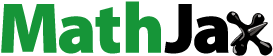
Abstract
Sago starch is an underutilized starch due to its low functionality in food formulation. Modification is effective in improving starch functional properties. In this study, the starch were modified to enhance its functional properties. The starch was microwave-heated with different treatment durations of 5, 10, 15, or 20 min, separately, yielding microwave-heated starches (MHT1, MHT2, MHT3, and MHT4). The modified starches have irregular shaped granules and fissures while maintaining their mean diameters. The degree of double helix had increased, indicating more double helix structure of amylose formed. The modified starches’ moisture content, solubility, and paste clarity were significantly decreased. The MHT4 had its swelling power and syneresis increase. In conclusion, the treatment duration influenced the physicochemical properties of the sago starch, which is related to the formation of amylose double helix structure. Further study should focus on the formation of double helix structure in starch and its impact towards food structure.
Resumen
El almidón de sagú es infrautilizado debido asu baja funcionalidad para la formulación de alimentos. Su modificación puede resultar eficaz para mejorar sus propiedades funcionales. El presente estudio modificó el almidón para lograr este objetivo. Para ello, el almidón fue calentado por microondas, realizándose tratamientos de diferentes duraciones, 5, 10, 15 o20 minutos, por separado, lo que dio lugar aalmidones calentados por microondas (MHT1, MHT2, MHT3 yMHT4). Los almidones modificados de esta manera presentaron gránulos yfisuras de forma irregular, manteniendo sus diámetros medios. Se constató que aumentó el grado de doble hélice, lo que indica que se formó una estructura adicional de doble hélice de la amilosa. Por otra parte, el grado de humedad, la solubilidad yla claridad de la pasta de los almidones modificados disminuyeron significativamente. El MHT4 incrementó su poder de hinchamiento ysu sinéresis. En conclusión, se comprobó que la duración del tratamiento incidió en las propiedades fisicoquímicas del almidón de sagú relacionadas con la formación de la estructura de doble hélice de la amilosa. Estudios adicionales deberían centrarse en la formación de la estructura de doble hélice en el almidón yen determinar su efecto en la estructura de los alimentos.
PALABRAS CLAVE:
Introduction
Sago starch is extracted from the pith of a palm plant, Metroxylon sagu. It is mainly produced and exported from Southeast Asia, especially Malaysia. Malaysia exports about 44,000 tonnes of sago starch yearly to many countries including Japan and Europe (Othman et al., Citation2015). Sago starch is considered underutilized when compared to starch from other sources, such as potato, corn, and rice. Studies have been conducted to improve the physicochemical properties of sago starch. Like other starches such as corn starch, modification of sago starch is essential to improve its properties and increase its usability. Various modification techniques, such as hydrolyzed-hydroxypropylation, octenyl succinic anhydride (OSA), alkaline, heat moisture, and gamma-irradiation treatments have been applied to sago starch (Abiddin et al., Citation2018; Oladzadabbasabadi et al., Citation2017; Othman et al., Citation2015; Ying et al., Citation2020). There are three main types of modification techniques, namely, physical, chemical, and enzymatic treatments. Examples of commonly used physical treatment are the Heat Moisture Treatment (HMT) and heating-cooling cycles (Alimi & Workneh, Citation2018; C Zhang et al., Citation2018). Meanwhile, the chemical treatment of starches has been conducted using various chemicals which mainly forms crosslinking and/or substitutions. Commonly used chemicals are octenyl succinic anhydride (OSA), phosphoryl chloride, and acetic anhydride (Singh & Nath, Citation2012; Xiao et al., Citation2018). Additionally, some other chemicals or compounds are also introduced in treatment of starches such as citric acid, lactic acid, ascorbic acid, amino acid, and pectin (Shaikh et al., Citation2019; Galvão et al., Citation2018; Ji, Citation2018; B Zhang et al., Citation2018). The enzymatic treatment on the other hand utilizes amylase, amylosucrase, pullulanase, and many more (Huang et al., Citation2016; Lee et al., Citation2018). Other modification techniques utilize a combination of these three techniques to improve starch properties for example, HMT-amino acid treatment and OSA-pullulanase treatment (Ji & Yu, Citation2018; Chang et al., Citation2019).
Factors considered in choosing a suitable technique for starch treatment include safety issues, characteristics of products, and cost for the modification. Physical treatment is favoured over chemical treatment due to food safety considerations and compliance to regulations. Enzymatic treatment is not a production cost-friendly approach. Examples of physical treatments are heat moisture, multiple freezing-thawing, pre-gelatinization, autoclave, and microwave heat treatments (Akanbi, Kadiri, & Gbadamosi, Citation2019; Alimi & Workneh, Citation2018; Oyeyinka et al., Citation2019; Tao et al., Citation2016; Yousif et al., Citation2012). Among the physical treatment techniques, microwave heat treatment is an energy-efficient method. The irradiation causes the polar and ionic molecules in starch granules to vibrate, causing frictions that generate heat energy (Wang et al., Citation2019). This allows a uniform heating of starch compared to conventional oven heating treatment. Microwave heat treatment had been applied to various starches such as waxy maize, potato, and Bambara groundnut starches (Oyeyinka et al., Citation2019; Xie et al., Citation2013; Yang et al., Citation2017). Microwave irradiation can cause changes in the internal structures and morphology of starch granules Oyeyinka et al., Citation2019. These changes can lead to improved physicochemical and functional properties of native starch.
Sago starch is considered the most abundant starch isolated in Malaysia. Although large quantities of sago starch is produced, its lack of functionality rendered the starch to be underutilized. Modification is necessary to improve its functionality. Unlike other modifications applied on sago starch, there is limited information on the effects of microwave heat treatment on its physicochemical properties. In this study, the sago starch underwent microwave heat treatment with four different treatment durations. The purpose of this study is to enhance the functional properties of sago starch in order to increase its utilization in the food industry.
Materials and Methods
Materials
Food grade sago starch was provided by CRAUN Research Sdn Bhd, Kuching, Sarawak, Malaysia. All chemicals used were analytical reagent grade unless stated otherwise. Amylose from potato was purchased from Sigma-Aldrich (CAS9005-82-7). Potassium bromide powder was purchased from Pike Technologies. Other chemicals used were acquired from HmbG chemicals.
