short-term positive energy balance, thus influencing satiation and the strength of post-ingestive appetite inhibition (Blundell & Macdiarmid, Citation1997).
As presented in , the four HF groups were found to have apparently (p < .05) higher feed efficiencies (12.7–15.3) than the NC group. Fat component was obviously a major factor leading to a change in feed efficiency. As compared to the HF control, a significant (p < .05) decline (−17%) in the feed efficiency of BC198S38 group was noted. These results implicated that taking the mixture of B. coagulans BC198 and L. paracasei S38 would reduce feed efficiency more effectively.
3.2. Visceral fat weight
reveals that the total visceral fat accumulation in rats from the HF control (11.3 g/100 g) and other three sample groups (7.9–10.4 g/100 g) was markedly (p < .05) higher than that fed the NC diet (5.5 g/100 g). This indicated that the high-fat diet could effectively induce visceral fat accumulation in rats. The apparent differences in visceral fat sizes were visually presented in .
Table 3. Relative size of visceral fat pads among different groups.
Tabla 3. Tamaño relativo de los panículos de grasa visceral de los diferentes grupos
Figure 1. Visceral fats mass among different groups. (A) perirenal fat; (B) mesenteric fat; (C) epididymal fat.
Figura 1. Masa de grasa visceral de los diferentes grupos. (A) grasa perirrenal; (B) grasa mesentérica; (C) grasa epididimal
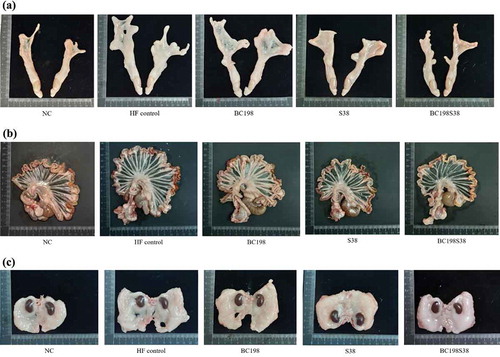
The amounts of visceral fats between the HF control and BC198 groups were comparable to each other (). Compared with the HF control group, the levels of epididymal fat and total visceral fat in the S38 group were found to be markedly (p < .05) decreased by about 26% and 15%, respectively. It seemed that the administration of L. paracasei S38 had a pronounced effect on reducing body fat accumulation. These results were in agreement with the findings from Tanida et al. (Citation2008) that the consumption of L. paracasei at a dose of 5 × 109 CFU could reduce body weight and abdominal fat weight.
In the BC198S38 group, the levels of perirenal, epididymal, and total visceral fat pads were apparently (p < .05) decreased (−36%, −26% and −30%, respectively) in contrast to the HF control. It was worth noting that the supply of multi-strain (i.e. a mixture of B. coagulans BC198 and L. paracasei S38) at a relatively lower dose (1 x 108 CFU) appeared to reduce the accumulation of visceral fats even more effectively over the supplementation of single strains (5 x 109 CFU). It was clear that the greater efficacy with multi-strain treatment was not due to a higher microbial dose. On the contrary, the results implied that it might be a consequence of the synergistic interactions between strains. The growth of B. coagulans would consume free oxygen in the intestine and facilitate the multiplication of anaerobic microbes including Lactobacillus spp. and Bifidobacterium spp. (Cao et al., Citation2020). A study by Haldar and Gandhi (Citation2016) also demonstrated that the incorporation of Bacillus organisms into a lactobacilli culture would act synergistically by producing acid and other metabolites, as a consequence to facilitate the lactobacilli growth in the gut of rats as well as to achieve more obvious health-promoting effects.
3.3. Lipid accumulation and morphology in liver
The liver lipid contents of BC198, S38, and BC198S38 groups were 250.7, 233.1, and 195.7 mg/g, respectively. There was a significant liver lipid reduction (p < .05) among the three sample groups (from −13% to −32%) in comparison with that of HF control group. It should be noted that the consumption of multi-strain (i.e. B. coagulans BC198 + L. paracasei S38) could render the most favorable outcome in terms of reducing liver lipid content. The concomitant decrease in the levels of liver lipid () and visceral fat () followed a parallel trend. Many researchers have also discussed that the inclusion of probiotics (B. coagulans, L. plantarum, L. paracasei, and others) in high-fat or high-cholesterol diets could effectively decrease lipid accumulation in liver (Aminlari et al., Citation2018; Chiu et al., Citation2006; El-Shafie et al., Citation2009; Kim et al., Citation2017).
