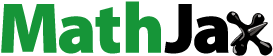
ABSTRACT
β-lactoglobulin and its thermolysin-derived hydrolysates were compared regarding the potential for microencapsulating of the flavonoids from yellow onion skins. Antioxidant activity of powders was of 500.28 ± 2.89 mMol Trolox/g dry weight (DW) for hydrolysates (P1) and 480.11 ± 4.45 mMol Trolox/g DW for whole protein (P2). Both powders showed antidiabetic activity against α-glucosidase, with inhibitory effect of 44.79 ± 0.58% for P1 and 32.76 ± 2.08% for P2. The bioinformatics analysis indicated that the Ala32-Ser37, Val59-Ile72, Leu111-Tyr118 and Val139-Ala148 amino acid sequences are particularly important for exerting potential antidiabetic activity. The finest powder was P2, with small 1–2 µm microspherosomes, whereas the peptides caused the agglutination of the flavonoids, leading to coacervates larger than 20 μm. Such an integrative approach might be useful in terms of recovering health-promoting bioactives from underutilized sources, such as onion skins and whey, contributing to the management and valorization of by-products, simultaneous with the development of innovative ingredients for functional applications.
Resumen
Este estudio se propuso comparar la β-lactoglobulina y sus hidrolizados derivados de la termolisina con respecto al potencial de microencapsulación de los flavonoides de la piel de cebolla amarilla. La actividad antioxidante de los polvos fue de 500.28 ± 2.89 mMol Trolox/g de peso seco (DW) para los hidrolizados (P1) y de 480.11 ± 4.45 mMol Trolox/g de DW para la proteína entera (P2). Así, se constató que ambos polvos mostraron actividad antidiabética contra la α-glucosidasa, con un efecto inhibitorio de 44.79 ± 0.58% para P1 y 32.76 ± 2.08% para P2. Además, el análisis bioinformático indicó que las secuencias de aminoácidos Ala32-Ser37, Val59-Ile72, Leu111-Tyr118 y Val139-Ala148 son particularmente importantes para ejercer una posible actividad antidiabética. El polvo más fino fue P2, con pequeños microesferosomas de 1–2 µm; los péptidos provocaron la aglutinación de flavonoides, dando lugar a coacervados mayores de 20 μm. Este enfoque integrador podría ser útil para recuperar bioactivos que promueven la salud a partir de fuentes subutilizadas, como la piel de cebolla y el suero de leche, contribuyendo a la gestión y la valorización de los subproductos, simultáneamente con el desarrollo de ingredientes innovadores para aplicaciones funcionales.
1. Introduction
In recent years, the development of a healthier lifestyle, based on the consumption of value-added products, and health-boosting foods and drinks, with the potential to lower the risk of specific illness, has become increasingly popular (Mark et al., Citation2019). Food-derived bioactive compounds have gained attention due to their potential health benefits in reducing the risks of many diseases, such as diabetes, cancer, cardiovascular diseases, diabetes, neurodegenerative disorders, and obesity caused by oxidative stress (Chai et al., Citation2020; Oh, Citation2016). These food-sourced bioactive compounds include phytochemical, such as phenolics (Velderrain-Rodriguez et al., Citation2014), fatty acids (Kruk et al., Citation2016), vitamins (Katouzian & Jafari, Citation2016), proteins and related bioactive peptides (Dhaval et al., Citation2016) and bioactive polysaccharides (Jiang et al., Citation2016).
Phenolic compounds are a diverse group of plant secondary metabolites found in vegetables, fruits, and cereals, widely categorized into the following groups: phenolic acids, flavonoids, stilbenes, tannins, lignans, coumarins, curcuminoids, and quinones (Basli et al., Citation2017). Numerous studies have shown that phenol-enriched diets can help in preventing a wide-range of diseases, such as cancers, diabetes, heart disease, neurodegenerative and cardiovascular diseases, and aging (Shahidi & Ambigaipalan, Citation2015). Mark et al. (Citation2019) highlighted the fact that food consumption is the main way for humans to uptake phenolic compounds, considering that fruits, vegetables, and beverages are all rich in phenolics. However, Piechowiak et al. (Citation2020) suggested that antioxidant deficiency in a daily diet is a cause for organism ageing and occurrence of numerous diseases of affluence being a consequence of oxidative stress. Therefore, new sources of antioxidants for human consumption must be identified and used in development of smart delivery systems for bioactive compounds in foods.
Onion (Allium cepa L.) is one of the major vegetable crops grown in Europe. Onion processing at industrial scale causes large amount of wastes estimated to 500, 000 tonnes annually (Choi et al., Citation2015) being an important source of polyphenols. As a function of variety, climate, soil and agrotechnical conditions of growing, levels varying from 2 to 10 g/kg of polyphenols of the skin weight were determined (Piechowiak et al., Citation2020). Therefore, there is an emerging need to manage onion wastes by recovering important bioactive compounds from plant processing by-products. The skin or outer scales of onion bulbs contain high amount of phenolics and flavonoids, mostly in their glycosides form of quercetin, kaempferol, and myricetin derivatives. However, due to their molecular structure, phenolic compounds are susceptible to degradation due to external factors like light, heat, or pH changes (Munin & Edwards-Lévy, Citation2011). To overcome the poor processing stability and bioavailability of polyphenols, various micro- and nanocapsules delivery systems have been developed, therefore improving the taste, dissolving hydrophobic compounds into the aqueous phase by incorporating them into hydrophilic microcapsules, providing protection from hazardous environmental conditions, and improving absorption through the intestinal mucus and epithelium (Livney, Citation2010). Therefore, encapsulation is a technique aimed to improve their processing stability, bioavailability after administration, extend their shelf-life, and make them easy to handle (Sansone et al., Citation2011). Encapsulation is a technique used to entrap active ingredients within wall materials to form microcapsules (Del Mar Contreras et al., Citation2011). Del Mar Contreras et al. (Citation2011) suggested that the selection of the coating material is a crucial step in development, since it determines the features and properties of the resulting microcapsules. For example, encapsulation has been successfully applied in the food sector to maintain good quality and characteristics, such as aqueous solubility, texture, taste, coloring strength, and stability, during processing and storage (Chai et al., Citation2020).
Whey is an abundant and low-cost by-product of the dairy industry with high nutritional, functional and biological value (Ge et al., Citation2019). β-lactoglobulin (β-LG) has been widely used as encapsulating material in functional foods due to its high nutritional value, biodegradability, biocompatibility, and pepsin-resistant ability (Akdeniz et al., Citation2018). In addition, due to its high surface activity, this protein acts as film-forming and emulsifying agent (Norkaew et al., Citation2019). One of the major concern regarding β-LG is its acid-resistant property in gastric juice, thus contributing to the protection of bioactives loaded with β-LG particles, therefore improving their bioavailability (Dai et al., Citation2020). Additionally, β-LG may act as a precursor for several functional peptides, to be used in different food applications as texturizing and stabilizing agents (Leeb et al., Citation2020). Whey proteins peptides can lower blood pressure through different mechanisms, including the well-known inhibition of angiotensin-converting enzyme (ACE), as well as rennin and pro-rennin enzymes, blocking calcium channels and AT1 receptors and stimulation of nitric oxide production, among others (Ozorio et al., Citation2019). However, when considering whey proteins or β-lactoglobulin as a substrate for biologically active peptides, several factors should be considered, such as: economic feasibility and the enzyme specificity to deliver targeted function. For example, it has been reported that the antioxidant effect is inherent to the protease specificity and to the hydrolysis conditions (Hernández-Ledesma et al., Citation2005). Additionally, technologies that allow the transformation of whey into other products are increasingly focused, since such processes potentially act both as valorization strategies and effluent management practices (Folmer Corrêa et al., Citation2014). Therefore, considering the above-mentioned issues and the value-added potential of whey protein hydrolysates for human health, the development of the enzyme-based technology should be considered as an interesting approach contributing to the management of these polluting by-products, and also for the discovery of new bioactive peptides.
