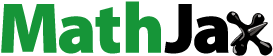
ABSTRACT
The objective of this study was to incorporate avocado peel (AEE) and coconut (CEE) extracts as antifungal additive in the development of gelatin and carboxymethylcellulose coatings to extend shelf life in strawberries. Fractions of 200 mg/L of extracts were incorporated in the coating and characterized during storage at 2°C. AEE and CEE in the coating delayed significantly (P < .05) weight loss (40%), softening, color, and compositional changes (°Brix, sugars, titratable acidity). Rhizopus stolonifer and Aspergillus niger strains were isolated and inoculated to evaluate the effectiveness of the coatings on strawberries. The use of the coating + plant extracts decreased the proliferation of fungi by 45% compared to the uncoated fruit and extended the shelf life for 15 days. With these findings, it is suggested that biopolymeric coatings + plant extracts can be used as a potential method of ecological preservation in strawberries against microbial deterioration.
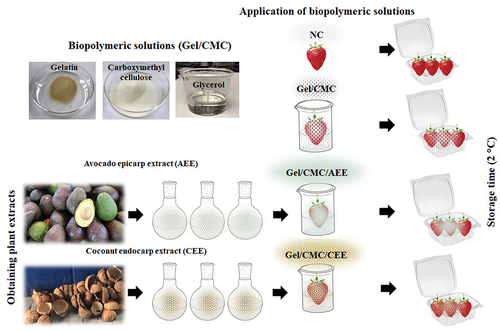
RESUMEN
El objetivo de este estudio fue incorporar extractos de cáscara de aguacate (ECA) y coco (EEC) como aditivo antifúngico en el desarrollo de recubrimientos de gelatina y carboximetilcelulosa para extender la vida de anaquel en fresas. Se incorporaron fracciones de 200 mg/L de extractos en el recubrimiento y se caracterizaron durante el almacenamiento a 2°C. Los ECA y EEC en el recubrimiento retrasaron significativamente (P <.05) la pérdida de peso (40%), el ablandamiento, el color y los cambios de composicionales (°Brix, azúcares, acidez titulable) en el fruto. Se aislaron e inocularon cepas de Rhizopus stolonifer y Aspergillus niger para evaluar la efectividad de los recubrimientos en fresas. El uso del recubrimiento + extractos de plantas disminuyó la proliferación de hongos en un 45% en comparación con los frutos sin recubrimiento y extendió la vida de anaquel durante 15 días. Con estos hallazgos, se sugiere que los recubrimientos biopoliméricos + extractos de plantas pueden usarse como un método potencial de conservación ecológica en fresas contra el deterioro microbiano.
1. Introduction
Strawberries (Fragaria x ananassa) are a non-climacteric fruit that must be harvested with full maturity to achieve excellent nutritional composition. However, due to its accelerated metabolism and postharvest activities, it can have a reduced shelf life (Q. Li et al., Citation2020). The softness and turgor of the fruit walls leave it exposed to mechanical injuries, softening, and even more deterioration due to fungal rot in the postharvest period (Muley & Singhal, Citation2020). Fungal disease caused by fungi Botrytis cinerea, Rhizopus stolonifer, Aspergillus niger, and Colletotrichum acutatum are the most infectious and harmful in strawberry postharvest management (Swett et al., Citation2020; Ventura-Aguilar et al., Citation2018). Consequently, fruit rotting is an economic vulnerability even in well-managed fields. This can lead to economic losses that exceed up to 50% of the total harvest worldwide. For this reason, the difficulty of preserving the fruit and reducing shelf life has led to the commercialization of the strawberry in frozen form or processed as jam or jelly. However, this processing reduces nutritional properties and the possibility of fresh consumption of strawberries (Octavia & Choo, Citation2017).
Due to the nutritional and economic importance of strawberries, different preservation techniques have been studied to reduce losses and extend the commercial fruit life. Pre-cooling and refrigeration are widely used to preserve quality and ensure food safety and innocuousness (Zhao et al., Citation2019). Likewise, modified and controlled atmospheres can manage strawberries through commercialization chains (Anami et al., Citation2020). Other techniques such as radiation (Shankar et al., Citation2021), high-temperature and sonication (Yildiz & Aadil, Citation2020), ultraviolet light (Janisiewicz et al., Citation2021), electromagnetic fields (Giannoglou et al., Citation2021), osmotic treatments (Jiang et al., Citation2021), and polymeric coatings (Abdollahzadeh et al., Citation2021) have been evaluated for food preservation. However, the application of these treatments at an industrial level has been restricted by external environmental factors such as relative humidity and temperature changes (Bovi et al., Citation2018). Thus, biopolymeric active coatings have received particular attention due to their biodegradability and fruit affinity characteristics. Coatings can be formed individually or combined with different biopolymers, waxes, mucilages, and other components related to food. Thereby, gelatin-based coatings have been extensively used and receive special attention due to their distinctive functional properties as coating-forming capacity, gel formation, good oxygen barrier, and considerably improving the sensory characteristics of food (Cardoso et al., Citation2016; Kingwascharapong et al., Citation2020; López-Palestina et al., Citation2019; Xiong et al., Citation2021). Likewise, gelatin coatings are water-soluble, non-toxic, biodegradable, low cost, and biocompatible with lipids and polysaccharides (Gómez-Guillén et al., Citation2011; Ramos et al., Citation2016). However, gelatin’s limited thermal stability and mechanical properties reduce its potential application (Etxabide et al., Citation2017; Luo et al., Citation2022). These properties have been enhanced when interacting with polymers such as casein (Khedri et al., Citation2021; Pellá et al., Citation2020), chitosan (Hajji et al., Citation2021; Nagarajan et al., Citation2021), starch (Friedrich et al., Citation2020; Pattanayaiying et al., Citation2019), Persian gum (Khorram et al., Citation2017; Sharif et al., Citation2021), alginate (Abdallah et al., Citation2018; De Souza et al., Citation2021), and carboxymethylcellulose (Salama et al., Citation2019; Samsi et al., Citation2019).
Carboxymethylcellulose (CMC) and gelatin (Gel) represent an ecological, compatible, and a highly biodegradable alternative as an edible coating for food preservation. The combination of CMC and gelatin has been reported to have a range of benefits like increased viscoelasticity, resistance to oil/fat, enhanced coating-forming properties, ability to extend the shelf-life of fruit and vegetables. Additionally, Gel/CMC biopolymeric matrix can form transparent edible coatings-films and could be a carrier for active additives as antioxidants and antimicrobial agents (Esteghlal et al., Citation2018; Mu et al., Citation2012; Panahirad et al., Citation2021).
Furthermore, biopolymeric active coatings are useful as vehicles to transport food additives, natural antimicrobials, antioxidants, pigments, essential oils, and natural extracts to avoid the deterioration of the fruit and extend the shelf life (Muley & Singhal, Citation2020). Plant extracts with the potential for microbial inhibition have been suggested to lengthen shelf life by controlling fungal development. In this regard, the application of essential oils (EO´s) in strawberry fruit such as those obtained from cinnamon (Cinnamomum zeylanicum; Chu et al., Citation2020) and clove (Syzygium aromaticum; Hasheminejad & Khodaiyan, Citation2020) can inhibit the growth of various fungal species such as Penicillium expansum (Da Rocha Neto et al., Citation2019), B. cinerea (Campos-Requena et al., Citation2017), and R. stolonifer (Oliveira et al., Citation2019). However, EO´s can alter the sensory properties of the fruit although the inhibition may only be temporary due to EO´s volatile nature. Thus, alternatives are needed that do not alter the sensory attributes and that do not lose their antimicrobial effect by volatilization. Consequently, other antifungal materials have been tested such as olive leaf extracts (Khemakhem et al., Citation2018), pecan nut (Yemmireddy et al., Citation2020), banana peel (Zhang et al., Citation2020), coconut shell (Prakash et al., Citation2018), and avocado peel (Melgar et al., Citation2018).
Avocado and coconut processing has increased for obtaining products like guacamole, avocado pulp, coconut flour, coconut sugar, coconut cheese, cosmetic products like face creams, and oils generating a high content of peels and seeds residues (Del Castillo-Llamosas et al., Citation2021). The peels represent 7–24% of the dry weight of the fruit, which commonly does not have industrial use despite the high content of bioactive compounds (Adilah et al., Citation2018). Furthermore, avocado peels are known for their antioxidant and antimicrobial properties. It contains a high content of compounds, including flavonoids, phenolic acids, organic acids, lignans, and fatty acids (Ramos-Aguilar et al., Citation2021; Salazar-López et al., Citation2020). The coconut shells and husks represent a source of fiber and many phenolic compounds (Tanwar et al., Citation2021). The phenolic compounds in coconut endocarp are due to the presence of quercetin, kaempferol, chlorogenic acid, catechin, and epicatechin (N. Li et al., Citation2021). Several authors indicated that phenolic compounds have antioxidant, antiradical, antibacterial, and antifungal activity (Christ-Ribeiro et al., Citation2019; Liu et al., Citation2021; Mekam et al., Citation2019; Rashed et al., Citation2014; Sagdic et al., Citation2011).
