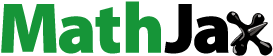
ABSTRACT
This research studies the impacts of microcrystalline cellulose (MCC) concentrations on the physicochemical properties of spray-dried water-in-oil-in-water (W/O/W) vitamin C double emulsion powder Four MCC concentration (1%, 1.5%, 2%, and 2.5%) were used to produce the vitamin C double emulsion powder. The results of the emulsion’s creaming index, flow behaviour index, zeta value, and particle size indicated that these parameters decreased when the MCC concentration increased. Scanning electronic microscopy (SEM) analysis showed that the higher MCC concentration resulted in a smoother surface In general, the MCC concentration increased, the particle size, hygroscopicity, solubility, water content, and water activity of the double emulsion powder decreased. Double emulsion powder containing 2% MCC presented the highest level of antioxidant activity and was mostly stable after storage for 56 days. Before and after drying, transmission electron microscope (TEM) analysis of the morphological characteristics of the liquid emulsion showed that the concentration of 2% MCC in the emulsion could form a W/O/W double emulsion structure. In conclusion, the addition of MCC significantly affect the properties of vitamin C double emulsion.
KEYWORDS:
1. Introduction
Antioxidants, administered to humans as components of food or as certain preventive drugs, have been gaining popularity in recent years. The function of antioxidants in food is to prevent or inhibit food damage due to oxidation (Gulcin, Citation2020). Recent studies also suggest that antioxidants could be capable of neutralizing reactive oxygen species, reducing the risk of cancer and cardiovascular disease (Schilder et al., Citation2020). A critical antioxidant is ascorbic acid (AA) or vitamin C, a powerful antioxidant found in fruits and vegetables. Vitamin C is also used as a food additive to prevent food quality losses due to oxidation (Comunian et al., Citation2013; Zhang et al., Citation2021). On the other hand, that vitamin C is easily degraded (Comunian et al., Citation2013). High pH or temperature, dissolved oxygen, presence of metal ions, and vitamin C’s ionization in aqueous solutions all accelerate vitamin C degradation. The stability of vitamin C also depends on the product formulation, packaging, and storage conditions. Degradation of vitamin C is usually accompanied by a yellowish discoloration and can occur quickly (Parhizkar et al., Citation2018; Stamford, Citation2012).
The Encapsulation is an effective alternative to prevent the degradation of active compounds such as vitamin C. Microencapsulation is the process of coating core components with wall materials to produce micrometre or nanometre scale capsules (Akhavan Mahdavi et al., Citation2016) Proteins and carbohydrates are used to create micron size structures used to trap active labile ingredients. In the microencapsulation method, the water in oil in water (W/O/W) emulsion technique can be used effectively to encapsulate hydrophilic antioxidants by placing them into the internal phase (Berendsen et al., Citation2015; Snoussi et al., Citation2020). Water-in-oil-in-water (W/O/W) double emulsion is a microencapsulation step widely used as a carrier for hydrophilic active ingredients such as anthocyanins and procyanidins (Shaddel et al., Citation2018). Double emulsion W/O/W is a liquid multiphase system with a water-in-oil (W/O) emulsion dispersed in a secondary continuous external water phase (W2) in the form of fine droplets (Lamba et al., Citation2015; Schuh et al., Citation2013). The basic thermodynamics (Su et al., Citation2006) and kinetics (Sapei et al., Citation2012) that affect the instability of multiple emulsions have limited their application in food systems despite their enormous potential.
Some emulsions can become unstable due to droplet flocculation, phase separation during processing and storage, loss of encapsulation from the inner aqueous phase, and Ostwald ripening (diffusion of water from small to large droplets through the oil phase as a result of different internal pressures/Laplace pressure) (Benichou et al., Citation2004; Hino et al., Citation2001; Lamba et al., Citation2015). An alternative to form micro/nano-emulsions with high stability is through Pickering emulsions (Spyropoulos et al., Citation2019). As solid particles (solids) are added to emulsion preparation, particles can be at the droplet interface and provide resistance to coalescence, fusion, and Ostwald maturation. This state is known as a Pickering-type emulsion. Pickering emulsions have several good benefits compared to conventional surfactant-based emulsions, including making the need for fewer emulsifiers, being efficient, not challenging to use, increasing stability, and reducing susceptibility to environmental stresses (Bai et al., Citation2018). Microcrystalline cellulose (MCC), has been effectively applied for emulsion Pickering because of its amphiphilic Pickering properties, wettability, high aspect ratio, irreversible adsorption, and its ability to self-assemble at the oil in water (O/W) interface (Bai et al., Citation2018; Wang et al., Citation2019). Previous research (Xu et al., Citation2016) has been carried out regarding using MCC to stabilize droplets of soy protein hydrolysate isolate in a curcumin emulsion. This study’ indicated that the addition of MCC significantly increases the stability of the curcumin emulsion against the freeze-thaw cycle. It is influenced by the ability of the MCC chain that surrounds the curcumin droplets attached to the soybean protein hydrolyzate isolate.
Increasing the stability of double emulsion products can also be improved by spray drying. Therefore, based on these reports (Berendsen et al., Citation2015; Fang et al., Citation2019; Zhao et al., Citation2020) increase in the stability of multiple emulsions, spray drying was investigated to form a W/O/W emulsion where a core/active material is embedded in a solid continuous matrix. Consequently, spray drying and different MCC concentrations were investigated to stabilize vitamin C in double emulsion encapsulations.