Figure 2. Variations in (A) total lipids and (B) morphology of livers among different diet groups. CV represents the central vein. The black and green arrow indicate areas of inflammation and lipid accumulation, respectively.
Figura 2. Variaciones de (A) los lípidos totales y (B) la morfología de los hígados entre los diferentes grupos de dieta. El CV representa la vena central. Las flechas negra y verde indican las zonas de inflamación y acumulación de lípidos, respectivamente
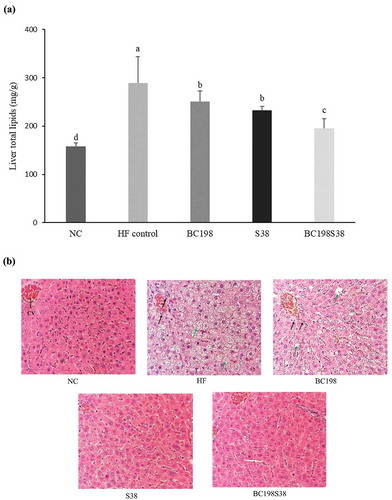
As illustrated in , the results of H&E staining of liver tissue. No abnormal change was observed in the hepatocytes of NC group. As for the liver of rats in HF control, the liver cells were generally enlarged and abounded with lipid deposition, especially around the central vein. High-fat diet intake is one of the leading causes of excessive fat accumulation in liver (Inoue et al., Citation2005). The liver tissues of the rats supplemented with probiotics demonstrated improvement in lipid deposition, especially those fed the single strain (L. paracasei S38) and multi-strain sample.
3.4. Assessments on fecal moisture, weight, fat, and SCFAs
In , fresh fecal weight in the NC group (11.7 g/day) was 2.2–2.4 folds higher (p < .05) than those of the four HF groups (4.8–5.3 g/day). It was worth noting that there were no substantial differences in fecal moisture levels among all experimental groups. Furthermore, the fecal fat excretion in the HF control (45.7 mg/g) were significantly (p < .05) elevated by 52% as compared with the NC group (30.0 mg/g). The increment of fecal fat output was probably associated with the higher fat contents in HF diets. Among the four HF groups, a markedly higher (p < .05) fecal fat excretion (about 1.1-fold) was observed in the BC198S38 group (50.6 mg/g). As previously reported by Jun et al. (Citation2010), a higher fecal fat output was basically associated with a lesser extent of dietary fat absorption. These results demonstrated that the feeding of multi-strain mixture would lead to a higher fecal fat output in this study.
Table 4. Variations in levels of fecal moisture, fecal weight, fecal fat, and fecal SCFAs among different groups.
Tabla 4. Variaciones de los niveles de humedad fecal, peso fecal, grasa fecal y SCFA fecales de los diferentes grupos
The fecal SCFA profiles in revealed that the administration of HF control diet would result in significant (p < .05) decrease in the total SCFA levels in comparison to the NC diet. Our results demonstrated the capability of the two microbial strains used in the present study in restoring the low abundance of SCFA-production induced by HF diets. More specifically, drastic (p < .05) elevations in the concentrations of acetic acid (2.0- to 2.2-fold) and total SCFAs (1.7- to 1.8-fold) in the L. paracasei S38 and multi-strain groups were observed relative to the HF group. The consumption of L. paracasei S38 would render a higher production of total SCFAs in gut. According to Aoki et al. (Citation2017), acetate levels showed a significant negative correlation with the amount of visceral fat and adipocyte size.
It was interesting to note that the inclusion of B. coagulans BC198 in the multi-strain group (BC198S38) would result in two-fold increase in the butyric acid concentrations among the four HF groups. A strategy to supplement a mixture of Lactobacilli spp. and Bifidobacterium spp. has been found capable of contributing positively to the production of SCFAs (LeBlanc et al., Citation2017). As previously reported, short-chain fatty acid-mediated sympathetic activation and suppressed insulin signaling in adipocytes, which inhibited fat accumulation in adipose tissue and promoted the metabolism of unincorporated lipids, thereby controlling body energy utilization (Kimura et al., Citation2011, Citation2013). It was believed that SCFAs played an important role in maintaining metabolic homoeostasis.
3.5. Microbiota composition
As shown in , Firmicutes and Bacteroidetes were the major microbial phyla among the five groups. The HF diet apparently increased the relative proportion of Firmicutes and Bacteroidetes. Notably, the consumption of a mixture of B. coagulans BC198 and L. paracasei S38 effectively reduced the abundance of Firmicutes. The population of Bacteroidetes in BC198S38 group was higher than those of other HF groups.
