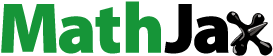
ABSTRACT
The Leucaena leucocephala seeds (LLS) cotyledon proteins, globulins, and glutelins were independently hydrolyzed by Alcalase, Trypsin, and α-Chymotrypsin up to 180 min. The degree of hydrolysis (DH), antioxidant, ACE-inhibitory, and α-Amylase inhibition were assessed. The higher DH values were 76.75% and 82.71% for globulin α-Chymotrypsin, and glutelin Alcalase hydrolysates, respectively. The glutelin hydrolyzed with Trypsin showed the higher DPPH• antioxidant activity while the higher ABTS antioxidant activity was for globulin Alcalase hydrolysate after 180 min digestion. The highest ACE-inhibitory activity was 95.44% for globulin-Alcalase hydrolysate at 180 min; while, for the α-Amylase inhibition assay, glutelin-Alcalase showed the highest values at 100 min of reaction. LLS cotyledon protein concentrates and hydrolysates with relatively low mimosine content were obtained after protein extraction. Such findings indicate the possibility of getting bioactive peptides from LLS cotyledon proteins using enzyme digestions and might be utilized for functional foods with physiological enhancer effects.
RESUMEN
El concentrado proteico y las fracciones de globulinas y glutelinas del cotiledón de semillas de Leucaena leucocephala (LLS) fueron hidrolizados con Alcalasa, Tripsina y α-quimotripsina hasta 180 min. El grado de hidrólisis (DH), actividades antioxidante e inhibitoria de las enzimas convertidora de angiotensina (ACE-I) y α-amilasa fueron medidas. Los grados de hidrólisis más altos fueron 76.75% y 82.71% para los hidrolizados de globulina-α quimotripsina y glutelina-Alcalasa, respectivamente. El hidrolizado de glutelina con Tripsina mostró la mayor actividad antioxidante con DPPH, mientras que el hidrolizado de globulina con alcalasa fue el más alto a los 180 min en el método ABTS. La mayor actividad de ACE-I (95.44%) se observó en el hidrolizado de globulinas-Alcalasa. Por el contrario, el hidrolizado de glutelinas-Alcalasa a los 100 min mostró la mayor actividad. Se obtuvieron concentrados proteicos e hidrolizados con un contenido de mimosina relativamente bajo. Estos resultados indican la posibilidad de obtener péptidos bioactivos a partir del cotiledón de LLS usando digestión enzimática los cuales podrían utilizarse en alimentos funcionales con efectos fisiológicos.
Introduction
In the last decades, the degenerative disease rate has increased due to lifestyle changes (Delles et al., Citation2018). Nowadays, diseases such as hypertension and other cardiovascular are the leading cause of morbidity and mortality in Western countries. About 20% of the adult population suffers from hypertension (Fan et al., Citation2019). Moreover, CDC (Citation2020) reported a prevalence of 45% hypertensive adults in the United States. The control of blood pressure is mediated by various regulatory mechanisms in the body, including the angiotensin I-converting enzyme (ACE-I) (Wu et al., Citation2006). This enzyme produces angiotensin II, using angiotensin I as substrate. The final product of ACE-I is the octapeptide Angiotensin II, which causes peripheral vasoconstriction, and sodium reabsorption in the kidney while increasing body fluids.
Additionally, angiotensin III, produced from angiotensin II, controls the production of aldosterone and raises blood pressure (Martinez-Maqueda et al., Citation2012). The discovery of bioactive peptides in snake venom led to the synthesis of ACE-I inhibitors such as captopril and enalapril, currently used to treat hypertension in humans. However, synthetic drugs could produce side effects such as cough, taste alterations, and skin rashes. Therefore, natural ACE-I inhibitors’ interest has increased (Daskaya-Dikmen et al., Citation2017).
On the other hand, reactive oxygen species (ROS) such as the superoxide radical, hydrogen peroxide, and hydroxyl radicals are physiological metabolites formed due to aerobic organisms’ respiration (Siow & Gan, Citation2013; Yea et al., Citation2014). ROS are unstable and can react rapidly with membrane lipids, proteins, and DNA, resulting in cell damage (Rudolph et al., Citation2017). The imbalance between oxidizing species and endogenous antioxidants causes oxidative stress. Moreover, oxidative stress has been associated with degenerative diseases like cardiovascular disorders and type II diabetes (Martinez-Leo et al., Citation2019). The intake of antioxidants could reduce the morbidity and mortality associated with coronary heart diseases, as demonstrated in several studies (Durak et al., Citation2013). Consequently, the search for food antioxidants that could protect the body from ROS and retard many chronic diseases’ evolution is steadily increasing (López-Barrios et al., Citation2014).
Studies on foods as natural sources of ACE-I inhibitors and antioxidants fall clearly within the scope of functional foods research. ACE-I inhibitory and antioxidant peptides derived from food proteins are excellent potential ingredients in functional foods (Torruco-Uco et al., Citation2009). These protein fragments may be released from the parent protein in vivo (i.e. during gastrointestinal digestion) or in vitro (i.e. during food processing). Bioactive peptides with ACE-I α-amylase-inhibitory and antioxidant activities have been produced through commercial enzyme digestion (Barbana & Boye, Citation2010).
The Guaje (Leucaena leucocephala) is a legume crop grown predominantly in Mexico’s tropical and subtropical areas. L. leucocephala seeds are currently used as a dietary protein source in both human and animal diets. However, they could be an excellent raw material of protein concentrates (Zárate et al., Citation2005). L. leucocephala is not recommended for extensive human consumption due to mimosine. Mimosine is an alkaloid that causes hair loss, growth retardation, cataract, goiter, and decreased fertility, and mortality in non-ruminant mammals. Furthermore, L. leucocephala seed protein isolates with relatively low mimosine levels have been prepared successfully by isoelectric precipitation of seed kernel proteins; it has been used to overcome mimosine toxicity since mimosine was left in the supernatant (Sethi & Kulkarni, Citation1993).
Protein extracts have been used to produce hydrolysates with improved functionality and/or nutritional properties (López-Barrios et al., Citation2014). Vegetable proteins are exciting, and legumes are especially promising because of their high protein content and diverse physiological activities in humans, for instance, antihypertensive and antioxidant effects (Lammi et al., Citation2019; Rudolph et al., Citation2017). Guaje protein hydrolysates may also represent a source of bioactive peptides with potential applications in developing novel physiologically functional foods, enhancing the value of the hydrolysates and overall value of the starting material, i.e. protein extracts.