2. Materials and methods
2.1. Materials
Ascorbic acid (Merck, Germany), commercial soybean oil (Salim Ivomas), Maltodextrin DE 10–12 (Cargill), DPPH (2,2-Diphenyl-1-picrylhydrazyl) (Tokyo Chemical Industry Co., Ltd., Japan) Folin-Ciocealteau (Merck, Germany), methanol (Merck, Germany), Microcrystalline cellulose (Vivapur) colloidal type MCG 811 F containing carboxymethyl cellulose (CMC) of 11.3–18.8% (JRS Pharma CMBH & Co., n.d.), Sorbitan Tristearate (Span 65–Futura), and Whey Protein Concentrate (Avonlac 282).
2.2. Preparation of vitamin C double emulsion
Double emulsions were prepared following the modified method reported by Xu et al. (Citation2016). Two types of emulsifier were used, Span 65 and mixture of WPC and MCC. Span 65 is the emulsifier for water in oil emulsion (W/O) and it is soluble in oil, while the mixture of WPC and MCC is the emulsifier of oil in water. The W/O/W emulsion was obtained by dispersing the first water phase (W1) into the oil phase (O) to form a water-in-oil emulsion (W1/O) with homogenization at 14,000 rpm for 5 minutes, then a water-in-oil emulsion (W1/O)) was dispersed into the second aqueous phase (W2) by homogenization at 14,000 rpm for 5 minutes to form a W1/O/W2 double emulsion. The W1/O volume fraction was set at 20%. The W1/O emulsion phase was added to the W2 phase (80%) with a total volume of 100 ml at room temperature. The first water phase (W1) has the composition (demineralized water 4.8 g + Vitamin C 1.2 g). The oil phase (O) contains (13.3 g soybean oil +0.7 g emulsifier namely sorbitan tristearate (Span 65)), while the second aqueous phase (W2) contains (42 g demineralized water +25 g maltodextrin +7 g citric acid +5 g WPC (whey protein concentrate) + MCC). MCC was added as a treatment for the addition of 1% w/v MCC for treatment (P1), 1.5% w/v (P2), 2% w/v (P3), and the addition of 2.5% w/v MCC for treatment P4 of total W/O/W volume. The citric acid is added to maintain the equal osmotic pressure between two water systems (W1/O/W2).
The composition of four treatments is presented in . The formed W/O/W liquid emulsion was stored in a dark glass bottle at 25°C for stability testing for 56 days.
Table 1. Ingredient composition of W/O/W emulsion for each treatment.
2.3. Preparation of vitamin C double emulsion powder by spray drying
The W/O/W double emulsion (400 g) was dried in a laboratory-scale spray dryer equipped with a 0.5 mm nozzle atomizer (mini spray dryer B-290, Buchi, Switzerland). The emulsion was kept at constant room temperature and slowly stirred with a magnetic stirrer during spray drying. The aspirator rate was set at 80%, the feed rate was 1 L/hour, the inlet temperature was 150°C, and the outlet temperature ranged from 63°C to 75°C. The dry powder was collected and stored in plastic containers at −80°C for further analysis (Zhao et al., Citation2020).
2.4. Characteristics of double emulsion W/O/W
2.4.1. Creaming index
The new double emulsion was transferred in a 15 mL test tube to a height of approximately 6 cm and stored at 4°C for 0, 7, 14, 21 & 28 days. The weekly measurements of the emulsion over the clear serum was measured (ht) and compared with the initial emulsion height (h0) to determine the creaming index (CI).
2.4.2. Emulsion morphological structure with TEM
The emulsion morphological structure of the W/O/W emulsion was measured using a TEM HT7700 (Hitachi Tokyo, Japan). The dry W/O/W emulsion sample was dispersed in a suitable solution (5 mg sample in 10 ml aquadest) then homogenized using a vortex, then 5 µl was taken and dripped onto the parafilm. Then, a 400-mesh grid on top of the W/O/W emulsion sample (plastic on the bottom) was kept, allowing it to absorb for 10 minutes. The excess liquid was wiped off using filter paper. Then, Uranyl Acetate dye was added and allowed to sit for 10–30 seconds. Finally, any excess liquid was wiped off.
2.4.3. Rheological properties (apparent viscosity)
The viscosity measurement of the W/OW emulsion was measured using a Raypa RP 1 rotational viscometer (Barcelona, Spain) at 60 rpm, 100 rpm, and 200 rpm. Then, a table was created containing N (rpm), ln(4πN), and lnµApp based on the formula below (Toledo, Citation2007)
where:
The resulting data was then plotted in a graph where the regression equation was obtained to find the n and K (flow consistency index) values. When n < 1, = 1 or > 1 the solution is considered to show pseudoplastic, newtonian dilatant flow.
2.4.4. Zeta potential
The zeta potential of the vitamin C double emulsion was measured at 25°C based on the micro-electrophoresis technique with Zetasizer (SZ-100 nanoparticle series, Horiba, Japan). Data is the average of at least three replicates.
2.4.5. Particle size analysis
The samples were diluted with deionized water (0.01%, w/v), and a Zetasizer was used to quantify the particle size of the vitamin C double emulsion using dynamic light scattering (DLS) at 25°C (SZ-100 nanopartica series, Horiba, Japan).
2.5. Characteristics of stability and antioxidant activity of double emulsion W/O/W powder
2.5.1. Scanning electronic microscopy (SEM)
Morphological analysis was performed using FESEM (Field Emission Scanning Electron Microscopy) analysis. Powder samples were placed on the sample grid and coated with carbon tape. The investigation was then carried out with FE-SEM (Thermo Scientific-Quattro S, U.S.A.) on 5000× magnification with an accelerating voltage of 3.00 kV and a high vacuum method.