Modification of Sago Starch
Microwave heating treatment of native sago starch was conducted as described by Marta et al. (Citation2019) with slight modifications (Marta et al., Citation2019). The moisture content of the sago starch was adjusted to 30% by the addition of distilled water. The starch was then heated using a microwave oven (ELBA EMO-2505, 900 W) with treatment durations of 5, 10, 15 and 20 (MHT1, MHT2, MHT3, and MHT4) minutes, separately. The microwave heated starches obtained were further oven-dried at 50 °C. The starches were kept in plastic zip-lock bags until further analysis.
Moisture and Ash Content
The moisture content of the starches was determined by oven drying at 105 °C overnight. Meanwhile, the ash content was determined by heating the starches in a muffle furnace at 550 °C for 6 hours.
Apparent Amylose Content
Apparent amylose content in native and modified starches were assessed as described by Shi and Gao (Citation2011). Samples of 100 mg dry starch were mixed with 95 % ethanol with a starch:ethanol ratio of 1:10. The samples were gelatinized by adding 9.2 mL of 1.0 M sodium hydroxide (NaOH) solution and stored at ambient temperature for 20 – 24 hours. After adjusting to 100 mL with distilled water, aliquots of 5 mL of the solution was transferred into 100 mL volumetric flasks. Exactly 1.0 mL of 1 M acetic acid and 2.0 mL of iodine solution (0.2% iodine (I2) in 2% potassium iodide (KI) solution) were added. The volume was made up to 100 mL with distilled water. The absorbance was measured at 620 nm after 20 min with 5 mL 0.09 M NaOH used as a blank, to which acetic acid (1.0 mL) and iodine solution (2.0 mL) were added for a total volume of 100 mL. Amylose from potato was used as standard (Shi & Gao, Citation2011).
Swelling Power and Solubility
The swelling power and solubility were measured by suspending starch in distilled water at a concentration of 2% w/v in water baths at 55, 65, 75, 85, and 95 °C for 1 h, separately (Huang et al., Citation2016). The suspension was cooled to room temperature and centrifuged at 1000g for 15 min. The liquid supernatant was poured out carefully and evaporated overnight at 105 °C. The swollen starch sediment was weighed. Swelling power and solubility were calculated using the following equations:
where SW is the weight of wet sediment, and Starchdry is the weight of dry starch.
Water and Oil Binding Capacity
The water and oil-binding capacities were determined using a method described by Yousif et al. (Citation2012). The determination of water-binding capacity was done by mixing starch in distilled water at a ratio of 1:15 in a weighed centrifuge tube. The tube was agitated on a vortex mixer for 2 min and subsequently centrifuged at 1300g for 20 min. The clear supernatant was decanted and discarded. The adhering water drops were removed. The centrifuge tube with its content was weighed. Water binding capacity (WBC) is expressed as the weight of water in grams bound by 1 g of dried sample.
Meanwhile, for the oil-binding capacity (OBC) determination, a ratio of 1:10 (starch:oil) was mixed in a weighed centrifuge tube. The tube was agitated on a vortex mixer for 2 min and then centrifuged at 1300g for 20 min. The centrifuge tube with its starch content was weighed after excess oil has been removed. Oil binding capacity is expressed as grams of oil bound by 1 g of dried sample (Yousif et al., Citation2012). Soy and palm oils were used for the determination of oil binding capacity.
Syneresis
A starch suspension was prepared by mixing starch with distilled water to a concentration of 2% w/v. The starch suspension was heated at 85 °C for 30 min in a water bath, followed by rapid cooling in an ice-water bath to room temperature. The starch samples were stored for 2, 4, 6, and 8 days at 4 °C, separately. Syneresis was measured as the percentage of water released after centrifugation at 3000g for 15 min (Yousif et al., Citation2012).
Paste Clarity
Paste clarity of the starch suspension samples was determined by measuring light transmittance of starch pastes using the method described in Huang et al. (Citation2016). Starch aqueous suspension of 2% w/v was heated in a boiling water bath with stirring for 1 h. After cooling to room temperature, the light transmittance of the starch paste was determined with a UV-Vis spectrophotometer at 640 nm (Huang et al., Citation2016).
Fourier Transform Infrared Spectroscopy (FTIR)
Fourier transform infrared spectra of native and modified starches were analyzed using the potassium bromide (KBr) method within a spectral range of 4000–650 cm–1,with a resolution of 4 cm–1 and 64 scans (Huang et al., Citation2016).
Scanning Electron Microscopy (SEM)
Samples were secured on a specimen holder using black double-sided tape and then coated with platinum in a vacuum evaporator. Structural properties of the samples were assessed with a scanning electron microscope at 10 kV accelerating voltage (Dura & Rosell, Citation2016).
Statistical Analysis
The experiments were conducted in triplicates and the data were analyzed using Analysis of Variance (ANOVA) followed by post hoc, Tukey’s test in Statistical Package for Social Sciences (IBM® SPSS® Statistics Version 20).
Results and Discussion
Granules morphology for native and modified sago starches
shows the SEM images of native and microwave heated starches at a magnification of 800×. The granules for the native starch showed oval and round-shaped granules which are similar to the findings of previous reports (Othman et al., Citation2015). The granule diameters were between 14.6 and 56.2 µm, with a mean diameter of 35.43 µm. The surface of the native starch granules was smooth. Meanwhile, the microwave heated starches had irregularly shaped granules with diameters ranging from 8.5 to 55.4 µm. The irregular shaped granules are similar to those of microwaved Bambara groundnut starch (Oyeyinka et al., Citation2019). The average diameters for MHT1, MHT2, MHT3, and MHT4 were 35.87, 31.60, 32.56, and 32.48 µm, respectively, with no significant differences from granules of native starch. The slight reduction in the diameter of the granules was possibly due to the rupture of the original granules. Microwave treatment disrupts the crystalline region of starch granules by causing water molecules to collide and produces friction, which results in high heat energy within the granule structure (Wang et al., Citation2019; Xie et al., Citation2013). This suggests that the high molecular weight amylopectin and/or amylose undergoes bond breakage, forming shorter amylopectin and/or amylose (Xie et al., Citation2013). The range for the diameter of modified starches was similar with those of gamma-irradiated sago starch reported by Othman et al. (Citation2015). Pinholes, fissures, and fractures were observed on all microwave heated starch granule surfaces. There was evidence that the granule for MHT4 starts to lose its granule integrity in the presence of partially gelatinized granules. This indicated that prolonged microwave heat treatment caused more disruption to the granule structure.