Numerous studies are focused on whey protein peptides with different biological functions, but, to our current knowledge, there are no studies using β-LG peptides as encapsulation material. Therefore, this study aimed to test the possibility of using β-LG and its hydrolysates as coating materials for flavonoids extracted from yellow onions skins. The extraction of flavonoids was performed by a well-known, frequently used ethanolic combined with ultrasound-assisted extraction methods, whereas the extract was characterized in terms of individual and total flavonoids, total polyphenols content, and antioxidant activity. The β-LG hydrolysates were obtained by enzymatic hydrolysis using thermolysin. The microencapsulation experiments involved the use of hydrolysates with Mw lower than 10 kDa (P1) and β-LG solutions (P2), at the same protein concentration. The resulting freeze-dried powders were characterized in terms of encapsulation efficiency, phytochemical content, antioxidant activity and antidiabetic potential. Structural and morphological particularities of the samples were analyzed by using confocal laser electron microscopy. The results obtained in this study could bring certain benefits in terms of exploiting the bioactive potential of both phytochemicals and bioactive peptides, for developing health-benefits promoting formulas, with improved functional properties.
2. Materials and methods
2.1. Chemicals
Bovine β-LG (99% purity), quercetin, 2,2-diphenyl-1-picrylhydrazyl (DPPH), 6-hydroxy-2,5,7,8-tetramethylchromane-2-carboxylic acid (Trolox), ethanol, sodium hydroxide, Folin-Ciocalteu reagent, gallic acid and thermolysin from Geobacillus stearothermophilus (type X, lyophilized powder, 30–175 units/mg protein) were obtained from Sigma Aldrich Steinheim (Darmstadt, Germany).
2.2. Solvent ultrasound-assisted extraction of flavonoids from onion skins
Yellow onions were purchased from a local market (Galati, Romania) in June, 2019. The outer layers of onions were collected, cleaned with distilled water, dried at 40°C for 2 h, milled and used for further analysis. The phenolic extract was obtained by mixing 50 g of the sample with 450 ml of 70% ethanol solution and glacial acetic acid (ratio 9:1, v/v). The extraction was performed using a sonication water bath for at 40°C for 30 min, followed by centrifugation at 5000x g for 10 minutes at 4°C. The supernatant was collected, and the extraction was repeated three times. After extraction, the collected supernatants were pooled together and concentrated under reduced pressure at 40°C. The obtained extract was dissolved in ultrapure water, characterized and used for microencapsulation experiments.
2.3. Phytochemical and antioxidant profiling of the extract and of microencapsulated powders
The extract was characterized in terms of flavonoids, polyphenolic contents and antioxidant activity by using the aluminum chloride, Folin-Ciocâlteu and DPPH methods, respectively, as described by Milea et al. (Citation2019). In order to characterise the phenolic compounds of powders, the protocols described by Oancea et al. (Citation2018) were employed for assaying the total flavonoids, total polyphenol contents and antioxidant activity. Therefore, the total polyphenolic content was measured using the Folin-Ciocâlteu method, involving the use of the 0.2 mL of the extract or powder extract, 15 mL of distillate water and 1 mL of Folin-Ciocâlteu reagent. The mixture was mixed thoroughly before incubating under dark at room temperature for 5 min. Finally, 3.0 mL of saturated Na2CO3 was added and the solutions were incubated 2 h under dark at room temperature before measuring absorbance at 765 nm using spectrophotometer. Measurement was done in triplicate. Total phenolics content was reported as gallic acid (GAE) equivalents/g dry weight (DW) based on gallic acid standard curve.
The total flavonoid content was determined by colorimetrically method, involving the mixing of the samples (0.25 mL) with deionized water (2 mL) and 5% NaNO2 (0.15 mL) and the reaction was allowed to occur for 5 min. Then, 10% AlCl3.6H2O (0.15 mL) was added, and the mixture was allowed to stand for another 5 min. The mixture was added with 1 M NaOH (1 mL), mixed and kept in the dark for 15 min. The absorbance was determined at 510 nm. For the blank, the extracting solvent was used instead of the extract. A standard curve using quercetin was used to estimate the total flavonoids, which is expressed as mg of quercetin equivalents (QE) per g of DW.
The DPPH radical scavenging method was used to estimate the antioxidant activity, involving the use of 0.1 mMol solution of methanolic DPPH solution. The initial absorbance of the DPPH in ethanol was measured at 515 nm and did not change throughout the period of assay. Aliquots (0.1 ml) of each sample were added to 2.9 ml of DPPH solution. Discolorations were measured at 515 nm after incubation for 60 min at 25°C in the dark. Measurements were performed at least in triplicate and expressed as mMol Trolox/g DW using a calibration curve.
2.4. High-performance liquid chromatography of the flavonoids profile in the extract
The chromatographic analysis of the extract was undertaken on a Thermo Finnigan Surveyor HPLC-DAD equipment, controlled by the Xcalibur software (Finnigan Surveyor LC, Thermo Scientific, USA). The column used for the identification of the targeted compounds was a C18 Synergi 4 u Fusion-RP 80A column, 150 mm x 4.6 mm, particle size of 4 μm (Phenomenex, California, USA). The bioactive compounds (quercetin and its derivatives) were separated using an elution gradient composed of two mobile phases: 100% methanol (A) and 10% formic acid (B), with an elution gradient as follows: 0–20 min, 9–35% (A); 20–30 min, 35% (A); 30–40 min, 35–50% (A); 40–55 min, 50–9% (A). The flow rate was 1 mL/min, with an injection volume of 10 μL, with detection at wavelength of 360 nm. The compounds were identified and quantified in regard to their retention time and by comparing with the available standards and with data reported by other studies.
2.5. Thermolysin assisted hydrolysis of β-lactoglobulin
In order to obtain peptides, β-LG solution of 5 mg/mL (w/v) was prepared in 10 mM Tris-HCl buffer, and the pH was adjusted to 8.0 using 1 M NaOH. The thermolysin-assisted hydrolysis of β-LG was carried out for 2 h at 70°C, using an enzyme:substrate ratio of 1:50, as previously described by Milea et al. (Citation2019). The enzyme was inactivated by heating the solutions at 95°C for 5 min. Prior to microencapsulation experiments, in order to obtain the β-LG peptides, the hydrolysates were subjected to centrifugation at 9000xg for 25 min at 4°C, using centrifugal filter devices, Amicon Ultra-15, 10 kDa (Sigma Aldrich Steinheim, Darmstadt, Germany). The centrifugation step allowed removing the enzyme used for β-LG hydrolysis. The hydrolysis degree was determined as described by Milea et al. (Citation2019), by using the o-phthaldialdehyde method.