2.5.2. Particle size analysis of double emulsion powder
The samples were diluted with deionized water (0.01%, w/v), and a Zetasizer was used to quantify the particle size of the vitamin C double emulsion powder using dynamic light scattering (DLS) at 25°C (SZ-100 nanopartica series, Horiba, Japan).
2.5.3. Water activity
The water activity was determined using AQUALAB lite equipment (Decagon Devices, Pullman, WA, U.S.A.).
2.5.4. Hygroscopicity rate
The hygroscopicity rate determines the moisture absorbed by the powders when exposed at 75% RH at room temperature. Samples (500 µg) were placed in a desiccator containing supersaturated NaCl (75 ± 2% RH). The samples were weighed every 60 minutes. The hygroscopicity rate was calculated from the slope of moisture content on a dry basis against time (in minutes).
2.5.5. Solubility analysis
A sample of 1 g was added to 100 mL of distilled water in a beaker glass. The solution was stirred using a magnetic stirrer for 5 minutes at 40°C. The solution was then filtered using Whatmann filter paper no. 1 size of pore diameter 11 µm. 20 mL of filtrate was transferred into a petri dish, then dried in an oven at a temperature of 105 ± 2°C for 5 hours. The percentage of solubility is calculated by gravimetry.
2.5.6. DPPH scavenging activity and antioxidant stability
Antioxidant activity was determined as the ability to scavenge free radicals from the DPPH (1,1-diphenyl-2-picrylhydrazyl) reagent. A powder sample containing 300 mg was dissolved in 25 mL of methanol. After a vigorous shake, the mixture was allowed to rest at room temperature for 30 minutes. Then, ascorbic acid was used as a standard reference compound and the absorbance was measured using a spectrophotometer (Rayleigh Spectrophotometer UV VIS-9200, China) at 517 nm. The initial antioxidant activity was estimated using the log dose inhibition curve and expressed as the IC50 value, or the sample concentration required to inhibit 50% of the DPPH free radicals. The following equation is used to determine the percentage (%) of the DPPH scavenging effect:
where:
Abss is the sample concentration value, and Absb is the starting absorbance. This value of Abss should reflect the concentration of the sample solution in the cuvette (or other mixing vessels) in the absence of any DPPH and should account for the dilution caused by the addition of DPPH. When there is no sample present, such as when checking the stability of the measuring system, the Absb value is sometimes referred to as the “control” value. Additionally, the measuring process is expected to ensure a constant total DPPH concentration (Zhao et al., Citation2020).
3. Results and discussion
3.1. Characteristics of double emulsion W/O/W Liquid
3.1.1. Creaming index
Previous to the emulsion’s total coalescence, creaming is the first stage. The height of the resulting cream layer was measured against the size of the whole emulsion to determine the creaming index, which assesses the emulsion’s stability during storage (Hong et al., Citation2018) at room temperature storage (±25°C). A study conducted by Ahsan et al. (Citation2020) states that the higher the creaming index value, the lower the stability of the emulsion is, meaning that more oil that breaks and separates from the emulsion system.
In general, all samples of double emulsion Pickering vitamin C during 21 days of storage did not show any creaming or other forms of instability (). It showed that adding MCC to the vitamin C double emulsion maintained the stability of the emulsion system for up to 21 days.
Figure 1. Creaming index of double emulsion W/O/W vitamin C during storage.
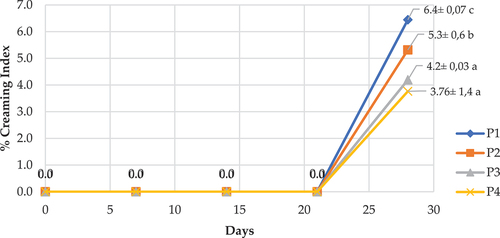
Double emulsion Pickering W/O/W Vitamin C made with MCC showed that lowering the MCC concentratiion from 2.5% to 1% (samples P1 to P4) increased the creaming index value after 21 days of storage from 3.76 ± 1.4 to 6.4 ± 0.07 (). The high value of the creaming index in the treatment sample P1= (MCC 1%) could be caused by the flocculation of the emulsion components. Molyneux (Citation2004) and Holmberg et al. (Citation2004), state that several mechanisms can cause emulsion degradation over time, including gravity separation, droplet aggregation (or flocculation), Ostwald maturation, and droplet coalescence. According to McClements (Citation2016) and Bai et al. (Citation2019), flocculation is caused by relationships between droplet groups or by the attraction between droplets, which causes the particle mass to agglomerate together while each drop keeps its original size.
The addition of MCC shows its role of MCC as solid particles that function as Pickering in multiple emulsion systems. It follows the research conducted by Xie et al. (Citation2019b) and Kargar et al. (Citation2012) that mentions that MCC is suitable for use as a Pickering emulsion because it has amphiphilic properties, wettability, high aspect ratio, irreversible adsorption, and the ability to self-assemble at the O/W interface. Conducted by Xie et al. (Citation2019b) and Leister and Karbstein (Citation2020) considering MCC as a pickering emulsion, which prevents emulsion degradation by allowing MCC (solid particles) to wet each other partially. In each liquid phase, specifically the oil and water phase (partial double wettability), the particles are adsorbed at the oil and water interface to form a mechanical barrier, reducing the tendency to flocculate, while the MCC combined with a high concentration makes the non-adsorbed particles act as the thickening agent in the aqueous phase to form a gel continuously.
