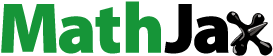
ABSTRACT
Both the magnetic field treatment and the microwave treatment can affect the concentration of aroma compounds in liquor, but there are few researches on the composite treatment that combining the two. In this study, the effects of separate pulsed magnetic field (PMF), microwave field, and PMF-microwave composite treatment on Chinese soy-sauce flavor liquor were investigated. The concentration changes of various aroma compounds in liquor were analyzed by gas chromatograph, and the change of water-ethanol association structure in liquor was analyzed by proton nuclear magnetic resonance spectroscopy. Sensory evaluation of liquor subjected to different processes was carried out. The results showed that the sensory characteristic of liquor after composite treatment was improved, and the change of aroma compounds is close to that in traditional aging. The PMF treatment can significantly improve the taste of liquor. The results prove that the PMF-microwave composite treatment is a potential artificial aging method.
Graphical abstract
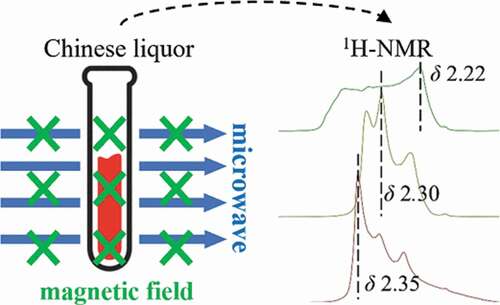
RESUMEN
Tanto el tratamiento con campo magnético como el tratamiento con microondas pueden afectar la concentración de los compuestos aromáticos del licor. Sin embargo, existen pocas investigaciones sobre el tratamiento que combina ambos procesos. En este estudio se investigaron los efectos del campo magnético pulsado (PMF) y del campo de microondas por separado, así como del tratamiento combinado de PMF y microondas en el licor de sabor a salsa de soya china. Para ello se analizaron los cambios de concentración de varios compuestos aromáticos del licor mediante cromatografía de gases, mientras que el cambio de la estructura de asociación agua-etanol en el licor se analizó empleando espectroscopia de resonancia magnética nuclear de protones. Posteriormente, se llevó a cabo una evaluación sensorial del licor sometido a diferentes procesos. Los resultados de dicha evaluación dieron cuenta de que, tras el tratamiento combinado, la característica sensorial del licor mejoró y el cambio de los compuestos aromáticos se acercó al del añejamiento tradicional. En este sentido se comprobó que el tratamiento con PMF puede mejorar significativamente el sabor del licor. Los resultados permiten concluir que el tratamiento combinado de PMF y microondas constituye un potencial método de añejamiento artificial.
1. Introduction
Chinese liquor, also known as Baijiu, is one of the oldest distilled spirits in the world, and it has an important position in Chinese culture and history (Liu & Sun, Citation2018). Due to the complicated production process, Chinese liquor has a variety of flavors and compositions. So far, people have detected more than 1,000 volatile compounds in Chinese liquor, including esters, alcohols, acids, aldehydes, polyphenols, aromatic hydrocarbons, and so on (Jin et al., Citation2017; Liu & Sun, Citation2018). The other compounds in liquor, except water and ethanol, are generally referred to as aroma compounds.
The taste of freshly brewed liquor is usually pungent, and it needs to be stored for a period of time before having its best taste, which the storing process is called aging. The aging process has a long cycle, often ranging from several months to several years, with low efficiency and high volatilization. Therefore, artificial aging technologies come into being, and many technologies have been developed. Some examples are micro-oxygen treatment (Schmidtke et al., Citation2011), ultrasonic treatment (Zheng & Sun, Citation2006), microwave treatment (Hu et al., Citation2019), magnetic field treatment (Zhang et al., Citation2008), electric field treatment (López et al., Citation2008), high-gravity treatment(Ma et al., Citation2013), etc. These aging technologies have different aging principles, and each has its own advantages and disadvantages. For example, both the micro-oxygenation method and microwave method can significantly affect the composition of the wine, but the micro-oxygen method introduces external oxygen into the wine while accidental operation may cause over-oxidation, and the microwave method easily causes a large amount of low-boiling aroma compounds to evaporate. As for magnetic field treatment and electric field treatment, the aging effects are often closely related to the equipment. As a result, single technology sometimes cannot meet our needs, so it is worth investigating to combine different technologies to create a new aging technology (Dai, Citation2019).
Both magnetic field treatment and microwave treatment can impact liquor, and the idea of combining magnetic field and microwave is promising. Researches have shown that the changes of some compounds in the liquor after magnetic field treatment are in line with the laws of traditional aging while taking a much shorter time (Su et al., Citation2009; Zhang et al., Citation2008). Microwave treatment can also effectively change the total ester content in the liquor (Yu et al., Citation2013). In some chemical reactions, obvious magnetic field effects can be observed, and matching magnetic field and microwave can further affect this magnetic field effect (Steiner & Ulrich, Citation1989). Similarly, in the engineering field, the phenomenon of magnetoplasticity has attracted attention. If a matching microwave is applied while a magnetic field is applied, the aforementioned magnetoplasticity effect will usually be more significant (Buchachenko, Citation2019). These remarkable effects of the combined treatment of microwaves and magnetic fields are instructive. Countless chemical reactions occur in liquor, and it is worth exploring whether different combinations of magnetic fields and microwaves can bring new changes to liquor.
In this study, we used different pulsed magnetic fields (PMF) treatment, microwave treatment, and the combination of PMF and microwave treatment to process soy-sauce flavor Chinese liquor. Compared with the use of permanent magnets to provide magnetic fields in previous studies, PMF makes the range of magnetic field strength larger and easier to control. The effects of separate PMF process, separate microwave process, and PMF-microwave composite process on liquor were analyzed, respectively, and the mechanism was discussed.
