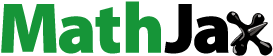
ABSTRACT
A pretreatment experiment of preparing starch syrup with α-amylase and twin-screw extrusion together with broken rice of resistant starch rice was studied. Results showed that the content of reducing sugar in the extrudate was 23.52% when the moisture content of the material was 25%, the screw speed was 210 r/min, the barrel temperature was 85°C, and the addition amount of α-amylase was 90 U/g. The influence of various factors was ranked as follows: material ratio > screw speed > α-amylase addition > barrel temperature. After the extrusion, the contents of starch and resistant starch were not significantly changed, fat content was reduced, and protein and ash contents increased. Starch in the rice was degraded, and the particle structure was destroyed, while the crystal structure changed from type A to type V. Thermodynamic properties and solubility both changed. Rice syrup has a high transparency, good rheology, and a more delicate and soft taste.
1 Introduction
At present, the per capita consumption of starch sugar in China is relatively low, and the raw material of starch sugar is mainly from corn starch. In order to reduce the use of corn starch, relieve the shortage of corn production, and improve the added value of rice in China, broken rice of resistant starch rice is used to produce starch syrup. Rice starch syrup is rich in nutrients, such as minerals and amino acids, which may affect food composition (Okafor et al., Citation2019). Therefore, rice syrup is added to baking and beer products, fruit drinks, and other food products as a substitute for sugar (Ofoedu et al., Citation2020).
Resistant starch rice is a kind of functional rice because resistant starch has many physiological functions, such as preventing intestinal diseases, reducing blood glucose level after meals, lowering blood lipid level, controlling body weight, and promoting the absorption of trace elements (Öztürk & Mutlu, Citation2019). However, although resistant starch rice is rich in nutrients, its poor taste leads to limited utilization. Hence, making full use of resistant starch rice through processing methods has become an important research topic.
Extrusion is a common method for starch modification and food pretreatment, and has the advantages of high efficiency, continuity, low energy consumption, and low pollution. It has been widely used in the production and processing of grains (Saeleaw et al., Citation2012; Ye et al., Citation2018). The extrusion process is a complex physical, chemical, and biological reaction process. The processing performance and quality of extruded products are influenced by extrusion process parameters, such as barrel temperature, screw speed, screw configuration, die pressure, torque, and raw material properties, including composition ratio, particle size, and water content (Dalbhagat et al., Citation2019; Pardhi et al., Citation2019; Ravindran et al., Citation2011; Sumargo et al., Citation2016). Enzyme-treated extrusion is a novel type of grain extrusion and can realize gelatinization, liquifaction and biotransformation efficiency simultaneously (Xu et al., Citation2015). Extrusion expansion not only significantly change the physical configuration, structure and crystallinity (Mir et al., Citation2016) of rice grains but also destroy the cellular structure for starch escape, after binding with the enzyme, which accelerates the transformation of starch into simple sugar (Zeng et al., Citation2017). Commonly, higher reducing sugar content and shorter starch syrup liquefaction time contribute to a higher conversion rate of starch syrup. Starch is mainly used as a raw material in the production of starch syrup, whereas rice is rarely used as a raw material. Permanasari et al. (Citation2018) produced glucose syrup through red sorghum starch, while Lin et al. (Citation2013) prepared starch syrup with rice as a raw material. Starch syrup was prepared by pretreating materials through soaking, wet milling, and alkali extraction. The pretreatment process is complicated, and the production cost is high. Currently, processing technologies involving pretreatment through extruding enzymolysis for starch syrup production under low-temperature conditions and using resistant starch rice as a raw material are rarely reported. Therefore, in this research, broken rice of resistant starch rice was used as a raw material, and was pretreated with extrusion and enzymes under low temperature for starch syrup production. The influence of amylase addition, extruder screw speed, extruder temperature, and material ratio on the reducing sugar content of extruded materials during low-temperature extrusion is discussed. The components of resistant starch rice before and after extrusion, and its surface structure, crystal structure, thermodynamics, solubility and other properties were studied. The results would serve as technical references for solving problems in the pretreatment process for starch syrup production.