However, the avocado epicarp and coconut endocarp extracts have not been incorporated in a biopolymeric matrix as an alternative for the conservation of strawberry fruit against phytopathogens. Thus, the objective of this work was to develop gelatin-carboxymethylcellulose coatings incorporated with extracts of avocado epicarp and coconut endocarp applied on strawberry fruit to extend their shelf life. Moreover, to determine their application were investigated; a) the postharvest behavior of coated strawberry through compositional changes during storage, and b) the effects of coatings on controlling fungal development in strawberry fruit using R. stolonifer and A. niger strains.
2. Materials and methods
2.1. Obtaining plant extracts
Avocado (Persea americana Mill.) and coconut (Cocos nucifera) fruit were collected from a local market in Texcoco, Mexico at consumption maturity. Avocado epicarp extract (AEE) and coconut endocarp extract (CEE) were obtained according to Aguilar-Méndez et al. (Citation2020), with some modifications. The avocado epicarp and coconut endocarp were dried separately in a digital oven (Fe-294AD Feligneo, México) at 40 ± 2°C. The dried product was pulverized in a disc mill (148–2, The Bauer Co., USA) and sieved through 250 μm stainless steel mesh (Montinox, Montiel Inoxidables, Mexico). Dry samples of 12.5 g avocado epicarp and 15 g coconut endocarp were weighed and placed in a 500 mL mixture of water:ethanol at ratios of 7:3, 3:7, respectively. After sonication in water for 60 min (TI-H-5, Elma, Germany), at a frequency of 45 kHz, during at 65°C. Then, the samples were centrifuged at 1750 rpm (K International, Waukegan, USA), for 15 min and filtered on Whatman paper No. 3 (Merck, Kenilworth, NJ, USA). The extracts were concentrated on a rotary evaporator at 45°C for 4 h (RE-500; Yamato Japan), and were lyophilized (FreeZone 4.5 Freeze Dry System, Labconco, USA) and stored in an amber flask until use.
2.2. Preparation of biopolymer solutions
A solution consisting of type B gelatin, CMC (both purchased) from Sigma-Aldrich (USA), and glycerol (Merk Inc., Germany) each at 0.5% (w/v) was prepared using 125 mL water with stirring at 90 ± 2°C at 600 rpm for 30 min on a grill (Thermlyne, lowa, USA). Three solutions were prepared: The first was based on gelatin (Gel) and CMC (Gel/CMC) and was kept without any additional additives; the second had AEE added at 200 mg/L (Gel/CMC/AEE); and the third had CEE added at 200 mg/L concentration (Gel/CMC/CEE).
2.3. Application of polymeric solutions in fruit
Fresh strawberries were purchased from a local market in Texcoco, Mexico. Strawberries of uniform size, shape and colour, without any signs of mechanical damage or fungal decay, were selected for this study. The strawberries were washed with water, sanitized by immersion in a 1% (w/w) sodium hypochlorite solution for 1 min, and rinsed with distilled water. Four batches of strawberries were created to study the solutions on the fruit, the first one was handled with any treatment and labelled as no covering (NC) and the three ones were treated with biopolymers: Gel/CMC, Gel/CMC/AEE, and Gel/CMC/CEE. The polymer solutions were applied to the fruit with an immersion method and exposure to airflow from a fan (OBF851-LA013, Oster, China) operating at 300 rpm for 20 min to dry the surface at room temperature. The material from each group was organized in batches of 15 fruit. These were placed at 2 ± 1°C and 93% relative humidity in open polyethylene terephthalate containers with a capacity of 1 L. Each treatment was performed in triplicate, and the evaluations were performed on 0, 3, 6, 9, 12, and 15 days of storage.
2.3.1. Weight loss
All treatments were weighed at the beginning (0 d) and upon removal from storage with an analytical balance (Ax224, Ohaus, Taiwan) with a precision of 0.0001 g. According to the initial weight, the cumulative weight loss in percentage was determined based on an AOAC (Citation1994).
2.3.2. Firmness
The firmness was determined in three points of the fruit with a texture analyzer TA-XT2i (Stable Micro Systems, England). The firmness was expressed in Newtons (N) as the average of all the measurements.
2.3.3. Total soluble solids (°Brix), reducing sugars, and titratable acidity (TA)
Total soluble solids (TSS) were expressed as percentages and measured with a refractometer (LH-T50, Lohand Biological, China) in a drop of fruit juice. Reducing sugar content was measured by the Lane-Eynon method using the procedure described by AOAC (Citation1998). Titratable acidity (TA) was determined by taking 5 mL samples of juice and titrated with 0.1 N NaOH using phenolphthalein as an indicator. The results were expressed as a percentage of citric acid.
2.3.4. Color index
Color measurements were recorded using a high-resolution colorimeter (NH300, China) for each treatment and storage time. Values of a* (greenness/redness) and b* (blue/yellowish) were recorded to calculate chromaticity (C*) and tone angle (Hue) according to the following EquationEquations (1)(1)
(1) and (Equation2
(2)
(2) ):
A blank was taken as standard L* = 94.512, a* = 0.417, and b* = 0.350.
2.3.5. Fungal growth analysis
2.3.5.1. Molds
R. stolonifer and A. niger were isolated from strawberry fruit and placed in potato-dextrose-agar (PDA) culture medium at 24°C. An aqueous 0.5% Tween 80 solution was prepared from which 9 mL were taken and mixed with 1 mL inoculum of each strain. Successive dilutions were made in the range 102 to 107 spores/mL. The solutions were adjusted with 0.5% Tween-80 at 106 spores/mL.
2.3.5.2. Strawberry inoculation and coating
Strawberry inoculation and coating were performed as reported by Park et al. (Citation2005) with some modifications. Five fruits were used per treatment. These were free of defects and had a uniform color. The samples were disinfected with a 1% sodium hypochlorite solution for 1 min with two rinses with sterile water followed by a forced air dry (OBF851-LA013, Oster, China) at 300 rpm for 20 min at room temperature. Inoculations were performed by spraying 0.5 mL of 106 mL/spore of R. stolonifer or A. niger solution and subsequent drying at room temperature for 20 min. The strawberries were then immersed in coating solutions (NC, Gel/CMC, Gel/CMC/AEE, Gel/CMC/CEE) for 60s under a hood and with forced air again as shown in . All batches were kept at 2°C for 15 days. The fungal development (%) was evaluated at intervals of five days inspected daily during the storage period. Strawberry fruit showing surface mycelial development were considered decayed (EquationEquation 3(3)
(3) ).
Figure 1. Schematic illustration of the mold inoculation process in strawberries with no covering (NC), gelatin-carboxymethylcellulose coating (Gel/CMC), gelatin-carboxymethylcellulose-avocado epicarp extract coating (Gel/CMC/AEE) and gelatin-carboxymethylcellulose- coconut endocarp extract coating (Gel/CMC/CEE) (coconut endocarp extract) stored at 2°C for 15 days.
Figura 1. Ilustración esquemática del proceso de inoculación de hongos en fresas sin cobertura (NC), recubrimiento de gelatina-carboximetilcelulosa (Gel/CMC), recubrimiento de gelatina-carboximetilcelulosa-extracto de epicarpio de aguacate (Gel/CMC/AEE) y recubrimiento de gelatina-carboximetilcelulosa-extracto de endocarpio de coco (Gel/CMC/CEE) almacenadas a 2°C durante 15 días.
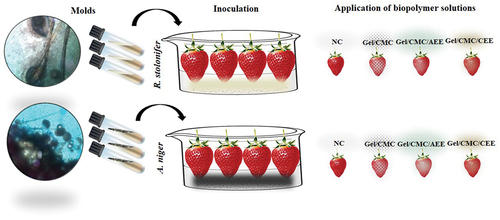
2.4. Statistical analysis
Evaluation of compositional changes was performed as a 4 × 6 factorial arrangement in a completely randomized design. One factor was the treatment to which the fruit were subjected (NC, Gel/CMC, Gel/CMC/AEE, Gel/CMC/CEE). The other was time with six levels (days of storage). The evaluation phase of the fungal growth of extracts was carried out as a 2 × 4 factorial arrangement housed in a completely randomized design where one of the factors was inoculation (fruit inoculated with R. stolonifer and A. niger) and the other variable was type of coating applied. Analysis of variance and comparison of treatment means were conducted with Tukey’s test with a significance level of 0.05. All evaluations were performed in triplicate and analysis was performed using Minitab 7.0 program.
3. Results and discussion
3.1. Weight loss
The fruit of all treatments experienced significant and continuous weight loss during storage (P < .05), as shown in . The greatest loss occurred in NC fruit at a rate of 15.71% d−1, which contrasted with the rates of 7.22%, 2.73%, and 3.49% d−1 observed in the fruit of Gel/CMC, Gel/CMC/AEE, and Gel/CMC/CEE, respectively, with a significant difference among groups. At the end of each storage, the weight loss was 94.7% in NC on day 6, 84.7% in Gel/CMC on day 12, 39.1% in Gel/CMC/AEE on day 15, and 52.2% in Gel/CMC/CEE on day 15. Coating with AEE was more effective in reducing weight loss than the covering incorporated with CEE. This difference between extracts could be since the avocado peel has a greater number of phenolic compounds, in addition to a natural antifungal compound called persin (Bowen et al., Citation2018; Salazar-López et al., Citation2020).