2. Materials and methods
2.1. Samples
The liquor samples used in the experiment were alc. 53% Vol soy-sauce flavor Chinese liquor (Sichuan Langjiu Group Co., Ltd., China).
Multi-element standard samples (Shanghai Rui Analysis Scientific Instrument Co., LTD., China) containing 15 compounds and 22 compounds were used (denoted as 15-MS and 22-MS respectively). The composition and corresponding concentration of each MS sample are shown in Table S1.
2.2. Apparatus
The composite processing equipment is assembled with PMF equipment and microwave equipment and was self-made by the laboratory (as shown in ). The PMF equipment can output a rectangular-wave magnetic field with adjustable intensity. By adjusting the current intensity to the power supply, magnetic fields of different intensities can be generated at the magnet yoke gap (marked by red dot in )). The specific intensity value of the PMF between the magneto yokes is detected by a HT-201 digital teslameter (Shanghai Hengtong Magneto-electric Technology Co., LTD., China). The intensity values in this study refer to the maximum peak value of the PMF. The microwave equipment can generate continuous microwave with a frequency of 2.45 GHz, which is transmitted to the sample through the rectangular waveguide. The output power of the microwave is in the range of 250 ~ 600 W, and the power is also controlled by adjusting the input current. The power employed in this study was 250 W.
Figure 1. Schematic of processing equipment (a) the PMF equipment, (b) the microwave equipment, and (c) the composite processing equipment, the direction of the microwave is perpendicular to the direction of the magnetic field.
Figura 1. Esquema del equipo de procesado (a) el equipo PMF; (b) el equipo de microondas; y (c) el equipo de procesado combinado. La dirección de las microondas es perpendicular a la dirección del campo magnético
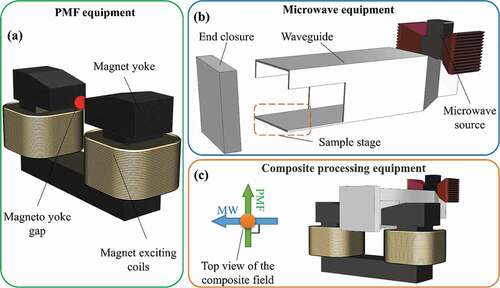
2.3. Sample treatment and analysis
The samples were sub-packaged into 5 mL glass test tubes and placed on the sample stages. Each process processed three unsealed samples. Separate PMF treatment, separate microwave treatment, and PMF-microwave composite treatment were used in the procedure. For conciseness, the processes were numbered. Process 0 represented the control group. Process 1 and 2 represented the separate PMF treatment process with intensities of 0.09 T and 0.7 T, respectively. Process 3 represented the separate microwave treatment process. Process 4 and 5 represented composite PMF and microwave treatment process, where the intensities of the PMF were 0.09 T and 0.7 T, respectively, and the directions of the microwave and the magnetic field were kept perpendicular to each other. The processing time was 250 s for all treatment processes, and the treatment operations were carried out at room temperature.
2.4. Sample analysis
2.4.1. Gas chromatography (GC) analysis
A GC-2010 gas chromatograph and an LZP-930 column were used to analyze the aroma compounds in liquor. GC-2010 gas chromatograph was equipped with an AOC-20i autosampler and a hydrogen flame ionization detector (FID) (Shimadzu Corporation, Japan). The chromatographic column was the special column LZP-930 (18 m × 0.53 mm, 1.0 m) for liquor analysis (Lanzhou Institute of Physical Chemistry, China). The samples were filtered with 0.33
m organic filter membrane before injection, and then samples of 1
L were injected at the splitless mode. Samples of 1
at the splitless mode were injected. Helium was used as carrier gas with a flow rate of 400 mL/min, and the gas pressure was 0.04 MPa. The injector temperature and the detector temperature were both 200°C. The flow rate of hydrogen and air were 40 and 400 mL/min, respectively, and the flow rate of makeup flow was 30 mL/min. The column temperature was kept at 35°C for 3 min, then it was raised to 135°C at a rate of 10°C/min and kept at 135°C for 1 min, and then it was raised to 180°C at a rate of 15°C/min and kept at 180°C for 5 min.
2.4.2. The proton nuclear magnetic resonance (1H-NMR) analysis
The proton nuclear magnetic resonance (1H-NMR) spectroscopy was employed to characterize the structure of molecules. The NMR spectra of liquor samples were recorded on JEOL-ECS600 (1H NMR). CDCl3 was used as the solvent. Spectra were referenced internally to the residual proton resonance in CDCl3 ( 7.26 ppm). Chemical shifts (
) were reported as part per million (ppm). The data of 1H-NMR spectrogram were conducted by MestReNova 14.1 (www.mestrec.com).
2.4.3. Qualitative and quantitative analysis
Qualitative analysis: The 22-MS sample was injected into GC to obtain the corresponding chromatogram, then the obtained chromatogram was compared with a chromatogram provided by the manufacturer at the time of factory inspection to determine compounds. Under the same GC conditions, the Chinese liquor sample was directly injected. The chromatogram of the MS sample was compared to the retention time to determine the compounds represented by peaks in the chromatogram of the liquor sample.
Quantitative analysis: The 15-MS sample was injected into the GC five times continuously, then calculated the relative standard deviations (RSDs) of the compounds. The result was shown in Table S2. Then the GC can be directly used for liquor analysis if the parameters were qualified. The concentration of each compound in the 22-MS sample used in the experiment, calculated by the one-point external standard method, was similar to the actual liquor sample. The value of peak area was used for calculation, and changes in the concentration of each compound after different processes were obtained.
In the formula, and
are the concentration and peak area of compound i in the injection volume of the liquor sample.
and
are the concentration and peak area of compound i contained in the 22-MS sample.
is the change in the concentration of compound i in the treated liquor sample.
is the concentration of compound i in the sample of the untreated group (sample 1). The three sets of samples processed by different treatment were detected by GC and the average
value and standard deviation (SD) of each aroma compound were calculated.