Figure 1. Scanning electron microscopy images of starch granules; (a) native sago starch, (b) microwave heated sago starch (5 min treatment) (MHT1), (c) microwave heated sago starch (10 min treatment) (MHT2), (d) microwave heated sago starch (15 min treatment) (MHT3), and (e) microwave heated sago starch (20 min treatment) (MHT4) at 800× magnification.
Figura 1. Imágenes de microscopía electrónica de barrido de gránulos de almidón; (a) almidón de sagú nativo, (b) almidón de sagú calentado por microondas (tratamiento de 5 minutos) (MHT1), (c) almidón de sagú calentado por microondas (tratamiento de 10 minutos) (MHT2), (d) almidón de sagú calentado por microondas (tratamiento de 15 minutos) (MHT3), y (e) almidón de sagú calentado por microondas (tratamiento de 20 minutos) (MHT4) a 800× aumentos
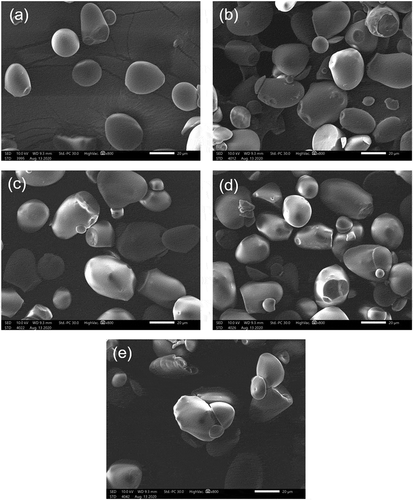
Fourier Transform Infrared Spectroscopy (FTIR)
There were no new or missing peaks observed from microwave-heated starches when compared to the native starch (). However, there were differences in their absorbance strength for each peak. The broad peak at 3000–3600 cm–1 represents the –OH group vibrational stretches. The broad peak for the microwave heated starch showed higher intensity compared to the native starch. This is possibly due to the formation of short amylose chains which increases the number of hydroxyl groups (Ratnaningsih et al., Citation2019). A C–H stretching vibration was observed at 2932–2933 cm–1. The peak at 926 cm–1 was recorded for the stretching of C–O bonds in C–O–C, indicating the vibration of α-1,4 glycosidic bonding. Meanwhile, a sharp peak at 1649 cm–1 was observed, related to the water bending in the amorphous region of starch granules. Apart from that, a peak at 1006 cm–1 indicating the intramolecular hydrogen bonding in the granules was detected for all starch samples.
Figure 2. The Fourier Transform Infrared spectra for (a) native sago starch, (b) microwave heated sago starch (5 min treatment) (MHT1), (c) microwave heated sago starch (10 min treatment) (MHT2), (d) microwave heated sago starch (15 min treatment) (MHT3), and (e) microwave heated sago starch (20 min treatment) (MHT4).
Figura 2. Espectros infrarrojos por transformada de Fourier para (a) almidón de sagú nativo, (b) almidón de sagú calentado por microondas (tratamiento de 5 minutos) (MHT1), (c) almidón de sagú calentado por microondas (tratamiento de 10 minutos) (MHT2), (d) almidón de sagú calentado por microondas (tratamiento de 15 minutos) (MHT3), y (e) almidón de sagú calentado por microondas (tratamiento de 20 minutos) (MHT4)
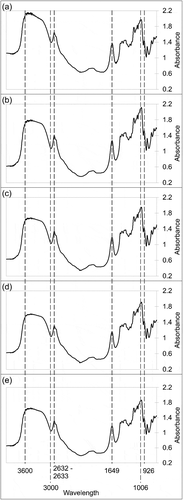
The internal changes in the degree of order and degree of double helix can be evaluated using ratios of 1047/1023 and 995/1023, respectively (Zhou et al., Citation2019). The 1047/1023 ratios for native, MHT1, MHT2, MHT3, and MHT4 were 0.965, 0.962, 0.964, 0.964, and 0.965, respectively. The degree of order for MHT1, MHT2, and MHT3 were lower compared to that of the native, as previously reported for microwaved pea starch (Zhou et al., Citation2019). This implied a less compact packing density of the double helixes upon reorientation on the starch granule surface (Zhou et al., Citation2019). However, MHT4 had the same degree of order with native starch which may be due to reorganization of the amylose and amylopectin in the granule structure. Meanwhile, the 995/1023 ratios for native, MHT1, MHT2, MHT3, and MHT4 were 1.014, 1.023, 1.030, 1.029, and 1.023, respectively. This suggested the modified starch had a higher degree of double helix (Zhou et al., Citation2019).
Moisture and Ash Content
The moisture and ash contents of native and modified sago starches are shown in . Based on the result, all microwave treated starch showed significantly lower moisture content than native sago starch. However, the modified sago starch did not show statistical differences with each other, where their moisture content were within 11.18–11.86 %. The native starch moisture content was similar to the findings of previous work by Othman et al. (Citation2015) (Othman et al., Citation2015). However, another report on the moisture content of sago starch gave a value of 8.5 %, which was lower than the one obtained in this study (Azfaralariff et al., Citation2020). The lower moisture content of modified starches might be due to the overnight drying. The low moisture content would help in preventing fungal or bacterial growth, which would further improve the starch shelf-life.
Table 1. The moisture, ash, and amylose contents for the native and modified starches
Tabla 1. Contenido de humedad, cenizas y amilosa de los almidones nativos y los modificados
Meanwhile, there was no significant difference in ash content for microwave treated starches, although slight changes were observed for MHT2, MHT3, and MHT4 when compared to native starch. The highest content was recorded for MHT4 at 0.15 %, while the lowest was for MHT2 and MHT3, both at 0.12 %. This was higher than the ash content recorded in previous work by Azfaralariff et al. (Citation2020) which was 0.04 %, but lower than the results of Liestianty et al. (Citation2016) (Azfaralariff et al., Citation2020; Liestianty et al., Citation2016). Ash content is linked to the availability of minerals in the sample (Liestianty et al., Citation2016). Another factor contributing towards ash content is the origin of the starch. The starch used in this study originated from Sarawak, Malaysia while the starch used by Liestianty et al. (Citation2016) was from Northern Maluku, Indonesia.