2 Materials and methods
2.1 Raw materials and chemicals
Broken rice of resistant starch rice was obtained from Jiangxi Academy of Agricultural Sciences, and high-temperature α-amylase (food grade) was purchased from Wankang Biological Technology Co., Ltd. All chemicals and reagents used were of analytical grade.
2.2 Extrusion method for raw material pretreatment
Resistant starch rice was crushed, and 1 kg of rice flour was weighed. The moisture content of the material and amount of enzyme were adjusted, and the screw speed of the twin-screw extruder and the temperature of the extrusion barrel were controlled. The feeding rate was fixed to 15 kg/h. After pretreatment with the twin-screw extruder, the material was dried and crushed. The crushed rice flour was then passed through the 80-mesh sieve for subsequent use.
2.3 Research on the influence of extrusion processing conditions on the reducing sugars in rice
2.3.1 Single-factor experiment
Resistant starch rice was used as the raw material and was extruded through the twin-screw extruder (DS32II, Jinan Saixin Machinery Co., Ltd.). The content of the reducing sugar after extrusion was used as the indicator, and water content was adjusted to 15%, 20%, 25%, 30%, and 35%). The extrusion barrel temperature was set as 75°C, 85°C, 95°C, 105°C, and 115°C). The extrusion screw speeds were 105, 140, 175, 210, and 245 rpm. The amounts of amylase were 60, 70, 80, 90, and 100 U/g). The influence of four factors on the reducing sugar of rice after extrusion was studied. When a single factor was selected, the conditions of the remaining factors were as follows: feeding rate 5 kg/h; water content 25%; extrusion barrel temperature 95°C; extrusion screw speed 210 rpm; and enzyme dosage 80 U/g. The experiment was repeated three times.
2.3.2 Orthogonal experiment
Given that the reducing sugar content of the extrudate was influenced by screw speed after extrusion, extrusion barrel temperature, material ratio, and α-amylase addition amount, a four-factor and three-level orthogonal test () was carried out on the reducing sugar content of the resistant starch rice through extruding enzyme according to the results of the single-factor experiment. Three experiments were conducted, and the reducing sugar content of the extrudate was used as the indicator.
Table 1. Orthogonal test design.
2.4 Research on the nutritional composition and structural characteristics of resistant starch rice before and after extrusion
2.4.1 Influence of extrusion on the chemical properties of the resistant starch rice
Starch, protein, fat, ash, and the other components of the resistant starch rice before and after extrusion were determined and analyzed. The samples were tested in parallel at least three times.
Measurement of starch: acid hydrolysis method GB5009.9–2016;
Measurement of protein: GB5009.5–2016 determination of protein in food;
Measurement of fat: GB5009.6–2016 determination of fat in food;
Measurement of ash: GB5009.4–2016 determination of ash in food;
Measurement of reducing sugar content: direct titration method in GB/T5009.7–2008
2.4.2 Influence of extrusion on the particle morphology of the resistant starch rice
Changes in the morphology and structures of rice flour particles before and after treatment were tested using a scanning electron microscope (SU8100, Hitachi, Japan). The sample was initially dispersed in 1% (w/v) alcohol. A small amount of each sample was directly placed on the surface of a glass slide. The samples were then dried at 32°C for 24 h and plated with gold. The processed samples were inspected and observed with a scanning electron microscope at an accelerating voltage of 5.00 kV, distance of 15.1 mm, and magnification of 5000 × .
2.4.3 Influence of extrusion on the crystal structure of the resistant starch rice
The diffraction patterns of the samples were obtained using an X-ray diffraction instrument (D8 Advance, Bruker, Germany). The angle of the scanned diffraction area ranged from 5° to 30°, the target voltage was 30 kV, the current was 30 mA, the scanning speed was 2°/min, and the step size was 0.02. Changes in crystalline and amorphous regions were observed according to the diffraction patterns.
2.4.4 Analysis of the effect of extrusion on the thermodynamic properties of the resistant starch rice
Approximately 13.3 mg of each sample was placed in an aluminum crucible, and distilled water was added with the proportion was 1:2 (Wsample/Wwater). The crucibles were sealed and stored at room temperature for 24 h. The measurement and scanning temperatures were set at a range of 0–200°C through DSC (200F3, NETZSCH, Germany) and scanning rate of 10°C/min. The empty crucible was used as the reference, and the flow rate was set at 20 mL/min.