2.4.4. Detection and analysis of sample volume and temperature
Taking into account the evaporation loss of the sample solvent during the treatment, for a more in-depth quantitative comparison, the volume change and maximum temperature of the sample after each treatment were detected. The change of temperature was monitored by an electronic temperature controller (Shanghai Xiya Electronics Co., LTD., China). Three groups of samples are processed for each process to measure the volume change and maximum temperature and take the average value. The change in compound mass per unit initial volume after the treatment is calculated as follows:
In the formula, is the initial volume of organic matter in liquor.
is the coefficient of volume loss of organic matter during treatment.
and
are the mass of compound i in the untreated and the treated liquor sample, respectively.
is the change in the mass of compound i in the treated liquor sample.
is the change in mass per unit initial volume.
2.4.5. Sensory evaluation
Three professional tasters from a distillery in China conducted sensory evaluations of the liquor treated by different processes. The tasters tasted and evaluated the untreated liquor and treated liquor separately, with an interval of at least 10 minutes between each tasting. The color, taste (fullness, mellowness, sweetness), and aftertaste of liquor were scored, with a score ranging from 1 to 5. The full score of the evaluation was 30. The higher the score, the better the overall sensory characteristic of the liquor. The scores of several tasters were calculated as the average and standard deviation, and used as the final sensory evaluation results.
3. Results
3.1. Effects of different treatments on the liquor
The RSDs of detected compounds were all less than 2%, indicating good repeatability (Wang & Zhu, Citation2017). The typical gas chromatogram of the liquor sample is shown in . After GC analysis, 18 significant peaks were detected, and each peak was well separated.
Figure 2. Typical gas chromatogram of an experimental liquor sample.
Figura 2. Cromatograma de gases típico de una muestra de licor experimental
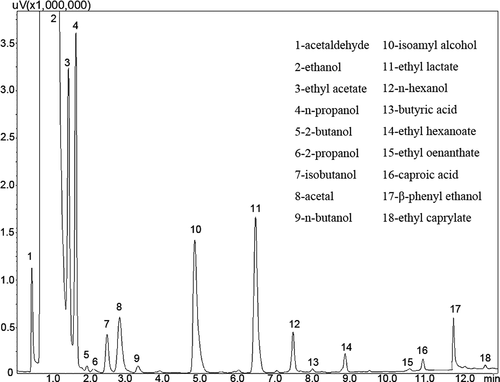
Qualitative and quantitative analyses were performed on the liquor samples subjected to different treatments. The concentration changes of each compound are shown in .
Table 1. The average change and standard deviation of the concentration of aroma compounds for the liquor subjected to different processes.
Tabla 1. Cambio medio y desviación estándar de la concentración de compuestos aromáticos en el licor sometido a diferentes procesos
As shown in , the detected aroma compounds were divided into four categories, and the variation trends of them after different treatment processes were observed. Different compounds have different contributions to the flavor of the liquor. Esters serve as the main contributors to Chinese liquor’s flavor, and they provide a floral and fruity smell and a unique soy sauce taste for the liquor. Alcohols play the second most important role in contributing fragrance, providing sweet and fruity flavors. The acids have a cheesy and sweaty taste, but organic acids can enrich and balance the taste of liquor. Aldehydes and acetals (collectively referred to as aldehydes here) provide a floral and fruity smell on the one hand and a fresh and green flavor on the other. The change in the taste of liquor is attributed to the change of compounds, which can be assessed by the sensory evaluation.
Figure 3. Changes in the concentration of aroma compounds in the liquor samples subjected to different processes.
Figura 3. Cambios en la concentración de compuestos aromáticos de las muestras de licor sometidas a diferentes procesos
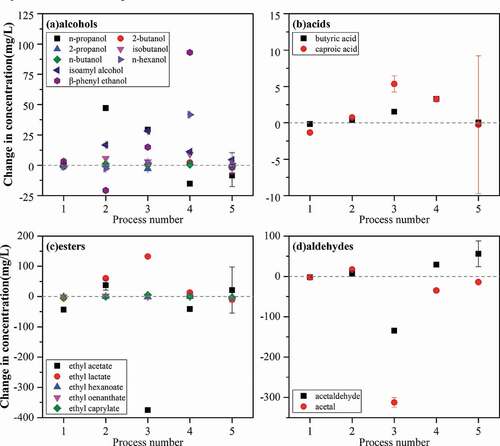
3.1.1. Effects of the PMF treatment on the aroma compounds in liquor
The effects of the PMF with different intensities on the aroma compounds in liquor were compared. As shown in , process 2 had a more significant effect on liquor than process 1. After process 1, the concentration of all aroma compounds in the liquor kept constant, except for the decrease of ethyl acetate. After process 2, the concentrations of most alcohols, esters, and aldehydes in the liquor were increased. Among them, the concentrations of n-propanol, ethyl lactate, and ethyl acetate had significant increases, increasing by 29.825 mg/L, 60.273 mg/L, and 37.731 mg/L, respectively. In the meantime, the concentration of β-phenyl ethanol decreased. The concentration of other compounds changed little. The above results showed that separate PMF with weak intensity had little effect on the composition in the liquor. In contrast, the concentrations of multiple alcohols and esters in the liquor were increased after processed by strong PMF, and the overall concentration of aroma compounds tended to increase.