3.1.2. Emulsion morphological structure with TEM
The structure of the vitamin C double emulsion morphology of the Pickering emulsion before spray drying and of the rehydrated powder obtained by spray drying was observed for samples with 2% MCC (P3) by Transmission Electron Microscope (TEM) at a magnification of 5,000–30,000×. In , the large black circle is a W1/O emulsion system containing Vitamin C and Emulsifier Span 65. The dark circle on the droplet with a white dot included in the W1/O system is probably a vitamin C solution that is still stable.
Figure 2. Morphology of the double emulsion of vitamin C in sample P3 (MCC = 2%) (a) morphology of double emulsion before drying, (b) morphology of spray-dried double emulsion after rehydration.
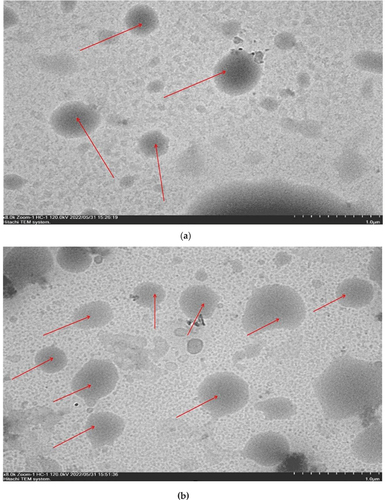
The morphological structure of the emulsion shown in is the structure of the P3 treatment emulsion with the emulsifier concentration of MCC (2%) as Pickering emulsion, and it can be seen that. Another study related to the dark areas of emulsion droplets was carried out by Leister and Karbstein (Citation2020), which suggests that stable double emulsion droplets contain several internal drops, resulting in black droplets. In the study by Shaddel et al. (Citation2018), the presence of white droplets and their movement on a microscope slide that remained stable without phase separation indicated the formation of a stable emulsion.
Most microcapsules are also circular and have a very smooth surface. Because it can directly affect the release and penetration of the active core compound from the microcapsules into the medium, its shape is fundamental. Research related to the shape of the emulsion was also carried out by Santos et al. (Citation2015), mentioning the double emulsion images found and taken using an optical microscope. The droplets on the microscope slide indicate that the emulsion has successfully formed. The following is an observation of the morphological structure of the rehydrated vitamin C double emulsion powder in .
The morphological structure of the emulsion shown in is the morphological structure of the P3 treatment emulsion powder, which went through a spray drying process and then rehydrated before observing the morphology of the emulsion with the TEM tool. The W1/O emulsion system containing vitamin C was still visible in the form of large black circles with white dots included in the W1/O system, possibly the vitamin C solution, which was still stable even though it had passed the spray drying process. It suggests that adding a combination of MCC concentrations as Pickering emulsion has a significant effect in maintaining the emulsion system.
According to a prior study, the solid particles used in the cellulose modification investigation were MCC (Costa et al., Citation2019). MCC is a naturally occurring component that is biocompatible and biodegradable. MCC also can endure high temperatures in the emulsion system, making the emulsion system safer when exposed to heat during the drying process. According to another study (Bai et al., Citation2019; Costa et al., Citation2019) MCC significantly reduces emulsion instability.
3.1.3. Rheological properties (apparent viscosity)
Apparent viscosity in emulsion systems refers to the thickness at a specific shear rate. Since apparent viscosity depends on the shear rate, determining the shear rate used to experiment is very important. In carrying out rheological studies design to replicate some naturally occurring processes in food, it is crucial to choose the appropriate shear rate to use when measuring the apparent viscosity of a nonideal liquid (McClements, Citation2015; Xie et al., Citation2019a) Based on this previous report, the apparent viscosity value of the samples in this study was determined at the same shear rate, i.e. 913–1100 mPa.s. Viscosity measurements of double emulsion liquid samples at the same shear rate (30 rpm) yielded 1100, 1070, 965 and 785 mPa.s for treatment samples P1, P2, P3 and P4, respectively.
Emulsion W/O/W treatment P1 with MCC (1%) had the highest apparent viscosity value, followed by treatments P2, P3, and P4, respectively. presents the flow behaviour index of vitamin c double emulsion. The flow behaviour index of the vitamin C double emulsion liquid of the P1, P2, P3, and P4 treatments, which is 0.4035, 0.3954, 0.3497, and 0.3059, was significantly affected by the inclusion of MCC as Pickering in the vitamin C double emulsion system. It indicates that the lower the index value and the lower the apparent viscosity value of the double emulsion vitamin C, the higher the concentration of MCC employed in the system. The lower number of n displays how far the emulsion liquid deviates from a perfect liquid system (Newtonian Fluid).
Figure 3. Value of n (flow behaviour index) of double emulsion vitamin C at various treatment.
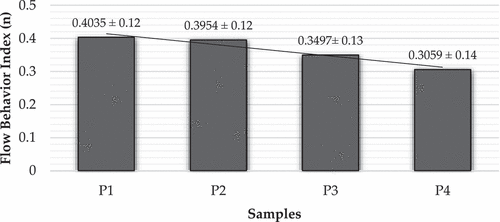
Previous studies (Imeson, Citation2010; Krawczyk et al., Citation2009), have shown the ability to adjust viscosity, gel formation, surface area, thixotropy, or water binding through the interaction of microcrystalline cellulose with other hydrocolloids and supporting process conditions, multidimensional properties of MCC components.