Further in silico protein digestion was carried out to be able to identify the resulting peptides. The PeptideCutter tool on ExPASy server (https://www.expasy.org/) and the amino acid sequence available in the Uniprot database entry code P02754 were considered to identify the thermolysin cleavage sites and to further simulate the complete hydrolysis of β-LG (), in agreement with the experimental tests. The signal peptide (position 1–16) from P02754 was excluded when generating the peptide mixture.
Figure 1. Three-dimension model of β-lactoglobulin. (a) The location of the protein regions susceptible to hydrolysis with thermolysin, as predicted by PeptideCutter tool, is highlighted in blue. (b) The amino acid sequences with potential antidiabetic potential are highlighted in blue (Ala32-Serr37), red (Val59-Ile72), orange (Leu111-Tyr118) and green (Val139-Ala148).
Figura 1. Modelo tridimensional de β-lactoglobulina. (a) La ubicación de las regiones de la proteína susceptibles de hidrólisis con termolisina, como predice la herramienta PeptideCutter, está resaltada en azul. (b) Las secuencias de aminoácidos con potencial antidiabético se destacan en azul (Ala32-Ser37), rojo (Val59-Ile72), naranja (Leu111-Tyr118) y verde (Val139-Ala148)
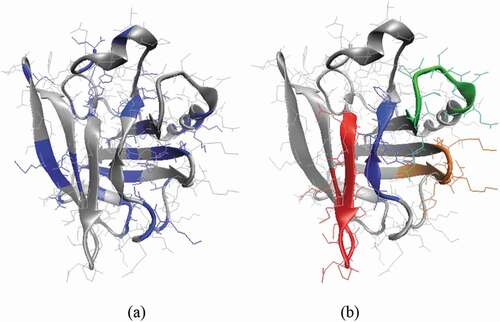
2.6. Microencapsulation of onion skins flavonoids extract
Two sets of microencapsulation experiments were performed, leading to two freeze-dried powders. The β-LG hydrolysates (approximatively 20 mL) were mixed with 0.5 g of the previously obtained extract and further subjected to ultrasonication for 75 min at 40.0 ± 1.0°C. Further, the sample were frozen at −70°C, and freeze-dried (CHRIST Alpha 1–4 LD plus, Germany) at −42°C under a pressure of 0.10 mBar for 48 h. The resulting powder, containing peptide with a molecular weight less than 10 kDa as encapsulating material was coded P1. The protocol was repeated using native β-LG as coating material, to obtain the P2 sample. Finally, the powders (P1 and P2) were collected and packed in metalized bags and stored under refrigeration until analysis.
2.7. Microencapsulation efficiency of flavonoids
The microencapsulation efficiency was determined by assessing the surface flavonoids content (SFC) and total flavonoid content (TFC) of the powders, expressed as mg of quercetin equivalents per g dry weight of powder (mg QE/g DW). In order to quantify the content of the flavonoids located at the surface of the microparticle (SFC), 50 mg of powder was mixed with 5 mL of ethanol and methanol (1:1). These dispersions were stirred at room temperature for 1 min and then centrifuged at 4000x g and 4°C for 10 min. For TFC, 50 mg of powder were accurately weighed and dispersed in 5 mL of a mixture of ethanol, acetic acid, and water (50:8:42, v/v/v). The resulting dispersion was vortexed (1 min), followed by ultrasonication for 30 min at 40°C ± 1.0°C, to break the microcapsules. The supernatant was centrifuged at 14,000x g for 10 min and then filtered. The microencapsulation efficiency (EE, %) was calculated using Equation (1):
EE (%) = x 100 (1)
2.8. Structural characteristics of microencapsulated powders by confocal laser scanning microscopy
The confocal laser scanning microscopy (CLSM) analysis was employed to assess the structural appearance of the microencapsulated powders. The CLSM method was rather similar to the one used by Milea et al. (Citation2019) with slight modifications. The images were captured with a Zeiss confocal laser scanning system (LSM710) equipped with several types of lasers such as a diode laser (405 nm), Ar-laser (458, 488, 514 nm), DPSS laser (diode-pumped solid state – 561 nm) and HeNe-laser (633 nm). The microcapsule powders were observed with a 20× apochromatic objective, zoom 1. The obtained 3D images were rendered and processed by ZEN 2012 SP1 software (Black Edition).
2.9. Antidiabetic properties of the powders
The microcapsule powders were tested as antidiabetic agents for the inhibition of α-amylase and α-glucosidase.
2.9.1. α-Glucosidase inhibition
For the α-glucosidase inhibition experiments, 50 μL of powder solutions (10 mg/mL in a mixture of methanol: acetic acid: distillate water in a ratio of 25:4:21) were added to 50 μL of 1 mg/mL α-glucosidase solution (0.1 M phosphate sodium buffer – PBS, pH 6.9) and incubated for 10 min at 25°C. A 50 μL aliquots of 25 mM p-nitrophenyl-α-D-glucopyranoside solution in 0.1 M PBS (pH 6.9) was added and incubated at 37°C for 15 min. Then, 800 μL of 0.2 M sodium carbonate was added, followed by reading the absorbance at 405 nm. Enzyme inhibition was calculated using the following equation:
Inhibition (%) = x 100 (2)
where A0 is the absorbance of the control (blank, without powder addition) and As is the absorbance in presence of the powder solution.
2.9.2. α-Amylase inhibition
For the α-amylase inhibitory effect, 500 μL of powder solutions (10 mg/mL in a mixture of methanol: acetic acid: distillate water in a ratio of 25:4:21) were added to 500 μL of 13 U/mL α-amylase and incubated in test tubes at 25°C for 10 min. Further, 500 μL of 1% soluble starch solution was added to each tube and incubated for another 15 min. Finally, one mL of dinitro- salicylic acid reagent was added, and the tubes were placed in 100°C water bath for 5 min. The mixture was diluted with 10 mL of distilled water and absorbance was read at 540 nm. The inhibition was estimated using Equation (2).
2.10. Statistical analysis of data
Triplicate measurements were performed, and the mean values ± standard deviations were calculated. In order to identify significant differences, experimental data were subjected to one-way analysis of variance (ANOVA) after running the normality and homoscedasticity tests. The Tukey method with a 95% confidence interval was employed for post-hoc analysis; p < .05 was considered to be statistically significant. The statistical analysis was carried out using Minitab 18 software.