Apparent Amylose Content
Generally, the amylose content of modified starches increases as the treatment duration increases. The MHT4 and MHT1 showed a slightly higher amylose content with percentages of 35.37 and 33.69 % () respectively than native starch. However, based on statistical analysis, no significant difference was observed in the percentage of amylose content between native and treated starches. The amylose content of native starch in this study was slightly higher compared to previously published values (Othman et al., Citation2015). This may be due to the different growth stages of the sago palm used before being processed to yield starch (Uthumporn et al., Citation2014). Another possible reason is the extraction method used wherein treatment was conducted during the isolation process. In a previous study on gamma-irradiated sago starch, the post-treatment amylose content of the starch was decreased (Othman et al., Citation2015). The increase in amylose content for the modified starches were caused by microwave heating treatment. Microwave-heating treatment was reported to break the α-(1,6) glycosidic linkage more effectively than the α-(1,4) glycosidic linkage (Yang et al., Citation2017). This suggested the possible breakdown of amylopectin side chains in the starch, forming shorter and less branched amylopectin and/or amylose chains. This was reflected by the increase in the percentage of amylose content. The increase of amylose content was previously reported to be in line with the increase in the resistant starch amount (Shi & Gao, Citation2011). Resistant starch is a starch that resist digestion by human upper digestion tract which reduces the food glycaemic response.
Swelling Power and Solubility
shows the swelling power of native and microwave treated starches. Although there was a decrease in swelling power at a temperature of 85 °C, the swelling power of the starches generally increases as the temperature increases. A drastic increase in swelling power was observed between 65 and 75 °C, similar to the previous report on microwaved Bambara groundnut starch (Oyeyinka et al., Citation2019). Generally, all modified starch at all tested temperatures showed higher swelling power than native sago starch. The swelling power of modified starches was significantly higher compared to native starch at temperatures of 65 and 95 °C. This is aligned with a report by Zhou et al. (Citation2019) on microwaved pea starch except for 95 °C (Zhou et al., Citation2019), but contradicts with the gamma-irradiated sago starch findings reported by Othman et al. (Citation2015) (Othman et al., Citation2015). However, no significant differences were observed among the modified starches at these temperatures. Meanwhile, at temperatures of 75 and 85 °C, there was a significant difference between modified starches and native starch, except for MHT2. No pattern was observed as the treatment duration increases. This was different from microwaved Bambara groundnut starch where its swelling power reduces as the treatment duration increases (Oyeyinka et al., Citation2019). The amount of amylopectin normally influences the swelling power of starches. Generally, when the amount of amylopectin decreases, the swelling power will be reduced (Othman et al., Citation2015). Additionally, the amorphous region state and high hydrophilicity of the starches may influence the swelling power of the starches (Włodarczyk-Stasiak et al., Citation2019). In addition, swelling power was negatively correlated to the degree of double helix. This was also supported by the higher water-binding capacity of the microwaved starches compared to native starch where there was a positive correlation between water-binding capacity and swelling power. Higher water-binding capacity indicates higher availability of hydroxyl groups for hydrogen bonding between starch and water molecules, which contributed to the higher hydrophilicity of starch.
Figure 3. The (a) swelling power and (b) percentage solubility for the native and modified starches at various temperatures; error bars represent the standard deviation for each sample (NS-native sago starch; MHT1-microwave heated sago starch (5 min treatment); MHT2-microwave heated sago starch (10 min treatment); MHT3-microwave heated sago starch (15 min treatment); MHT4-microwave heated sago starch (20 min treatment)).
Figura 3. (a) Poder de hinchamiento y (b) porcentaje de solubilidad de los almidones nativos y los modificados a distintas temperaturas; las barras de error representan la desviación estándar de cada muestra [almidón de sagú nativo NS; almidón de sagú calentado por microondas MHT1 (tratamiento de 5 minutos); almidón de sagú calentado por microondas MHT2 (tratamiento de 10 minutos); almidón de sagú calentado por microondas MHT3 (tratamiento de 15 minutos); almidón de sagú calentado por microondas MHT4 (tratamiento de 20 minutos)]
![Figure 3. The (a) swelling power and (b) percentage solubility for the native and modified starches at various temperatures; error bars represent the standard deviation for each sample (NS-native sago starch; MHT1-microwave heated sago starch (5 min treatment); MHT2-microwave heated sago starch (10 min treatment); MHT3-microwave heated sago starch (15 min treatment); MHT4-microwave heated sago starch (20 min treatment)).Figura 3. (a) Poder de hinchamiento y (b) porcentaje de solubilidad de los almidones nativos y los modificados a distintas temperaturas; las barras de error representan la desviación estándar de cada muestra [almidón de sagú nativo NS; almidón de sagú calentado por microondas MHT1 (tratamiento de 5 minutos); almidón de sagú calentado por microondas MHT2 (tratamiento de 10 minutos); almidón de sagú calentado por microondas MHT3 (tratamiento de 15 minutos); almidón de sagú calentado por microondas MHT4 (tratamiento de 20 minutos)]](/cms/asset/32cc94d7-097f-4d11-a1ad-2bf9eceb73fb/tcyt_a_1934550_f0003_b.gif)
Based on , the percentage of solubility showed a general increase in the solubility of tested starches as the temperature increases, similar to the findings of a report on modified lotus seed starch (Zeng et al., Citation2015). All starches showed their highest solubility at 95 °C. It was observed that at temperatures of 95 °C, native starch had the highest solubility with a value of 8.67 %. However, there were no significant differences between all tested starches at temperatures of 65 °C up to 85 °C. At 95 °C, the microwave heated starches showed a significant decrease in their solubility compared to native starch. Yet, there was a difference among the modified starches where MHT1 solubility was significantly different from MHT3. The increase in the solubility of starch as temperature increases is associated with the destruction of crystal structure at high heat energy (Zeng et al., Citation2015).