3.1.2. Effects of the microwave treatment on the aroma compounds in liquor
The microwave treatment process had a very significant effect on the composition of the liquor. After process 3, the concentration of alcohols showed an overall increasing trend. The concentrations of n-propanol, isoamyl alcohol, and β-phenyl ethanol increased the most after the treatment process. In the meantime, the concentrations of acetaldehyde and acetal were greatly decreased, decreasing by 134.214 mg/L and 312.358 mg/L, respectively. As for esters, the concentrations of ethyl acetate and ethyl lactate changed drastically, decreasing by 374. 824 mg/L and increasing by 132.511 mg/L, respectively. The concentrations of other compounds were almost unchanged. The effects of microwave treatment were different from the effects of PMF treatment. After the microwave treatment process, the concentrations of alcohols and acids in the liquor were increased, while the concentrations of esters and aldehydes decreased significantly. The overall aroma compounds tended to decrease after the microwave treatment. From the results, the microwave treatment process may lead to the loss of special flavors of the Chinese liquor.
3.1.3. Effects of the PMF-microwave composite treatment on the aroma compounds in liquor
The composite PMF and microwave treatment had a significant effect on some compounds in the liquor. Process 4 had significant effects on alcohols, aldehydes, and esters in Chinese liquor. After process 4, the overall concentration of alcohols increased. Among them, the concentration of n-hexanol and β-phenyl ethanol increased the most, increasing by 41.696 mg/L and 93.120 mg/L, respectively, while the concentration of n-propanol decreased slightly. For aldehydes, the concentration of acetaldehyde increased by 29.057 mg/L, while the concentration of acetal decreased by 34.620 mg/L. For esters, the ethyl acetate decreased by 41.308 mg/L. The concentrations of other compounds had no evident changes. Process 5 had no discernible effect on most compounds in the liquor. After process 5, only the concentration of n-propanol among alcohols was decreased by 8.5 mg/L. Among aldehydes, the concentration of acetaldehyde increased by about 55.9 mg/L, while the concentration of acetal decreased by about 13.9 mg/L. For esters, the concentrations of ethyl acetate and ethyl lactate increased by 21.7 mg/L and decreased by 10.3 mg/L, respectively. In addition to the above compounds, the concentration of other compounds in the liquor changed slightly. After the composite treatment, the overall concentration of aroma compounds in the liquor increased. Among them, the concentration of various alcohols and acids increased, while the concentration of esters and aldehydes did not change much.
3.2. Effect of different treatments on hydrogen-bond structure in liquor
The main components in liquor are water and ethanol, and both of them are easy to form hydrogen bonds. The hydrogen-bonding structure of water-ethanol molecules in Chinese liquor has been studied, and it can be affected by the alcohol concentration, trace elements, storage time, and so on (Mizuno et al., Citation1995; Nose & Hojo, Citation2006; Qiao et al., Citation2014; Zeng & Fan, Citation2010). For distilled spirits, the changes of the hydrogen-bonding structure have been regarded as being related to the taste (Nishimura & Matsuyama, Citation1989). However, there has not been in-depth and systematic research to reveal the scientific relationship between the hydrogen-bonding structure and the taste of liquor. Still, it is undeniable that the analysis of hydrogen-bonding structure is an effective means to develop artificial aging technology.
1H-NMR was used to reveal the hydrogen-bonding structure of the liquor in this study. As shown in , there are four peaks in the typical 1H-NMR spectrum of the liquor. The analysis of multiple peaks was as follow: 1H NMR (600 MHz, CHLOROFORM-D) δ 3.66 (q, J = 7.1 Hz, 2 H), 2.27 (d, J = 35.4 Hz, 1H), 1.19 (t, J = 7.1 Hz, 3 H).
The position and the shape of the -OH peak were related to the proton’s chemical environment. In the water-ethanol system, the hydrogen-bonding interaction caused the chemical shift of the related protons to increase, and associated molecules with different structures are formed(Qiao et al., Citation2014). Due to the high water content in the liquor, the peak at about δ 2.25 was formed by the coincide of the hydroxyl peak of ethanol and the water peak, which is also a characteristic peak that can reflect the change of hydrogen-bonding structure (Fulmer et al., Citation2010). The -OH peaks in 1H-NMR of the liquor for different treatments are shown in . Similarly, the -OH peaks are wide and accompanied by sharp peaks. The position of the highest sharp peak of each -OH peak is marked for comparison. The -OH peak of the control sample is a triplet peak. After process 1, the peak position of the main pinnacle moved slightly to the right (the chemical shift (δ) was smaller), and the split of the peak was still visible. After process 2, the main pinnacle moved further to the right, and the peak became flat and wide. After process 3, 4, and 5, the pinnacle in -OH peak gradually shifted to the right, the shape of it became smooth, and the peak width gradually increased.
Figure 5. The -OH peaks in 1H NMR spectrum of liquor subjected to different processes.
Figura 5. Picos de -OH en el espectro de 1H-NMR del licor sometido a diferentes procesos
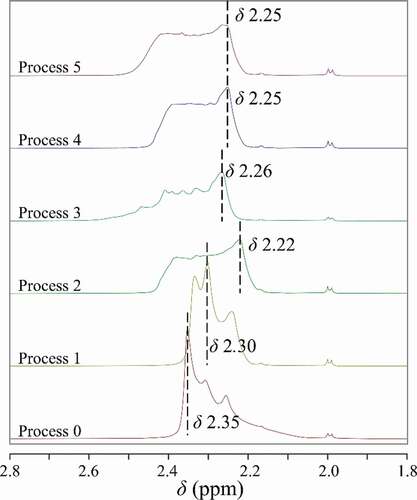
The results showed that, for PMF treatments, the low-intensity PMF had a slight effect on the hydrogen-bonding structure, and the high-intensity PMF may directly destroy the original water-ethanol associations and form more associated molecules with different structures. As for microwave treatment and two composite processes, the water-ethanol association is all weakened after processes. Throughout the five processes, processes 2, 4 and 5 had a prominent effect on the weakening of water-ethanol association.