Figure 3. Relative abundance of different phyla in the microbiota (A) Overall abundance, and (B) Firmicutes/Bacteroidetes ratio.
Figura 3. Abundancia relativa de diferentes filas en la microbiota (A) Abundancia general, y (B) Proporción de Firmicutes/Bacteroidetes.
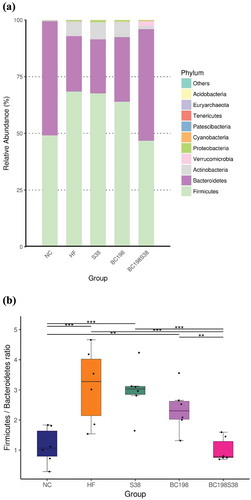
The Firmicutes/Bacteroidetes ratios among the NC, HF control, S38, BC198, and BC198S38 groups were found to be 1.1, 3.4, 3.0, 2.5 and 0.8, respectively. A significantly (p < .05) lower Firmicutes/Bacteroidetes ratio was observed in the BC198S38 group (). High-fat diet consumption increased the population of Firmicutes and reduced the population of Bacteroidetes (Ismail et al., Citation2011). It was also reported that the abundance of Firmicutes was positively associated with adiposity (Zhang et al., Citation2020). Nevertheless, other studies have found the negative or no correlation, suggesting that the association between obesity and gut microbiota were likely to be influenced by several factors (Fayrman et al., Citation2019). Thus, the relationship between the ratio of microbiota and obesity have so far been inconclusive.
The PCoA score plots () based on sequences demonstrated an apparent separation of community compositions in the fresh fecal samples between HF group and BC198S38 group. BC198S38 group had a similar structure to that of the NC group.
Figure 4. The PCoA score plots of fecal operational taxonomic units.
Figura 4. Gráfica de la puntuación del PCoA correspondiente a las unidades taxonómicas operacionales fecales
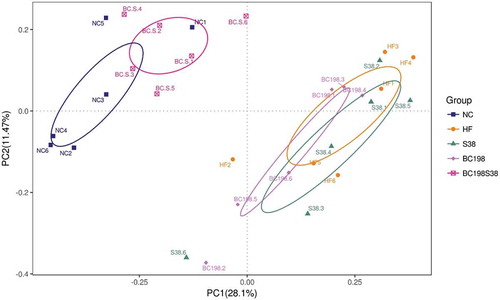
After the LEfSe analysis, and show the taxonomic shifts in microbiomes among all five groups. Results revealed that Lactobacillus reuteri, Clostridiaceae, and Clostridium sensu stricto 1 were the featured bacteria in the HF group Supplementation of multi-strain mixture brought down the abundance of these bacterial taxa to a level comparable to that observed in NC group. It has been demonstrated that L. reuteri played a role in inducing obesity and was found capable of causing weight gain in children suffering from Kwashiorkor disease (Harakeh et al., Citation2016). Some evidence suggested a positive relationship between high fat consumption and increased abundance of the family Clostridiaceae (Martinez-Guryn et al., Citation2018). Clostridium sensu stricto and Dorea was positively correlated with indicators of bodyweight, and might be potentially regarded as one of the biomarkers to predict obesity-related metabolic abnormalities (Zeng et al., Citation2019).
Figure 5. Taxonomic shifts in microbiomes among different groups. (A) Heatmap of fecal bacterial on relative abundance. (B) Taxonomic cladogram obtained using LEfSe analysis of the 16S sequences in fecal samples.
Figura 5. Cambios taxonómicos en los microbiomas de los diferentes grupos. (A) Mapa de calor de las bacterias fecales en abundancia relativa. (B) Cladograma taxonómico obtenido usando el análisis LEfSe de las secuencias 16S en muestras fecales
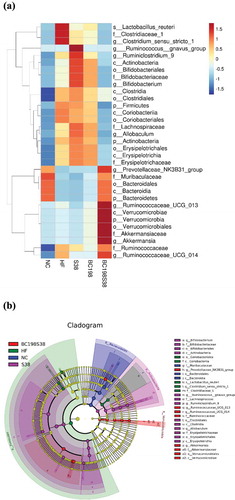
Next, notably higher relative abundances of Bacteroidetes, Muribaculaceae, and Prevotellaceae NK3B31 group were observed in both the NC and BC198S38 groups, meanwhile an opposite trend in the relative abundance of Firmicutes were seen. It was interesting to note that the results in and revealed that Verrucomicrobiales, Akkermansiaceae, and Akkermansia were the featured bacterial taxa in the BC198S38 group. It was believed that the higher abundance of genera Akkermansia and Bacteroides after the treatment with multi-strain mixture might play a beneficial role in murine reduced response to high-fat diet-induced obesity. An increase in abundance of Akkermansia spp. has been linked to improve metabolic disorders in obesity. For instance, Akkermansia muciniphila has been proven to be beneficial to improve the metabolic disorders associated with obesity (Xu et al., Citation2020).