3.1.4. Zeta potential
The zeta potential measures the magnitude of the attractive or repulsive forces between particles. The potential presence of attractive forces helps prevent droplet coalescence, resulting in emulsion stability (Premi & Sharma, Citation2017). The magnitude of the zeta potential predicts colloidal stability. Nanoparticles with Zeta potential values less than +25 mV or more significant less than −25 mV are often unstable. Lower values indicate higher van der Waal affinity, which leads to emulsion aggregation and consequent instability (Nanocomposix, Citation2020).
W/O/W vitamin C emulsion with MCC for all treatments showed a zeta potential value that was closer to the zeta value −25 mV on day 0 and indicated that increasing the MCC concentration, the closer and larger it was from a zeta value of −25 mV (Ahsan et al., Citation2020). Related studies showed that as the MCC concentration increased, the overall charge on the curcumin emulsion droplets grew more negative. The zeta potential of the curcumin droplets decreased significantly with the lower concentration of MCC (Xu et al., Citation2016). The following shows the potential zeta value of the W/O/W double emulsion vitamin C.
Table 2. Zeta potential double emulsion Pickering W/O/W (water-in-oil-in-water) vitamin C at various storage.
The zeta value for the double emulsion of vitamin C at storage day 0, showed an increasing trend with the increasing ratio of MCC, namely P1 (−24.7 mV), P2 (−27.0 mV), P3 (−27.0 mV) and P4 (−30.0 mV). The use of MCC in vitamin C double emulsion significantly affected the measurement results based on the Zeta value. Supporting research was carried out by (Kargar et al., Citation2012; Xie et al., Citation2019b), which claimed that at the same drop in pH, the increase in zeta potential in MCC particles on both sides was much more significant. It is the primary cause of the emulsion stabilised by MCC particles’ enhanced lipid oxidation stability.
All treatments in the zeta potential measurement from storage day 15 and 30 showed a change in the zeta potential value, which was reduced from its absolute value at 28 days of storage. Supporting research was carried out by Leister and Karbstein (Leister & Karbstein, Citation2020), which presented that the use of MCC, which is larger than CMC, in the manufacture of Pickering emulsions increased the repulsion and stability of the emulsion during storage even its zeta potential having decrease trend.
3.1.5. Particle size analysis
Particle size is an important factor in the success of emulsion Pickering in multiple emulsion technologies in various emulsion technologies for use in food and other applications (Xiao et al., Citation2016). The vitamin C double emulsion particle size was tested using a Particle Size Analyzer (PSA) and the Laser Diffraction technique. displays the findings of the particle size test of the vitamin C double emulsion with MCC added as Pickering.
Table 3. The vitamin C double emulsion particle size assay with Pickering of MCC.
The particle size test results of the vitamin C double emulsion shows that the particle size value was getting smaller for the treatment with the addition of higher MCC concentrations. The average particle size of vitamin C double emulsion from treatments P1 to P4 ranged from 2.27 ± 0.46 to 0.24 ± 0.09 μm. The greater the concentration of MCC added, the lower the particle size value of the vitamin C double emulsion as in the P4 treatment (0.24 ± 0.09 μm). It shows the role of MCC as a Pickering emulsion in reducing the particle size of the emulsion formed. Studies conducted by Wei and Huang (Citation2020) suggest that high concentrations influence the formation of tiny droplets. While the study by Xia et al. (Citation2021) indicates that small droplets are more desirable in stabilizing Pickering emulsions. The higher the MCC concentration, the more particles there will be at the oil–water interface, which will help stabilize the higher interfacial area and prevent aggregation.
Other research (Winuprasith & Suphantharika, Citation2015; Xu et al., Citation2016) suggest that MCC in colloidal form can thicken the continuous phase between droplets while forming a weak three-dimensional network expected to help stabilize the emulsion. According to the study results of (Krawczyk et al., Citation2009; Xiao et al., Citation2016), due to the repulsion of negatively charged cellulose crystals, well-hydrated MCC solids can form a three-dimensional network. Research conducted by Yaginuma and Kijima (Citation2006) has found that this network structure helps prevent insoluble particles from settling in the cocoa drink. In their study (Xu et al., Citation2016), the three-dimensional network prevented the incorporation of droplets in the curcumin emulsion in their research.
3.2. Characteristics of stability and antioxidant activity of double emulsion W/O/W powder
3.2.1. Scanning electronic microscopy (SEM)
The microcapsules structure of double emulsion powder containing modified spray drying vitamin C with different concentrations of MCC was evaluated by (Scanning Electron Microscopy) (Mlalila et al., Citation2014). The morphology of the double emulsion powder microcapsules is in .
presents a photo of the surface morphology of the microcapsule powder obtained using SEM for each treatment P1, P2, P3, and P4. SEM observations verified that most of the microcapsules obtained are spherical but varied in size. In this test, the microcapsules had a smooth surface with a slight indentation, as in the P1 and P2 treatments. Furthermore, the powder consisting of the WPC/MCC complex in treatments P3 and P4 indicates that the higher concentration of MCC as a Pickering agent of the W/O/W emulsion simultaneously and sequentially result in a smoother surface with fewer surface dents and indentations than P1 and P2. In a previous study regarding the presence of MCC in the emulsion for structure, as observed by SEM, for (Ahsan et al., Citation2020) microcapsules produced with (methylated microcrystalline cellulose) (Mmcc). The absence of cracks and the lack of disintegration of the droplets emulsion system indicated that Mmcc and soybean oil have a synergistic relationship (Nasatto et al., Citation2015). The synergistic relationship of Mmcc and oil cause Mmcc to be adsorbed onto the surface of oil droplets and form a different barrier shell. Furthermore, the adsorbed cellulose layer (interface stabilization increases with increasing molecular weight) can strengthen the shell to prevent emulsion droplets from being disturbed.