Starch swelling power and solubility are normally related to the amount of amylose, amylopectin leached, and the hydrophilic properties of these components (Othman et al., Citation2015; Xiao et al., Citation2018). In this study however, the increase in amylose content for MHT1 and MHT4 does not significantly shows an increase in solubility. The modified starch may undergo reorientation in their granule which prevents leaching. The increase in the degree of double helices may have restricted the leaching of amylose. The double helix amylose will use their hydroxyl group to attract and form hydrogen bonds between its molecules. This will reduce the hydrophilic properties of amylose and subsequently reduce the swelling power and solubility of the starch. Meanwhile, the low swelling power and solubility at 65°C might be due to the amorphous region in which the starch granules were not gelatinized at low temperature (Zeng et al., Citation2015). The reported gelatinization temperature for native sago starch was between 69.4 and 70.1ºC (Okazaki, Citation2018).
Water and Oil Binding Capacities
There was an increasing pattern in the water-binding capacity as treatment durations increase (). However, there were no observed statistical differences between the modified starches and native starch. The increase in water-binding capacity was observed for heat moisture treated iburu starch (Alimi & Workneh, Citation2018). The water absorption is related to the amylopectin content of starch as the increase in amylopectin will cause lower water absorption (Alimi & Workneh, Citation2018). Increase in amylose normally increases the water-binding capacity of starch (Li et al., Citation2020). However, based on , the increase in amylose did not seem to influence the water-binding capacity of starch. This was probably due to the effect of the double helix structures in the starch where the water-binding capacity of the starches had a negative correlation with the degree of double helix (r = -0.997, p < 0.05). Increase in the formation of double helix structure will reduce the available binding sites for water molecules, hence reducing the water-binding capacity. The water-binding capacity of modified starches is significantly higher than those of soy and palm oil, indicating enhanced hydrophilicity in the modified starches.
Table 2. Percentage of water, soy oil and palm oil binding capacities of native and modified starches
Tabla 2. Porcentaje de capacidad de unión de agua, aceite de soya y aceite de palma de los almidones nativos y los modificados
Meanwhile, modified starches showed a decrease in oil-binding capacity with palm oil. However, there was no noticeable pattern for soy oil. Some differences were displayed between soy oil and palm oil-binding capacities; palm oil showed lower oil-binding capacity compared to soy oil. This slight difference may be due to the composition of the respective oils such as the quantity of polysaturated, monosaturated, and saturated fatty acids in the oils. The length of the fatty acids may also influence binding capacities. Oil binding capacity is linked to physical entrapment of oil by capillary attraction which is related to presence of pores (Sirivongpaisal, Citation2008). Although the modified starch have more pores than those of native, the size of the pores might be to large causing reduction on the capillary attraction, whilst reduces the oil-binding capacities of modified starches (Jung et al., Citation2017).
Syneresis
The syneresis of native and microwave heated starches is shown in . Based on the figure, native starch, MHT3, and MHT4 displayed a general increase in syneresis as storage duration increases. This is different from MHT1 and MHT2 where the syneresis decreased at day 8. There was no significant difference for the percentage of syneresis on day 2. For day 4, MHT1 showed the lowest syneresis value, which was also significantly lower compared to other tested starches. Meanwhile, the percentage of syneresis of microwave heated starches was significantly higher compared to native starch on day 6. On day 8 of storage, there was no significant difference between microwave heated starches with native starch, except for MHT4 where it had the highest percentage of water loss. Syneresis of gels is related to the freeze-thaw stability of the gels. It is unknown whether amylose content influences syneresis. Based on many studies, contradictory results were observed on the influence of high amylose content on the syneresis of starch gels (Srichuwong et al., Citation2012). Contradicting results were also observed for the effect of amylopectin chain. Jobling et al. (Citation2002) reported the short-chain amylopectin in starch can reduce the syneresis of starch gels (Jobling et al., Citation2002). However, this is different from a report by Y Zhang et al. (Citation2019) where a lower degree of polymerization of amylopectin yielded the highest syneresis when compared to a higher polymerization degree (Y Zhang et al., Citation2019). The microstructure of starch may influence the stability of syneresis. Y Zhang et al. (Citation2019) reported the formation of loose structure for low syneresis gel while a compact structure is seen for a higher syneresis gel (Y Zhang et al., Citation2019). Moreover, the origin of starch also plays a large role in the syneresis of starch gels (Srichuwong et al., Citation2012). High syneresis will reduce the utilisation of starch in frozen food products such as yogurt. It is unfavourable to have food products with high syneresis as it reduces the texture, taste, and quality of the food product.
Figure 4. Percentage of water loss from gels of native and microwave heated starches; error bars represent the standard deviation for each sample (NS-native sago starch; MHT1-microwave heated sago starch (5 min treatment); MHT2-microwave heated sago starch (10 min treatment); MHT3-microwave heated sago starch (15 min treatment); MHT4-microwave heated sago starch (20 min treatment)).