According to the LDA scores as illustrated in , the specific bacterial taxa characterized in different groups showed that six taxa were affected by feeding HF control diet, and nine taxa were enriched in the BC198S38 group. In more detail, the most abundant genus in the HF control group was Clostridium sensu stricto 1 while those in the BC198S38 group included Prevotellaceae NK3B31 group, Ruminococcaceae UCG 013, and Akkermansia.
Figure 6. Histogram of the linear discriminant analysis scores computed for taxa differentially abundant among various groups. Thresholds > 4.0 are shown.
Figura 6. Histograma de las puntuaciones del análisis discriminante lineal computado para los taxones diferencialmente abundantes entre varios grupos. Se muestran los umbrales > 4.0
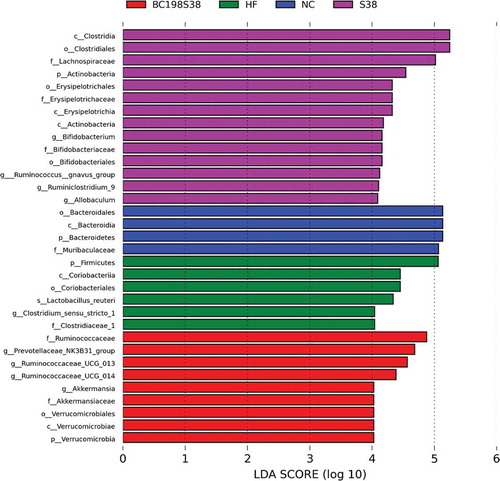
A further comparison of gut microbiota composition between the HF control and BC198S38 groups was shown in . The analysis on taxa abundance demonstrated that the HF control group had higher abundances of Lactobacillus, Collinsella, and Clostridium sensu stricto 1, whereas the BC198S38 group had Prevotellaceae NK3B31 group, Ruminococcaceae UCG 013, and Akkermansia to be the dominant taxa. The microbial composition of these two diet groups appeared to be distinct. It was inferred that the apparently higher fecal concentrations of total SCFAs in BC198S38 group () were in part attributed to the dominant abundance of Prevotellaceae NK3B31 group which was positively associated with increased SCFAs (Guo et al., Citation2019). It was reported that an elevation in SCFA production might be involved in signaling to the host by increasing fasting-induced adipocyte factor as well as reducing histone deacetylase activity and peroxisome proliferator-activated receptor gamma expression, thereby regulating metabolic physiology and decreasing fat accumulation (Lukovac et al., Citation2014; Xu et al., Citation2020). As compared to the bacterial profile in , a relatively lower diversity of microbial composition was observed in . Taken together, these findings suggested that the supplementation of multi-strain samples in rats fed high fat diet might assist to restore the balance of intestinal microbiome.
Figure 7. Variations in the composition of gut microbiota. (A) Changes between HF control and BC198S38 groups. (B) Changes between NC and BC198S38 groups.
Figura 7. Variaciones en la composición de la microbiota intestinal. (A) Cambios entre el control del HF y los grupos BC198S38. (B) Cambios entre los grupos NC y BC198S38
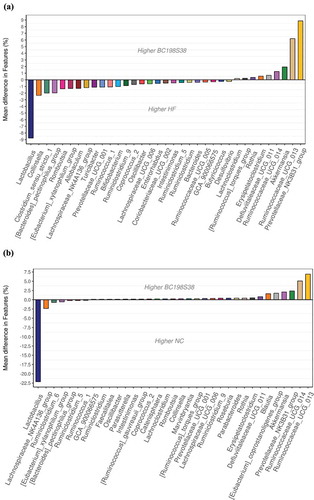
Previous studies have demonstrated that the consumption of high-fat diets would lead to dysbiosis within the gut and increased level of plasma endotoxin coinciding with general inflammation that eventually promoted metabolic disorder (Harakeh et al., Citation2016). A decrease in mucus thickness as well as increased gut permeability were also associated with obesity. It has been reported that certain species of gut bacteria (i.e. Akkermansia muciniphila) might interact with the host to decrease serum level of inflammatory cytokines, regulate gut permeability by tight-junction proteins, reverse obesity by mediating adipocyte metabolism, and also decrease lipid accumulation associated with the LDL receptor pathway via the reduction of apoB48 and apoB100 on LDLs (Everard et al., Citation2013; Xu et al., Citation2020). Based on these findings in the present study, it was inferred that the increased abundance of taxa, such as Akkermansia, Prevotellaceae NK3B31 group, in the BC198S38 group might stimulate SCFA production, alleviate gut disruption, and reduce the risk of obesity.