This work’s objective was to determine in vitro antioxidant, ABTS•+ and DPPH• scavenging activities, ACE-I, and α-Amylase inhibitory properties for Leucaena leucocephala seeds (LLS) cotyledon protein hydrolyzed with Alcalase, Trypsin, and α-Chymotrypsin. Additionally, the mimosine reduction during the preparation of protein concentrates was evaluated.
Materials and methods
ACE (EC: 3.4.15.1, from porcine kidney, 0.5 U), α-Amylase (EC: 3.2.1.1, from porcine pancreas, 2 U), Alcalase® (EC: 3.4.21.62, from Bacillus licheniformis, ≥2.4 U/g protein), Trypsin® (EC: 3.4.21.4 from bovine pancreas, 2000 U/mg protein), α-Chymotrypsin® (EC: 3.4.21.1 from bovine pancreas, ≥40 U/mg protein) and mimosine, were purchased from Sigma, St. Louis, MO, USA while HPLC-grade acetonitrile was purchased from Fisher Scientific, Nepean, ON, Canada. All other chemicals were reagent and obtained from Sigma or Fisher Scientific. HPLC-grade water was generated by a Milli-Q system (Millipore, Bedford, MA, USA).
Raw material preparation
The pods of L. leucocephala, without visible morphological alterations, were obtained at a local market in Oaxaca, Mexico. First, the seeds were separated from the pod and soaked in distilled water for 2 h. Afterward, seeds were manually dehulled, and the cotyledon obtained was air-dried at 60°C during 48 h (BinderTM ED 23, Germany), ground in an electric miller (KrupsTM GX4100, Germany) and sieved through a 625 μm mesh. Finally, LLS flour was kept at −40°C until usage.
Protein concentrates preparation
Globulins and glutelins protein concentrates were obtained by isoelectric precipitation following the method of Rodriguez-Ambriz et al. (Citation2005). Briefly, to generate globulins concentrates, the LLS cotyledon flour was mixed with NaCl 5% solution in a 1:10 (w/v) ratio and then stirred for 2 h at room temperature with mechanical agitation. After this time, the suspension was centrifuged at 5000 x g for 15 min at 4°C (Thermo Scientific SorvallTM ST 16). After that, the supernatant was collected, and the extractions were repeated twice on the remaining seed flour at the same flour/solvent ratio. For glutelins extraction, the LLS cotyledon flour was suspended in water (10% w/v), and the pH was adjusted to 9, adding 1 N NaOH, using the same conditions previously described. The supernatant of each extract, collected separately, was joined, and the protein from both supernatants was acid precipitated (pH 6) with 0.1 N HCl and recovered by centrifugation (5000 x g, 15 min, 4°C). The supernatants were discarded. Afterward, each protein pellet was washed twice with distilled water, centrifuged (5000 x g for 15 min at 4°C), and immediately lyophilized (Labconco™ Triad 740040, USA). Once lyophilized, globulins and glutelins concentrates were stored at −40°C until use.
Protein hydrolysis
Globulin and glutelin enzymatic hydrolysis were carried out according to Boschin et al. (Citation2014). Three independent enzymes: Alcalase, Trypsin, and α-Chymotrypsin, were used in a completely randomized block design. The globulin or glutelin concentrates were dispersed in water to obtain a 1% solution (w/v), and the enzyme: substrate ratio was 1:100 (w:v). Hydrolysis with Alcalase was performed at 50°C and pH 8, while hydrolysis with Trypsin and α-Chymotrypsin were conducted at 37°C and pH 7.8. The pH was kept constant during the hydrolysis process by adding 0.1 N HCl or 0.1 N NaOH. Aliquots were removed at time intervals from 0, each 20 min until 180 min, and immediately heated at 95°C in a water bath for 15 min to stop the enzyme reaction. Then the hydrolysates were obtained by centrifugation at 10,000 g for 15 min and stored at −40°C until use.
Degree of hydrolysis measurement
The degree of hydrolysis (DH) was calculated according to Nielsen et al. (Citation2001) as DH = h/htot × 100, where htot is the total number of peptide bonds per protein equivalent, and h is the number of hydrolyzed bonds. The htot factor is dependent on the amino acid composition of the raw material. For LLS, values for α, β, and htot were 1, 0.4, and 8.3, respectively (Nielsen et al., Citation2001) for specific raw materials. Triplicate determinations were performed and measured at 340 nm, using a microplate reader (xMarkTM Microplate Spectrophotometer). The value of h was calculated using EquationEquations (1(1)
(1) ) and (Equation2
(2)
(2) ).
Where, serine-NH2 = meq serine NH2/g protein; X = g sample; P = protein % in sample; 0.1 is the sample volume.
DPPH• scavenging activity
The DPPH• (2,2-diphenyl-1-picryl- hydrazyl-hydrate) scavenging activity of LL globulins and glutelins hydrolysates was measured by the method of Bkhairia et al. (Citation2016). Twenty microliters of the sample were applied to a 96-well plate, and 200 μL of 125 µM DPPH solution in methanol was added. The sample’s absorbance was determined at 515 nm after 90 min of incubation. The control was a mixture of 20 μL distilled water and 200 μL of 125 µM DPPH. The DPPH scavenging activity was calculated using EquationEquation (3)(3)
(3) .
ABTS•+ method
The ABTS•+ (2,2′-azino-bis(3-ethylbenzothiazoline-6- sulfonic acid) scavenging activity of LLS hydrolysates was determined by the method of Karamać et al. (Citation2014) carrying out the reaction on 96 multi-well plates. The ABTS•+ radical was prepared by the reaction of 5 mL of 7 mM ABTS solution and 88 μL of 140 mM potassium persulfate solution. The mixture was kept in the dark at room temperature for 16 h. After this time, 1 mL of the mixture was diluted with ethanol to obtain absorbance from 0.70 ± 0.05 at 734 nm. Then, 20 µL portions of the sample were applied onto a 96-well plate, and 200 µL of ABTS•+ solution was added. The absorbance was read after 6 min at 734 nm. Deionized water was used as control. Percentage inhibition of the ABTS•+ radical was then calculated using EquationEquation (4)(4)
(4) .
Angiotensin I-converting enzyme inhibitory assay
ACE inhibitory activity was performed by the in vitro method of Wu et al. (Citation2002). ACE 0.1 U/mL and 5 mM HHL (hyppuryl-histidyl-leucine) were prepared by dissolution in 100 mM borate buffer (100 mM, pH 8.3) supplemented with 300 mM NaCl. Also, 5 mM of HA (control) and 5 mM Captopril (standard ACE inhibitor) were dissolved in distilled water. The reaction system was: 10 µL HHL, 10 µL ACE, 40 µL ACE inhibitors (hydrolysates), and 40 µL 100 mM borate buffer (100 mM, pH 8.3). The reaction mixture was incubated at 37°C for 30 min, and then 250 µL HCl was added.