3. Results
3.1. Thermolysin assisted hydrolysis of β-lactoglobulin
The hydrolysis degree accounted for 31.94 ± 0.49%. The major whey protein β-LG is well known to act as a precursor for several functional peptides (Dai et al., Citation2020). For example, in a tryptic digestion, the presence of five bioactive peptides was identified in the total hydrolysate by Chatterton et al. (Citation2006). Hydrolysis of bovine β-LG with thermolysin was carried out at 37°C for 5, 10, 20, and 30 min, by Hernandez-Ledesma et al. (Citation2006) who identified a complex peptidic profile with 26 peptide peaks in a hydrolysates obtained after only 5 min of hydrolysis, at an enzyme:substrate ratio of 1:20. These authors were able to identify a total of 25 peptides. The thermolysin is able to cleave the adjacent peptide bonds to hydrophobic and/or aromatic amino acids, such as Ile, Leu, Phe, Tyr, and Val (). Further, these authors suggested that the majority of the peptides were large, hydrophobic, with the most intensive signal for the sequences SAPLRVY f(36–42), VAGTWYS f(15–21), and LIVTQTMKG f(1–9), and lower for three sequences ELK, VEELK, and KPTPEG f(47–52), thus including the most buried parts of bovine β-LG molecules. This is possible due to the unfolding of the buried zones, including hydrophobic amino acids, thus becoming accessible for thermolysin at the alkaline pH and high temperature used in hydrolysis experiments (70°C).
However, these authors identified the peptide LQKW, corresponding to the fragment f(58–61), that had been previously described as a potent angiotensin-converting enzyme-inhibitor. When simulating the hydrolysis degree of β-LG gathered with thermolysin in the present experimental study, a mixture of peptides consisting of 3 to 14 amino acid residues was obtained, in addition to the dipeptides and free amino acids (), which is in good agreement with the results reported by Condurache et al. (Citation2019).
Table 1. Peptides released through complete thermolysin assisted hydrolysis of β-lactoglobulin (β-LG). The cleavage sites were predicted using dedicated ExPASy tools available on-line. The peptides with antidiabetic potential were identified by checking the sequences similarity against the BioDADPep database, and are provided in bold.
Tabla 1. Péptidos liberados mediante hidrólisis completa de β-lactoglobulina (β-LG) asistida por termolisina. Los sitios de clivaje se predijeron utilizando herramientas específicas de ExPASy disponibles en línea. Los péptidos con potencial antidiabético se identificaron comprobando la similitud de las secuencias con la base de datos BioDADPep y se presentan en negrita
Because of the differences regarding the hydrolysis conditions, such as hydrolysis temperature, time and enzyme to substrate ratio, part of the sequences reported by Hernandez-Ledesma et al. (Citation2006) were not identified when carrying out the in silico hydrolysis of the protein in our study. For instance, the sequences SAPLRVY f(36–42) and LIVTQTMKG f(1–9) got split into dipeptides and tripeptides, respectively. On the other hand, the KPTPEG f(47–52) sequence was found to belong to the longest peptide (Val59-Ile72) yielded under the experimental conditions considered in the present study Furthermore, the LQKW tetrapeptide described by Hernandez-Ledesma et al. (Citation2006) appears to be part of the Leu74-Cys82 sequence with higher molecular weight of 1106.22 Da. Regarding the biological activity, as indicated by Condurache et al. (Citation2019), the ASDIS (Ala42-Ser46) and LDAQSAP (Leu48-Pro54) sequences are comprised in a longer peptide released through trypsin digestion of β-LG, namely AASDISLLDAQSAPLR which proved to exert efficient bactericidal effects against the Gram-positive bacteria (Pellegrini et al., Citation2001).
Whey protein concentrate enriched in β-LG was hydrolyzed by Del Mar Contreras et al. (Citation2011), using thermolysin to produce hydrolyzates with antioxidant activity. These authors obtained the highest radical scavenging activity (2.321 mMol Trolox/mg protein) for the 3 kDa-permeate obtained after 24 h incubation with thermolysin at an enzyme:substrate ratio of 1:10 (w/w), suggesting that low molecular weight peptides are the main responsible for antioxidant activity. However, in our study, β-LG showed no antioxidant activity, whereas its <10 kDa hydrolysates had a radical scavenging activity of 1.26 ± 0.16 mMol Trolox/mg protein.
3.2. Extract characterization, microencapsulation and phytochemical characterization of the powders
For safety, environmental and economical sustainability, green, or eco-friendly extraction processes are being developed using various methods such as microwave-assisted extraction, supercritical fluid extraction, accelerated solvent extraction, enzyme-assisted extraction and ultrasound-assisted extraction (USAE) (Bamba et al., Citation2018). Therefore, in this study, a preliminary optimization extraction step was performed, involving the comparative use of two techniques, such as organic solvent solid-liquid extraction and solvent USAE extraction, from the perspectives of optimal recovery of flavonoids from yellow onion skins. The preliminary results (data not shown) allowed the selection of USAE extract for further experiments, given the phytochemical profile, energy saving, and the use of moderate temperatures. Therefore, the yellow onion skins extract obtained by USAE showed a total flavonoid content of 228.68 ± 3.09 mg QE/g DW, a total polyphenolic compounds content of 96.06.27 ± 2.70 mg gallic acid equivalents (GAE)/g DW, yielding an antioxidant activity of 495.89 ± 2.42 mM Trolox equivalents/g DW. Singh et al. (Citation2016) used 70% ethanol for ultrasound-assisted extraction of bioactive compounds from onions, and reported similar values for flavonoids of 212.3 ± 14.6 mg QE/g DW and a higher amount of phenolic compounds (418.0 ± 34.4 mg GAE/g DW).
In order to determine the chromatographic profile of the yellow onion skins’ extract (), a 55 minutes long method was undertaken. The profile allowed to identify 5 compounds, namely quercetin 7,4-diglucoside, quercetin 3,4-diglucoside, quercetin 4-glucoside, quercetin, and kaempferol. The major compound found was quercetin 3,4-diglucoside with a content of 19.29%, followed by quercetin that revealed a content of with a concentration of 7.30% of the total extracted flavonoid compounds. The first identified compound was quercetin 7,4-diglucoside, which displayed a content of 2.79% of the total extracted flavonoid compounds whereas kaempferol displayed a content of less than 1%. The quantification of the compounds was achieved based on their standard calibration curves. Quercetin 3,4-diglucoside displayed as the major compound a concentration of 1.52 mg/g DW, whereas quercetin showed a concentration of 0.65 mg/g DW.
Figure 2. HPLC analysis of the onion skins’ extract: Peak (1) – quercetin 7,4-diglucoside; Peak (2) - quercetin 3,4-diglucoside; Peak (3) - quercetin 4-glucoside; Peak (4) -quercetin; Peak (5) - kaempferol.
Figura 2. Análisis HPLC del extracto de piel de cebolla: Pico (1) – quercetina 7,4-diglucósido; Pico (2) – quercetina 3,4-diglucósido; Pico (3) – quercetina 4-glucósido; Pico (4) -quercetina; Pico (5) – kaempferol
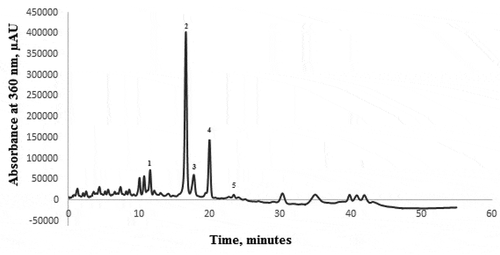
Our results were rather similar to those reported byAlbishi et al. (Citation2013) who studied the biologically active compounds, namely quercetin, and its derivates extraction, from 4 varieties of onion, yellow, white, red, and pearl. In their analysis, quercetin, quercetin-3,4-O-diglucoside and kaempferol were reported to exist in their free form, but the highest concentration was recorded by quercetin-3,4-O-diglucoside.