3.3. The change in mass per initial volume of the liquor sample after treatment
During PMF treatment, the temperature and volume of the liquor samples did not change in any way. After microwave and composite processing, the maximum temperature after processing reached 78.2°C and 65.6°C, and the volume loss coefficient were 0.063 and 0.013, respectively. The change in mass per initial volume of aroma compound in liquor sample after treatment are shown in . The detailed data are shown in Table S3.
Figure 6. The change in mass per initial volume of aroma compound in liquor.
Figura 6. Cambio de masa por volumen inicial del compuesto aromático en el licor
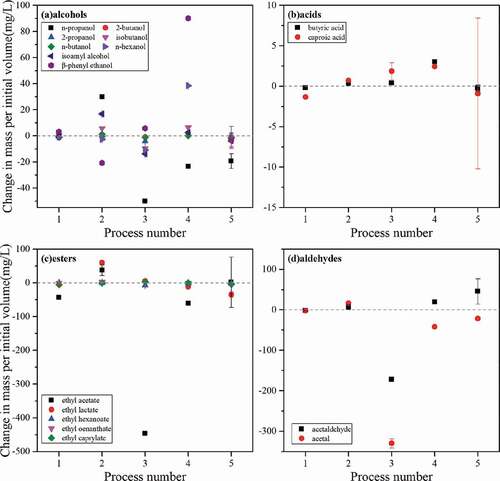
The mass per initial volume of each compound in the sample after treatment can be regarded as the concentration that assuming the volume does not change during the treatment process. Comparing and 2, the results showed that the data in the two figures were consistent since the solvent volume loss coefficient after PMF treatment was 0. After microwave treatment, whether it is alcohols, esters or aldehydes, the total content was significantly reduced, and the acid content was slightly increased. After the compound treatment, due to the small volume loss of the liquor sample, the mass change value of each aroma compound was slightly smaller than the measured concentration change value, but the overall variation trend was the same as that described in 3.1.3.
3.4. Effects of different treatments on the sensory characteristics of liquor
There are many compounds in Chinese liquor. Physical and chemical testing can only determine the main components of liquor, but so far there is no equipment that can directly determine the sensory characteristics of liquor through the content of compounds, and can only be evaluated and tested by people’s senses. The sensory evaluation scores and visualization results of the liquor samples treated by different processes are shown in and , respectively.
Table 2. The sensory evaluation scores of liquor subjected to different processes.
Tabla 2. Puntuación de la evaluación sensorial del licor sometido a diferentes procesos
Figure 7. The visualized results of sensory evaluation of liquor samples subjected to different processes.
Figura 7. Resultados visualizados en la evaluación sensorial aplicada a las muestras de licor sometidas a diferentes procesos
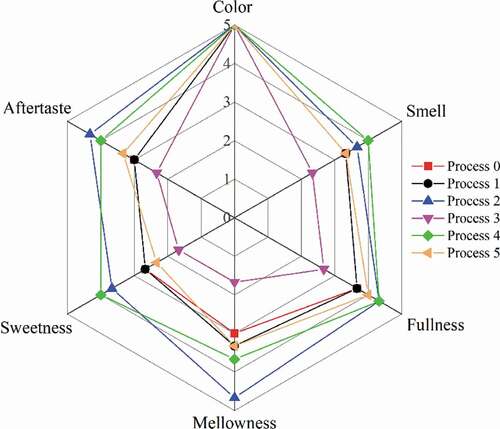
The untreated liquor had a full aroma, a heavy taste, and a little bit of pungency, with a medium aftertaste in the mouth. After process 1 and process 5, the sensory characteristics of the liquor did not change significantly. After process 2 and process 4, the taste of the liquor was richer and softer, the sweetness was increased, and the aftertaste became longer. Overall, the taste was better. After process 3, the smell of the liquor becomes lighter, the taste was unbalanced, and it was more pungent, and the aftertaste became shorter, which can be said to have a negative effect on the taste.
4. Discussions
4.1. The aging effects of different treatments on liquor
After component analysis, 1H-NMR analysis, and sensory analysis, it is obvious that processes 2, 3, and 4 all have a significant impact on the liquor. Among them, processes 2 and 4 are beneficial to improve the taste of liquor, but it is necessary to compare with the changes of compounds in the traditional aging process to determine whether they are beneficial to the aging of liquor.
According to the reference (Meng & Tian, Citation2015), the concentrations of esters and alcohols compounds in soy-sauce flavor Chinese liquor gradually decrease during 0–24 months of aging, while the concentrations of acids and aldehydes increase. In this study, the concentration changes of acids and aldehydes in liquor treated by process 2 are consistent with those during traditional aging, while the changes of other compounds are opposite. After process 3 and 4, the concentration changes of esters and acids in the liquor are both consistent with those during traditional aging, and the changes of other compounds are opposite.
In order to compare the aging effect of process 2, 3 and 4, the ratio of the changes in the concentration of compounds in the liquor is analyzed. The concentration changes of aroma compounds in typical soy-sauce flavor Chinese liquor during traditional aging for 24 months are obtained from Meng & Tian (Citation2015), and those in liquor treated by process 2, 3 and 4 are calculated from . These data are normalized: the magnitude of concentration change of acids is defined as 1, the change magnitudes of other compounds are determined based on this, and the increase and decrease in concentration are represented by + and – respectively. The concentration change ratio of aroma compounds after different treatments is shown in the , in which the data points can form different quadrilaterals. The closer the quadrilateral represented by a process is to that of traditional aging, the more coordinated and balanced the change in compounds caused by the process. It is shown that the change ratio of compounds in the liquor treated by process 4 is the closest to that of traditional aging. The increase of esters and aldehydes in liquor after process 2 is dramatically higher than that in traditional aging. Process 3 leads to a drastic reduction of esters and aldehydes in liquor, resulting in a large loss of aroma compounds.
Figure 8. The normalized change in concentration of the liquor subjected to different processes.