2.4.5 Influence of extrusion on the solubility of the resistant starch rice
Exactly 1.0 g of sample was placed in a centrifuge tube, and 50 mL of distilled water was added. The dispersion solutions were stirred and heated at 60°C, 70°C, 80°C, and 90°C for 30 min. The gelatinized sample was cooled to room temperature and then centrifuged at 6000 r/min for 20 min. The supernatant was dried to a constant weight at 110°C. Dried mass was the mass of the soluble part, and the remaining mass after the supernatant was removed was the mass of water swelling. Each sample was tested at least five times.
2.6 Determination of physical and chemical properties of rice syrup
2.6.1 Chromatic analysis of rice syrup
The homogeneous samples were placed into the sample box for testing. Color indicator lightness L *, red-green index a *, and yellow-blue index b * were read through the instrument screen.
The color difference is calculated using
CIE 1976 (L * a * b *) color space color difference formula (1):
where ∆L * is the brightness difference between samples; ∆a * is the red-green index difference in color space; and ∆b * is the yellow-blue index difference between samples in color space.
2.6.2 Determination of the rheological characteristics of rice syrup
The prepared rice syrup was placed on the rheometer determination platform, the 20 mm diameter plate and steady-state test procedures were selected, the gap and the temperature were set to 1.000 and 25°C, while the shear rate was 0–120 s−1. The shear stress changes with the rate of shear throughout the process.
2.7 Statistical analysis of data
All experiments were conducted in triplicate. ANOVA and Duncan test were performed to determine significant differences between means (P < 0.05) with SPSS 24, and graphic presentations were performed using Origin 8.6.
3. Results and discussion
3.1 Influence of extrusion processing conditions on the reducing sugars in resistant starch rice
3.1.1 Influence of material water on reducing sugars in resistant rice
As shown in , reducing sugar content increased and then decreased with increasing water content in the material. The highest proportion of reducing sugar content (19.68%) was obtained at 25% water content. Shearing force increased with decreasing water content, and a molten state was difficult to achieve. It was reported that α-amylase could act on amylose, which made starch rapid degradation and produce oligosaccharides. Then, oligosaccharides were slowly hydrolyzed, thus producing glucose and maltose (Tester et al., Citation2006). In addition, when α-amylase acted on amyarch, it produced small molecular glucose, maltose, and some limit dextrin, which consisted of some glucose groups (Konsula & Liakopoulou-Kyriakides, Citation2004). Furthermore, enzymes facilitated the degradation of amylose to sugars in a low-moisture environment (Budiasih et al., Citation2007). The high water content in the material was conducive to the enzymatic hydrolysis reaction. A material with a high water content exhibited good fluidity inside a cavity, and its residence time is short. Thus, the material was subjected to screw shear force for a short time. This condition is not conducive to the microporation of starch granule structures (Jongsutjarittam & Charoenrein, Citation2014). The optimal material concentration of the natural rice raw material extrudate was 25%.
Figure 1. Influence of extrusion processing conditions on the reducing sugars in resistant starch rice. (a) Influence of material water content on rice reducing sugar. (b) Influence of reaction temperature on rice reducing sugar. (c) Influence of extrusion processing rotation on rice reducing sugars. (d) Influence of extrusion enzyme addition amount on the rice reducing sugars.
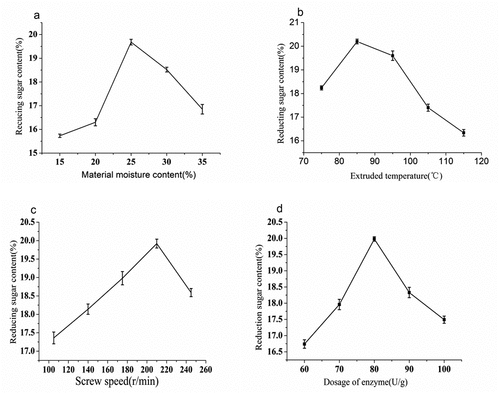
3.1.2 Influence of temperature of extrusion processing on the reducing sugars in resistant rice
As shown in , when the temperature reached 85°C, the reducing sugar content reached its maximum value of 20.2%. As the material absorbed heat during extrusion, it provided an appropriate temperature for enzymatic hydrolysis reactions. As the optimal reaction temperature of high-temperature-resistant α-amylase is 80–100°C, the temperature in the extrusion increased with material temperature, the optimum reaction temperature was reached in several minutes, and enzyme inactivation was achieved under the action of high temperature and high pressure at the extrusion head. When the extrusion temperature was more than 85°C, the protease was easily inactivated, the action time was reduced, and enzymolysis was incomplete, although the degree of gelatinization of rice starch and the action chance of liquefaction enzyme increased. Therefore, an extrusion temperature of 85°C was used.