The powders obtained by freeze-drying showed different encapsulation efficiency, as a function of molecular weight. Therefore, the encapsulation efficiency values were 43.77 ± 0.78% for P1 and 61.07 ± 1.07% for P2. The highest ability to entrap flavonoids from onion skins extract was exhibited by β-LG, and decreased as the size of polypeptide chains decreased. In our previous study, whey proteins hydrolysates, pectin and maltodextrin were used to encapsulate flavonoids extracted from yellow onions skins by freeze-drying (Milea et al., Citation2019). Encapsulation efficiency depended on the biopolymers composition, ranging from 55.95 ± 0.44% to 66.46 ± 0.18%, as a function of maltodextrin:pectin ratio. To the best of our knowledge, no other studies are available exploiting the potential of peptides from different sources as biopolymeric wall materials used in encapsulation.
The phytochemical profile of extract and of the two variants are comparatively shown in . Although the encapsulation efficiency was significantly lower, it can be seen from that P1 variant showed a higher content in phytochemicals and antioxidant activity when compared with P2. Therefore, P1 showed a higher ability to entrap flavonoids and polyphenols.
Table 2. Phytochemical profile of the extract and powder samples obtained by microencapsulating flavonoids in different wall materials: peptides obtained through β-lactoglobulin hydrolysis by thermolysin (P1) and β-lactoglobulin (P2).
Tabla 2. Perfil fitoquímico de muestras de extracto y polvo obtenidas por microencapsulación de flavonoides en diferentes materiales de pared: péptidos obtenidos mediante hidrólisis de β-lactoglobulina asistida por termolisina (P1) y β-lactoglobulina (P2)
The presence of peptides lead to an increased antioxidant activity in P1 (). Milea et al. (Citation2019) encapsulated polyphenolic compounds from yellow onion skins using as coating materials maltodextrin, pectin and whey protein hydrolysates in different ratio. They reported lower levels of flavonoids, polyphenols and the antioxidant activity compared to those obtained in the present study; flavonoids varied from 98.12 ± 0.55 to 103.75 ± 0.57 mg QE/g DW, polyphenols from to 53.53 ± 1.71 to 69.26 ± 1.03 mg GAE/g DW and antioxidant activity ranging from 280.61 ± 3.08 to 337.57 ± 0.89 mM Trolox/g DW. The difference in concentration of polyphenolics between the two studies could be explained by different core:wall material ratio and the use of different adjuvants, such as pectin and maltodextrin. Akdeniz et al. (Citation2018) encapsulated phenolics from onion skins in combinations of maltodextrin, gum Arabic, casein, or whey protein concentrate and reported values for total phenolic compounds values from 161.36 ± 1.38 to 259.73 ± 0.93 mg GAE/g DW. Horincar et al. (Citation2019) used whey protein-chitosan and whey protein-maltodextrin-pectin as wall materials in different ratios and reported values for total flavonoid content of 5.84 ± 0.23 mg QE/g DW and 104.97 ± 5.02 mg QE/g DW, respectively, with antioxidant activity of 175.93 ± 1.50 mM Trolox/g DW and 269.20 ± 3.59 mM Trolox/g DW.
3.3. Structure and morphology of the powders
The confocal microscopy analysis was used to analyze the microstructural design of the powders obtained through the microencapsulation of the valuable bioactives from the yellow onion skins using β-LG and its hydrolysates as coating materials. The confocal images for the powder are shown in .
Figure 3. Confocal laser scanning microscopy images obtained with the ZEN 2012 SP1 software (Black Edition) on the powder samples obtained by encapsulating flavonoids in different wall materials: peptides obtained through β-lactoglobulin thermolysin assisted hydrolysis (a) and β-lactoglobulin (b).
Figura 3. Imágenes de microscopía de escaneo láser confocal obtenidas con el software ZEN 2012 SP1 (Black Edition) sobre las muestras de polvo obtenidas por encapsulación de flavonoides en diferentes materiales de pared: péptidos obtenidos por hidrólisis de β-lactoglobulina asistida por termolisina (a) y β-lactoglobulina (b)
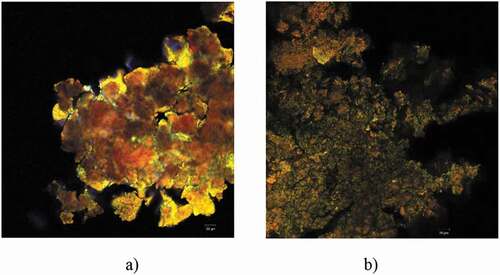
The finest powder was P2, which presented uniform microscopic appearance, having small microspherosomes with diameters between 1 and 2 µm, with emission predominantly in the green domain (550 nm). The tendency of the microspherosomes to aggregate into clusters can be observed in . This fact is well correlated to the obtained results regarding the microencapsulation efficiency. Nonetheless, the use of the β-LG hydrolysates obviously changed the structure of the microparticles. The peptides with molecular weight smaller than 10 kDa (shown in yellow) caused the agglutination of the polyphenolic compounds and flavonoids, leading to coacervates larger than 20 μm, having the emission spectrum conveyed to the red domain (630–650 nm) (). The microscopic structure of the powders obtained through these microencapsulation variants was more uniform to the powders previously obtained by Horincar et al. (Citation2019) or by Milea et al. (Citation2019). Depending on hydrolysis conditions, the β-LG hydrolysates usually contain a different range of peptides with different lengths, charges, hydrophobicity, molecular weight, hygroscopicity, and surface properties, properties that make it challenging to achieve a high and uniform encapsulation efficiency (Mohan et al., Citation2015).
3.4. Antidiabetic potential
It has been reported that whey protein peptides are powerful acute stimulants of insulin secretion and can enhance the insulin response when supplied in a mixed meal (Nilsson et al., Citation2004; Uchida et al., Citation2011). Frid et al. (Citation2011) reported that acute administration of whey protein–supplemented meals to individuals with type 2 diabetes was associated with significant increments in postprandial insulin responses and higher levels of glucose-dependent insulinotropic polypeptide, but not postprandial glucagon-like peptide-1. However, Tulipano et al. (Citation2011) suggested that Ile-Pro-Ala, an enzyme dipeptidyl peptidase-4 inhibitor (DDP-4), was isolated from the hydrolysates of β-LG. In our study, inhibition efficiency decreased in the following order P1 > P2, and the powders were generally more active against α-glucosidase that on α-amylase, at the concentration tested (10 mg/mL). The highest effect against α-glucosidase of 44.79 ± 0.58% was observed in case of P1, followed by P2 with 32.76 ± 2.08% (). The P1 was as well the more effective against α-amylase, with an inhibitory activity of 5.28 ± 0.31%, when compared with the inhibitory effect of P2 of 2.24 ± 0.79%.
Table 3. Effect of the microencapsulated powders containing flavonoids in different wall materials: peptides obtained through β-lactoglobulin hydrolysis by thermolysin (P1) and β-lactoglobulin (P2) on the enzymes related to carbohydrate metabolism. Results are reported as inhibitory effect (%).