Samples were analyzed using an RP-HPLC in an Agilent Technologies 1200 series (Palo Alto, CA, USA) equipped with a Zorbax Eclipse XDB–C18 column (5 µm, 4.6 µm i.d. × 150 mm, Agilent, USA) at 25°C. The injection volume was 10 µL. The column was eluted (0.5 mL/min) with two solvent systems (A) 0.05% TFA in water and (B) acetonitrile (ACN), with a 5-60% ACN gradient for the first 10 min, maintained for 2 min at 60% ACN, then returned to 5% ACN for 1 min, followed by isocratic elution for 4 min. Hypuric acid was detected in a diode array detector set a 225 nm. The inhibitory rate was calculated as described by Wu et al. (Citation2002).
α-Amylase inhibitory assay
The determination of α-Amylase inhibitory activity was conducted according to the method described by Siow et al. (Citation2017). In brief, 100 μL of the sample and 100 μL of sodium phosphate buffer 20 mM (with NaCl 6 mM, pH 6.9) containing α-Amylase solution (2 U/mL) were mixed and incubated for 10 min at 25°C. After this time, 100 μL of starch solution (1% w/v) in sodium phosphate buffer 20 mM (with NaCl 6 mM, pH 6.9) was then added to start the reaction. The reaction mixture was incubated at 25°C for 10 min and then boiled for 5 min in a water bath after adding 200 μL of dinitrosalicylic acid reagent (DNS) to halt the reaction. The mixture was cooled to room temperature and diluted with 3 mL of distilled water. The absorbance of the sample, control 1 (a mixture of starch solution and sample, without the addition of the enzyme), and control 2 (a mixture of starch solution and enzyme, without the addition of the sample) was measured at 540 nm using a UV-Vis spectrophotometer (xMarkTM Microplate Spectrophotometer). The α-Amylase inhibitory activity was expressed as percent inhibition.
Mimosine content
Mimosine content was determined in the LLS cotyledon flour saline, alkaline extracts, the protein concentrates (globulins and glutelins), and the 180 min protein hydrolysates following the protocol of Rodrigues-Corrêa et al. (Citation2019). Briefly, the dry LLS cotyledon flour (20 mg) was mixed in a proportion of 1:10 (w/v) with NaCl 5% or NaOH 1 M pH 8; both mixtures were vortexed for 1 min. All tube samples were centrifuged at 5000 x g for 15 min at 4°C, and the supernatants were filtered through a 0.2 μm membrane. Samples were analyzed in an Agilent Technologies 1200 series with a Zorbax Eclipse XDB–C18 column (5 µm, 4.6 µm i.d. × 150 mm) at 60℃. A total of 10 μL of sample solution was injected into the column and eluted with a mobile phase of 0.02 M orthophosphoric acid (a constant flow of 1 mL/min). Mimosine detection was done at 280 nm, showing a retention time of 1.48 ± 0.042 min. Mimosine content in the supernatants was calculated using a calibration curve (from 0.5-0.0001 mg/mL).
Statistical analysis
Results were expressed as the mean ± standard deviation (SD) of six determinations. Tukey’s HSD test performed a comparison of the means at a 5% level of significance. A Two-way ANOVA for pH values, enzyme, and their interaction was also conducted to identify significant factors affecting the resulting data. The correlation was estimated by the method of Pearson (P < .05) using the software Minitab 18 (Minitab Inc., State College, PA, USA).
Results and discussion
Protein hydrolysis
Alcalase, Trypsin, and α-Chymotrypsin were used to produce hydrolysates from LLS cotyledon globulin and glutelin protein concentrates. The DH behaved similarly between the independent enzymatic systems with the higher values of 78.40%, 72.71%, and 42.52% for globulins with α-Chymotrypsin, Alcalase, and Trypsin respectively at 180 min. Simultaneously, DH values for glutelin fraction were 82.71%, 62.75%, and 40.64% with Alcalase, α-Chymotrypsin, and Trypsin hydrolysis at 180 min, respectively (). These values were higher than those reported for other non-conventional legumes like Vigna subterranea, where maximum DH was 38%, 27.5%, and 22% using Alcalase, Thermolysin, and Trypsin, respectively, after 24 h hydrolysis (Mune Mune et al., Citation2018). And, even higher than the DH values observed for lentil hydrolysates (Lens culinaris var. Castellana) produced by Alcalase, Savinase, and Protamex (23%, 15%, and 11%) after 4 h of hydrolysis (Garcia-Mora et al., Citation2014). The variation in DH observed in is probably the result of protease specificity and protein source. Microbial proteases such as Alcalase (an industrial alkaline protease from Bacillus licheniformis) can produce hydrolysates with biological activities, similar to animal (gastrointestinal) Trypsin and α-Chymotrypsin or plant-derived proteases. This is consistent with previous reports, since the hydrolysates with Alcalase exhibited the greatest DH for the underutilized legume Macrotyloma uniflorum (45%), for Phaseolus lunatus (40%), and Phaseolus vulgaris (50%) protein (Bhaskar et al., Citation2019; Torruco-Uco et al., Citation2009). Furthermore, Alcalase presents broad specificity and hydrolyzes most peptide bonds, which produce small molecular weight peptides, especially those containing aromatic amino acid residues (Phe and Tyr), and releasing peptides with hydrophobic amino acids residues such as Trp, Leu, Ile, Val, and Met. At the same time, Trypsin cleaves mainly C‐terminal to hydrophilic amino acid residues such as Arg and Lys. α-Chymotrypsin hydrolyzes specifically peptide bonds formed by C‐terminal aromatic amino acids residues of Tyr, Phe, Trp, and Leu, and to a lower extent, Gln, Ser, and Thr. Several researches reported that protein hydrolysates with a DH value around or higher to 10% have a promising medical application usage. On the other hand, extensive hydrolysis produced low molecular weight peptides with hydrophobic amino acid residues associated with bioactivities such as antioxidant or ACE inhibition), while hydrolysates with lower DH values could be used to improve the functional properties of flours and foods (Clemente et al., Citation1999; Zhong et al., Citation2007). Regarding the ACE-inhibitory bioactive peptide properties, the ACE-I prefers substrates containing hydrophobic (aromatic or branched lateral chain) amino acid residues. In contrast, hydrophobic (Ala, Leu, Phe, Val, Pro, and Gly) and hydrophilic amino acids (Cys, Met, His, and Ser) have been proposed to inhibit the α-Amylase activity. Alcalase and α-Chymotrypsin are suitable for releasing bioactive peptides, such as those with ACE-I and α-Amylase inhibitory action. Consequently, our findings suggest that in both LLS globulins and glutelins hydrolysates, the hydrophobic amino acid content is comparable to that previously reported in other protein sources when hydrolyzed with Alcalase, α-Chymotrypsin, and Trypsin.