Figura 4. Porcentaje de pérdida de agua de los geles de almidones nativos y los calentados por microondas; las barras de error representan la desviación estándar de cada muestra [almidón de sagú nativo NS; almidón de sagú calentado por microondas MHT1 (tratamiento de 5 minutos); almidón de sagú calentado por microondas MHT2 (tratamiento de 10 minutos); almidón de sagú calentado por microondas MHT3 (tratamiento de 15 minutos); almidón de sagú calentado por microondas MHT4 (tratamiento de 20 minutos)]
![Figure 4. Percentage of water loss from gels of native and microwave heated starches; error bars represent the standard deviation for each sample (NS-native sago starch; MHT1-microwave heated sago starch (5 min treatment); MHT2-microwave heated sago starch (10 min treatment); MHT3-microwave heated sago starch (15 min treatment); MHT4-microwave heated sago starch (20 min treatment)).Figura 4. Porcentaje de pérdida de agua de los geles de almidones nativos y los calentados por microondas; las barras de error representan la desviación estándar de cada muestra [almidón de sagú nativo NS; almidón de sagú calentado por microondas MHT1 (tratamiento de 5 minutos); almidón de sagú calentado por microondas MHT2 (tratamiento de 10 minutos); almidón de sagú calentado por microondas MHT3 (tratamiento de 15 minutos); almidón de sagú calentado por microondas MHT4 (tratamiento de 20 minutos)]](/cms/asset/67917a93-8214-4479-9f04-96944c38ced5/tcyt_a_1934550_f0004_b.gif)
Paste Clarity
The paste clarity of native and microwave-heated starches is shown in . The modified starches had a significant decrease in their paste clarity compared to native starch. This may be due to the lower solubility of the modified starch as shown in . A pattern was observed where the percentage of transmittance decreases as the duration of microwave heat treatment increases. Sago starch paste clarity was lower than those of potato and tapioca starches, but higher than those of maize, wheat, and rice starches (Hsieh et al., Citation2019). Paste clarity is essential to produce clear beverages and coloured food products. The lowering of paste clarity means that these modified starches are not suitable for food products that require clarity such as fibre drinks. The reduction in paste clarity may be a result of the retrogradation process when the gels cooled (Lima et al., Citation2020). Retrogradation will influence turbidity, causing a lowering in percentage of transmittance for the starch paste (Lima et al., Citation2020). The pH of the solution during treatment can affect the clarity as a higher pH results in a decrease of transmittance, especially in the chemical treatment of starch (Muhammad et al., Citation2000). The current study also observed the increase in pH values of modified starches (pH 8.33 – 8.48) when compared to the native starch (pH 8.18). The presence of electronegative groups and reducing sugar content also affects paste clarity (Lima et al., Citation2020).
Figure 5. Percentage transmittance for the paste clarity of native and microwave heated starches; different letter after the mean value indicate the sample belongs to a different group (p < 0.05); error bars represent the standard deviation for each sample (NS-native sago starch; MHT1-microwave heated sago starch (5 min treatment); MHT2-microwave heated sago starch (10 min treatment); MHT3-microwave heated sago starch (15 min treatment); MHT4-microwave heated sago starch (20 min treatment)).
Figura 5. Porcentaje de transmitancia para la claridad de la pasta de los almidones nativos y los calentados por microondas; una letra diferente después del valor medio indica que la muestra pertenece a un grupo diferente (p < 0.05); las barras de error representan la desviación estándar de cada muestra [NS-almidón de sagú nativo; MHT1-almidón de sagú calentado por microondas (5 min de tratamiento); MHT2-almidón de sagú calentado por microondas (10 min de tratamiento); MHT3-almidón de sagú calentado por microondas (15 min de tratamiento); MHT4-almidón de sagú calentado por microondas (20 min de tratamiento)]
![Figure 5. Percentage transmittance for the paste clarity of native and microwave heated starches; different letter after the mean value indicate the sample belongs to a different group (p < 0.05); error bars represent the standard deviation for each sample (NS-native sago starch; MHT1-microwave heated sago starch (5 min treatment); MHT2-microwave heated sago starch (10 min treatment); MHT3-microwave heated sago starch (15 min treatment); MHT4-microwave heated sago starch (20 min treatment)).Figura 5. Porcentaje de transmitancia para la claridad de la pasta de los almidones nativos y los calentados por microondas; una letra diferente después del valor medio indica que la muestra pertenece a un grupo diferente (p < 0.05); las barras de error representan la desviación estándar de cada muestra [NS-almidón de sagú nativo; MHT1-almidón de sagú calentado por microondas (5 min de tratamiento); MHT2-almidón de sagú calentado por microondas (10 min de tratamiento); MHT3-almidón de sagú calentado por microondas (15 min de tratamiento); MHT4-almidón de sagú calentado por microondas (20 min de tratamiento)]](/cms/asset/8bdb215e-35a5-4882-8233-bf0eb5afa3ec/tcyt_a_1934550_f0005_b.gif)
Conclusion
The physicochemical properties of sago starch were affected by the microwave heat treatment. The increase in treatment duration caused an increase in irregularities of starch granule shape, resulting in appearances of fissures and ruptures. The duration of treatment also affects other physicochemical properties where there is an increase in swelling power, water-binding capacity, amylose content and syneresis. Additionally, there is a decrease in solubility at 95 °C, oil-binding capacity and paste clarity. Negative correlation between the degree of double helix with swelling power, water-binding capacity and oil-binding capacity was also observed. A positive correlation with paste clarity indicates the importance of double helix structure towards the properties of starch. Future study may be required to understand the formation of double helix structure in the microwaved starch and its effects on food structure.
Conflict of interest
There is no known conflict of interest.
Acknowledgement
The authors would like to thank the Sarawak Research and Development Council for the financial support through a research grant (RDCRG/CAT/2019/26). The authors acknowledged the Ministry for Higher Education Malaysia and Universiti Malaysia Sarawak for the financial support for a PhD candidate. The authors also thank CRAUN Research Sdn Bhd for providing the sago starch used in this study.