3.2.2. Particle size analysis of double emulsion powder
The particle size test of the vitamin C double emulsion powder was carried out after the rehydration process with distilled water using the Particle Size Analyzer (PSA) with the Laser Diffraction method. The test results for the particle size of the vitamin C double emulsion with the addition of MCC as Pickering after drying can be seen in . The particle size of vitamin C double emulsion after rehydration showed values greater than 1 μm. The size value is significantly bigger and different from its originally vitamin C double emulsion (). The spray drying process seemed to be the cause for the particle size increase.
Table 4. Particle size of spray dried vitamin C double emulsion after rehydration.
The particle size of the vitamin C double emulsion after drying was modified by adding MCC concentrations, as shown in . In general, the average particle size of the powder double emulsion gradually decreasing. The higher the concentration of MCC, the smaller the vitamin C double emulsion particle size after drying. On the other hand, variations in particle size differences can be caused by the combination of emulsifiers, namely WPC and MCC, in the vitamin C double emulsion system. According to (Lamba et al., Citation2015), the processing conditions, formulation, and type of emulsifier used all impact the particle size in multiple emulsions (Ahsan et al., Citation2020; Krawczyk et al., Citation2009; Yegya Raman & Aichele, Citation2020). have also confirmed that other solid particles (MCC) will bind to all potential oil-water surfaces, thereby reducing coalescence dramatically. The emulsion droplets produced by the stabilization mechanism of this mixed emulsifying system are smaller than those produced by surfactants or solid particles alone.
The Polydispersity Index (PDI) describes the distribution of particles of various sizes in the emulsion system (Clayton et al., Citation2016; Vicente et al., Citation2018). A PDI value close to 0 means that the emulsion has a uniform distribution (Xu et al., Citation2011). Stable emulsions with PDI values less than 0.3 are called monodispersions, whereas PDI values greater than 0.3 indicate a polydispersity behaviour reflecting an unstable emulsion (Das & Chaudhury, Citation2011; Floury et al., Citation2000; Toledo Hijo et al., Citation2015). As observed in , all vitamin C double emulsion systems after spray drying had PDI > 0.30, which means they have heterogeneous particle size distribution or show high polydispersion. However, the PDI value tends to decrease when the MCC concentration increases. It can occur due to the interaction of emulsifiers or surfactants such as WPC and MCC, which were used in manufacturing the vitamin C double emulsion system (Floury et al., Citation2000)
Research by Toledo Hijo et al. (Citation2015) suggests that higher concentrations of WPC and polysaccharides correlate with higher PDI. This is most likely due to fluid motion and polymer–polymer interactions. As the viscosity of the continuous phase increases, so does the minimum shear force required to separate the particles. The input energy is insufficient to cause droplet loss under these conditions, resulting in a higher coarse droplet and broader dispersion. Other studies (Anandharamakrishnan & Ishwarya, Citation2015; Campelo et al., Citation2017) have concluded that spray drying can produce a larger PDI because the spray drying approach tends to create various droplet sizes. An increase in droplet size after drying can also be formed compared to before drying takes place. It occurs because part of the emulsion breaks when it passes through the nozzle during the spray drying process, increasing the size of the particle droplets that form after going through the process. According to research by Winuprasith and Suphantharika (Citation2015) & Xu et al. (Citation2016), MCC can thicken the continuous phase among droplets while also building a three-dimensional network in colloidal form. Particle aggregation soon after drying may result from this. Previous research (Dhar et al., Citation2012) describes that the adhesion (attractive force) and collision force between the particles in the spray drying chamber result in agglomeration.
3.2.3. Water activity
Water activity (aw) is an essential variable for the safety and stability of food powders because it affects chemical properties and determines shelf life. Since the reaction rate decreases for aw < 0.3, the food product is considered stable (Do & Nguyen, Citation2018). Aw values and dry basis water content values of microcapsules are summarized in .
Table 5. aw and moisture content of spray dried vitamin C double emulsion.
illustrates that the aw and water content of the double emulsion of vitamin C drying with WPC and MCC showed lower aw values and water content when higher MCC concentrations were used in the P4 treatment. Previous work by Grishkewich et al. (Citation2017) showed that a decrease in gum arabic content from 9% to 7% and an increase in MCC concentration from 0% to 2% resulting in a significant reduction in the water content (p < .05) of the final product, with values decreasing from 4.54% to 3.20%. The presence of MCC can cause changes in particle configuration, which can explain the decrease in water content. MCC added to the formulation resulted in a finer powder with a semi-crystalline structure and almost no spacing between powder particles.
The water content and water activity (aw) of the vitamin C double emulsion, after spray drying, yielded aw values between 0.29 ± 0.002 to 0.19 ± 0.001 and dry basis water content values between 1.86 ± 0.07 to 1.58 ± 0.4, respectively. Although powders with aw below 0.6 are microbiologically stable. At water activity levels close to 0.3, lipid oxidation is challenging to achieve, lipid oxidation is enhanced by aw levels below 0.2 and above 0.5. Although oil/water emulsions are challenging to distribute because they are physically unstable due to oil breakdown and oxidation, and also susceptible to microbiological growth, converting liquid emulsions into dry emulsified powders by spray drying is one of the effective techniques to overcome these drawbacks. The spray drying of liquid emulsion produces stable powders which can be rehydrated to return to the original emulsion (Barbosa-Cánovas et al., Citation2005)
3.2.4. Hygroscopicity rate
The presence of water can affect powder flowability and contribute to lipid oxidation. Hence, hygroscopicity is a significant quality characteristic of encapsulated oils (Habibi et al., Citation2010). In a previous study (Premi & Sharma, Citation2017), greater hygroscopicity was primarily observed in powder products resulting from spray drying. The frequency at which a material picks up moisture from the environment in grams per hour is known as the hygroscopicity rate. The results of the observation of the hygroscopicity rate can be seen in .