Figura 8. Cambio normalizado de la concentración del licor sometido a diferentes procesos
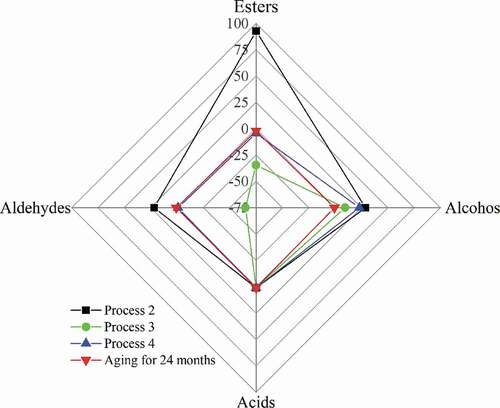
The above results show that process 4 improves the liquor quality the best among the five processes in this study because of the improvement in sensory characteristics and the similar variation of aroma compounds to that of traditional aging. Process 2 also improves the taste of the liquor, but the variation of aroma compounds is different from that of traditional aging. Process 3 is harmful to the taste of liquor and causes a large loss of flavor compounds.
4.2. The mechanism of different treatments on liquor
4.2.1. The mechanism of PMF treatment on liquor
After the PMF treatment, the changes in the liquor are mainly reflected in the increase in the concentration of total aroma compounds, the weakening of the water-ethanol hydrogen-bonding structure, and the improvement of taste.
PMF can affect the associative structures in liquor, which in turn can affect taste of liquor and chemical reactions in it. Compared with other physical fields, the energy of the magnetic field is low. so the thermal energy generated by the magnetic field has a negligible effect on the system(Pan, Citation2015). It is generally believed that the external magnetic field can align the polar molecules in liquor, which affects the hydrogen-bonding structure between molecules. When a magnetic field is applied, the hydrogen bonds between the molecules are distorted or even broken in the process, and the structure of larger associated molecules is depolymerized(Toledo et al., Citation2008). The magnetic field used in this study is applied as continuous pulses, which will cause a more significant effect. In PMF process, there is a rising stage and a falling stage of magnetic field intensity during each pulse, and the magnetic flux changes dramatically during these stages. In the liquor, there are some closed associations can be regarded as the closed loops. When the magnetic flux of the closed-loop changes, an induced current is generated in it, and the direction of the induced current is opposite at the rising and falling stages of the pulse. As a result, the PMF produces opposite forces on the molecules periodically in the liquor, and the associations in the liquor are agitated, making the above-mentioned depolymerization more significant. The effects brought by the PMF are closely related to the intensity of the magnetic field, so the weak magnetic field has almost no effect on the liquor, which can be confirmed by the 1H-NMR results here.
After the PMF treatment, the taste of the liquor becomes obviously more mellow, which is consistent with other reports, and one view is that the taste of liquor is closely related to the intermolecular structures (Xiao et al., Citation2004). The direct relationship between intermolecular structure and taste has not been ascertained, but the weaker the water-ethanol associative structures may lead to a more mellow taste in this study. The slight increase in the concentration of esters after process 2 can be explained from two aspects. On the one hand, the depolymerization of the PMF increases the entropy of the reaction system. On the other hand, the presence of the external magnetic field increases the exposure of the carbon ions in the carboxylic acid carbonyl group and promotes the interaction between the nucleophile and the carbocation. Consequently, the esterification reaction speeds up (Cui, Citation2007). As for alcohols, the specific mechanism of PMF on them is still vague. There are numerous compounds in liquor and some of the mechanisms we have explored so far are just a few of them. In other solution systems, the magnetic field has been found to be able to affect free radical reactions (Mclauchlan, Citation1981; Rodegers, Citation2009; Steiner & Ulrich, Citation1989), catalytic oxidation reactions (Lu et al., Citation2005), etc., which has an important reference for the study of the mechanism of PMF of liquor.
4.2.2. The mechanism of microwave treatment on liquor
The mechanism of microwave aging on liquor is usually discussed from two aspects: thermal effect and non-thermal effect(Antonio et al., Citation2005; Q. Jin, Citation2001). The thermal effect of microwave treatment increases the temperature of the liquor, promotes the volatilization of low-boiling ingredients, and thus affects the concentration of aroma compounds in the liquor(Hu et al., Citation2019). The non-thermal effect is still a controversial matter, and it is difficult to separate from thermal effect in mechanistic studies. Therefore, the effect of microwave on liquor is mainly explained from the thermal effect (Antonio et al., Citation2005).
In this study, the temperature of the liquor sample increased to 78.2°C after microwave treatment, which is almost equal to the boiling point of the ethanol. The concentration of some alcohols and esters increased after treatment, which can be attributed to the decrease in solvent volume caused by the thermal effect, because the change in their mass is not obvious. The greatly reduced concentration of volatile aldehydes can also be ascribed to the volatilization of themselves. The large reduction in ethyl acetate is due to the fact that microwave can promote the hydrolysis of esters when the processing time is short (Dai, Citation2019), and the hydrolysis rate of ethyl acetate is significantly higher than that of other common ethyl esters when the alcohol content is 53 v/v% (Han et al., Citation2009). The increase in concentration of acids can also be attributed to the hydrolysis of esters. In addition, the weakened water-ethanol associations observed in 1H-NMR can be explained by the loss of ethanol in liquor, which causes the decrease in the overall number of hydrogen bonds.