Tabla 3. Efecto de los polvos microencapsulados que contienen flavonoides en diferentes materiales de pared: péptidos obtenidos a través de hidrólisis de β-lactoglobulina asistida por termolisina (P1) y β-lactoglobulina (P2) en las enzimas relacionadas con el metabolismo de los carbohidratos. Los resultados se comunican como efecto inhibitorio (%)
In order to identify the peptides potentially responsible for this results, the amino acid sequences generated through the in silico protein digestion were checked against the content of BioDADPep database, which includes therapeutic peptides with antidiabetic potential (Roy & Teron, Citation2019). No complete overlap between the peptides released through thermolysin assisted hydrolysis of β-LG and the items of the BioDADPep repository was found. Anyway, important sequence similarities were found as follows: four peptides from BioDADPep, namely VAGTWY(Power et al., Citation2014), LKPTPEGDLEIL (Lacroix & Li-Chan, Citation2014), VLVLDTDYK and TPEVDDEALEK (Silveira et al., Citation2013), matched the amino acid sequences Ala32-Ser37 (AGTWYS), Val59-Ile72 (VEELKPTPEGDLEI), Leu111-Tyr118 (LDTDYKKY) and Val139-Ala148 (VRTPEVDDEA) located on outer regions of β-LG () and released by thermolysin. The antidiabetic potential of these peptides was assigned to the DPP-4 inhibitory activity (Lacroix & Li-Chan, Citation2014; Power et al., Citation2014; Silveira et al., Citation2013). Anyway, the in vivo digestion might result in a more advanced degradation of these oligopeptides. The PeptideCutter tool was employed to check if the peptides released through thermolysin assisted hydrolysis of β-LG are further susceptible to hydrolysis by the digestive enzymes. The only peptide with predicted antidiabetic potential which is not hydrolyzed by the digestive enzymes is Val139-Ala148. Three potential pepsin cleavage sites were found in the Val59-Ile72 sequence, whereas both chymotrypsin and pepsin can recognize and hydrolyze at least two peptide bonds of the active regions of Ala32-Ser37 and Leu111-Tyr118 sequences. Uchida et al. (Citation2011) showed that trypsin-treated β-lactoglobulin exerted a concentration-dependent inhibition of DPP-4, with an IC50 value of 210 μM. The most active peptide was Val-Ala-Gly-Thr-Trp-Tyr, corresponding to the f(15–19) sequence from β-lactoglobulin, which had an IC50 value of 174 μM.
Additionally, the use of phytochemicals, such as onion flavonoids, for treating diabetes is not just viewed as an alternative to pharmaceuticals, which transiently lower the blood glucose, preventing heart disease and high blood pressure, and also enhancing the antioxidant system, insulin action, and secretion (Vinayagam & Xu, Citation2015). Our results agree with previous reports on the higher inhibitory activity against α-glucosidase of natural compounds from plants, comparatively with α-amylase (Kwon et al., Citation2007). Moreover, and similar to what is generally reported in the literature, samples had a stronger inhibitory capacity towards microbial α-glucosidase than to α-glucosidase of mammalian origin (Shai et al., Citation2011).
4. Conclusions
The consumers increasingly requiring for “label friendly” products which are fabricated by natural and sustainable ingredients, requires innovation from the perspectives of developing health-promoting, natural alternatives for synthetic additives. In this study, β-lactoglobulin was used to produce bioactive peptides, further used to microencapsulate flavonoids extracted from yellow onion skins. The comparative analysis of the microcapsules obtained by freeze-drying using peptides with molecular weight lower than 10 kDa and β-lactoglobulin highlighted the effect of peptides on regulating the antioxidant and antidiabetic activities, whereas the peptides increased the particle size of the microcapsules. The β-lactoglobulin hydrolysis by thermolysin was simulated using the PeptideCutter tool, and four resulting peptides, namely Ala32-Ser37, Val59-Ile72, Leu111-Tyr118 and Val139-Ala148, were found to exert antidiabetic potential though dipeptidyl peptidase-4 inhibitory activity.
The obtained results may support the hypothesis that β-lactoglobulin and its peptides can be used as an innovative approach to encapsulate flavonoids to further develop nutraceuticals for synthetic additive substitutions, but additionally studies are necessary to critically design and characterize their properties so that it can be applied in food. Therefore, our further studies are focused on identification and quantification of β-lactoglobulin resulting in peptides through thermolysin hydrolysis.
Conflicts of Interest
The authors declare no conflict of interest.
Author contribution
Conceptualization, S.A.M, N.S. and I.A.; methodology, S.A.M, E.E., V.B., N.S. and I.A.; software, I.A.; validation, formal analysis, N.S.; investigation, N.S. and I.A.; resources, G.R.; writing—original draft preparation, N.S. and I.A.; writing—review and editing, N.S. and I.A.; funding acquisition, G.E.B. All authors have read and agreed to the published version of the manuscript.
Acknowledgements
This work was supported by the project “Excellence, performance and competitiveness in the Research, Development, and Innovation activities at “Dunarea de Jos” University of Galati,” acronym “EXPERT,” financed by the Romanian Ministry of Research and Innovation in the framework of Programme 1-Development of the national research and development system, Sub-programme 1.2-Institutional Performance-Projects for financing excellence in Research, Development, and Innovation, Contract no. 14PFE/17.10.2018. The authors are grateful for the technical support offered by the Grant POSCCE ID 1815, cod SMIS 48745 (www. moras.ugal.ro). The Integrated Center for Research, Expertise and Technological Transfer in Food Industry is acknowledged for providing technical support.
Additional information
Funding
References
- Akdeniz, N., Sumnu, G., & Sahin, S. (2018). Microencapsulation of phenolic compounds extracted from onion (Allium cepa) skin. Journal of Food Processing and Preservation, 42(7), e13648. https://doi.org/https://doi.org/10.1111/jfpp.13648
- Albishi, T., John, A. J., Al-Khalifa, S. A., & Shahidi, F. (2013). Antioxidative phenolic constituents of skins of onion varieties and their activities. Journal of Functonal Foods, 5(3), 1191–1203. https://doi.org/https://doi.org/10.1016/j.jff.2013.04.002
- Bamba, B. S. B., Shi, J., Tranchant, C. C., Xue, S. J., Forney, C. F., & Lim, L.-T. (2018). Influence of Extraction Conditions on Ultrasound-Assisted Recovery of Bioactive Phenolics from Blueberry Pomace and Their Antioxidant Activity. Molecules, 23(7), 1685. https://doi.org/https://doi.org/10.3390/molecules23071685
- Basli, A., Belkacem, N., & Amrani, I. (2017). Health benefits of phenolic compounds against cancers. In M. Soto-Hernandez, M. Palma-Tenango, & M. D. R. Garcia-Mateos (Eds.), Phenolic compounds – Biological activity (pp. Ch. 10). InTech.