Figure 2. Effect of treatments: strawberries with no covering (NC), gelatin-carboxymethylcellulose coating (Gel/CMC), gelatin-carboxymethylcellulose-avocado epicarp extract coating (Gel/CMC/AEE) and gelatin-carboxymethylcellulose- coconut endocarp extract coating (Gel/CMC/CEE) (coconut endocarp extract) on (A) Cumulative weight loss (B) Firmness of strawberries stored at 2°C for 15 days. The error bars represent standard deviations of triplicate assays with the confidence interval of 95%.
Figura 2. Efecto de los tratamientos: fresas sin cobertura (NC), gelatina-carboximetilcelulosa (Gel/CMC), gelatina-carboximetilcelulosa-extracto de epicarpio de aguacate (Gel/CMC/AEE) y gelatina-carboximetilcelulosa-extracto de endocarpio de coco (Gel/CMC/CEE) (A) Pérdida de peso acumulada (B) Firmeza de fresas almacenadas a 2°C durante 15 días. Las barras de error representan desviaciones estándar de ensayos por triplicado con el intervalo de confianza del 95%.
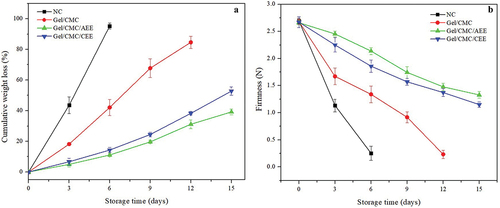
Weight loss increased considerably as storage time increases, and as expected, the greatest weight loss occurred in untreated fruit (NC). Conversely, a reduction in weight loss was observed in the Gel/CMC treatment as in the strawberries coated with AEE and CEE. This reduction in weight loss in the fruit with coatings could be caused by the formation of a semi-permeable and protective layer that could lead to a lower respiration rate. Consequently, this led to a lower production of CO2 and delayed water vapor transfer thus prolonging the commercial life of strawberries by 5 days. The permeability and the barrier against gases (O2 and CO2) in biopolymeric coatings depend directly on storage conditions such as relative humidity and temperature (Hernández-Muñoz et al., Citation2008). Physiological weight loss is a common phenomenon in fruit caused mainly by transpiration (evaporation of moisture through the skin) due to a vapor pressure deficit (dependent on the gradient between the fruit tissue/atmosphere/temperature; Hübert & Lang, Citation2012). Therefore, incorporating AEE and CEE in the hydrophilic biopolymeric matrix reduces the permeability to water leading to less water loss during storage. This is demonstrated by the reduction in weight loss in coated fruit versus NC fruit. Muley and Singhal (Citation2020) observed the same trend in strawberries covered with a layer of chitosan and isolated whey protein. This phenomenon correlated a gradual weight loss with storage time as a function of moisture loss and respiration in strawberries.
Another factor that promotes weight loss is fungal development. Microorganisms accelerate the glycolysis process, which leads to a loss of carbohydrate reserves in the fruit (Sogvar et al., Citation2016). Additionally, fungal infections in NC and Gel/CMC batches leads to a physiological imbalance that quickly results in natural senescence of the fruit, thus causing a change in color (cell necrosis), tissue degradation, and the appearance of physical lesions associated with the colonization of microorganisms. These microorganisms induce morphological changes, structural alterations, and disorganization at the molecular level (Valenzuela et al., Citation2015). Consequently, Ventura-Aguilar et al. (Citation2018) demonstrated that the conservation of strawberries with Hibiscus sabdariffa extracts in combination with the essential oil of cinnamon and chitosan on the growth of Colletotrichum fragariae sustains high effectiveness of natural extracts for microbial control. In summary, the incorporation of AEE and CEE in the matrix Gel/CMC, decreased the water vapor transfer in the fruit and significantly reduced the fungal development thus slowing glycolysis and preserving the integrity of tissues and allowing less loss of accumulated weight.
3.2. Firmness
Continuous loss of fruit firmness also occurred in all treatments with an average rate of 0.41, 0.19, 0.09, and 0.10 N d−1 in NC, Gel/CMC, Gel/CMC/AEE, and Gel/CMC/CEE, respectively; the difference was significant among all treatments (). The initial firmness was 2.68 N, and softening occurred up to 0.25 N in NC, 0.23 N in Gel/CMC, 1.33 in Gel/CMC/AEE, and 1.15 in Gel/CMC/CEE at the end of the storage. The NC fruit could only be handled for 6 days in storage. At that time, they had lost 90.5% of their consistency, which contrasted with the fruit handled with the Gel/CMC coating that had firmness reduced by 50.7%. Those treated with Gel/CMC/AEE and Gel/CMC/CEE had firmness reduced by 20.4 and 30.9% on day 6, respectively. After 15 days of storage, the fruit treated with Gel/CMC/AEE and Gel/CMC/CEE presented 50.7 and 57.0% loss of consistency in their tissues, which was less than what occurred in the fruit of the Gel/CMC treatment at 12 days of storage. These results indicate that the most effective treatment to maintain firmness was the one that incorporated AEE followed by the one that used CEE followed by the one that was based only on the Gel/CMC coating. These results could be since the plant extracts reduced the proliferation of fungi. Likewise, they kept the walls of the plant tissue of the strawberries stable and without deterioration. Similarly, Nasrin et al. (Citation2017) pointed out a reduction of firmness effect in strawberries coated with aloe vera.
The change in firmness is generally associated with the deterioration rate that indicates the loss of consistency of the fruit tissues and favors microbial development. Softening is a phenomenon that generally accompanies the ripening of fruit, but it develops even in the senescence phase due to degradation by depolymerization of cell walls (Posé et al., Citation2019; Prasanna et al., Citation2007). In particular, the breaking of the pectin chains is a critical factor in the changes in the texture of strawberry fruit (Wang et al., Citation2018).
In addition to firmness, another essential characteristic that determines the postharvest handling period is fungal development. The cell wall constitutes a barrier for the access of fungal species. Furthermore, the cell wall allows the release of oligalacturonide fragments with a degree of polymerization between 10 and 15 units of the pectin chains that exert an antimicrobial defense activity (Osorio et al., Citation2008). However, in the presence of pectin, fungal species such as A. niger favor the production of enzymes that cause the breaking of the pectin chains into galacturonic acid units that in turn lead to degradation of the cell wall and favor greater fungal development (Q. Li et al., Citation2020). Therefore, the presence of the antimicrobial properties of the natural extracts and the development of a physical barrier through the biopolymer matrix delayed softening and resulted in a longer shelf life.
These results confirm that the Gel/CMC coatings incorporated with AEE and CEE could improve the preservation characteristics of the fruit more effectively than the fruit that only have the Gel/CMC coating and NC fruit through two mechanisms as mentioned earlier: 1) The retardation of the process of rot prevents the development of fungal species in strawberries; and 2) the reduction in the rate of degradation of cell walls due to the formation of a physical barrier, which makes pectin inaccessible for its hydrolysis by the action of fungal species.
3.3. Total soluble solids (°Brix), reducing sugars and titratable acidity (TA)
The initial TSS content was 7.99 °Brix. During storage, the TSS increased in the fruit for all treatments (). After 3 days, the content was similar across all groups with a value of 8.20 °Brix. Subsequently, in fruit treated with Gel/CMC, the TSS value increased to 9.31 °Brix, and the contrast was significant among fruit of different treatments. At the end of storage, the TSS content was 8.97 °Brix in Gel/CMC (day 9), 9.10 °Brix in Gel/CMC/AEE (day 15), and 8.55 °Brix in Gel/CMC/CEE (day 15). That is, the fruit with coatings incorporated with AEE followed by the CEE coatings were those that reached the lowest levels of TSS versus NC fruit.
Table 1. Soluble solids, reducing sugars, titratable acidity of no covering (NC) and coated strawberries (Gel/CMC, Gel/CMC/AEE and Gel/CMC/CEE) stored at 2°C.