4.2.3. The mechanism of PMF-microwave composite treatment on liquor
When PMF and microwave are combined, the various effects mentioned above are superimposed and resulting in a special processing effect. During the composite process, the concentration of esters slightly decreased, which is the result of the combination of PMF’s promoting effect and the microwave’s inhibiting effect on the esterification reaction. The further change in concentration of acids is also attributed to the hydrolysis of esters. The decrease in concentration of aldehydes is due to the thermal effect of microwave. The increase in concentration of alcohols is mainly considered to be the contribution of PMF. Interestingly, in PMF treatment, a higher intensity leads to a more obvious change of liquor. While in composite treatment, the treatment effect is better when the PMF intensity is lower. This can be explained by the electron spin resonance effect. The composite field under resonant conditions has a significantly higher impact on some chemical reactions and material properties than under non-resonant conditions (Golovin & Morgunov, Citation1999; Masahiro et al., Citation1993; Okazaki & Toriyama, Citation1993) and the magnetic field of 0.09 T and the microwave at 2.45 GHz just meet the resonant conditions. In conclusion, the coupling between the various effects of PMF and microwave leads to a very similar effect between process 4 and the traditional aging process, and the quality of the liquor is therefore improved.
5. Conclusion
The aging effects of PMF treatment, microwave treatment and PMF-microwave composite treatment on soy-sauce flavor Chinese liquor are investigated. As a new treatment technology, the 0.09 T PMF-microwave composite treatment can improve the quality of the liquor. The sensory characteristic of the liquor after treated by the treatment is better and the change trend in concentration of aroma compounds is similar to that in traditional aging. The taste of liquor treated by 0.7 T PMF is more mellow, but the change trend of aroma compounds is somewhat different from that in traditional aging. As for liquor processed by microwave treatment, the aroma substances are greatly lost and the taste is worse. These results prove the potential of PMF-microwave treatment in liquor aging.
The mechanisms of the above processes on liquor aging are discussed. The mechanism of PMF treatment is mainly due to the change that PMF causes on molecular associative structures. The effect of microwave is mainly attributed to the thermal effect. The effect of composite treatment is the result of the coupling of the separate effects of PMF and microwave. Further research is necessary in order to know the relationship between the taste and water-ethanol associations and the effect of electron spin resonance on liquor quality.
Abbreviations
PMF | = | Pulsed magnetic field |
GC | = | Gas chromatography |
1H-NMR | = | Proton nuclear magnetic resonance spectroscopy |
MS | = | Multi-element standard |
RSD/SD | = | Relative standard deviation/standard deviation |
Author’s contributions
Conceptualization, D.N. and Z.C.; methodology, D.N.; validation, D.N.; formal analysis, D.N.; investigation, D.N.; resources, Z.C.; data curation, D.N.; writing-original draft preparation, D.N.; writing-review and editing, X.Z. and Z.C.; visualization, D.N.; supervision, Z.C.; project administration, Z.C., Y.W., and W.J.; funding acquisition, Z.C., K.L.; all authors have read and agreed to the published version of the manuscript.
Supplemental Material
Download MS Word (28.2 KB)Disclosure statement
No potential conflict of interest was reported by the authors.
Supplementary material
Supplemental data for this article can be accessed on the publisher’s website
Additional information
Funding
References
- Antonio, D., Díaz-Ortiz, Á., & A, M. (2005). Microwaves in organic synthesis. Thermal and non-thermal microwave effects. Chemical Society Reviews, 34(2), 164–178. https://doi.org/https://doi.org/10.1039/B411438H
- Buchachenko, A. L. (2019). Microwave stimulation of dislocations and the magnetic control of the earthquake core. Physics-Uspekhi, 62(1), 46–53. https://doi.org/https://doi.org/10.3367/UFNe.2018.03.038301
- Cui, G. (2007). Influence of magnetic field on dynamics of the essential reactions. Tianjin University.
- Dai, J. (2019). Application of microwave and infrared technology in liquor aging. Henan University of Science and Technology.
- Fulmer, G. R., Miller, A. J. M., Sherden, N. H., Gottlieb, H. E., Nudelman, A., Stoltz, B. M., Bercaw, J. E., & Goldberg, K. I. (2010). NMR chemical shifts of trace impurities: Common laboratory solvents, organics, and gases in deuterated solvents relevant to the organometallic chemist. Organometallics, 29(9), 2176–2179. https://doi.org/https://doi.org/10.1021/om100106e
- Golovin, Y. I., & Morgunov, R. B. (1999). Effect of a weak magnetic field on the state of structural defects and the plasticity of ionic crystals. Journal of Experimental and Theoretical Physics, 88(2), 332–341. https://doi.org/https://doi.org/10.1134/1.558801
- Han, X., Zhang, W., Wang, Y., & Wang, Y. (2009). Preliminary research on hydrolysis reaction of four main kinds of ethyl esters. Liquor-Making Science & Technology, (4), 17–20. https://doi.org/https://doi.org/10.13746/j.njkj.2009.04.010
- Hu, S., Luan, D., Fan, Y., & Lai, K. (2019). Study on the microwave aging of spirit and optimiztion of its process. Food & Machinery, 35(3), 189–194. https://doi.org/https://doi.org/10.13652/j.issn.1003-5788.2019.03.034
- Jin, G., Zhu, Y., & Xu, Y. (2017). Mystery behind Chinese liquor fermentation. Trends in Food Science & Technology, 63, 18–28. https://doi.org/https://doi.org/10.1016/j.tifs.2017.02.016
- Jin, Q. (2001). Microwave chemistry. Science Press.