Limitations to this study include performing analyses on gut integrity and potential cellular mechanisms associated with the synergistic effect. Although our above findings have demonstrated that the supplementation of B. coagulans BC198 and L. paracasei S38 as a multi-strain mixture appeared to work synergistically to prevent obesity, detailed prospective studies on molecular mechanism associated with the synergistic effect are required in future studies for the understanding of the putative beneficial effects of multi-strain probiotics on obese.
4. Conclusion
The consumption of L. paracasei S38 was capable of reducing visceral fat and liver lipid. A multi-strain mixture (i.e. B. coagulans BC198 + L. paracasei S38) could likely be more beneficial than single strain to reduce visceral fat accumulation and liver lipid levels. It was also found to be more effective in increasing the levels of total SCFAs and population of Bacteroidetes in the fresh fecal samples. This multi-strain microbial mixture appeared to work synergistically and showed greater efficacy than their component strains administered separately. These results suggested that the supplementation of B. coagulans BC198 and L. paracasei S38 as a multi-strain mixture shall be able to be an up-and-coming way to promote body fat reduction, but clinical trials are needed to verify the effect.
Author contributions
Conceptualization, C.F.C. and C.E.H.; Methodology, C.E.H., F.J.D., F.N.Y., and S.H.W.; Formal Analysis, C.E.H., F.J.D., F.N.Y., and S.H.W; Investigation, C.E.H., F.N.Y., Y.C.T., W.J.C., and H.F.C.; Resources, C.F.C. and W.J.C; Data Curation, C.E.H, F.N.Y., and Y.C.T.; Writing – Original Draft Preparation, C.E.H., F.J.D., and H.F.C.; Writing – Review & Editing, C.F.C., C.E.H., F.N.Y., and Y.C.T.; Visualization, C.F.C., C.E.H., and F.N.Y.; Supervision, C.F.C., and W.J.C.; Project Administration, C.F.C. All authors have read and agreed to the published version of the manuscript.
Acknowledgments
This work was supported by the Ministry of Science and Technology of the Republic of China (MOST 106-2320-B-005-006-MY3; MOST 109-2320-B-005-002). Authors thank Linn Chuah, Jia-Ting Lin, and Chiou-Yeong Saw for their technical supports. Sequence data have been deposited in the NCBI Sequence Read Archive (SRA) database under BioProject PRJNA649374 (SRA project accession number, SRR12342615; sample accession numbers, SAMN15665186-SAMN15665187, SAMN15665189-SAMN15665190, SAMN15665192, SAMN15665202-SAMN15665206, SAMN15665210, SAMN15665212- SAMN15665215, SAMN15665218-SAMN15665224, SAMN15665333-SAMN15665334, SAMN15665336-SAMN15665337, SAMN15665354-SAMN15665355, SAMN15665387, SAMN15665389).
Disclosure statement
No potential conflict of interest was reported by the authors.