Figure 1. Degree of hydrolysis depending on reaction time (min) in (a) globulins and (b) glutelins of Leucaena leucocephala seed cotyledon protein concentrates by Alcalase, Trypsin, and α-Chymotrypsin. Analysis were done in triplicates. Different letters on each line indicate significantly different at P < .05.
Figura 1. Grado de hidrólisis dependiente del tiempo de reacción (min) en (a) globulinas y (b) glutelinas de los concentrados proteicos del cotiledón de Leucaena leucocephala con Alcalasa, Tripsina y α-quimotripsina. Los análisis de hicieron por triplicado. Diferentes letras en una misma línea indican diferencias significativas a P < .05
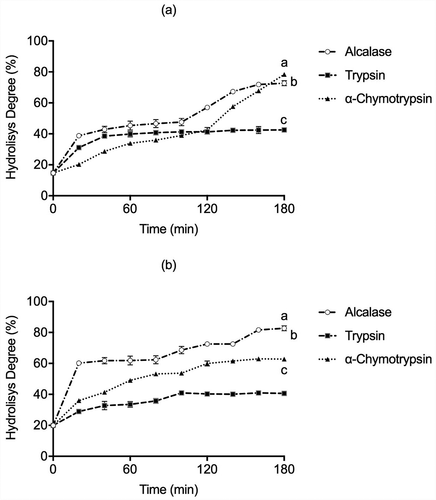
Antioxidant activity by DPPH
In this study, free radical scavenging activities of the hydrolysates prepared with Alcalase, Trypsin, and α-Chymotrypsin were determined by the DPPH• method, used to measure the ability of antioxidant compounds to donate electrons or hydrogen ion to free radicals to form a stable species (). The highest DPPH• antioxidant activity was observed for glutelins fraction hydrolysates using Trypsin (37.11%) and by α-Chymotrypsin (36.43%), while the globulin hydrolysate produced by α-Chymotrypsin showed higher activity (33.63%) than those prepared with Alcalase and Trypsin after 180 min of hydrolysis. Similar results were reported for Bambara groundnut (Vigna subterranea) hydrolysates generated with Alcalase, Trypsin, and Pepsin, being the DPPH• radical-scavenging activity around 38% to 40% (Arise et al., Citation2016). Zhang et al. (Citation2010) reported 29% DPPH activity in alcalase soy hydrolysates, and Valdez-Ortiz et al. (Citation2012) reported that Azufrado Higuera bean hydrolyzed with Alcalase showed a 44% DPPH scavenging activity. The Alcalase hydrolysate of African yam bean seed (Sphenostylis stenocarpa) showed around 35% of DPPH• radical-scavenging activity (Ajibola et al., Citation2011). On the other hand, Pigeon pea (Cajanus cajan) protein hydrolyzed with Pepsin-Pancreatin presented 36.30% of DPPH• discoloration (Olagunju et al., Citation2018), indicating the release and exposure of peptides with enhanced radical scavenging activity during enzymatic proteolysis.
Figure 2. DPPH• antioxidant activity in (a) globulins and (b) glutelins of Leucaena leucocephala seed cotyledon protein hydrolysates by Alcalase, Trypsin, and α-Chymotrypsin. All experiments were done in triplicates. Different letters on each line indicate significantly different at P < .05.
Figura 2. Actividad antioxidante por DPPH en (a) globulinas y (b) glutelinas en los hidrolizados proteicos de proteínas de cotiledón de Leucaena leucocephala producidos con Alcalasa, Tripsina y α-quimotripsina. Los análisis de hicieron por triplicado. Diferentes letras en una misma línea indican diferencias significativas a P < .05
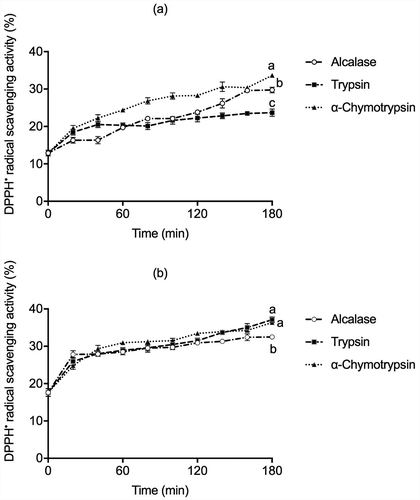
Furthermore, extensive protein hydrolysis with Pepsin-Pancreatin would likely release smaller-sized peptides that could easily interact with free radicals. It is well known that hydrophobic amino acids and particularly His, Pro, Met, Cys, Tyr, Trp, and Phe may enhance bioactive peptides’ antioxidant activity through proton donation DPPH• radicals (Durak et al., Citation2013; López-Barrios et al., Citation2014).
ABTS antioxidant activity
The results of ABTS˙+ scavenging activity are reported in . All hydrolysates from globulins and glutelins with Alcalase, Trypsin, and α–Chymotrypsin showed high ABTS•+ scavenging activity, in the range of 60-85%, during the first 20 min of hydrolysis. These results were higher than the 53.3% of ABTS•+ scavenging activity of Pinto beans (Phaseolus vulgaris cv. Pinto) hydrolysate after 30 min of Protamex treatment (Ngoh & Gan, Citation2016). Valdez-Ortiz et al. (Citation2012) reported 73% and 75.3% for ABTS•+ scavenging activity of Azufrado beans (Phaseolus vulgaris) protein hydrolysate obtained by Pepsin and Pancreatin, respectively, after two h of hydrolysis. Olagunju et al. (Citation2018) reported there was no significant difference (P < .05) between ABTS•+ scavenging activity of pancreatin (73.8%) and Pepsin-Pancreatin (71.1%) from Pigeon pea (Cajanus cajan) hydrolysates (4 h of hydrolysis). The assessment of the ABTS•+ radical scavenging activity is used in both types of hydrophilic and lipophilic compounds. A high degree of hydrolysis and consequently high content of released amino acid groups should increase the hydrolysates’ antioxidant capacity (López-Barrios et al., Citation2014; Torruco-Uco et al., Citation2009). However, these findings suggest that the high ABTS•+ radical scavenging activity in the early stages of hydrolysis with the proteases cannot be directly attributed to the DH as the main factor but to the structure of the peptide and the sequence of amino acid. Other factors that could modulate the inactivation of ABTS free radical are the increase in polarity due to the release of low-molecular-weight peptides, the increase in the number of ionizable groups acting as proton donors, and the exposure of hydrophobic side-chain groups (Leu, Pro, His, Cys, and Met) and aromatic amino acids (Trp, Tyr, and Phe) residues (Yi-Shen et al., Citation2018).