- Liu, H., & Sun, B. (2018). Effect of fermentation processing on the flavor of Baijiu. Journal of Agricultural and Food Chemistry, 66(22), 5425–5432. https://doi.org/https://doi.org/10.1021/acs.jafc.8b00692
- López, N., Puértolas, E., Condón, S., Álvarez, I., & Raso, J. (2008). Effects of pulsed electric fields on the extraction of phenolic compounds during the fermentation of must of Tempranillo grapes. Innovative Food Science & Emerging Technologies, 9(4), 477–482. https://doi.org/https://doi.org/10.1016/j.ifset.2007.11.001
- Lu, X., Zhai, J., Zhao, C., & Li, H. (2005). Magnetic-assisted micro-ozone applied to oxidize reactive blue dye simulated wastewater. Chemical Journal on Internet, 7(11), 71. https://www.mdpi.org/cji/cji/2005/07b071ne.ht
- Ma, Y., Qiao, H., Wang, W., Chen, T., Du, X., Zhai, X., & Zhang, S. (2013). Variations in physicochemical properties of Chinese Fenjiu during storage and high-gravity technology of liquor aging. International Journal of Food Properties, 17(4), 923–936. https://doi.org/https://doi.org/10.1080/10942912.2012.678536
- Masahiro, M., Fujiwara, Y., & Tanimoto, Y. (1993). Product yield-detected ESR studies of photochemical reaction of a bifunctional chain molecule. Magnetic field and microwave effects*. Zeitschrift Für Physikalische Chemie, 180, 223–233. https://doi.org/https://doi.org/10.1524/zpch.1993.180.Part_1_2.223
- Mclauchlan, K. A. (1981). The effects of magnetic-fields on chemical-reactions. Science Progress, 67(268), 509–529. https://www.jstor.org/stable/43420544
- Meng, W., & Tian, Z. (2015). The change rules of the flavoring compounds of Jiangxiang Baijiu (Liquor) during the storage period. Liquor-Making Science & Technology, (7), 21–27. https://doi.org/https://doi.org/10.13746/j.njkj.2015227
- Mizuno, K., Miyashita, Y., & Shindo, Y. (1995). NMR and FT-IR studies of hydrogen bonds in ethanol-water mixtures. The Journal of Physical Chemistry, 99(10), 3225–3228. https://doi.org/https://doi.org/10.1021/j100010a037
- Nishimura, K., & Matsuyama, R. (1989). Maturation and maturation chemistry. In J. R., Piggott, R., Sharp, & R. E. B., Duncan (Eds.), Science & Technologies of Whiskies (pp. 244-253). Essex: Longman Scientific & Technical.
- Nose, A., & Hojo, M. (2006). Hydrogen bonding of water-ethanol in alcoholic beverages. Journal of Bioscience and Bioengineering, 102(4), 269–280. https://doi.org/https://doi.org/10.1263/jbb.102.269
- Okazaki, M., & Toriyama, K. (1993). Pulse mode product-yield-detected ESR on the photoreduction of anthraquinone in SDS micellar solution: Reaction control by a microwave pulse. Bulletin of the Chemical Society of Japan, 66(7), 1892–1896. https://doi.org/https://doi.org/10.1246/bcsj.66.1892
- Pan, H. (2015). The study of magnetic field effects based on free-radical reactions in solution. Huazhong University of Science & Technology.
- Qiao, H., Zhang, S., Wang, H., Wei, J., & Sun, T. (2014). Research progress in ethanol-water association in Baijiu(Liquor). Liquor-Making Science & Technology, (3), 18–77. https://doi.org/https://doi.org/10.13746/j.njkj.2014.03.02
- Rodegers, C. T. (2009). Magnetic field effects in chemical systems. Pure and Applied Chemistry, 81(1), 19–43. https://doi.org/https://doi.org/10.1351/PAC-CON-08-10-18
- Schmidtke, L. M., Clark, A. C., & Scollary, G. R. (2011). Micro-oxygenation of red wine: Techniques, applications, and outcomes. Critical Reviews in Food Science and Nutrition, 51(2), 115–131. https://doi.org/https://doi.org/10.1080/10408390903434548
- Steiner, U. E., & Ulrich, T. (1989). Magnetic field effects in chemical kinetics and related phenomena. Chemical Reviews, 89(1), 51–147. https://doi.org/http://dx.doi.org/10.1021/cr00091a003
- Su, H. N., Huang, W. D., Zhang, J. C., & Wang, X. (2009). Effect of magnetic field on the contents of proanthocyanidins in young red wines. Science and Technology of Food Industry, 30(11), 112–115. https://doi.org/https://doi.org/10.13386/j.issn1002-0306.2009.11.027
- Toledo, E. J. L., Ramalho, T. C., & Magriotis, Z. M. (2008). Influence of magnetic field on physical–chemical properties of the liquid water: Insights from experimental and theoretical models. Journal of Molecular Structure, 888(1–3), 409–415. https://doi.org/https://doi.org/10.1016/j.molstruc.2008.01.010
- Wang, S., & Zhu, J. (2017). Analytical chemistry. Science Press.
- Xiao, L. M., Zeng, X. A., Chen, Y., & Yang, H. F. (2004). FTIR analysis of fresh dry red wine treated by electromagnetic field. Food Science, 25(1), 152–155. https://doi.org/https://doi.org/10.3321/j.issn:1002-6630.2004.01.039
- Yang, X. Zeng, & X. Fan, R. (2010). Study on the hydrogen bonding in the ethanol-water system by 1H NMR. Science and Technology of Food Industry, 32(7), 112–114+118. https://doi.org/https://doi.org/10.13386/j.issn1002-0306.2011.07.070
- Yu, L., Liu, J., Feng, W., & Bai, W. (2013). Effects of aging method on physicochemical properties and volatile aroma of mangosteen wine. Modern Food Science and Technology, 29(10), 2443–2446. https://doi.org/https://doi.org/10.13982/j.mfst.1673-9078.2013.10.032
- Zhang, Q., Chen, X., Zhan, J., Tian, R., Wang, X., & Huang, W. (2008). Effect of magnetic field on the contents of phenolic compounds in red wines. Science and Technology of Food Industry, 29(9), 108–111. https://doi.org/https://doi.org/10.13386/j.issn1002-0306.2008.09.022
- Zheng, L., & Sun, D. W. (2006). Innovative applications of power ultrasound during food freezing processes—a review. Trends in Food Science & Technology, 17(1), 16–23. https://doi.org/https://doi.org/10.1016/j.tifs.2005.08.010