Additional information
Funding
References
- Aminlari, L., Shekarforoush, S. S., Hosseinzadeh, S., Nazifi, S., Sajedianfard, J., & Eskandari, M. H. (2018). Effect of probiotics Bacillus coagulans and Lactobacillus plantarum on lipid profile and feces bacteria of rats fed cholesterol-enriched diet. Probiotics and Antimicrobial Proteins, 11(4), 1163–1171. https://doi.org/10.1007/s12602-018-9480-1
- Aoki, R., Kamikado, K., Suda, W., Takii, H., Mikami, Y., Suganuma, N., Hattori, M., & Koga, Y. (2017). A proliferative probiotic Bifidobacterium strain in the gut ameliorates progression of metabolic disorders via microbiota modulation and acetate elevation. Scientific Reports, 7(1), 43522. https://doi.org/10.1038/srep43522
- Aronsson, L., Huang, Y., Parini, P., Korach-André, M., Håkansson, J., Gustafsson, J. Å., Pettersson, S., Arulampalam, V., & Rafter, J. (2010). Decreased fat storage by Lactobacillus paracasei is associated with increased levels of angiopoietin-like 4 protein (ANGPTL4). PLoS One, 5(9), 1–7. https://doi.org/10.1371/journal.pone.0013087
- Blundell, J. E., & Macdiarmid, J. I. (1997). Fat as a risk factor for overconsumption: Satiation, satiety, and patterns of eating. Journal of the American Dietetic Association, 97(7), 63–69. https://doi.org/10.1016/S0002-8223(97)00733-5
- Cao, J., Yu, Z., Liu, W., Zhao, J., Zhang, H., Zhai, Q., & Chen, W. (2020). Probiotic characteristics of Bacillus coagulans and associated implications for human health and diseases. Journal of Functional Foods, 64, 1–11. https://doi.org/10.1016/j.jff.2019.103643
- Chapman, C. M. C., Gibson, G. R., & Rowland, I. (2011). Health benefits of probiotics: Are mixtures more effective than single strains? European Journal of Nutrition, 50(1), 1–17. https://doi.org/10.1007/s00394-010-0166-z
- Chiu, C. H., Lu, T. Y., Tseng, Y. Y., & Pan, T. M. (2006). The effects of Lactobacillus-fermented milk on lipid metabolism in hamsters fed on high-cholesterol diet. Applied Microbiology and Biotechnology, 71(2), 238–245. https://doi.org/10.1007/s00253-005-0145-0
- Chu, H. F., Pan, M. H., Ho, C. T., Tseng, Y. H., Wang, W. W., & Chau, C. F. (2014). Variations in the efficacy of resistant maltodextrin on body fat reduction in rats fed different high-fat models. Journal of Agricultural and Food Chemistry, 62(1), 192–197. https://doi.org/10.1021/jf404809v
- Dahiya, D. K., Renuka, P. M., Shandilya, U. K., Dhewa, T., Kumar, N., Kumar, S., Puniya, A. K., & Shukla, P. (2017). Gut microbiota modulation and its relationship with obesity using prebiotic fibers and probiotics: A review. Frontiers in Microbiology, 8(563), 1–17. https://doi.org/10.3389/fmicb.2017.00563
- Edgar, R. C. (2013). UPARSE: Highly accurate OTU sequences from microbial amplicon reads. Nature Methods, 10(10), 996–998. https://doi.org/10.1038/nmeth.2604
- El-Shafie, H. A., Yahia, N. I., Ali, H. A., Khalil, F. A., El-Kady, E. M., & Moustafa, Y. A. (2009). Hypocholesterolemic action of Lactobacillus plantarum NRRL-B-4524 and Lactobacillus paracasei in mice with hypercholesterolemia induced by diet. Australian Journal of Basic and Applied Sciences, 3(1), 218–228. http://ajbasweb.com/old/ajbas/2009/218-228.pdf
- Everard, A., Belzer, C., Geurts, L., Ouwerkerk, J. P., Druart, C., Bindels, L. B., Guiot, Y., Derrien, M., Muccioli, G. G., Delzenne, N. M., de Vos, W. M., & Cani, P. D. (2013). Cross-talk between Akkermansia muciniphila and intestinal epithelium controls diet-induced obesity. Proceedings of the National Academy of Sciences of the United States of America, 110(22), 9066–9071. https://doi.org/10.1073/pnas.1219451110
- Fayrman, M., Galindo, R. J., Rubin, D. J., Mize, D. L., Anzola, I., Urrutia, M. A., Ramos, C., Pasquel, F. J., Haw, J. S., Vellanki, P., Wang, H., Albury, B. S., Weaver, R., Cardona, S., & Umpierrez, G. E. (2019). A randomized controlled trial on the safety and efficacy of exenatide therapy for the inpatient management of general medicine and surgery patients with type 2 diabetes. Diabetes Care, 42(3), 450–456. https://doi.org/10.2337/dc18-1760
- Folch, J., Lees, M., & Sloane Stanley, G. H. (1957). A simple method for the isolation and purification of total lipids from animal tissues. Journal of Biological Chemistry, 226(1), 497–509. https://www.jbc.org/content/226/1/497.full.pdf