Figure 3. ABTS•+ antioxidant activity in (a) globulins and (b) glutelins of Leucaena leucocephala seed cotyledon protein hydrolysates by Alcalase, Trypsin, and α-Chymotrypsin. Experiments were done in triplicates. Different letters on each line indicate significantly different at P < .05.
Figura 3. Actividad antioxidante por ABTS en (a)globulinas y (b)glutelinas en los hidrolizados proteicos de proteínas de cotiledón de Leucaena leucocephala producidos con Alcalasa, Tripsina y α-quimotripsina. Los análisis de hicieron por triplicado. Diferentes letras en una misma línea indican diferencias significativas a P < .05
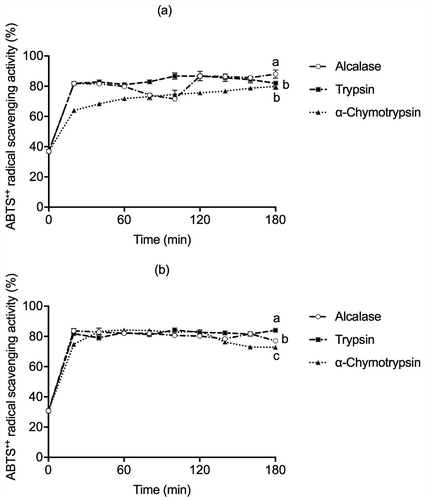
ACE inhibitory activity
The bioactive peptides with high antioxidant activity may also be able to reduce blood pressure in cardiovascular diseases (Martinez-Maqueda et al., Citation2012); thus, angiotensin-converting enzyme (ACE) inhibitory activity of protein hydrolysates was also measured (). The glutelin Alcalase hydrolysis showed an ACE inhibitory activity of around 85% within 60 min of reaction. It reached its highest activity (95.44%) at 180 min, while in globulins, the Trypsin and Alcalase hydrolysates showed an 88.47% and 86.93% of ACE inhibitory activity, respectively. Similar results have been observed for different protein hydrolysates, Horse gram (Macrotyloma uniflorum) hydrolysate with a DH of 40% using Alcalase after 3 h of reaction, suggesting that there might be an optimal DH, after which the hydrolysis of ACE inhibitory peptides leads to the production of inactive peptides or free amino acids (Bhaskar et al., Citation2019). Our ACE inhibition values were significantly lower than the positive control, captopril (98.56% of ACE inhibition) but higher than those of Lentil (Lens culinaris var. Castellana) hydrolyzed with Alcalase (71%), Savinase (63%), Protamex (41%) and Corolase 7089 (50%) regardless of hydrolysis time (2 h). The ACE-inhibitory activity could be directly associated with chain length and peptide sequence (Garcia-Mora et al., Citation2014). Furthermore, Guo et al. (Citation2018) observed that Lupin protein hydrolyzed with Alcalase, Trypsin, and Pepsin displayed a 56.25% within 60 min, 65% after 120 min, and 67.21% ACE inhibition at 15 min, respectively. ACE–inhibitory efficiency is also associated with short peptide chains containing between 3-12 amino acid residues, interacting with the ACE-I active site’s three subsites. Aromatic and hydrophobic amino acids (Phe, Tyr, Pro, and Trp) have been reported as the most favorable C-terminal residues for ACE inhibition (Wu et al., Citation2006). Thus, the ACE inhibition may be associated to the different peptide endings caused by enzymatic proteolysis (López-Barrios et al., Citation2014).
Figure 4. ACE-I inhibition activity in (a) globulins and (b) glutelins of Leucaena leucocephala seed cotyledon protein hydrolysates from globulin and glutelin by Alcalase, Trypsin, and α-Chymotrypsin. Experiments were done in triplicates. Different letters on each line indicate significantly different at P < .05.
Figura 4. Actividad inhibitoria de la enzima convertidora de angiotensina (ACE-I) en (a)globulinas y (b)glutelinas en los hidrolizados proteicos de proteínas de cotiledón de Leucaena leucocephala producidos con Alcalasa, Tripsina y α-quimotripsina. Los análisis de hicieron por triplicado. Diferentes letras en una misma línea indican diferencias significativas a P < .05
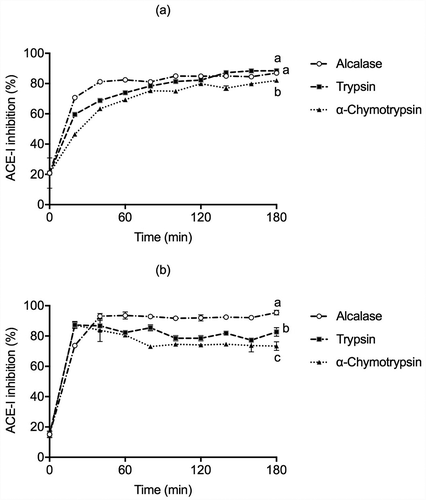
α-Amylase inhibitory activity
α-Amylase is one of the main enzymes involved in the digestion of dietary starch, releasing oligosaccharides that can be further broken down into glucose, which is fast absorbed by the body. Therefore, α-Amylase activity inhibition is an effective strategy for managing blood glucose in diabetic patients (Lammi et al., Citation2019). In , results suggested that the hydrolysis time and the kind of proteases used were affected by cotyledon proteins’ activity. Hence, Alcalase globulin hydrolysate (hydrolysis time from 20 min to 180 min) displayed a higher inhibition of 74.21% at 100 min than glutelin Alcalase hydrolysate with the higher α-Amylase inhibition activity of 58.45% at 100 min. Furthermore, Trypsin and α-Chymotrypsin globulins hydrolysates reach the highest α-Amylase inhibitory activity up to 48.42% and 55.43% within 80 and 100 min, respectively; but displayed a slight decline at 120 min. However, after 180 min of the enzymatic reaction, lower α-Amylase inhibition activity and significant differences were found, whereas Alcalase glutelins treatment exhibited a maximum value at 100 min (91.51%). The α-Chymotrypsin and Trypsin glutelin hydrolysis gradually increased α-Amylase inhibitory activity of hydrolysates. In contrast, hydrolysis of more than 60 and 100 min resulted in a rapid reduction of α-Amylase inhibition activity.
Figure 5. α-Amylase inhibition activity in (a) globulins and (b) glutelins of Leucaena leucocephala seed cotyledon protein hydrolysates from globulin and glutelin by Alcalase, Trypsin, and α-Chymotrypsin. Experiments were done in triplicates. Different letters on each line indicate significantly different at P < .05.