- Chai, J., Jiang, P., Wang, P., Jiang, Y., Li, D., Bao, W., Liu, B., Liu, B., Zhao, L., Norde, W., Yuan, Q., Ren, F., & Li, Y. (2020). The intelligent delivery systems for bioactive compounds in foods: Physicochemical and physiological conditions, absorption mechanisms, obstacles and responsive strategies. Trends in Food Science & Technology, 78, 144–154. https://doi.org/https://doi.org/10.1016/j.tifs.2018.06.003
- Chatterton, D. E. W., Smithers, G., Roupas, P., & Brodkorb, A. (2006). Bioactivity of β-lactoglobulin and α-lactalbumin. Technological implications for processing. International Dairy Journal, 16(11), 1229–1240. https://doi.org/https://doi.org/10.1016/j.idairyj.2006.06.001
- Choi, I., Cho, E., Moon, J., & Bae, H. (2015). Onion skin waste as a valorization resource for the by-products quercetin and biosugar. Food Chemistry, 188, 537–542. https://doi.org/https://doi.org/10.1016/j.foodchem.2015.05.028
- Condurache, N. N., Aprodu, I., Crăciunescu, O., Tatia, R., Horincar, G., Barbu, V., Stănciuc, N., Râpeanu, G., Bahrim, G. E., Oancea, A., & Stănciuc, N. (2019). Probing the functionality of bioactives from eggplant peel extracts through extraction and microencapsulation in different polymers and whey protein hydrolysates. Food and Bioprocess Technology, 12(8), 1316–1329. https://doi.org/https://doi.org/10.1007/s11947-019-02302-1
- Dai, W., Ruan, C., Sun, Y., Gao, X., & Liang, J. (2020). Controlled release and antioxidant activity of chitosan and ß-lactoglobulin complex nanoparticles loaded with epigallocatechin gallate. Colloids and Surfaces: Biointerfaces, 188, 110802. https://doi.org/https://doi.org/10.1016/j.colsurfb.2020.110802
- Del Mar Contreras, M., Hernández-Ledesma, B., Amigo, L., Martín-Álvarez, P. J., & Recio, I. (2011). Production of antioxidant hydrolyzates from a whey protein concentrate with thermolysin: Optimization by response surface methodology. LWT - Food Science & Technology, 44(1), 9–15. https://doi.org/https://doi.org/10.1016/j.lwt.2010.06.017
- Dhaval, A., Yadav, N., & Purwar, S. (2016). Potential Applications of Food Derived Bioactive Peptides in Management of Health. International Journal of Peptide Research and Therapeutics, 22, 377–398. https://doi.org/https://doi.org/10.1007/s10989-016-9514-z
- Folmer Corrêa, A. P., Daroit, D. J., Fontoura, R., Meister Meira, S. M., Segalin, J., & Brandelli, A. (2014). Hydrolysates of sheep cheese whey as a source of bioactive peptides with antioxidant and angiotensin-converting enzyme inhibitory activities. Peptides, 61, 48–55. https://doi.org/https://doi.org/10.1016/j.peptides.2014.09.001
- Frid, A. H., Nilsson, M., Holst, J. J., & Björck, I. M. (2011). Effect of whey on blood glucose and insulin responses to composite breakfast and lunch meals in type 2 diabetic subjects. The American Journal of Clinical Nutrition, 82(1), 69–75. https://doi.org/https://doi.org/10.1093/ajcn.82.1.69
- Ge, J., Yue, P., Wang, S., Chi, J., Liang, J., Sun, Y., Yue, P., & Yue, P. (2019). Nanocomplexes composed of chitosan derivatives and ß-Lactoglobulin as a carrier for anthocyanins: Preparation, stability and bioavailability in vitro.. Food Research International, 116, 336–345. https://doi.org/https://doi.org/10.1016/j.foodres.2018.08.045
- Hernández-Ledesma, B., Dávalos, B., Bartolomé, B., & Amigo, L. (2005). Preparation of antioxidant enzymatic hydrolysates from α-lactalbumin and β-lactoglobulin. Identification of active peptides by HPLC-MS/MS. Journal of Agricultural and Food Chemistry, 53(3), 588–593. https://doi.org/https://doi.org/10.1021/jf048626m
- Hernandez-Ledesma, B., Ramos, M., Recio, I., & Amigo, L. (2006). Effect of β-lactoglobulin hydrolysis with thermolysin under denaturing temperatures on the release of bioactive peptides. Journal of Chromatography. A, 1116(1–2), 31–37. https://doi.org/https://doi.org/10.1016/j.chroma.2006.03.0061-2
- Horincar, G., Aprodu, I., Barbu, V., Râpeanu, G., Bahrim, G. E., & Stănciuc, N. (2019). Interactions of flavonoids from yellow onion skins with whey proteins: Mechanisms of binding and microencapsulation with different combinations of polymers. Spectrochimica Acta A, 215, 158–167. https://doi.org/https://doi.org/10.1016/j.saa.2019.02.100
- Jiang, S., Liu, C., Wang, X., Xiong, L., & Sun, Q. (2016). Physicochemical properties of starch nocomposite films enhanced by self-assembled potato starch nanoparticles. LWT-Food Science & Technology, 69, 251–257. https://doi.org/https://doi.org/10.1016/j.lwt.2016.01.053
- Katouzian, I., & Jafari, S. M. (2016). Nano-encapsulation as a promising approach for targeted delivery and controlled release of vitamins. Trends Food Science & Technology, 53, 34–48. https://doi.org/https://doi.org/10.1016/j.tifs.2016.05.002
- Kruk, J., Szymanska, R., Nowicka, B., & Dluzewska, J. (2016). Function of isoprenoid quinones and chromanols during oxidative stress in plants. New Biotechnology, 33(5), 636–643. https://doi.org/https://doi.org/10.1016/j.nbt.2016.02.010
- Kwon, O., Eck, P., Chen, S., Corpe, C. P., Lee, J. H., Kruhlak, M., & Levine, M. (2007). Inhibition of the intestinal glucose transporter GLUT2 by flavonoids. FASEB Journal, 21(2), 366–377. https://doi.org/https://doi.org/10.1096/fj.06-6620com
- Lacroix, I. M., & Li-Chan, E. C. (2014). Isolation and characterization of peptides with dipeptidyl peptidase-IV inhibitory activity from pepsin-treated bovine whey proteins. Peptides, 54, 39–48. https://doi.org/https://doi.org/10.1016/j.peptides.2014.01.002
- Leeb, E., Stefan, T., Letzel, T., Hinrichs, J., & Kulozik, U. (2020). Tryptic hydrolysis of βlactoglobulin: A generic approach to describe the hydrolysis kinetic and release of peptides. International Dairy Journal, 105, 104666. https://doi.org/https://doi.org/10.1016/j.idairyj.2020.104666
- Livney, Y. D. (2010). Milk proteins as vehicles for bioactives. Current Opinion Colloid & Interface Science, 15(1–2), 73–83. https://doi.org/https://doi.org/10.1016/j.cocis.2009.11.002