- Guo, Y., Huang, C., Liu, L., Fu, X., Lu, Y., Zheng, J., Mei, Q., Huang, Z., Fan, J., Lu, L., & Zeng, Y. (2019). Paneth cell ablation aggravates pancreatic and intestinal injuries in a rat model of acute necrotizing pancreatitis after normal and high-fat diet. Mediators of Inflammation, 2019, 1–19. https://doi.org/10.1155/2019/8474523
- Haldar, L., & Gandhi, D. N. (2016). Effect of oral administration of Bacillus coagulans B37 and Bacillus pumilus B9 strains on fecal coliforms, Lactobacillus and Bacillus spp. in rat animal model. Veterinary World, 9(7), 766–772. https://doi.org/10.14202/vetworld.2016.766-772
- Harakeh, S. M., Khan, I., Kumosani, T., Barbour, E., Almasaudi, S. B., Bahijri, S. M., Alfadul, S. M., Ajabnoor, G., & Azhar, E. I. (2016). Gut microbiota: A contributing factor to obesity. Frontiers in Cellular and Infection Microbiology, 6(95), 1–11. https://doi.org/10.3389/fcimb.2016.00095
- Inoue, M., Ohtake, T., Motomura, W., Takahashi, N., Hosoki, Y., Miyoshi, S., Suzuki, Y., Saito, H., Kohgo, Y., & Okumura, T. (2005). Increased expression of PPARγ in high fat diet-induced liver steatosis in mice. Biochemical and Biophysical Research Communications, 336(1), 215–222. https://doi.org/10.1016/j.bbrc.2005.08.070
- Ismail, N. A., Ragab, S. H., Abd ElBaky, A., Shoeib, A. R., Alhosary, Y., & Fekry, D. (2011). Frequency of Firmicutes and Bacteroidetes in gut microbiota in obese and normal weight Egyptian children and adults. Archives of Medical Science, 7(3), 501–507. https://doi.org/10.5114/aoms.2011.23418
- Jun, S. C., Jung, E. Y., Kang, D. H., Kim, J. M., Chang, U. J., & Suh, H. J. (2010). Vitamin C increases the fecal fat excretion by chitosan in guinea-pigs, thereby reducing body weight gain. Phytotherapy Research, 24(8), 1234–1241. https://doi.org/10.1002/ptr.2970
- Kao, W. M., Chang, C. R., Chang, T. J., Li, S. Y., Chen, W. J., & Chau, C. F. (2018). Inclusion of fructooligosaccharide and resistant maltodextrin in high fat diets promotes simultaneous improvements on body fat reduction and fecal parameters. Molecules, 23(9), 1–9. https://doi.org/10.3390/molecules23092169
- Kim, S. J., Park, S. H., Sin, H. S., Jang, S. H., Lee, S. W., Kim, S. Y., Kwon, B., Yu, K. Y., Kim, S. Y., & Yang, D. K. (2017). Hypocholesterolemic effects of probiotic mixture on diet-induced hypercholesterolemic rats. Nutrients, 9(3), 1–10. https://doi.org/10.3390/nu9030293
- Kimura, I., Inoue, D., Maeda, T., Hara, T., Ichimura, A., Miyauchi, S., Kobayashi, M., Hirasawa, A., & Tsujimoto, G. (2011). Short-chain fatty acids and ketones directly regulate sympathetic nervous system via G protein-coupled receptor 41 (GPR41). Proceedings of the National Academy of Sciences of the United States of America, 108(19), 8030–8035. https://doi.org/10.1073/pnas.1016088108
- Kimura, I., Ozawa, K., Inoue, D., Imamura, T., Kimura, K., Maeda, T., Terasawa, K., Kashihara, D., Hirano, K., Tani, T., Takahashi, T., Miyauchi, S., Shioi, G., Inoue, H., & Tsujimoto, G. (2013). The gut microbiota suppresses insulin-mediated fat accumulation via the short-chain fatty acid receptor GPR43. Nature Communications, 4(1), 1–12. https://doi.org/10.1038/ncomms2852
- Komatsuzaki, N., Aoki-Yoshida, A., & Shima, J. (2017). Effects of live Lactobacillus paracasei NFRI 7415 on the intestinal immune system and intestinal microflora of mice. International Journal of Biotechnology and Food Science, 5(4), 56–62. https://doi.org/http://dx.doi.org/10.22158/fsns.v2n3p57
- LeBlanc, J. G., Chain, F., Martín, R., Bermúdez-Humarán, L. G., Courau, S., & Langella, P. (2017). Beneficial effects on host energy metabolism of short-chain fatty acids and vitamins produced by commensal and probiotic bacteria. Microbial Cell Factories, 16(1), 1–10. https://doi.org/10.1186/s12934-017-0691-z
- Ley, R. E., Bäckhed, F., Turnbaugh, P., Lozupone, C. A., Knight, R. D., & Gordon, J. I. (2005). Obesity alters gut microbial ecology. Proceedings of the National Academy of Sciences of the United States of America, 102(31), 11070–11075. https://doi.org/10.1073/pnas.0504978102
- Ley, R. E., Turnbaugh, P. J., Klein, S., & Gordon, J. I. (2006). Microbial ecology: Human gut microbes associated with obesity. Nature, 444(7122), 1022–1023. https://doi.org/10.1038/4441022a
- Lukovac, S., Belzer, C., Pellis, L., Keijser, B., de Vos, W. M., Montijn, R. C., & Roeselers, G. (2014). Differential modulation by Akkermansia muciniphila and Faecalibacterium prausnitzii of host peripheral lipid metabolism and histone acetylation in mouse gut organoids. mBio, 5 (4), e01438–14. 12. https://doi.org/10.1128/mBio.01438-14.