Tabla 1. Sólidos solubles, azúcares reductores, acidez titulable de fresas no recubiertas (NC) y recubiertas (Gel/CMC, Gel/CMC/AEE y Gel/CMC/CEE) almacenadas a 2°C
The TSS content is mainly determined by the presence of sugars (Nunes et al., Citation2006). Thus, the reducing sugar content follows the trend observed in the TSS results. The reducing sugar content of the fruit at the beginning of storage was 3.48%, and the behavior of the treatment (NC) group showed a moderate increase in these compounds to 3.7% on day 3 and then a significant increase to 4.86% on day 6. The Gel/CMC, Gel/CMC/AEE, and Gel/CMC/CEE groups had a decrease in the content of reducing sugars between the beginning and 3 days to 3.04% with no difference between them. There was then a continuous increase to 4.50%, 4.55%, and 4.94%, respectively, at the end of each storage (). These results show that incorporating AEE and CEE in the Gel/CMC matrix modifies the consumption of sugars in the fruit versus NC fruit. This change can be observed in two stages. In the first stage, the fruit coated with Gel/CMC had an increase in reducing sugar consumption in the initial storage phase versus NC fruit. As a result of the application of the biopolymers, the barrier properties formed by the external tissues were modified including the cuticle and the biopolymeric gas layer of the fruit shell (De Medeiros et al., Citation2012). This modification of the barrier properties limited the supply of oxygen, and consequently, the supply of energy for the metabolic functions had to be evaluated with ATP obtained from oxidative phosphorylation. Finally, to address the deficiency through this metabolic process, it is necessary to increase the energy obtained from glycolysis for which the consumption of sugars as a substrate had to be increased. Therefore, a higher consumption of sugars will be observed in coated fruit as a substrate of the respiratory process (Chu et al., Citation2020). These results were similar to Quintana et al. (Citation2021) in strawberries covered with chitosan and natural extracts/essential oils from different plants. The increase in the TSS was due to the metabolic process (converting starch and acids into sugar) during postharvest storage.
In the second stage starting from day 3 of storage, an increase was observed in the content of reducing sugars in the fruit treated with Gel/CMC, Gel/CMC/AEE, and Gel/CMC/CEE. This trend was caused by the solubilization of compounds and the breakdown of polymers of the cell walls. However, the TSS values in coated fruit reached their maximum at a lower rate at the end of storage. This behavior occurs because the coatings can reduce the respiration of the fruit and delay its ripening. Thus, the permeability of CO2 and O2 can be controlled by the coatings and slow down the ripening of the fruit because both gases are produced due to the degradation of sugars (Pinzon et al., Citation2020; Robles-Flores et al., Citation2018). Dong and Wang (Citation2017) have reported similar findings on coatings of CMC and garlic EO´s to preserve strawberries. The study pointed out that the gradual change in TSS content could be due to the deceleration of metabolic activity. Consequently, as mentioned above, a decrease in respiration, likewise, the loss of water in the fruit could cause changes in the TSS content and, at the same time, less fungal growth due to the lack of glucose.
On the other hand, fruit of all treatments had a titratable acidity (TA) of 0.99% at the beginning of storage, and this parameter decreased up to 0.56% in NC after storage of day 6, 0.61% in Gel/CMC at the end of storage of day 9, 0.59% in Gel/CMC/AEE, and 0.45% in Gel/CMC/CEE upon completion of the day 15 storage with modification rates of 0.093, 0.067, 0.039 and 0.033% d−1, respectively (). In general, the fruit treated with Gel/CMC/AEE and Gel/CMC/CEE were similar in most of the storage, except at the end when a significant difference was observed in the latter. The coated fruit with Gel/CMC/AEE and Gel/CMC/CEE reduced the rate of consumption of organic acids. The metabolism modification affects the fruit acidity.
Perdones et al. (Citation2012) have also reported a decrease in TA values attributed to over-ripening and rotting in strawberries treated with a chitosan matrix with lemon EO’s. Therefore, the acidity decreases due to the consumption of organic acids during respiration at storage (Riaz et al., Citation2021). According to Nunes et al. (Citation2006), the increase in TSS and the decrease in titratable acidity are associated with ripening. However, once this physiological process is completed, the modification of TSS is negligible and is attributed to the solubilization of polymers of the cell wall, which is consistent with the changes seen for firmness. These authors also showed that acidity could be expressed as a percentage of citric acid because it has the highest presence in strawberry fruit, and the content can decrease slightly or remain unchanged during postharvest storage. In conclusion, as mentioned earlier, the Gel/CMC coating incorporated with AEE and CEE could modify the barrier properties in consecutive stages: i) they increase the consumption of sugars in the short term, and ii) they reduce the permeability of gases and slow down the maturation and the consumption of organic acids. Thus, AEE and CEE incorporated in Gel/CMC coatings slow down the ripening of strawberries and, consequently reduce compositional changes.
3.4. Index color
Color undergoes some remarkable changes as fruit ages. It markedly impacts consumer acceptance. shows the color changes of the fruit with different treatments during storage (2°C). These values are denoted as colorimetric changes measured directly from the fruit tissue: chroma and tone angle.
Figure 3. External color evolution and colorimetric change (A) Chroma (B) Hue angle of no covering strawberries (NC), gelatin-carboxymethylcellulose-avocado epicarp extract coating (Gel/CMC/AEE) and gelatin-carboxymethylcellulose- coconut endocarp extract coating (Gel/CMC/CEE) (coconut endocarp extract) stored at 2°C. Vertical bars indicate standard deviation.
Figura 3. Evolución del color externo y cambio colorimétrico (A) Croma (B) Ángulo de tono de fresas sin recubrimiento (NC), gelatina-carboximetilcelulosa (Gel/CMC), gelatina-carboximetilcelulosa-extracto de epicarpio de aguacate (Gel/CMC/AEE) y gelatina-carboximetilcelulosa-extracto de endocarpio de coco (Gel/CMC/CEE) almacenadas a 2°C. Las barras verticales indican la desviación estándar.
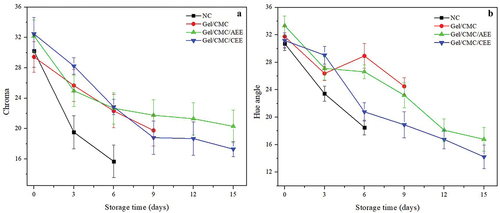
Chroma results are shown in . Significant changes (P < .05) in the chromatic value were observed between the fruit treated with NC and Gel/CMC compared to those incorporated with plant extracts on 3 and 6 days. However, throughout storage, all treatments exhibited coloration with less intensity (less vivid) as indicated by the chroma values, although the highest values were from the Gel/CMC/AEE and Gel/CMC/CEE treatments at the end of the study without significant differences between them. The chroma was reduced for Gel/CMC by around 33%. It was 20–25% for Gel/CMC/AEE and Gel/CMC/CEE treatments. The change in coloration is mainly due to the loss of water (transpiration). This phenomenon quickly leads to tissue degradation, causing an alteration of color in the fruit (senescence; Perdones et al., Citation2012). Therefore, coatings with plant extracts significantly decreased the rate of color change by avoiding tissue degradation.
The tone angle values are shown in . There were significant differences between the NC treatment and the rest of the treatments during storage. The change in hue, darkening, and oxidation of the cuticle was perceived on a different scale in all treatments. These changes may be symptoms of excessive maturity and depolymerization of tissues that occur during transpiration (dehydration) and senescence caused by the loss of intracellular fluids and tissue collapse (cell death; Hernández-Muñoz et al., Citation2008; Velickova et al., Citation2013). In addition, Soazo et al. (Citation2015) have found similar behavior in color variability and suggest that this phenomenon is due to the content of anthocyanins, which are not chemically stable in strawberries coated with whey protein-based matrix.
Consequently, as mentioned before, the coatings incorporated with AEE and CEE could protect the fruit from cuticle oxidation caused by excessive maturity by reducing transpiration and tissue collapse. These materials offer better coloration with only minor darkening.
3.5. Fungal growth analysis
Fungal development was observed and limited the handling of NC materials to only 6 days. Although the Gel/CMC treatment reduced microbial development, its progress indicated that the useful life of the fruit ended on day 9. This behavior contrasted with the materials treated with AEE and CEE, where handling greater than 15 days was achieved. The development of visible mold as a function of the different treatments are seen in a-b. All treatments had significant differences (P < .05). The coatings significantly decreased mold growth at the end of storage as shown in .
Figure 4. Evolution of fungal development (A) fungal decomposition of fruit inoculated with R. stolonifer (B) fungal decomposition of fruit inoculated with A. niger. Vertical bars indicate standard deviation.
Figura 4. Evolución del desarrollo fúngico (A) Descomposición fúngica de frutos inoculados con R. stolonifer (B) Descomposición fúngica de frutos inoculados con A. niger. Las barras verticales indican la desviación estándar.
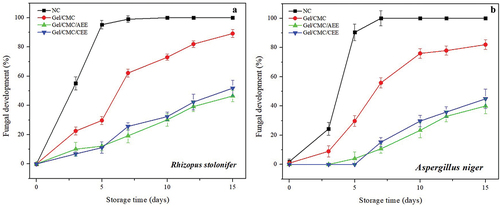
Figure 5. Illustration of the appearance and development of inoculated molds in strawberries stored at 2°C.
Figura 5. Ilustración de la apariencia y desarrollo de hongos inoculados en fresas almacenadas a 2°C.