Figure 5. Hygroscopicity rate of spray dried vitamin C double emulsion.
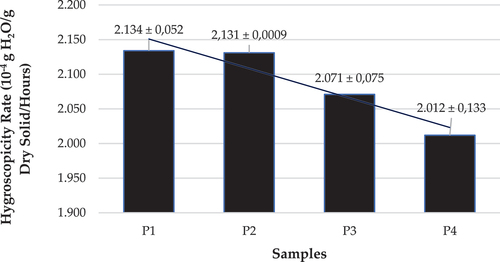
The hygroscopicity rate data presented in showed that the higher the given concentration of MCC or cellulose, the lower the hygroscopicity rate of the double emulsion vitamin C powder obtained by spray drying. A related study by Yousefi et al. (Citation2015) found that cellulose contains crystalline and amorphous regions, the sources of which can be separated using chemical or mechanical processes to make various crystalline cellulose products but depending on the processing method and source, such as microcrystalline cellulose (MCC), nanocrystalline cellulose (NCC), microfibrillated cellulose (MFC), nanofibrillated cellulose (NFC), or bacterial nanocrystalline cellulose (BNC). On the other hand, the drying process forms a crystal structure in the vitamin C double emulsion research conducted by Azubuike and Okhamafe (Citation2012) and Grishkewich et al. (Citation2017), suggests that the spray-dried powder containing cellulose has a higher crystal line region content. This observation is supported by research Ghorab et al. (Citation2014) who proposed that low crystallinity indicates a more irregular structure, therefore, correlates with amorphous powders, while high crystallinity implies an organized solid molecular structure, which corresponds to solid particles. Meanwhile, another research by Kanha et al. (Citation2021) has concluded that amorphous particles are more hygroscopic than crystalline because they absorb water when stored at low aw.
3.2.5. Solubility analysis
Solubility is another significant quality parameter of powdered products and can directly impact the reconstitution activity of spray-dried powders. According to Ghorab et al. (Citation2014), the percentage solubility describes the relationship between dry solid and powder mass. The results of the solubility observations can be seen in .
Figure 6. Percentage solubility of spray dried vitamin C double emulsion.
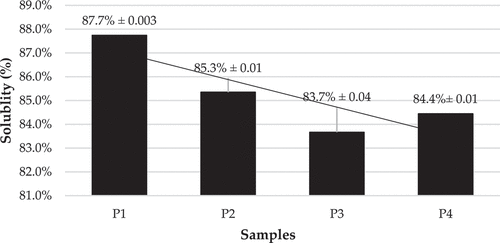
shows the solubility data of the vitamin C double emulsion after spray drying in treatment P1 with a concentration of MCC (1%) higher than the other treatments of P2, P3, and P4. Thus, it can be concluded that the higher the concentration of MCC given, the lower the percentage of the solubility of the vitamin C double emulsion sample as a result of spray drying. The crystalline region of MCC in the dried vitamin C double emulsion system affects the solubility of the formed vitamin C double emulsion powder. Related studies by Shokri and Adibki (Citation2013) and Ghorab et al. (Citation2014) suggest that the degree of crystallization, which distinguishes MCC from its cellulose source, is critical as it affects various parameters, including the stabilizing ability of suspensions and emulsions, thermal properties, and hydration capacity of MCC particles. It also affects the flow capacity of crystalline cellulose when used as an additive or ingredient in drug or food applications, the solubility of pomegranate juice powder, and the glass transition temperature (Tg). Research conducted by Azubuike and Okhamafe (Citation2012) explains how the addition of MCC substantially impacts solubility and Tg. Due to the MCC structured matrix of solid linear glucose polymer linked by intra- and intermolecular hydrogen bonds, crystalline cellulose affects the insoluble nature of the spray-dried pomegranate juice powder and dramatically reduces the solubility of all samples. Research related to the use of MCC in spray drying products was also carried out by Grishkewich et al. (Citation2017) found that the increase in the addition of MCC and the reduction of gum arabic was shown to have a substantial decrease in water content, bulk density, solubility in water, and antioxidant characteristics of the juice powder mulberry when dried by spray drying at a constant input air temperature and feed flow rate.
3.2.6. DPPH scavenging activity and antioxidant stability
Antioxidant activity test was carried out on double emulsion powder W/O/W vitamin C after spray drying for 56 days storage at room temperature. The data in shows that adding MCC concentration in the spray drying double emulsion vitamin C system gave a low IC50 result. The higher the concentration of MCC used, the lower the IC50 value for each treatment after drying. On storage day 0, the IC50 values for treatments P1, P2, P3, and P4 were as follows: 167.1; 166.2; 160.1 and 163.3. The IC50 value of all treatments showed a decreasing trend as the concentration of MCC increased. The IC50 value of double emulsion vitamin C after spray drying, which was stored for 56 days, can be seen in .