Figura 5. Actividad inhibitoria de la α-amilasa en (a)globulinas y (b)glutelinas en los hidrolizados proteicos de proteínas de cotiledón de Leucaena leucocephala producidos con Alcalasa, Tripsina y α-quimotripsina. Los análisis de hicieron por triplicado. Diferentes letras en una misma línea indican diferencias significativas a P < .05
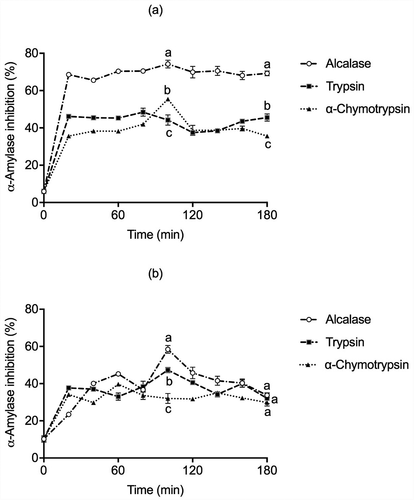
The high α-Amylase inhibitory activity for globulin α-Chymotrypsin and glutelin Alcalase hydrolysates could be due to the release of a smaller and narrower-size range of peptides generated within 20 min to 100 min of reaction. It was proposed that these peptides were bound at the substrate-binding site of α-Amylase, affecting the interaction to the carbohydrate by steric hindrance. Hydrophobic interactions may also contribute to conformational changes on the substrate-binding region of α-Amylase, inhibiting the hydrolysis process (Awosika & Aluko, Citation2019). These results are consistent with those reported by Oseguera-Toledo et al. (Citation2015), where the non-hydrolyzed protein had around 30% of α-Amylase inhibition. After 120 min of hydrolysis, all hydrolysates showed the highest inhibition of α-Amylase, approximately 67%. These promising results indicate that LLS cotyledon could be a protein source to produce bioactive peptides with potential anti-diabetic properties.
Mimosine content
shows the mimosine concentration in Leucaena leucocephala seeds (LLS) cotyledon, protein concentrates, hydrolysates, and the percentage of mimosine reduction (%). Most mimosine was extracted in the first step during the protein concentrates preparation, either with NaCl 5% or NaOH 1 N. After acid precipitation, mimosine was reduced by 87.66%. These results agree with previous reports (Ekpenyong, Citation1986; Rodrigues-Corrêa et al., Citation2019; Sethi & Kulkarni, Citation1993). The LLS mimosine concentration varies widely among the various cultivars of the species. Mimosine accounts for approximately 60% of the total free amino acids in LLS (Sethi & Kulkarni, Citation1995). The reduction in mimosine content could be related to its small size and high water solubility. Several reports claim that the preparation of seed-protein concentrates yields proteins relatively free of some inherent toxic constitutes (Bhat & Karim, Citation2009). LLS protein isolates prepared by isoelectric precipitation yielded relatively low mimosine levels (Sethi & Kulkarni, Citation1993). Thus, protein isolate preparation could be considered a means of using LLS proteins for food purposes, with minimal mimosine toxicity. The mimosine concentration decreases up to 64.92% in the next stepwise, the end of the protein hydrolysis reaction, especially in the glutelin fraction treated with Trypsin compared to the initial values. Thus, protein isolation and subsequent enzymatic hydrolysis could be considered as a way to utilize LLS cotyledon proteins with minimal interference from mimosine, avoiding its toxicity complications.
Table 1. Mimosine content and percentage of mimosine reduction (%) in extracts from cotyledon flour, protein (globulin and glutelin) concentrates and hydrolysates from Leucaena leucocephala.
Tabla 1. Contenido y porcentaje de reducción (%) de mimosina en los extractos de harina de cotiledón, concentrados proteicos (globulinas y glutelinas) e hidrolizados de Leucaena leucocephala.
Correlation coefficients
According to , there was a significant correlation between DH and all bioactivities of LLS cotyledon hydrolysates. The two-way ANOVA also indicated highly significant differences (P < .0001) between the reaction time and the kind of enzyme used. Thus, this might be the main contributor to the antioxidant, anti-α-Amylase, and anti-ACE activities of LLS cotyledon hydrolysates. Antioxidant assays, including ABTS•+, were especially strongly correlated with the anti-ACE and relatively strongly correlated to anti-α-Amylase activity assays at P < .05. Meanwhile, the DPPH• assay was not significantly associated with the α-Amylase inhibitory assay.
Table 2. Correlation table of bioactivity of the produced hydrolysates.
Tabla 2. Tabla de correlación de bioactividad de los hidrolizados proteicos
Conclusions
This study observed that protein hydrolysates derived from Leucaena leucocephala seed cotyledon possess antioxidant properties against ABTS•+ and DPPH• radicals. They presented the potential to inhibit enzymes related to chronic diseases as hypertension (ACE-I) and type-2 diabetes (relevant to the effect on α-Amylase). These functions could potentially find promising applications as nutraceutical ingredients to be used in functional foods to prevent and/or treat oxidative stress which constitutes relevant pathophysiology in many chronic diseases and provides added value to this crop. Therefore, protein isolation by isoelectric precipitation could be considered a means of utilizing L. leucocephala seed protein, with minimal interference from mimosine. Further research is required to isolate and characterize bioactive peptides with potent antioxidant, ACE, and α-Amylase inhibitory activity from Leucaena protein hydrolysates.
Acknowledgments
The first author acknowledges Consejo Nacional de Ciencia y Tecnología (CONACYT) for scholarship No. 305253.
Disclosure statement
No potential conflict of interest was reported by the author(s).