3.1.3 Influence of screw speed on the reducing sugars in resistant rice
As shown in , the highest amount of reducing sugar generated by extruding rice was obtained when the screw speed was 210 r/min. Shearing force increased with screw speed, whereas the shearing time between the material and extruder was shortened. Therefore, the starch molecules are less damaged, while the reducing sugar content increased and then decreased. Reducing sugar generated from enzymolysis by extruding rice at 210 r/min was 19.92%, increasing by 21.65% compared with the amount of reducing sugar generated before extrusion.
3.1.4 Influence of enzyme addition on reducing sugars in resistant rice
As shown in , reducing sugar content increased and then decreased with increasing enzyme addition amount in the material. When the enzyme addition amount of the material was 80 U/g, the reducing sugar content reached 19.98%. The α-Amylase was an endo-amylase and could arbitrarily cut the α-1,4 glycosidic bonds inside starch molecules, and thus degrading starch molecules rapidly. The color reaction of iodine was lost, the viscosity of the starch milk was reduced, and the reducing power of the hydrolyzate increased. In the extrusion process, shear force was crucial, and orderly loss could be observed in the amorphous zone. As shearing force increased, starch granules were ruptured, and thus starch was easily hydrolyzed by enzymes, and the conversion of starch into glucose was accelerated (Román et al., Citation2017; Zhang et al., Citation2014).
3.2 Orthogonal test of reducing sugar in resistant starch rice under extrusion
Factors affecting the enzymatic hydrolysis of rice during extrusion were ranked as A > C > D > B (), and the optimum process combination was A2B1C2D3, that is, the screw speed of the extrusion was 210 r/min, the temperature of the extrusion barrel was 85°C, the material ratio was 25%, and the amount of enzyme was 90 U/g.
Table 2. Results of orthogonal test.
3.3 Influence of extrusion on the chemical composition, structures, and properties of the resistant starch rice
3.3.1 Influence of low-temperature extrusion on the main composition of the resistant starch rice
As shown in , owing to the mechanical friction between the screw and material, between the material and the barrel, and inside the material, the material was extruded, stirred, and sheared intensively and thus further refined and homogenized. After the low-temperature extrusion of resistant starch rice, the total starch content decreased by 3.59%, and the resistant starch content decreased by 3.84%. The reason could be that the low extrusion temperature slightly damaged the starch. The fat content of the resistant starch rice after extrusion was significantly lower than that before extrusion possibly because of the formation of fat complexes between extrusion fat and starch (Wang et al., Citation2016). These complexes could form quickly at approximately 60°C, thus reducing the digestibility of rice, slowing down absorption, and lowering blood sugar (Jeong et al., Citation2021). In the entire low-temperature extrusion process, protein content increased by 10.16%. During the process of low-temperature extrusion, the material is subjected to screw shear extrusion to separate the starch and protein in the material. Therefore, the protein content is increased. This increase was consistent with the results of Liu et al. (Citation2011). The content of reducing sugar increased after the rice was extruded (Zeng et al., Citation2017). The reducing sugar content in rice flour increased 24.57-fold relative to that before extrusion.
Table 3. Main basic composition (the percentage content of dry basis, unit: %).
3.3.2 Influence of extrusion on the particle morphology of the resistant starch rice
As shown in , changes in the appearance of broken rice powder particles before and after the extrusion process were observed using a SUPRA 40/40VP scanning electron microscope. The observation results showed that the rice particles had regular shapes, smooth surfaces, and compact structures before extrusion, consistent with the observation results of Zavareze et al. (Citation2010). However, the extruded rice nearly had no complete particle shape, and the surface was irregular and appeared like scales, indicating that the starch was completely gelatinized after extrusion. In addition, the starch particles were increased which is conducive to enzymatic hydrolysis reaction.