Additional information
Funding
References
- Abiddin, N. F., Yusoff, A., & Ahmad, N. (2018). Effect of octenylsuccinylation on physicochemical, thermal, morphological and stability of octenyl succinic anhydride (OSA) modified sago starch. Food Hydrocolloids, 75(February), 138–146. doi:https://doi.org/10.1016/j.foodhyd.2017.09.003
- Akanbi, C. T., Kadiri, O., & Gbadamosi, S. O. (2019). Kinetics of starch digestion in native and modified sweetpotato starches from an orange fleshed cultivar. International Journal of Biological Macromolecules, 134(August), 946–953. doi:https://doi.org/10.1016/j.ijbiomac.2019.05.035
- Alimi, B. A., & Workneh, T. S. (2018). Structural and physicochemical properties of heat moisture treated and citric acid modified acha and iburu starches. Food Hydrocolloids, 81(August), 449–455. doi:https://doi.org/10.1016/j.foodhyd.2018.03.027
- Azfaralariff, A., Fazial, F. F., Sontanosamy, R. S., Nazar, M. F., & Lazim, A. M. (2020). Food-grade particle stabilized pickering emulsion using modified sago (Metroxylon sagu) starch nanocrystal. Journal of Food Engineering, 280(September), 109974. doi:https://doi.org/10.1016/j.jfoodeng.2020.109974
- Chang, R., Xiong, L., Li, M., Chen, H., Xiao, J., Wang, S., Qiu, L., Bian, X., Sun, C., & Sun, Q. (2019). Preparation of octenyl succinic anhydride-modified debranched starch vesicles for loading of hydrophilic functional ingredients. Food Hydrocolloids, 94(September), 546–552. doi:https://doi.org/10.1016/j.foodhyd.2019.04.006
- Dura, A., & Rosell, C. M. (2016). Physico-chemical properties of corn starch modified with cyclodextrin glycosyltransferase. International Journal of Biological Macromolecules, 87(June), 466–472. doi:https://doi.org/10.1016/j.ijbiomac.2016.03.012
- Galvão, A. M., Zambelli, R. A., Araújo, A. W., & Bastos, M. S. (2018). Edible coating based on modified corn starch/tomato powder: Effect on the quality of dough bread. LWT - Food Science and Technology, 89(March), 518–524. doi:https://doi.org/10.1016/j.lwt.2017.11.027
- Hsieh, C. F., Liu, W., Whaley, J. K., & Shi, Y. C. (2019). Structure and functional properties of waxy starches. Food Hydrocolloids, 94(September), 238–254. doi:https://doi.org/10.1016/j.foodhyd.2019.03.026
- Huang, H., Jiang, Q., Chen, Y., Li, X., Mao, X., Chen, X., Huang, L., & Gao, W. (2016). Preparation, physico–chemical characterization and biological activities of two modified starches from yam (Dioscorea Opposita Thunb.). Food Hydrocolloids, 55(April), 244–253. doi:https://doi.org/10.1016/j.foodhyd.2015.11.016
- Ji, Y. (2018). In vitro digestion and physicochemical characteristics of corn starch mixed with amino acid modified by low pressure treatment. Food Chemistry, 242(March), 421–426. doi:https://doi.org/10.1016/j.foodchem.2017.09.073
- Ji, Y., & Yu, J. (2018). In vitro digestion and physicochemical characteristics of corn starch mixed with amino acid modified by heat-moisture treatment. Food Hydrocolloids, 77(April), 720–725. doi:https://doi.org/10.1016/j.foodhyd.2017.11.013
- Jobling, S., Westcott, R., Tayal, A., Jeffcoat, R., & Schwall, G. (2002). Production of a freeze–thaw-stable potato starch by antisense inhibition of three starch synthase genes. Nature Biotechnology, 20(3), 295–299. doi:https://doi.org/10.1038/nbt0302-295
- Jung, Y.-S., Lee, B.-H., Yoo, S.-H., & Gomez-Casati, D. F. (2017). Physical structure and absorption properties of tailor-made porous starch granules produced by selected amylolytic enzymes. Plos One, 12(7), 0181372. doi:https://doi.org/10.1371/journal.pone.0181372
- Lee, E. S., Lee, B. H., Shin, D. U., Lim, M. Y., Chung, W. H., Park, C. S., Baik, M. Y., Nam, Y. D., & Seo, D. H. (2018). Amelioration of obesity in high-fat diet-fed mice by chestnut starch modified by amylosucrase from Deinococcus geothermalis. Food Hydrocolloids, 75(February), 22–32. doi:https://doi.org/10.1016/j.foodhyd.2017.09.019
- Li, C., Dhital, S., Gilbert, R. G., & Gidley, M. J. (2020). High-amylose wheat starch: Structural basis for water absorption and pasting properties. Carbohydrate Polymers, 245(October), 116557. doi:https://doi.org/10.1016/j.carbpol.2020.116557
- Liestianty, D., Rodianawati, I., & Patimah, M. (2016). Chemical composition of modified and fortified sago starch (Metroxylon sp) from Northern Maluku. International Journal of Applied Chemistry, 12(3)243–249. http://www.ripublication.com/ijac16/ijacv12n3_07.pdf
- Lima, D. C., Villar, J., Castanha, N., Maniglia, B. C., Junior, M. D., & Augusto, P. E. (2020). Ozone modification of arracacha starch: Effect on structure and functional properties. Food Hydrocolloids, 108(November), 106066. doi:https://doi.org/10.1016/j.foodhyd.2020.106066
- Marta, H., Cahyana, T., Arifin, H. R., & Khairani, L. (2019). Comparing the effect of four different thermal modification on physicochemical and pasting properties of breadfruit (Artocarpus altilis) starch. International Food Research Journal, 26(1), 269–276. http://www.ifrj.upm.edu.my/26%20(01)%202019/(30).pdf
- Muhammad, K., Hussin, F., Man, Y. C., Ghazali, H. M., & Kennedy, J. F. (2000). Effect of pH on phosphorylation of sago starch. Carbohydrate Polymers, 42(1), 85–90. doi:https://doi.org/10.1016/S0144-8617(99)00120-4
- Okazaki, M. (2018). The structure and characteristics of sago starch. In E. H., T. Y & J. D (Eds.), Sago Palm(pp. 247–259). Springer. doi:https://doi.org/10.1007/978-981-10-5269-9_18
- Oladzadabbasabadi, N., Ebadi, S., Nafchi, A. M., Karim, A., & Kiahosseini, S. R. (2017). Functional properties of dually modified sago starch/ĸ-carrageenan films: An alternative to gelatin in pharmaceutical capsules. Carbohydrate Polymers, 160(December), 43–51. doi:https://doi.org/10.1016/j.carbpol.2016.12.042
- Othman, Z., Hassan, O., & Hashim, K. (2015). Physicochemical and thermal properties of gamma-irradiated sago (Metroxylon sagu) starch. Radiation Physics and Chemistry, 109(April), 48–53. doi:https://doi.org/10.1016/j.radphyschem.2014.12.003