References
- Ajibola, C. F., Fashakin, J. B., Fagbemi, T. N., & Aluko, R. E. (2011). Effect of peptide size on antioxidant properties of African yam bean seed (Sphenostylis stenocarpa) protein hydrolysate fractions. International Journal of Molecular Sciences, 12(10), 6685–6702. https://doi.org/https://doi.org/10.3390/ijms12106685
- Arise, A. K., Alashi, A. M., Nwachukwu, I. D., Ijabadeniyi, O. A., Aluko, R. E., & Amonsou, E. O. (2016). Antioxidant activities of bambara groundnut (Vigna subterranea) protein hydrolysates and their membrane ultrafiltration fractions. Food & Function, 7(5), 2431–2437. https://doi.org/https://doi.org/10.1039/c6fo00057f
- Awosika, T. O., & Aluko, R. E. (2019). Inhibition of the in vitro activities of α-amylase, α-glucosidase and pancreatic lipase by yellow field pea (Pisum sativum L.) protein hydrolysates. International Journal of Food Science & Technology, 54(6), 2021–2034. https://doi.org/https://doi.org/10.1111/ijfs.14087
- Barbana, C., & Boye, J. I. (2010). Angiotensin I-converting enzyme inhibitory activity of chickpea and pea protein hydrolysates. Food Research International, 43(6), 1642–1649. https://doi.org/https://doi.org/10.1016/j.foodres.2010.05.003
- Bhaskar, B., Ananthanarayan, L., & Jamdar, S. (2019). Purification, identification, and characterization of novel angiotensin I-converting enzyme (ACE) inhibitory peptides from alcalase digested horse gram flour. LWT, 103, 155–161. https://doi.org/https://doi.org/10.1016/j.lwt.2018.12.059
- Bhat, R., & Karim, A. A. (2009). Exploring the nutritional potential of wild and underutilized legumes. Comprehensive Reviews in Food Science and Food Safety, 8(4), 305–331. https://doi.org/https://doi.org/10.1111/j.1541-4337.2009.00084.x
- Bkhairia, I., Ben Slama Ben Salem, R., Nasri, R., Jridi, M., Ghorbel, S., & Nasri, M. (2016). In-vitro antioxidant and functional properties of protein hydrolysates from golden grey mullet prepared by commercial, microbial and visceral proteases. Journal of Food Science and Technology, 53(7), 2902–2912. https://doi.org/https://doi.org/10.1007/s13197-016-2200-5
- Boschin, G., Scigliuolo, G. M., Resta, D., & Arnoldi, A. (2014). ACE-inhibitory activity of enzymatic protein hydrolysates from lupin and other legumes. Food Chemistry, 145, 34–40. https://doi.org/https://doi.org/10.1016/j.foodchem.2013.07.076
- CDC. (2020). Facts about hypertension. Retrieved September 30, from https://www.cdc.gov/bloodpressure/facts.htm
- Clemente, A., Vioque, J., Sanchez-Vioque, R., Pedroche, J., & Millán, F. (1999). Production of extensive chickpea (Cicer arietinum L.) protein hydrolysates with reduced antigenic activity. Journal of Agricultural and Food Chemistry, 47(9), 3776–3781. https://doi.org/https://doi.org/10.1021/jf981315p
- Daskaya-Dikmen, C., Yucetepe, A., Karbancioglu-Guler, F., Daskaya, H., & Ozcelik, B. (2017). Angiotensin-I-Converting Enzyme (ACE)-inhibitory peptides from plants. Nutrients, 9(4), 316. https://doi.org/https://doi.org/10.3390/nu9040316
- Delles, C., Carrick, E., Graham, D., & Nicklin, S. A. (2018). Utilizing proteomics to understand and define hypertension: Where are we and where do we go? Expert Review of Proteomics, 15(7), 581–592. https://doi.org/https://doi.org/10.1080/14789450.2018.1493927
- Durak, A., Baraniak, B., Jakubczyk, A., & Świeca, M. (2013). Biologically active peptides obtained by enzymatic hydrolysis of Adzuki bean seeds. Food Chemistry, 141(3), 2177–2183. https://doi.org/https://doi.org/10.1016/j.foodchem.2013.05.012
- Ekpenyong, T. E. (1986). Nutrient and amino acid composition of Leucaena leucocephala (Lam.) de Wit. Animal Feed Science and Technology, 15(3), 183–187. https://doi.org/https://doi.org/10.1016/0377-8401(86)90024-6
- Fan, H., Liao, W., & Wu, J. (2019). Molecular interactions, bioavailability, and cellular mechanisms of angiotensin-converting enzyme inhibitory peptides. Journal of Food Biochemistry, 43(1), e12572. https://doi.org/https://doi.org/10.1111/jfbc.12572
- Garcia-Mora, P., Peñas, E., Frias, J., & Martínez-Villaluenga, C. (2014). Savinase, the most suitable enzyme for releasing peptides from lentil (Lens culinaris var. Castellana) protein concentrates with multifunctional properties. Journal of Agricultural and Food Chemistry, 62(18), 4166–4174. https://doi.org/https://doi.org/10.1021/jf500849u
- Guo, X., Shang, W., Strappe, P., Zhou, Z., & Blanchard, C. (2018). Peptides derived from lupin proteins confer potent protection against oxidative stress. Journal of the Science of Food and Agriculture, 98(14), 5225–5234. https://doi.org/https://doi.org/10.1002/jsfa.9059
- Karamać, M., Kulczyk, A., & Sulewska, K. (2014). Antioxidant activity of hydrolysates prepared from flaxseed cake proteins using pancreatin. Polish Journal of Food and Nutrition Sciences, 64(4), 227–233. https://doi.org/https://doi.org/10.2478/pjfns-2013-0023
- Lammi, C., Aiello, G., Boschin, G., & Arnoldi, A. (2019). Multifunctional peptides for the prevention of cardiovascular disease: A new concept in the area of bioactive food-derived peptides. Journal of Functional Foods, 55, 135–145. https://doi.org/https://doi.org/10.1016/j.jff.2019.02.016
- López-Barrios, L., Gutiérrez-Uribe, J. A., & Serna-Saldívar, S. O. (2014). Bioactive peptides and hydrolysates from pulses and their potential use as functional ingredients. Journal of Food Science, 79(3), R273–R283. https://doi.org/https://doi.org/10.1111/1750-3841.12365
- Martinez-Leo, E. E., Martin-Ortega, A. M., Acevedo-Fernandez, J. J., Moo-Puc, R., & Segura-Campos, M. R. (2019). Peptides from Mucuna pruriens L., with protection and antioxidant in vitro effect on HeLa cell line. Journal of the Science of Food and Agriculture, 99(8), 4167–4173. https://doi.org/https://doi.org/10.1002/jsfa.9649
- Martinez-Maqueda, D., Miralles, B., Recio, I., & Hernandez-Ledesma, B. (2012). Antihypertensive peptides from food proteins: A review. Food & Function, 3(4), 350–361. https://doi.org/https://doi.org/10.1039/c2fo10192k