3.3.3 Influence of extrusion on the crystal structure of the resistant rice
The X-ray diffraction patterns of natural resistant starch rice and extrusion-resistant starch rice are shown in . In the X-ray diffraction patterns of natural resistant starch rice, typical A-shaped peaks were found at 2θ = 15.5°, 17°, 18.18°, and 23.4°. These peaks were also observed in the X-ray diffraction pattern of the crystal structure of starch molecules in general rice (Kuo et al., Citation2019). The extruded enzyme-hydrolyzed resistant starch rice showed a new high-intensity characteristic diffraction peak at 2θ of 20°. This peak belonged to a V-shaped structure (Ye et al., Citation2016). Moreover, the diffraction peak intensity at 20° was obviously stronger than that of natural resistant starch rice particles. After the extrusion of the natural resistant starch rice, the peak value increased relative to that of the rice without extrusion, and the peak shape was changed. These effects might be related to the complex (Sittipod & Shi, Citation2016) formed by amylose and free fat during extrusion. The increase of the complex content resulted in a strong diffraction peak that aggregated into a crystal structure. In addition, extrusion destroyed the original crystal structure of starch and promoted the formation of a new crystalline zone.
3.3.4 Changes in the thermodynamic properties of the resistant starch rice during extrusion
The influences of extrusion on the thermodynamic properties of starch are provided in . The initial temperature (T0), peak temperature (Tp), and terminated temperature (Tc) of the extruded resistant starch rice significantly increased (P < 0.05), which was possibly due to the formation of amylose – lipid complexes after extrusion. The amylose molecules and amylopectin chain interacted freely in the branched crystallization zone, and thus the fluidity of the amylopectin chain was reduced, and temperature increased (Sun et al., Citation2014). The gelatinization enthalpy (∆H) of starch in the sample after extrusion increased from 95.63 to 212.4 J/g. The reason was that the crystallization of rice starch under low-temperature extrusion was excellent, and the thermal stability of the granules was enhanced, increasing the gelatinization temperature and gelatinization enthalpy (Martínez et al., Citation2014). In addition, the high amylose content of resistant starch rice inhibited the destruction of the starch crystal structure during the heating and gelatinization process and led to an increase in gelatinization temperature and gelatinization enthalpy.
Table 4. Changes in thermodynamic properties before and after low-temperature extrusion.
3.3.5 Influence of extrusion on the resistant starch rice solubility during extrusion
As shown in , the solubility of the original resistant rice increased with temperatures from 60°C to 90°C. When the temperature was above 80°C, the solubility rapidly increased from 9.69% to 14.9%. The solubility of extruded rice powder was much higher than that of raw rice, reaching 66.86% at 60°C. The solubility of the extruded rice powder significantly increased at 70°C and reached 88.01% at 80°C. However, solubility showed no significant change with increasing temperature. Solubility reached a maximum of 91.46% at 90°C. The main reason was that under the conditions of high-temperature shearing force, some portions of amylopectin macromolecular substances in rice starch particles were degraded into small particles, such as amylose and dextrin, and some amylose molecules precipitated, thereby increasing solubility. The increase in solubility was beneficial to the hydrolysis of enzymes during liquefaction and saccharification.
3.4 Analysis of physicochemical properties of rice syrup
3.4.1 Analysis of color difference of rice syrup
The magnitude of the color value indicates the intensity of the color of rice syrup (Osuji et al., Citation2020). Color is an important index for starch sugar quality evaluation and directly affects people’s judgment of the quality of starch syrup products. The color of starch syrup requires colorless or slightly yellow, clear and transparent. Each sample was tested five times in parallel, and the average value was taken as the color index of extruded rice syrup. The relative standard deviation and ΔE values of the parallel test results were calculated, and the results are shown in .
Table 5. Parallel experimental test results of the color indicator.
The brightness value L* reflected the light transmission of the sample, or the shade of the sample color. The smaller the L* value, the worse the light transmission of the sample was, and the darker the sample color was. The distribution of a* and b* values in shows that the color of the samples was biased toward green and yellow tones, which was consistent with the visual results. The repeatability of the tests of the color indexes of the samples all performed well. The extruded rice syrup had high L* and good light transmission, and the a and b values decreased to different degrees. The ΔE value indicated the change of the total color of rice syrup. The larger the value, the greater the color difference was. ΔE was 5.02, indicating that extruded rice syrup had modified color and higher transparency than raw rice syrup.