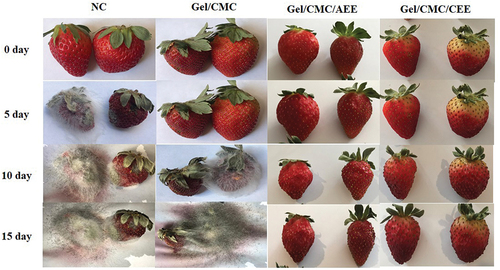
In the NC treatment, mold growth was observed starting at day 3 on fruit inoculated with R. stolonifer and A. niger. As expected, the strawberries showed accelerated fungal growth between 3 and 5 days of storage. Additionally, the refrigeration system to which they were subjected did not slow down the deterioration of the fruit (). Besides, the turgid, juicy, and soft texture of the strawberry fruit tissues generates ideal conditions for the growth and proliferation of various microorganisms (Lafarga et al., Citation2019). R. stolonifer is a microorganism that promotes wound diseases after harvest and is known as soft rot. A. niger is the most common species in the Aspergillus genus and causes black mold in fruit and vegetables (Jahani et al., Citation2020). Therefore, the inoculation of both fungi caused accelerated cell death in NC fruit.
The Gel/CMC coating reduced the rotting generated by the inoculation in strawberry fruit. However, the effect was not sufficient, and the rotting reached levels of almost 90% after 10 days of storage. The use of biopolymeric coatings with suitable physical, and microbiological properties has been recognized as an alternative for lengthening the shelf life of fresh vegetable products (Yousuf et al., Citation2018). Thus, the formation of a semi-permeable layer of Gel/CMC decelerated microbial growth in the fruit versus NC and extended the shelf life to 7 days. The use of Gel/CMC/AEE and Gel/CMC/CEE significantly affected fungal development so that it was almost nil at day 5 after the inoculation of molds and varied between 30.15 and 32.39% after day 10; it was located in the range of 41.7 and 44.9% for both molds after day 15. At day 15, the treatment Gel/CMC/AEE caused inhibition but was not significantly higher than the Gel/CMC/CEE.
In addition to the beneficial effect that biopolymeric coatings can generate by themselves, they can also serve as a vehicle for other agents with some specific properties (Chiralt et al., Citation2012). Thus, the direct application of antimicrobial compounds on the surface of food can have a limited effect due to limited diffusion into the product; however, this transport is improved when a coating is used as a vehicle because an effective concentration is maintained on the surface to prevent or reduce microbial development (Yousuf et al., Citation2018).
The antifungal properties of AEE have been explained by the presence of persin (Z, Z-1-acetoxy-2-hydroxy-4-oxoheneicosa-12, 15-diene) in addition to other substances such as epicatechins and other phenolics (Bowen et al., Citation2018). Likewise, it has been determined that the Hass avocado epicarp has a high content of condensable tannins (Jiménez-Velázquez et al., Citation2020) whose antifungal activity has been reported (Abu Zarin et al., Citation2016; Shohayeb et al., Citation2013). Similarly, coconut pericarp contains different types of polyphenols including catechins, epicatechins, kaempferol, condensable tannins (procyanidins B), ferulic acids, p-coumaric, etc. Its extract has been tested as an antimicrobial alternative against E. coli, S. enterica, S. aereus, F. pedrosoi, and R. stolonifer (Esquenazi et al., Citation2002; Sinsinwar et al., Citation2018). The fungicidal action has also been reported to be mediated by the interaction and composition of the different bioactive compounds and metabolites found in the extracts (Da Rocha Neto et al., Citation2019). The effect of natural extracts on the biopolymer matrix reduced the fungal growth caused by the inoculation of R. stolonifer and A. niger. Consequently, the shelf life of strawberries was extended to 15 days.
4. Conclusions
The results showed that the biopolymeric matrix of gelatin and carboxymethylcellulose applied on the surface of strawberries can extend their shelf life for 9 days at 2°C. On the other hand, extracts of avocado epicarp and coconut endocarp incorporated in the biopolymeric matrix extended strawberry fruit shelf life by more than 15 days. In addition, the shelf-life extension was accompanied by a significant delay in weight loss, softening, color behavior, and compositional changes (sugars and titratable acidity) compared with Gel/CMC coated fruit. Furthermore, the matrix incorporated with epicarp and endocarp extracts from avocado fruit (AEE) and coconut (CEE) inhibited the growth of R. stolonifer and A. niger in strawberries throughout storage.
Further research is needed to incorporate a more detailed mechanism through an appropriate experimental model for obtaining plant extracts and incorporating biopolymeric materials of a lipid nature to improve the hydrophilic-lipophilic balance of the coating. Furthermore, a sensory analysis will be carried out with a panel of trained tasters in future experiments. In conclusion, this work gives a new strategy of avocado epicarp, and coconut endocarp utilization as antifungal extracts and their incorporation in a biopolymeric matrix that can extend fruit shelf-life.
Acknowledgments
We thank the Instituto Politécnico Nacional (SIP Project: 20181062 and 20210011), for funding this work. Vargas-Torrico thanks the Consejo Nacional de Ciencia y Tecnología (CONACYT) and the Comisión de Operación y Fomento de Actividades Académicas del IPN (COFAA) for the scholarships granted.
Disclosure statement
No potential conflict of interest was reported by the author(s).
References
- Abdallah, M. R., Mohamed, M. A., Mohamed, H., & Emara, M. T. (2018). Application of alginate and gelatin-based edible coating materials as alternatives to traditional coating for improving the quality of pastirma. Food Science and Biotechnology, 27(6), 1589–1597. https://doi.org/10.1007/s10068-018-0393-2
- Abdollahzadeh, E., Nematollahi, A., & Hosseini, H. (2021). Composition of antimicrobial edible films and methods for assessing their antimicrobial activity: A review. Trends in Food Science & Technology, 110, 291–303. https://doi.org/10.1016/j.tifs.2021.01.084
- Abu Zarin, M., Wan, H. Y., Isha, A., & Armania, N. (2016). Antioxidant, antimicrobial and cytotoxic potential of condensed tannins from Leucaena leucocephala hybrid-Rendang. Food Science and Human Wellness, 5(2) , 65–75. https://doi.org/10.1016/j.fshw.2016.02.001
- Adilah, A. N., Jamilah, B., Noranizan, M. A., & Hanani, Z. A. N. (2018). Utilization of Mango peel extracts on the biodegradable films for active packaging. Food Packaging and Shelf Life, 16, 1–7. https://doi.org/10.1016/j.fpsl.2018.01.006
- Aguilar-Méndez, M. A., Campos-Arias, M. P., Quiroz-Reyes, C. N., Ronquillo-de Jesús, E., & Cruz-Hernández. (2020). Fruit peels as sources of bioactive compounds with antioxidant and antimicrobial properties. Revista de La Facultad de Ciencias Agrarias UNCuyo, 52(1) , 360–371. https://revistas.uncu.edu.ar/ojs/index.php/RFCA/article/view/2945
- Anami, J. M., Steffens, C. A., Moreira, M. A., Fernandes, R. C., Mosquera, D. J. C., & Amarante, C. V. T. (2020). Active modified atmosphere storage to preserve the quality of’San Andreas’ strawberries harvest at two ripening stages. Revista Brasileira de Fruticultura, 42(1). https://doi.org/10.1590/0100-29452020099
- AOAC. (1994). Official methods of Analysis of Association of Official Analytical Chemists (AOAC) International. 16th ed. Method 942.15.
- AOAC. (1998). AOAC peer-verified methods program manual on policies and procedures.