- Oyeyinka, S. A., Umaru, E., Olatunde, S. J., & Joseph, J. K. (2019). Effect of short microwave heating time in the physicochemical and functional properties of Bambara groundnut starch. Food Bioscience, 28(April), 36–41. doi:https://doi.org/10.1016/j.fbio.2019.01.005
- Ratnaningsih, N., Suparmo, H. E., & Marsono, Y. (2019). Physicochemical properties, invitro starch digestibility, and estimated glycemic index of resistant starch from cowpea (Vigna unguiculata) starch by autoclaving-cooling cycle. International Journal of Biological Macromolecules, 142(January), 191–200. doi:https://doi.org/10.1016/j.ijbiomac.2019.09.092
- Shaikh, F., Ali, T. M., Mustafa, G., & Hasnain, A. (2019). Comprative study on effects of citric and lactic acid treatment on morphological, functional, resistance starch fraction and glycemic index of corn and sorghum. International Journal of Biological Macromolecules, 135(August), 314–327. doi:https://doi.org/10.1016/j.ijbiomac.2019.05.115
- Shi, M. M., & Gao, Q. Y. (2011). Physicochemical properties, structure and in vitro digestion of resistant starch from waxy rice starch. Carbohydrate Polymers, 84(3), 1151–1157. doi:https://doi.org/10.1016/j.carbpol.2011.01.004
- Singh, A. V., & Nath, L. K. (2012). Synthesis and evaluation of physicochemical properties of cross-linked sago starch. International Journal of Biological Macromolecules, 50(1), 14–18. doi:https://doi.org/10.1016/j.ijbiomac.2011.09.003
- Sirivongpaisal, P. (2008). Structure and functional properties of starch and flour from bambarra groundnut. Songklanakarin Journal of Science and Technology, 30(1), 51–56. http://rdo.psu.ac.th/sjstweb/journal/30-Suppl-1/0125-3395-30-S1-51-56.pdf
- Srichuwong, S., Isono, N., Jiang, H., Mishima, T., & Hisamatsu, M. (2012). Freeze-thaw stability of starches from different botanical sources: Correlation with structural features. Carbohydrate Polymers, 87(2), 1275–1279. doi:https://doi.org/10.1016/j.carbpol.2011.09.004
- Tao, H., Zhang, B., Wu, F., Jin, Z., & Xu, X. (2016). Effect of multiple freezing/thawing-modified wheat starch on dough properties and bread quality using a reconstitution system. Journal of Cereal Science, 69(May), 132–137. doi:https://doi.org/10.1016/j.jcs.2016.03.001
- Uthumporn, U., Wahidah, N., & Karim, A. (2014). Physicochemical properties of starch from sago (Metroxylon sagu) palm grown in mineral soil at different growth stages. IOP Conference Series: Material Science and Engineering, 62, 1–11. doi:https://doi.org/10.1088/1757-899X/62/1/012026
- Wang, M., Sun, M., Zhang, Y., Chen, Y., Wu, Y., & Ouyang, J. (2019). Effect of microwave irradiation-retrogradation treatment on the digestive and physicochemical properties of starches with different crystallinity. Food Chemistry, 298(November), 125015. doi:https://doi.org/10.1016/j.foodchem.2019.125015
- Włodarczyk-Stasiak, M., Mazurek, A., Jamroz, J., Pikus, S., & Kowalski, R. (2019). Physicochemical properties and structure of hydrothermally modified starches. Food Hydrocolloids, 95(October), 88–97. doi:https://doi.org/10.1016/j.foodhyd.2019.04.024
- Xiao, L., Chen, J., Wang, X., Bai, R., Chen, D., & Liu, J. (2018). Structural and physicochemical properties of chemically modified Chinese water chestnut [Eleocharis dulcis (Burm. f.) Trin. ex Hensch] starches. International Journal of Biological Macromolecules, 120(December), 547–556. doi:https://doi.org/10.1016/j.ijbiomac.2018.08.161
- Xie, Y., Yan, M., Yuan, S., Sun, S., & Huo, Q. (2013). Effect of microwave treatment on the physicochemical properties of potato starch granules. Chemistry Central Journal, 7(113), 1–7. doi:https://doi.org/10.1186/1752-153X-7-113
- Yang, Q., Qi, L., Luo, Z., Kong, X., Xiao, Z., Wang, P., & Peng, X. (2017). Effect of microwave irradiation on internal molecular structure and physical properties of waxy maize starch. Food Hydrocolloids, 69(August), 473–482. doi:https://doi.org/10.1016/j.foodhyd.2017.03.011
- Ying, B. Z., Kamilah, H., Karim, A. A., & Utra, U. (2020). Effects of heat-moisture and alkali treatment on the enzymatic hydrolysis of porous sago (Metroxylon sagu) starch. Journal of Food Processing and Preservation, 44(5), 1–12. doi:https://doi.org/10.1111/jfpp.14419
- Yousif, E., Gadallah, M., & Sorour, A. M. (2012). Physico-chemical and rheological properties of modified corn starched and its effect on noodle quality. Annal of Agricultural Science, 57(1), 19–27. doi:https://doi.org/10.1016/j.aoas.2012.03.008
- Zeng, S., Wu, X., Lin, S., Zeng, H., Lu, X., Zhang, Y., & Zheng, B. (2015). Structural characteristics and physicochemical properties of lotus seed resistant starch prepared by different methods. Food Chemistry, 186(November), 213–222. doi:https://doi.org/10.1016/j.foodchem.2015.03.143
- Zhang, B., Bai, B., Pan, Y., Li, X. M., Cheng, J. S., & Chen, H. Q. (2018). Effects of pectin with different molecular weight on gelatinization behavior, textural properties, retrogradation and in vitro digestibility of corn starch. Food Chemistry, 264(October), 58–63. doi:https://doi.org/10.1016/j.foodchem.2018.05.011
- Zhang, C., Han, J. A., & Lim, S. T. (2018). Characteristics of some physically modified starches using mild heating and freeze-thawing. Food Hydrocolloids, 77(April), 894–901. doi:https://doi.org/10.1016/j.foodhyd.2017.11.035
- Zhang, Y., Li, B., Zhang, Y., Xu, F., Zhu, K., Li, S., & Dong, W. (2019). Effect of degree of polymerization of amylopectin on the gelatinization properties of jackfruit seed starch. Food Chemistry, 289(August), 152–159. doi:https://doi.org/10.1016/j.foodchem.2019.03.033
- Zhou, D., Ma, Z., Yin, X., Hu, X., & Boye, J. I. (2019). Structural characteristics and physicochemical properties of field pea starch modified by physical, enzymatic, and acid treatments. Food Hydrocolloids, 93(August), 386–394. doi:https://doi.org/10.1016/j.foodhyd.2019.02.048