3.4.2 Determination of rheological properties of rice syrup
Thixotropy refers to the natural structure of the sample. With the increase in shear rate, the internal structure was destroyed, and after the force was stopped, it took some time for the sample to return to its original structure. The larger the area of the thixotropic ring, the greater the destruction of the molecular structure of the rice syrup by external forces, the more obvious the viscosity change, the longer the time for the system to return to its initial state, and the poorer the taste of the syrup was. Conversely, the smaller the thixotropy, the higher the rheological stability was, the softer the taste was, and the more conducive to food processing and production (Singh & Kaur, Citation2017). From , it could be seen that the shear rates of the 2 samples were within 0–120 s−1 with different degrees of thixotropy. As shown in , the thixotropic ring area of the two samples was rice syrup > extruded rice syrup. In contrast, the extruded rice syrup was least damaged by the external force, and took the shortest time to return to the initial state, giving the best rheological stability. Therefore, the extruded rice syrup was easier to recover its original structure and had a more delicate and soft taste.
4 Conclusions
The optimum process conditions for processing resistant starch rice through extrusion and enzymatic hydrolysis were as follows: water content of the material of 25%; screw speed of 210 r/min; barrel temperature of 85°C, and addition amount of α-amylase of 90 U/g. The content of reducing sugar in the extrudate was 23.52% under such conditions. The reducing sugar content in resistant rice after extrusion increased 24.57-fold relative to that before extrusion. The nutritional composition of resistant starch rice changed after extrusion and enzymatic hydrolysis, and the total starch and resistant starch content were not significantly changed. By contrast, reducing sugar, protein, and fat contents were significantly changed. After extrusion, the rice nearly had no complete particle shape, and the distribution was tight. The particles aggregated after extrusion, and the surface was irregular and appeared like scales. In addition, the characteristic peaks of the crystal structure of the rice particles were obviously destroyed, while the thermal properties of the rice were significantly changed after extrusion. The initial temperature increased from 99.97°C to 107.81°C, peak temperature increased from 129.53°C to 139.66°C, and gelatinization enthalpy improved from 95.63 to 212.4 J/g. The solubility of rice after extrusion was significantly higher than that of original rice. In this experiment, the degree of starch saccharification is judged by the content of reducing sugar, namely DE value. Pretreatment of rice raw materials by extrusion enzymolysis composite technology improves the content of reducing sugar, changes the nutritional composition and structural characteristics of rice, is conducive to the later saccharification of starch syrup, so that starch can be fully saccharified, and can shorten the saccharification time, save energy consumption, and reduce the amount of enzyme. In particular, rice syrup has a good color, high transparency, good rheology, and a more delicate and soft taste, which is suitable for making food additives. The resistant starch particles could bind to residual protein, and the hydrophobic groups in such proteins had a strong interaction with starch particle, which made it was difficult to separate from starch particles, thus increasing the resistant starch content in syrup, and playing a certain therapeutic effect on diabetic people.
Disclosure statement
No potential conflict of interest was reported by the author(s).