- Bovi, G. G., Rux, G., Caleb, O. J., Herppich, W. B., Linke, M., Rauh, C., & Mahajan, P. V. (2018). Measurement and modelling of transpiration losses in packaged and unpackaged strawberries. Biosystems Engineering, 174, 1–9. https://doi.org/10.1016/j.biosystemseng.2018.06.012
- Bowen, J., Billing, D., Connolly, P., Smith, W., Cooney, J., & Burdon, J. (2018). Maturity, storage and ripening effects on anti-fungal compounds in the skin of ‘Hass’ avocado fruit. Postharvest Biology and Technology, 146, 43–50. https://doi.org/10.1016/j.postharvbio.2018.08.005
- Campos-Requena, V. H., Rivas, B. L., Pérez, M. A., Figueroa, C. R., Figueroa, N. E., & Sanfuentes, E. A. (2017). Thermoplastic starch/clay nanocomposites loaded with essential oil constituents as packaging for strawberries − In vivo antimicrobial synergy over Botrytis cinerea. Postharvest Biology and Technology, 129, 29–36. https://doi.org/10.1016/j.postharvbio.2017.03.005
- Cardoso, G. P., Dutra, M. P., Fontes, P. R., Ramos, A. D. L. S., Gomide, L. A. D. M., & Ramos, E. M. (2016). Selection of a chitosan gelatin-based edible coating for color preservation of beef in retail display. Meat Science, 114, 85–94. https://doi.org/10.1016/j.meatsci.2015.12.012
- Chiralt, A., Fabra, M., & Sánchez-González. (2012). Propiedades de las películas comestibles. Películas Y Recubrimientos Comestibles: Propiedades Y Aplicaciones En Alimentos, 21–57. https://scholar.google.com.mx/scholar?hl=es&as_sdt=0%2C5&q=Propiedades+de+las+pel%C3%ADculas+comestibles+chiralt+&btnG=
- Christ-Ribeiro, A., Graça, C. S., Kupski, L., Badiale-Furlong, E., & de Souza-soares, L. A. (2019). Cytotoxicity, antifungal and anti mycotoxins effects of phenolic compounds from fermented rice bran and Spirulina sp. Process Biochemistry, 80, 190–196. https://doi.org/10.1016/j.procbio.2019.02.007
- Chu, Y., Gao, C., Liu, X., Zhang, N., Xu, T., Feng, X., Yang, Y., Shen, X., & Tang, X. (2020). Improvement of storage quality of strawberries by pullulan coatings incorporated with cinnamon essential oil nanoemulsion. LWT, 122, 109054. https://doi.org/10.1016/j.lwt.2020.109054
- Da Rocha Neto, A. C., Navarro, B. B., Canton, L., Maraschin, M., & Di Piero, R. M. (2019). Antifungal activity of palmarosa (Cymbopogon martinii), tea tree (Melaleuca alternifolia) and star anise (Illicium verum) essential oils against Penicillium expansum and their mechanisms of action. LWT, 105, 385–392. https://doi.org/10.1016/j.lwt.2019.02.060
- de Medeiros, B. G. S., Pinheiro, A. C., Carneiro-da-cunha, M. G., & Vicente, A. A. (2012). Development and characterization of a nanomultilayer coating of pectin and chitosan – Evaluation of its gas barrier properties and application on ‘Tommy Atkins’ mangoes. Journal of Food Engineering, 110(3), 457–464. https://doi.org/10.1016/j.jfoodeng.2011.12.021
- de Souza, W. F. C., de Lucena, F. A., Da Silva, K. G., Martins, L. P., de Castro, R. J. S., & Sato, H. H. (2021). Influence of edible coatings composed of alginate, galactomannans, cashew gum, and gelatin on the shelf- life of grape cultivar ‘Italia’: Physicochemical and bioactive properties. LWT, 152, 112315. https://doi.org/10.1016/j.lwt.2021.112315
- Del Castillo-Llamosas, A., Rodríguez-Martínez, B., Del Río, P. G., Eibes, G., Garrote, G., & Gullón, B. (2021). Hydrothermal treatment of avocado peel waste for the simultaneous recovery of oligosaccharides and antioxidant phenolics. Bioresource Technology, 342, 125981. https://doi.org/10.1016/j.biortech.2021.125981
- Dong, F., & Wang, X. (2017). Effects of carboxymethyl cellulose incorporated with garlic essential oil composite coatings for improving quality of strawberries. International Journal of Biological Macromolecules, 104, 821–826. https://doi.org/10.1016/j.ijbiomac.2017.06.091
- Esquenazi, D., Wigg, M. D., Miranda, M. M. F. S., Rodrigues, H. M., Tostes, J. B. F., Rozental, S., Da Silva, A. J. R., & Alviano, C. S. (2002). Antimicrobial and antiviral activities of polyphenolics from Cocos nucifera Linn. (Palmae) husk fiber extract. Research in Microbiology, 153(10), 647–652. https://doi.org/10.1016/S0923-2508(02)01377-3
- Esteghlal, S., Niakousari, M., & Hosseini, S. M. H. (2018). Physical and mechanical properties of gelatin-CMC composite films under the influence of electrostatic interactions. International Journal of Biological Macromolecules, 114, 1–9. https://doi.org/10.1016/j.ijbiomac.2018.03.079
- Etxabide, A., Uranga, J., Guerrero, P., & de La Caba, K. (2017). Development of active gelatin films by means of valorisation of food processing waste: A review. Food Hydrocolloids, 68, 192–198. https://doi.org/10.1016/j.foodhyd.2016.08.021
- Friedrich, J. C. C., Silva, O. A., Faria, M. G. I., Colauto, N. B., Gazzin, Z. C., Colauto, G. A. L., Caetano, J., & Dragunski, D. C. (2020). Improved antioxidant activity of a starch and gelatin-based biodegradable coating containing Tetradenia riparia extract. International Journal of Biological Macromolecules, 165, 1038–1046. https://doi.org/10.1016/j.ijbiomac.2020.09.143
- Giannoglou, M., Xanthou, Z.-M., Chanioti, S., Stergiou, P., Christopoulos, M., Dimitrakellis, P., Efthimiadou, Α., Gogolides, Ε., & Katsaros, G. (2021). Effect of cold atmospheric plasma and pulsed electromagnetic fields on strawberry quality and shelf-life. Innovative Food Science & Emerging Technologies, 68, 102631. https://doi.org/10.1016/j.ifset.2021.102631
- Gómez-Guillén, M. C., Giménez, B., López-Caballero, M. E., & Montero, M. P. (2011). Functional and bioactive properties of collagen and gelatin from alternative sources: A review. Food Hydrocolloids, 25(8), 1813–1827. https://doi.org/10.1016/j.foodhyd.2011.02.007
- Hajji, S., Kchaou, H., Bkhairia, I., Ben Slama-Ben Salem, R., Boufi, S., Debeaufort, F., & Nasri, M. (2021). Conception of active food packaging films based on crab chitosan and gelatin enriched with crustacean protein hydrolysates with improved functional and biological properties. Food Hydrocolloids, 116, 106639. https://doi.org/10.1016/j.foodhyd.2021.106639
- Hasheminejad, N., & Khodaiyan, F. (2020). The effect of clove essential oil loaded chitosan nanoparticles on the shelf life and quality of pomegranate arils. Food Chemistry, 309, 125520. https://doi.org/10.1016/j.foodchem.2019.125520
- Hernández-Muñoz, P., Almenar, E., Valle, V. D., Velez, D., & Gavara, R. (2008). Effect of chitosan coating combined with postharvest calcium treatment on strawberry (Fragaria×ananassa) quality during refrigerated storage. Food Chemistry, 110(2) , 428–435. https://doi.org/10.1016/j.foodchem.2008.02.020
- Hübert, T., & Lang, C. (2012). Artificial fruit: Postharvest online monitoring of agricultural food by measuring humidity and temperature. International Journal of Thermophysics, 33(8–9), 1606–1615. https://doi.org/10.1007/s10765-011-1101-0
- Jahani, M., Pira, M., & Aminifard, M. H. (2020). Antifungal effects of essential oils against Aspergillus Niger in vitro and in vivo on pomegranate (Punica granatum) fruits. Scientia Horticulturae, 264, 109188. https://doi.org/10.1016/j.scienta.2020.109188
- Janisiewicz, W., Takeda, F., Evans, B., & Camp, M. (2021). Potential of far ultraviolet (UV) 222 nm light for management of strawberry fungal pathogens. Crop Protection, 150. https://doi.org/10.1016/j.cropro.2021.105791
- Jiang, J., Zhang, M., Devahastin, S., & Yu, D. (2021). Effect of ultrasound-assisted osmotic dehydration pretreatments on drying and quality characteristics of pulsed fluidized bed microwave freeze-dried strawberries. LWT, 145, 111300. https://doi.org/10.1016/j.lwt.2021.111300
- Jiménez-Velázquez, P., Valle-Guadarrama, S., Alia-Tejacal, I., Salinas-Moreno, Y., García-Cruz, L., Pérez-López, A., & Guerra-Ramírez, D. (2020). Separation of bioactive compounds from epicarp of ‘Hass’ avocado fruit through aqueous two-phase systems. Food and Bioproducts Processing, 123, 238–250. https://doi.org/10.1016/j.fbp.2020.07.004
- Khedri, S., Sadeghi, E., Rouhi, M., Delshadian, Z., Mortazavian, A. M., de Toledo Guimarães, J., Fallah, M., & Mohammadi, R. (2021). Bioactive edible films: Development and characterization of gelatin edible films incorporated with casein phosphopeptides. LWT, 138, 110649. https://doi.org/10.1016/j.lwt.2020.110649