- Magoc, T., & Salzberg, S. L. (2011). FLASH: Fast length adjustment of short reads to improve genome assemblies. Bioinformatics, 27(21), 2957–2963. https://doi.org/10.1093/bioinformatics/btr507
- Martinez-Guryn, K., Hubert, N., Frazier, K., Urlass, S., Musch, M. W., Ojeda, P., Pierre, J. F., Miyoshi, J., Sontag, T. J., & Reardon, C. A. (2018). Small intestine microbiota regulate host digestive and absorptive adaptive responses to dietary lipids. Cell Host & Microbe, 23(4), 458–469. https://doi.org/10.1016/j.chom.2018.03.011
- Shreiner, A. B., Kao, J. Y., & Young, V. B. (2015). The gut microbiome in health and in disease. Current Opinion in Gastroenterology, 31(1), 69–75. https://doi.org/10.1097/MOG.0000000000000139
- Tanida, M., Shen, J., Maeda, K., Horii, Y., Yamano, T., Fukushima, Y., & Nagai, K. (2008). High-fat diet-induced obesity is attenuated by probiotic strain Lactobacillus paracasei ST11 (NCC2461) in rats. Obesity Research & Clinical Practice, 2(3), 159–169. https://doi.org/10.1016/j.orcp.2008.04.003
- Wong, M. L., Inserra, A., Lewis, M. D., Mastronardi, C. A., Leong, L., Choo, J., Kentish, S., Xie, P., Morrison, M., Wesselingh, S. L., Rogers, G. B., & Licinio, J. (2016). Inflammasome signaling affects anxiety- and depressive-like behavior and gut microbiome composition. Molecular Psychiatry, 21(6), 797–805. https://doi.org/10.1038/mp.2016.46
- Xu, Y., Wang, N., Tan, H. Y., Li, S., Zhang, C., & Feng, Y. (2020). Function of Akkermansia muciniphila in Obesity: Interactions with lipid metabolism, immune response and gut systems. Frontiers in Microbiology, 11(219), 1–12. https://doi.org/10.3389/fmicb.2020.00219
- Yadav, H., Lee, J. H., Lloyd, J., Walter, P., & Rane, S. G. (2013). Beneficial metabolic effects of a probiotic via butyrate-induced GLP-1 hormone secretion. Journal of Biological Chemistry, 288(35), 25088–25097. https://doi.org/10.1074/jbc.M113.452516
- Yoo, S. R., Kim, Y. J., Park, D. Y., Jung, U. J., Jeon, S. M., Ahn, Y. T., Huh, C. S., McGregor, R., & Choi, M. S. (2013). Probiotics L. plantarum and L. curvatus in combination alter hepatic lipid metabolism and suppress diet-induced obesity. Obesity, 21(12), 2571–2578. https://doi.org/10.1002/oby.20428
- Zeng, Q., Li, D., He, Y., Li, Y., Yang, Z., Zhao, X., Liu, Y., Wang, Y., Sun, J., & Wang, F. (2019). Discrepant gut microbiota markers for the classification of obesity-related metabolic abnormalities. Scientific Reports, 9(1), 1–10. https://doi.org/10.1038/s41598-019-49462-w
- Zhang, Y., Liu, Y., Li, J., Xing, T., Jiang, Y., Zhang, L., & Gao, F. (2020). Dietary corn-resistant starch suppresses broiler abdominal fat deposition associated with the reduced cecal Firmicutes. Poultry Science, 99(11), 5827–5837. https://doi.org/10.1016/j.psj.2020.07.042