- Mune Mune, M. A., Minka, S. R., & Henle, T. (2018). Investigation on antioxidant, angiotensin converting enzyme and dipeptidyl peptidase IV inhibitory activity of Bambara bean protein hydrolysates. Food Chemistry, 250, 162–169. https://doi.org/https://doi.org/10.1016/j.foodchem.2018.01.001
- Ngoh, -Y.-Y., & Gan, C.-Y. (2016). Enzyme-assisted extraction and identification of antioxidative and α-amylase inhibitory peptides from Pinto beans (Phaseolus vulgaris cv. Pinto). Food Chemistry, 190, 331–337. https://doi.org/https://doi.org/10.1016/j.foodchem.2015.05.120
- Nielsen, P. M., Petersen, D., & Dambmann, C. (2001). Improved method for determining food protein degree of hydrolysis. Journal of Food Science, 66(5), 642–646. https://doi.org/https://doi.org/10.1111/j.1365-2621.2001.tb04614.x
- Olagunju, A. I., Omoba, O. S., Enujiugha, V. N., Alashi, A. M., & Aluko, R. E. (2018). Antioxidant properties, ACE/renin inhibitory activities of pigeon pea hydrolysates and effects on systolic blood pressure of spontaneously hypertensive rats. Food Science & Nutrition, 6(7), 1879–1889. https://doi.org/https://doi.org/10.1002/fsn3.740
- Oseguera-Toledo, M. E., Gonzalez de Mejia, E., & Amaya-Llano, S. L. (2015). Hard-to-cook bean (Phaseolus vulgaris L.) proteins hydrolyzed by alcalase and bromelain produced bioactive peptide fractions that inhibit targets of type-2 diabetes and oxidative stress. Food Research International, 76(Part 3), 839–851. https://doi.org/https://doi.org/10.1016/j.foodres.2015.07.046
- Rodrigues-Corrêa, K. C. D. S., Honda, M. D. H., Borthakur, D., & Fett-Neto, A. G. (2019). Mimosine accumulation in Leucaena leucocephala in response to stress signaling molecules and acute UV exposure. Plant Physiology and Biochemistry, 135, 432–440. https://doi.org/https://doi.org/10.1016/j.plaphy.2018.11.018
- Rodriguez-Ambriz, S. L., Martinez-Ayala, A. L., Millan, F., & Davila-Ortiz, G. (2005). Composition and functional properties of Lupinus campestris protein isolates. Plant Foods for Human Nutrition, 60(3), 99–107. https://doi.org/https://doi.org/10.1007/s11130-005-6835-z
- Rudolph, S., Lunow, D., Kaiser, S., & Henle, T. (2017). Identification and quantification of ACE-inhibiting peptides in enzymatic hydrolysates of plant proteins. Food Chemistry, 224, 19–25. https://doi.org/https://doi.org/10.1016/j.foodchem.2016.12.039
- Sethi, P., & Kulkarni, P. R. (1993). Fractionation of Leucaena seed-kernel proteins based on their solubility characteristics. Food Chemistry, 48(2), 173–177. https://doi.org/https://doi.org/10.1016/0308-8146(93)90053-I
- Sethi, P., & Kulkarni, P. R. (1995). Leucaena Leucocephala a nutrition profile. Food and Nutrition Bulletin, 16(3), 1–16. https://doi.org/https://doi.org/10.1177/156482659501600307
- Siow, H.-L., & Gan, C.-Y. (2013). Extraction of antioxidative and antihypertensive bioactive peptides from Parkia speciosa seeds. Food Chemistry, 141(4), 3435–3442. https://doi.org/https://doi.org/10.1016/j.foodchem.2013.06.030
- Siow, H. L., Lim, T. S., & Gan, C. Y. (2017). Development of a workflow for screening and identification of alpha-amylase inhibitory peptides from food source using an integrated bioinformatics-phage display approach: Case study - Cumin seed. Food Chemistry, 214, 67–76. https://doi.org/https://doi.org/10.1016/j.foodchem.2016.07.069
- Torruco-Uco, J., Chel-Guerrero, L., Martínez-Ayala, A., Dávila-Ortíz, G., & Betancur-Ancona, D. (2009). Angiotensin-I converting enzyme inhibitory and antioxidant activities of protein hydrolysates from Phaseolus lunatus and Phaseolus vulgaris seeds. LWT - Food Science and Technology, 42(10), 1597–1604. https://doi.org/https://doi.org/10.1016/j.lwt.2009.06.006
- Valdez-Ortiz, A., Fuentes-Gutiérrez, C. I., Germán-Báez, L. J., Gutiérrez-Dorado, R., & Medina-Godoy, S. (2012). Protein hydrolysates obtained from Azufrado (sulphur yellow) beans (Phaseolus vulgaris): Nutritional, ACE-inhibitory and antioxidative characterization. LWT - Food Science and Technology, 46(1), 91–96. https://doi.org/https://doi.org/10.1016/j.lwt.2011.10.021
- Wu, J., Aluko, R. E., & Muir, A. D. (2002). Improved method for direct high-performance liquid chromatography assay of angiotensin-converting enzyme-catalyzed reactions. Journal of Chromatography. A, 950(1–2), 125–130. https://doi.org/https://doi.org/10.1016/s0021-9673(02)00052-3
- Wu, J., Aluko, R. E., & Nakai, S. (2006). Structural requirements of Angiotensin I-converting enzyme inhibitory peptides: Quantitative structure-activity relationship study of di- and tripeptides. Journal of Agricultural and Food Chemistry, 54(3), 732–738. https://doi.org/https://doi.org/10.1021/jf051263l
- Yea, C. S., Ebrahimpour, A., Hamid, A. A., Bakar, J., Muhammad, K., & Saari, N. (2014). Winged bean [Psophorcarpus tetragonolobus (L.) DC] seeds as an underutilised plant source of bifunctional proteolysate and biopeptides. Food & Function, 5(5), 1007–1016. https://doi.org/https://doi.org/10.1039/c3fo60667h
- Yi-Shen, Z., Shuai, S., & FitzGerald, R. (2018). Mung bean proteins and peptides: Nutritional, functional and bioactive properties. Food & Nutrition Research, 62. https://doi.org/https://doi.org/10.29219/fnr.v62.1290
- Zárate, S., Pérez-Nasser, N., & Casas, A. (2005). Genetics of wild and managed populations of Leucaena esculenta Subsp. esculenta (Fabaceae; Mimosoideae) in La Montaña of Guerrero, Mexico. Genetic Resources and Crop Evolution, 52(7), 941–957. https://doi.org/https://doi.org/10.1007/s10722-003-6088-3
- Zhang, L., Li, J., & Zhou, K. (2010). Chelating and radical scavenging activities of soy protein hydrolysates prepared from microbial proteases and their effect on meat lipid peroxidation. Bioresource Technology, 101(7), 2084–2089. https://doi.org/https://doi.org/10.1016/j.biortech.2009.11.078
- Zhong, F., Zhang, X., Ma, J., & Shoemaker, C. F. (2007). Fractionation and identification of a novel hypocholesterolemic peptide derived from soy protein Alcalase hydrolysates. Food Research International, 40(6), 756–762. https://doi.org/https://doi.org/10.1016/j.foodres.2007.01.005