- Khemakhem, I., Abdelhedi, O., Trigui, I., Ayadi, M. A., & Bouaziz, M. (2018). Structural, antioxidant and antibacterial activities of polysaccharides extracted from olive leaves. International Journal of Biological Macromolecules, 106, 425–432. https://doi.org/10.1016/j.ijbiomac.2017.08.037
- Khorram, F., Ramezanian, A., & Hosseini, S. M. H. (2017). Shellac, gelatin and Persian gum as alternative coating for Orange fruit. Scientia Horticulturae, 225, 22–28. https://doi.org/10.1016/j.scienta.2017.06.045
- Kingwascharapong, P., Arisa, K., Karnjanapratum, S., Tanaka, F., & Tanaka, F. (2020). Effect of gelatin-based coating containing frog skin oil on the quality of persimmon and its characteristics. Scientia Horticulturae, 260, 108864. https://doi.org/10.1016/j.scienta.2019.108864
- Lafarga, T., Colás-Medà, P., Abadías, M., Aguiló-Aguayo, I., Bobo, G., & Viñas, I. (2019). Strategies to reduce microbial risk and improve quality of fresh and processed strawberries: A review. Innovative Food Science & Emerging Technologies, 52, 197–212. https://doi.org/10.1016/j.ifset.2018.12.012
- Li, N., Jiang, H., Yang, J., Wang, C., Wu, L., Hao, Y., & Liu, Y. (2021). Characterization of phenolic compounds and anti-acetylcholinase activity of coconut shells. Food Bioscience, 42, 101204. https://doi.org/10.1016/j.fbio.2021.101204
- Li, Q., Ray, C. S., Callow, N. V., Loman, A. A., Islam, S. M. M., & Ju, L.-K. (2020). Aspergillus Niger production of pectinase and α-galactosidase for enzymatic soy processing. Enzyme and Microbial Technology, 134, 109476. https://doi.org/10.1016/j.enzmictec.2019.109476
- Liu, Y., Benohoud, M., Galani Yamdeu, J. H., Gong, Y. Y., & Orfila, C. (2021). Green extraction of polyphenols from citrus peel by-products and their antifungal activity against Aspergillus flavus. Food Chemestry:X, 12, 100144. https://doi.org/10.1016/j.fochx.2021.100144
- López-Palestina, C. U., Aguirre-Mancilla, C. L., Raya-Pérez, J. C., Ramirez-Pimentel, J. G., Vargas-Torres, A., & Hernández-Fuentes, A. D. (2019). Physicochemical and antioxidant properties of gelatin-based films containing oily tomato extract (Solanum lycopersicum L.). CyTA - Journal of Food, 17(1), 142–150. https://doi.org/10.1080/19476337.2018.1564793
- Luo, Q., Hossen, M. A., Zeng, Y., Dai, J., Li, S., Qin, W., & Liu, Y. (2022). Gelatin-based composite films and their application in food packaging: A review. Journal of Food Engineering, 313, 110762. https://doi.org/10.1016/j.jfoodeng.2021.110762
- Mekam, P. N., Martini, S., Nguefack, J., Tagliazucchi, D., & Stefani, E. (2019). Phenolic compounds profile of water and ethanol extracts of Euphorbia hirta L. leaves showing antioxidant and antifungal properties. South African Journal of Botany, 127, 319–332. https://doi.org/10.1016/j.sajb.2019.11.001
- Melgar, B., Dias, M. I., Ciric, A., Sokovic, M., Garcia-Castello, E. M., Rodriguez-Lopez, A. D., Barros, L., & Ferreira, I. C. R. F. (2018). Bioactive characterization of Persea americana Mill. by-products: A rich source of inherent antioxidants. Industrial Crops and Products, 111, 212–218. https://doi.org/10.1016/j.indcrop.2017.10.024
- Mu, C., Guo, J., Li, X., Lin, W., & Li, D. (2012). Preparation and properties of dialdehyde carboxymethyl cellulose crosslinked gelatin edible films. Food Hydrocolloids, 27(1), 22–29. https://doi.org/10.1016/j.foodhyd.2011.09.005
- Muley, A. B., & Singhal, R. S. (2020). Extension of postharvest shelf life of strawberries (Fragaria ananassa) using a coating of chitosan-whey protein isolate conjugate. Food Chemistry, 329, 127213. https://doi.org/10.1016/j.foodchem.2020.127213
- Nagarajan, M., Rajasekaran, B., Benjakul, S., & Venkatachalam, K. (2021). Influence of chitosan-gelatin edible coating incorporated with longkong pericarp extract on refrigerated black tiger Shrimp (Penaeus monodon). Current Research in Food Science, 4, 345–353. https://doi.org/10.1016/j.crfs.2021.05.003
- Nasrin, T. A. A., Rahman, M. A., Hossain, M. A., Islam, M. N., & Arfin, M. S. (2017). Postharvest quality response of strawberries with aloe vera coating during refrigerated storage. The Journal of Horticultural Science and Biotechnology, 92(6), 598–605. https://doi.org/10.1080/14620316.2017.1324326
- Nunes, M. C. N., Brecht, J. K., Morais, A. M., & Sargent, S. A. (2006). Physicochemical changes during strawberry development in the field compared with those that occur in harvested fruit during storage. Journal of Food and Agriculture, 86(2), 180–190. https://doi.org/10.1002/jsfa.2314
- Octavia, L., & Choo, W. S. (2017). Folate, ascorbic acid, anthocyanin and colour changes in strawberry (Fragaria × annanasa) during refrigerated storage. LWT, 86, 652–659. https://doi.org/10.1016/j.lwt.2017.08.049
- Oliveira, J., Parisi, M. C. M., Baggio, J. S., Silva, P. P. M., Paviani, B., Spoto, M. H. F., & Gloria, E. M. (2019). Control of Rhizopus stolonifer in strawberries by the combination of essential oil with carboxymethylcellulose. International Journal of Food Microbiology, 292, 150–158. https://doi.org/10.1016/j.ijfoodmicro.2018.12.014
- Osorio, S., Castillejo, C., Quesada, M. A., Medina‐Escobar, N., Brownsey, G. J., Suau, R., Heredia, A., Botella, M. A., & Valpuesta, V. (2008). Partial demethylation of oligogalacturonides by pectin methyl esterase 1 is required for eliciting defence responses in wild strawberry (Fragaria vesca). The Plant Journal, 54(1), 43–55. https://doi.org/10.1111/j.1365-313X.2007.03398.x
- Panahirad, S., Dadpour, M., Peighambardoust, S. H., Soltanzadeh, M., Gullón, B., Alirezalu, K., & Lorenzo, J. M. (2021). Applications of carboxymethyl cellulose- and pectin-based active edible coatings in preservation of fruits and vegetables: A review. Trends in Food Science & Technology, 110, 663–673. https://doi.org/10.1016/j.tifs.2021.02.025
- Park, S. I., Stan, S. D., Daeschel, M. A., & Zhao, Y. (2005). Antifungal coatings on fresh strawberries (Fragaria× ananassa) to control mold growth during cold storage. Journal of Food Science, 70(4), M202–M207. https://doi.org/10.1111/j.1365-2621.2005.tb07189.x
- Pattanayaiying, R., Sane, A., Photjanataree, P., & Cutter, C. N. (2019). Thermoplastic starch/polybutylene adipate terephthalate film coated with gelatin containing nisin Z and lauric arginate for control of foodborne pathogens associated with chilled and frozen seafood. International Journal of Food Microbiology, 290, 59–67. https://doi.org/10.1016/j.ijfoodmicro.2018.09.015
- Pellá, M. C. G., Silva, O. A., Pellá, M. G., Beneton, A. G., Caetano, J., Simões, M. R., & Dragunski, D. C. (2020). Effect of gelatin and casein additions on starch edible biodegradable films for fruit surface coating. Food Chemistry, 309, 125764. https://doi.org/10.1016/j.foodchem.2019.125764
- Perdones, A., Sánchez-González, L., Chiralt, A., & Vargas, M. (2012). Effect of chitosan–lemon essential oil coatings on storage-keeping quality of strawberry. Postharvest Biology and Technology, 70, 32–41. https://doi.org/10.1016/j.postharvbio.2012.04.002
- Pinzon, M. I., Sanchez, L. T., Garcia, O. R., Gutierrez, R., Luna, J. C., & Villa, C. C. (2020). Increasing shelf life of strawberries (Fragaria ssp) by using a banana starch-chitosan-Aloe vera gel composite edible coating. International Journal of Food Science and Technology, 55(1), 92–98. https://doi.org/10.1111/ijfs.14254
- Posé, S., Paniagua, C., Matas, A. J., Gunning, A. P., Morris, V. J., Quesada, M. A., & Mercado, J. A. (2019). A nanostructural view of the cell wall disassembly process during fruit ripening and postharvest storage by atomic force microscopy. Trends in Food Science & Technology, 87, 47–58. https://doi.org/10.1016/j.tifs.2018.02.011
- Prakash, A., Vadivel, V., Banu, S. F., Nithyanand, P., Lalitha, C., & Brindha, P. (2018). Evaluation of antioxidant and antimicrobial properties of solvent extracts of agro-food by-products (cashew nut shell, coconut shell and groundnut hull). Agriculture and Natural Resources, 52(5), 451–459. https://doi.org/10.1016/j.anres.2018.10.018
- Prasanna, V., Prabha, T., & Tharanathan, R. (2007). Fruit ripening phenomena–an overview. Critical Reviews in Food Science and Nutrition, 47(1), 1–19. https://doi.org/10.1080/10408390600976841
- Quintana, S. E., Llalla, O., García-Risco, M. R., & Fornari, T. (2021). Comparison between essential oils and supercritical extracts into chitosan-based edible coatings on strawberry quality during cold storage. The Journal of Supercritical Fluids, 171, 105198. https://doi.org/10.1016/j.supflu.2021.105198