Additional information
Funding
References
- Budiasih, W. S., Hwan Kim, M., Byung Soon, I., Yoon Cha, J., & Hyung Ryu, G. (2007). Effects of feed moisture content on enzymatic hydrolysis of corn starch in twin-screw extruder and saccharification of the dried extrudates. Food Science and Biotechnology, 16(3), 381–385. https://doi.org/10.1088/1742-6596/61/1/107
- Dalbhagat, C. G., Mahato, D. K., & Mishra, H. N. (2019). Effect of extrusion processing on physicochemical, functional and nutritional characteristics of rice and rice-based products: A review. Trends in Food Science & Technology, 85, 226–240. https://doi.org/10.1016/j.tifs.2019.01.001
- Jeong, D., Lee, J. H., & Chung, H. J. (2021). Effect of molecular structure on phase transition behavior of rice starch with different amylose contents. Carbohydrate Polymers, 259, 1–9. https://doi.org/10.1016/j.carbpol.2021.117712
- Jongsutjarittam, O., & Charoenrein, S. (2014). The effect of moisture content on physicochemical properties of extruded waxy and non-waxy rice flour. Carbohydrate Polymers, 114, 133–140. https://doi.org/10.1016/j.carbpol.2014.07.074
- Konsula, Z., & Liakopoulou-Kyriakides, M. (2004). Hydrolysis of starches by the action of an α-amylase from bacillus subtilis. Process Biochemistry, 39(11), 1745–1749. https://doi.org/10.1016/j.procbio.2003.07.003
- Kuo, C. H., Shieh, C. J., Huang, S. M., Wang, H. M. D., & Huang, C. Y. (2019). The effect of extrusion puffing on the physicochemical properties of brown rice used for saccharification and Chinese rice wine fermentation. Food Hydrocolloids, 94, 363–370. https://doi.org/10.1016/j.foodhyd.2019.03.040
- Lin, Q., Xiao, H., Liu, G. Q., Liu, Z., Li, L., & Yu, F. (2013). Production of maltose syrup by enzymatic conversion of rice starch. Food and Bioprocess Technology, 6(1), 242–248. https://doi.org/10.1007/s11947-011-0681-9
- Liu, C., Zhang, Y., Liu, W., Wan, J., Wang, W., Wu, L., Zuo, N., Zhou, Y., & Yin, Z. (2011). Preparation, physicochemical and texture properties of texturized rice produce by improved extrusion cooking technology. Journal of Cereal Science, 54(3), 473–480. https://doi.org/10.1016/j.jcs.2011.09.001
- Martínez, M., Rosell, C. M., Gómez Pallarés, M., & Gómez, M. (2014). Effect of different extrusion treatments and particle size distribution on the physicochemical properties of rice flour. Food and Bioprocess Technology, 7(9), 2657–2665. https://doi.org/10.1007/s11947-014-1252-7
- Mir, S. A., Bosco, S. J. D., Shah, M. A., & Mir, M. M. (2016). Effect of puffing on physical and antioxidant properties of brown rice. Food Chemistry, 191, 139–146. https://doi.org/10.1016/j.foodchem.2014.11.025
- Ofoedu, C. E., Osuji, C. M., Omeire, G. C., Ojukwu, M., Okpala, C. O. R., & Korzeniowska, M. (2020). Functional properties of syrup from malted and unmalted rice of different varieties: A comparative study. Journal of Food Science, 85(10), 3081–3093. https://doi.org/10.1111/1750-3841.15446
- Okafor, D. C., Ofoedu, C. E., Nwakaudu, A., & Daramola, M. O. (2019). Enzymes as additives in starch processing: A short overview. Enzymes in Food Biotechnology, 149–168. Chapter 10. https://doi.org/10.1016/B978-0-12-813280-7.00010-4
- Osuji, C., Ofoedu, C. E., Omeire, G. C., & Ojukwu, M. (2020). Colour analysis of syrup from malted and unmalted rice of different varieties. Croatian Journal of Food Science and Technology, 12(1), 130–138. https://doi.org/10.17508/CJFST.2020.12.1.03
- Öztürk, S., & Mutlu, S. (2019). Physicochemical properties, modifications, and applications of resistant starches. In Starches for food application (pp. 297–332). Academic Press. https://doi.org/10.1016/B978-0-12-813280-7.00010-4
- Pardhi, S. D., Singh, B., Nayik, G. A., & Dar, B. N. (2019). Evaluation of functional properties of extruded snacks developed from brown rice grits by using response surface methodology. Journal of the Saudi Society of Agricultural Sciences, 18(1), 7–16. https://doi.org/10.1016/j.jssas.2016.11.006
- Permanasari, A. R., Yulistiani, F., Purnama, R. W., Widjaja, T., & Gunawan, S. (2018, June). The effect of substrate and enzyme concentration on the glucose syrup production from red sorghum starch by enzymatic hydrolysis. IOP Conference Series: Earth and Environmental Science, 160(1), 012002. IOP Publishing.