- Mark, R., Lyu, X., Lee, J. J. L., Parra-Saldivar, R., & Chen, Y. N. (2019). Sustainable production of natural phenolics for functional food applications. Journal of Functional Foods, 57, 233–254. https://doi.org/https://doi.org/10.1016/j.jff.2019.04.008
- Milea, A. S., Aprodu, I., Vasile, A. M., Barbu, V., Râpeanu, G., Bahrim, G. E., & Stănciuc, N. (2019). Widen the functionality of flavonoids from yellow onion skins through extraction and microencapsulation in whey proteins hydrolysates and different polymers. Journal of Food Engineering, 251, 29–35. https://doi.org/https://doi.org/10.1016/j.jfoodeng.2019.02.003
- Mohan, A., Rajendran, S. R. C. K., He, Q. S., Bazinet, L., & Udenigwe, C. C. (2015). Encapsulation of food protein hydrolysates and peptides: A review. RSC Advances Home, 5(97), 79270–79278. https://doi.org/https://doi.org/10.1039/C5RA13419F
- Munin, A., & Edwards-Lévy, F. (2011). Encapsulation of natural polyphenolic compounds; a review. Pharmacy, 3(4), 793–829. https://doi.org/https://doi.org/10.3390/pharmaceutics3040793
- Nilsson, M., Stenberg, M., Frid, A. H., Holst, J. J., & Björck, I. M. E. (2004). Glycemia and insulinemia in healthy subjects after lactose equivalent meals of milk and other food proteins: The role of plasma amino acids and incretins. The American Journal of Clinical Nutrition, 80(5), 1246–1253. https://doi.org/https://doi.org/10.1093/ajcn/80.5.1246
- Norkaew, O., Thitisuta, P., Mahatheeranont, S., Pawina, B., Sookwong, P., Yodpitak, S., & Lungkaphin, D. (2019). Effect of wall materials on some physico-chemical properties and release characteristics of encapsulated black rice anthocyanin microcapsules. Food Chemistry, 294, 493–502. https://doi.org/https://doi.org/10.1016/j.foodchem.2019.05.086
- Oancea, A. M., Hasan, M., Vasile, A. M., Barbu, V., Enachi, E., Bahrim, G., Răpeanu, G., Silvi, S., & Stănciuc, N. (2018). Functional evaluation of microencapsulated anthocyanins from sour cherries skins extract in whey proteins isolate. LWT – Food Science & Technology, 95, 129–134. https://doi.org/https://doi.org/10.1016/j.lwt.2018.04.083
- Oh, Y. S. (2016). Bioactive Compounds and Their Neuroprotective Effects in Diabetic Complications. Nutrients, 8(8), 472. https://doi.org/https://doi.org/10.3390/nu8080472
- Ozorio, L., Silva, L. P., Prates, M. V., Bloch, C., Takeiti, C. Y., Gomes, D. M., da Silva-santos,, Cabral, J. E., Deliza, R., Brígida, A. I. S., Furtado, A., Mellinger-Silva, C., & Cabral, L. M. C. (2019). L.M.C. Whey hydrolysate-based ingredient with dual functionality: From production to consumer’s evaluation. Food Research International, 122, 123–128. https://doi.org/https://doi.org/10.1016/j.foodres.2019.03.060
- Pellegrini, A., Dettling, C., Thomas, U., & Hunziker, P. (2001). Isolation and characterization of four bactericidal domains in the bovine β-lactoglobulin. Biochimica et biophysica acta, 1526(2), 131–140. https://doi.org/https://doi.org/10.1016/s0304-4165(01)00116-7
- Piechowiak, T., Grzelak-Błaszczyk, K., Bonikowski, R., & Balawejder, M. (2020). Optimization of extraction process of antioxidant compounds from yellow onion skin and their use in functional bread production. LWT - Food Science & Technology, 117, 108614. https://doi.org/https://doi.org/10.1016/j.lwt.2019.108614
- Power, O., Fernández, A., Norris, R., Riera, F. A., & Fitzgerald, R. J. (2014). Selective enrichment of bioactive properties during ultrafiltration of a tryptic digest of β-lactoglobulin. Journal of Functional Foods, 9, 38–47. https://doi.org/https://doi.org/10.1016/j.jff.2014.04.002
- Roy, S., & Teron, R. (2019). BioDADPep: A Bioinformatics database for antidiabetic peptides. Bioinformation, 15(11), 780. https://doi.org/https://doi.org/10.6026/97320630015780
- Sansone, F., Picerno, P., Mencherini, T., Villecco, F., D’Ursi, A. M., Aquino, R. P., & Lauro, M. R. (2011). Flavonoid microparticles by spray-drying: Influence of enhancers of the dissolution rate on properties and stability. Journal of Food Engineering, 103(2), 188–196. https://doi.org/https://doi.org/10.1016/j.jfoodeng.2010.10.015
- Shahidi, F., & Ambigaipalan, P. (2015). Phenolics and polyphenolics in foods, beverages and spices: Antioxidant activity and health effects – A review. Journal of Functional Foods, 18(Part B), 820–897. https://doi.org/https://doi.org/10.1016/j.jff.2015.06.018
- Shai, L. J., Magano, S. R., Lebelo, S. L., & Mogale, A. M. (2011). Inhibitory effects of five medicinal plants on rat alpha-glucosidase: Comparison with their effects on yeast alpha-glucosidase. Journal of Medical Plants Research, 5(13), 2863–2867. https://doi.org/https://doi.org/10.5897/JMPR.9000811
- Silveira, S. T., Martínez-Maqueda, D. I., Recio, I., & Hernández-Ledesma, B. (2013). Dipeptidyl peptidase-IV inhibitory peptides generated by tryptic hydrolysis of a whey protein concentrate rich in β-lactoglobulin. Food Chemistry, 141(2), 1072–1077. https://doi.org/https://doi.org/10.1016/j.foodchem.2013.03.056
- Singh, V., Krishan, P., & Shri, R. (2016). Extraction of antioxidant phytoconstituents from onion waste. Journal of Pharmacognosy and Phytochemistry, 6(1), 502–505. https://www.phytojournal.com/archives/?year=2017&vol=6&issue=1&page=8
- Tulipano, G., Sibilia, V., Caroli, A. M., & Cocchi, D. (2011). Whey proteins as source of dipeptidyl dipeptidase IV (dipeptidyl peptidase-4) inhibitors. Peptides, 32(4), 835–838. https://doi.org/https://doi.org/10.1016/j.peptides.2011.01.002
- Uchida, M., Ohshiba, Y., & Mogami, O. (2011). Novel dipeptidyl peptidase-4–Inhibiting peptide derived from β-Lactoglobulin. Journal of Pharmacological Sciences, 117(1), 63–66. https://doi.org/https://doi.org/10.1254/jphs.11089SC
- Velderrain-Rodriguez, G. R., Palafox-Carlos, H., Wall-Medrano, A., Ayala-Zavala, J. F., Chen, C. Y. O., Robles-Sanchez, M., Astiazaran-Garcia, H., Alvarez-Parrilla, E., & Gonzalez-Aguilar, G. A. (2014). Phenolic compounds: Their journey after intake. Food & Function, 5(2), 189–197. https://doi.org/https://doi.org/10.1039/c3fo60361j
- Vinayagam, R., & Xu, B. (2015). Antidiabetic properties of dietary flavonoids: A cellular mechanism review. Nutrition Metabolism, 12(60). https://doi.org/https://doi.org/10.1186/s12986-015-0057-7