- Ramos-Aguilar, A. L., Ornelas-Paz, J., Tapia-Vargas, L. M., Gardea-Béjar, A. A., Yahia, E. M., Ornelas-Paz, J. D. J., Ruiz-Cruz, S., Rios-Velasco, C., & Escalante-Minakata, P. (2021). Effect of cultivar on the content of selected phytochemicals in avocado peels. Food Research International, 140, 110024. https://doi.org/10.1016/j.foodres.2020.110024
- Ramos, M., Valdés, A., Beltrán, A., & Garrigós, M. C. (2016). Gelatin-based films and coatings for food packaging applications. Functional Coatings for Food Packaging Applications, 6 (4) , 41. https://doi.org/10.3390/coatings6040041
- Rashed, K., Ćirić, A., Glamočlija, J., & Soković, M. (2014). Antibacterial and antifungal activities of methanol extract and phenolic compounds from Diospyros virginiana L. Industrial Crops and Products, 59, 210–215. https://doi.org/10.1016/j.indcrop.2014.05.021
- Riaz, A., Aadil, R. M., Amoussa, A. M. O., Bashari, M., Abid, M., & Hashim, M. M. (2021). Application of chitosan‐based chitosan-based apple peel polyphenols edible coating on the preservation of strawberry (Fragaria ananassa cv Hongyan) fruit. Journal of Food Processing and Preservation, 45(1), e15018. https://doi.org/10.1111/jfpp.15018
- Robles-Flores, G. D. C., Abud-Archila, M., Ventura-Canseco, L. M. C., Meza-Gordillo, R., Grajales-Lagunes, A., Ruiz-Cabrera, M. A., & Gutiérrez-Miceli, F. A. (2018). Development and evaluation of a film and edible coating obtained from the cajanus cajan seed applied to fresh strawberry fruit. Food and Bioprocess Technology, 11(12), 2172–2181. https://doi.org/10.1007/s11947-018-2175-5
- Sagdic, O., Ozturk, I., Ozkan, G., Yetim, H., Ekici, L., & Yilmaz, M. T. (2011). RP-HPLC–DAD analysis of phenolic compounds in pomace extracts from five grape cultivars: Evaluation of their antioxidant, antiradical and antifungal activities in Orange and apple juices. Food Chemistry, 126(4), 1749–1758. https://doi.org/10.1016/j.foodchem.2010.12.075
- Salama, H. E., Abdel Aziz, M. S., & Alsehli, M. (2019). Carboxymethyl cellulose/sodium alginate/chitosan biguanidine hydrochloride ternary system for edible coatings. International Journal of Biological Macromolecules, 139, 614–620. https://doi.org/10.1016/j.ijbiomac.2019.08.008
- Salazar-López, N. J., Domínguez-Avila, J. A., Yahia, E. M., Belmonte-Herrera, B. H., Wall-Medrano, A., Montalvo-González, E., & González-Aguilar, G. A. (2020). Avocado fruit and by-products as potential sources of bioactive compounds. Food Research International, 138, 109774. https://doi.org/10.1016/j.foodres.2020.109774
- Samsi, M. S., Kamari, A., Din, S. M., & Lazar, G. (2019). Synthesis, characterization and application of gelatin–carboxymethyl cellulose blend films for preservation of cherry tomatoes and grapes. Journal of Food Science and Technology, 56(6), 3099–3108. https://doi.org/10.1007/s13197-019-03809-3
- Shankar, S., Khodaei, D., & Lacroix, M. (2021). Effect of chitosan/essential oils/silver nanoparticles composite films packaging and gamma irradiation on shelf life of strawberries. Food Hydrocolloids, 117, 106750. https://doi.org/10.1016/j.foodhyd.2021.106750
- Sharif, N., Falcó, I., Martínez-Abad, A., Sánchez, G., López-Rubio, A., & Fabra, M. J. (2021). On the use of persian gum for the development of antiviral edible coatings against murine norovirus of interest in blueberries. Polymers, 13(2), 224. https://doi.org/10.3390/polym13020224
- Shohayeb, M., Abdel-Hameed, E., & Bazaid, S. (2013). Antimicrobial activity of tannins and extracts of different parts of Conocarpus erectus L. International Journal of Pharma and Bio Science, 3(2) , 544–553. https://scholar.google.com.mx/scholar?hl=es&as_sdt=0%2C5&q=Antimicrobial+activity+of+tannins+and+extracts+of+different+parts+of+Conocarpus+erectus+L&btnG=
- Sinsinwar, S., Sarkar, M. K., Suriya, K. R., Nithyanand, P., & Vadivel, V. (2018). Use of agricultural waste (coconut shell) for the synthesis of silver nanoparticles and evaluation of their antibacterial activity against selected human pathogens. Microbial Pathogenesis, 124, 30–37. https://doi.org/10.1016/j.micpath.2018.08.025
- Soazo, M., Pérez, L. M., Rubiolo, A. C., & Verdini, R. A. (2015). Prefreezing application of whey protein‐based edible coating to maintain quality attributes of strawberries. International Journal of Food and Techonology, 50(3), 605–611. https://doi.org/10.1111/ijfs.12667
- Sogvar, O. B., Koushesh Saba, M., & Emamifar, A. (2016). Aloe vera and ascorbic acid coatings maintain postharvest quality and reduce microbial load of strawberry fruit. Postharvest Biology and Technology, 114, 29–35. https://doi.org/10.1016/j.postharvbio.2015.11.019
- Swett, C. L., Butler, B. B., Peres, N. A., Koivunen, E. E., Hellman, E. M., & Beaulieu, J. R. (2020). Using model-based fungicide programing to effectively control Botrytis and Anthracnose fruit rots in Mid-Atlantic strawberry fields and co-manage strawberry sap beetle (Stelidota geminate). Crop Protection, 134, 105175. https://doi.org/10.1016/j.cropro.2020.105175
- Tanwar, R., Gupta, V., Kumar, P., Kumar, A., Singh, S., & Gaikwad, K. K. (2021). Development and characterization of PVA-starch incorporated with coconut shell extract and sepiolite clay as an antioxidant film for active food packaging applications. International Journal of Biological Macromolecules, 185, 451–461. https://doi.org/10.1016/j.ijbiomac.2021.06.179
- Valenzuela, C., Tapia, C., López, L., Bunger, A., Escalona, V., & Abugoch, L. (2015). Effect of edible quinoa protein-chitosan based films on refrigerated strawberry (Fragaria×ananassa) quality. Electronic Journal of Biotechnology, 18(6), 406–411. https://doi.org/10.1016/j.ejbt.2015.09.001
- Velickova, E., Winkelhausen, E., Kuzmanova, S., Alves, V. D., & Moldão-Martins, M. (2013). Impact of chitosan-beeswax edible coatings on the quality of fresh strawberries (Fragaria ananassa cv Camarosa) under commercial storage conditions. LWT - Food Science and Technology, 52(2), 80–92. https://doi.org/10.1016/j.lwt.2013.02.004
- Ventura-Aguilar, R. I., Bautista-Baños, S., Flores-García, G., & Zavaleta-Avejar, L. (2018). Impact of chitosan based edible coatings functionalized with natural compounds on Colletotrichum fragariae development and the quality of strawberries. Food Chemistry, 262, 142–149. https://doi.org/10.1016/j.foodchem.2018.04.063
- Wang, D., Yeats, T. H., Uluisik, S., Rose, J. K. C., & Seymour, G. B. (2018). Fruit softening: Revisiting the role of pectin. Trends in Plant Science, 23(4), 302–310. https://doi.org/10.1016/j.tplants.2018.01.006
- Xiong, Y., Kamboj, M., Ajlouni, S., & Fang, Z. (2021). Incorporation of salmon bone gelatine with chitosan, gallic acid and clove oil as edible coating for the cold storage of fresh salmon fillet. Food Control, 125, 107994. https://doi.org/10.1016/j.foodcont.2021.107994
- Yemmireddy, V. K., Cason, C., Moreira, J., & Adhikari, A. (2020). Effect of pecan variety and the method of extraction on the antimicrobial activity of pecan shell extracts against different foodborne pathogens and their efficacy on food matrices. Food Control, 112, 107098. https://doi.org/10.1016/j.foodcont.2020.107098
- Yildiz, G., & Aadil, R. M. (2020). Comparison of high temperature-short time and sonication on selected parameters of strawberry juice during room temperature storage. Journal of Food Science and Technology, 57(4), 1462–1468. https://doi.org/10.1007/s13197-019-04181-y
- Yousuf, B., Qadri, O. S., & Srivastava, A. K. (2018). Recent developments in shelf-life extension of fresh-cut fruits and vegetables by application of different edible coatings: A review. LWT, 89, 198–209. https://doi.org/10.1016/j.lwt.2017.10.051
- Zhang, W., Li, X., & Jiang, W. (2020). Development of antioxidant chitosan film with banana peels extract and its application as coating in maintaining the storage quality of apple. International Journal of Biological Macromolecules, 154, 1205–1214. https://doi.org/10.1016/j.ijbiomac.2019.10.275
- Zhao, X., Xia, M., Wei, X., Xu, C., Luo, Z., & Mao, L. (2019). Consolidated cold and modified atmosphere package system for fresh strawberry supply chains. LWT, 109, 207–215. https://doi.org/10.1016/j.lwt.2019.04.032