- Ravindran, G., Carr, A., & Hardacre, A. (2011). A comparative study of the effects of three galactomannans on the functionality of extruded pea–rice blends. Food Chemistry, 124(4), 1620–1626. https://doi.org/10.1016/j.foodchem.2010.08.030
- Román, L., Martínez, M. M., Rosell, C. M., & Gómez, M. (2017). Changes in physicochemical properties and in vitro starch digestion of native and extruded maize flours subjected to branching enzyme and maltogenic α-amylase treatment. International Journal of Biological Macromolecules, 101, 326–333. https://doi.org/10.1016/j.ijbiomac.2017.03.109
- Saeleaw, M., Dürrschmid, K., & Schleining, G. (2012). The effect of extrusion conditions on mechanical-sound and sensory evaluation of rye expanded snack. Journal of Food Engineering, 110(4), 532–540. https://doi.org/10.1016/j.jfoodeng.2012.01.002
- Singh, S., & Kaur, M. (2017). Steady and dynamic shear rheology of starches from different oat cultivars in relation to their physicochemical and structural properties. International Journal of Food Properties, 20(12), 3282–3294. https://doi.org/10.1080/10942912.2017.1286504
- Sittipod, S., & Shi, Y. C. (2016). Changes of starch during parboiling of rice kernels. Journal of Cereal Science, 69, 238–244. https://doi.org/10.1016/j.jcs.2016.03.015
- Sumargo, F., Gulati, P., Weier, S. A., Clarke, J., & Rose, D. J. (2016). Effects of processing moisture on the physical properties and in vitro digestibility of starch and protein in extruded brown rice and pinto bean composite flours. Food Chemistry, 211, 726–733. https://doi.org/10.1016/j.foodchem.2016.05.097
- Sun, Q., Han, Z., Wang, L., & Xiong, L. (2014). Physicochemical differences between sorghum starch and sorghum flour modified by heat-moisture treatment. Food Chemistry, 145, 756–764. https://doi.org/10.1016/j.foodchem.2013.08.129
- Tester, R. F., Qi, X., & Karkalas, J. (2006). Hydrolysis of native starches with amylases. Animal Feed Science and Technology, 130(1–2), 39–54. https://doi.org/10.1016/j.anifeedsci.2006.01.016
- Wang, L., Duan, W., Zhou, S., Qian, H., Zhang, H., & Qi, X. (2016). Effects of extrusion conditions on the extrusion responses and the quality of brown rice pasta. Food Chemistry, 204, 320–325. https://doi.org/10.1016/j.foodchem.2016.02.053
- Xu, E., Wu, Z., Wang, F., Li, H., Xu, X., Jin, Z., & Jiao, A. (2015). Impact of high-shear extrusion combined with enzymatic hydrolysis on rice properties and Chinese rice wine fermentation. Food and Bioprocess Technology, 8(3), 589–604. https://doi.org/10.1007/s11947-014-1429-0
- Ye, J., Hu, X., Luo, S., Liu, W., Chen, J., Zeng, Z., & Liu, C. (2018). Properties of starch after extrusion: A review. Starch‐Stärke, 70(11–12), 1700110. https://doi.org/10.1002/star.201700110
- Ye, J., Hu, X., Zhang, F., Fang, C., Liu, C., & Luo, S. (2016). Freeze-thaw stability of rice starch modified by improved extrusion cooking technology. Carbohydrate Polymers, 151, 113–118. https://doi.org/10.1016/j.carbpol.2016.05.026
- Zavareze, E. D. R., Storck, C. R., de Castro, L. A. S., Schirmer, M. A., & Dias, A. R. G. (2010). Effect of heat-moisture treatment on rice starch of varying amylose content. Food Chemistry, 121(2), 358–365. https://doi.org/10.1016/j.foodchem.2009.12.036
- Zeng, Z., Li, Y., Ye, J., Liu, C., Hu, X., Luo, S., Gong, E., & Ye, J. (2017). The relationship between reducing sugars and phenolic retention of brown rice after enzymatic extrusion. Journal of Cereal Science, 74, 244–249. https://doi.org/10.1016/j.jcs.2017.02.016
- Zhang, Y., Liu, W., Liu, C., Luo, S., Li, T., Liu, Y., Wu, D., & Zuo, Y. (2014). Retrogradation behaviour of high-amylose rice starch prepared by improved extrusion cooking technology. Food Chemistry, 158, 255–261. https://doi.org/10.1016/j.foodchem.2014.02.072