Figure 7. IC50 value of spray dried vitamin C double emulsion during storage.
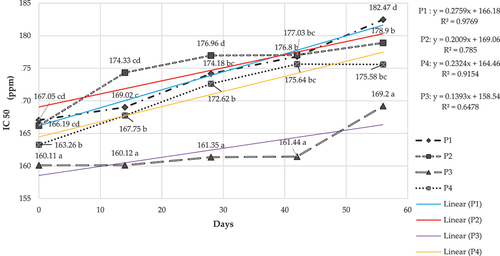
indicates that the antioxidant capacity was further decreased when the storage of the vitamin C powder emulsion was continued for up to 56 days. However, based on the measurement results of antioxidant capacity, the antioxidant value in treatments P1, P2, P3, and P4 experienced stability on days 28 and 42. However, there was a tendency to decrease when entering the 56th day of storage. Adding Pickering emulsion in the form of MCC in a double emulsion system of W/O/W vitamin C passed through spray drying showed differences in antioxidant activity and stability measured during 56 days of storage. The results of these measurements indicate the ability of the MCC in the dual emulsion system to maintain the active substance, in this case, the antioxidant vitamin C. Based on , it can be seen that the increasing concentration of MCC in the vitamin C double emulsion in treatments P1, P2, P3, and P4 showed its good ability to retain the active components in the emulsion system and also helps in the stability of the active components during storage up to day 56. Based on the rate of change of the antioxidant stability of the powder emulsion during storage, the slope value tends to decrease from the highest value in the P1 treatment (0.2759 ppm/day) and gets lower to the P2 (0.2009 ppm/day), P3 (0.1393 ppm/day) and P4 (0.2324 ppm/day) treatments. The lower the slope value, the better the emulsion system is made and can maintain changes in antioxidant stability as the active ingredient whose value is maintained. The slope value in the treatment P3 (0.1393), as the lowest, indicates the optimal concentration of MCC in the emulsion system that is made to withstand the rate of change in antioxidant stability. This was also backed up by the result of particle size and zeta potential of treatment P3. Particle size of the emulsion P3 was small and its zeta potential did not change much during storage. In addition, there were other studies in our group which previously reported very high instability of vitamin C in bulk form (not encapsulated) during storage and we used this as a reference as the basis for increasing the IC50 of the control (Vitamin C in unencapsulate form) which was around 6.03 ppm/day while in our results the increase in IC50 value was in the range of 0.1393 to 0.2759 ppm/day (Nurhadi et al., Citation2023). These data indicate that the double emulsion method can improve the stability of vitamin C during storage. Whereas Vitamin C control in previous studies (reference) found that the antioxidant capacity of unencapuslated Vitamin C changed very quickly.
Previous research on the ability of MCC in an emulsion system (Kargar et al., Citation2012) found that MCC particles are also proven to reduce the rate of lipid oxidation more effectively than modified starch (MS) particles. It is due to the ability of MCC to scavenge radicals and produce a more extensive interfacial layer around the oil droplets, which is affected by differences in particle size. The current study found that modifying the emulsion interfacial microstructure (Pickering emulsion), based on developing a thick interface around the oil droplets by organic matter particles, is an efficient way to slow down lipid oxidation. Another supporting study was conducted (Shokri & Adibki, Citation2013) to claim that in addition to probiotics, other food bioactive components, essential oils, and volatile compounds can be encapsulated to preserve them from degradation and evaporation. MCC produces coatings to protect important bioactive food components from moisture, oxygen, light, and temperature according to the effect of the coating.
4. Conclusion
The technology for making vitamin C double emulsion in P3 treatment with a ratio of MCC (2%), which was dried by spray drying, is the best treatment to produce the double emulsion powder. It is seen from its lowest Antioxidant Activity (IC50) value and the lowest rate of change in antioxidant stability during 56 days of storage, and the formation of the morphological structure of W/O/W double emulsion using TEM. The vitamin C double emulsion with 2% MCC had particle size 0.32 µm (3.52 µm after rehydration spray dried double emulsion), IC50 of dried double emulsion 160.11 ppm and the rate of IC50 changes 0.1393 ppm/days. In conclusion the addition of MCC despite of some limitation might stabilize the antioxidant capacity of vitamin C in the form W/O/W double emulsion.
Disclosure statement
No potential conflict of interest was reported by the author(s).
References
- Ahsan, H. M., Pei, Y., Luo, X., Wang, Y., Li, Y., Li, B., & Liu, S. (2020). Novel stable pickering emulsion based solid foams efficiently stabilized by microcrystalline cellulose/chitosan complex particles. Food Hydrocolloids, 108(100), 106044. https://doi.org/10.1016/j.foodhyd.2020.106044
- Ahsan, H. M., Zhang, X., Liu, Y., Wang, Y., Li, Y., Li, B., Wang, J., & Liu, S. (2020). Stable cellular foams and oil powders derived from methylated microcrystalline cellulose stabilized pickering emulsions. Food Hydrocolloids, 104(January), 105742. https://doi.org/10.1016/j.foodhyd.2020.105742
- Akhavan Mahdavi, S., Jafari, S. M., Assadpoor, E., & Dehnad, D. (2016). Microencapsulation optimization of natural anthocyanins with maltodextrin, gum Arabic and gelatin. International Journal of Biological Macromolecules, 85, 379–385. https://doi.org/10.1016/j.ijbiomac.2016.01.011
- Anandharamakrishnan, C., & Ishwarya, S. P. (2015). Introduction to spray drying. Spray Drying Techniques for Food Ingredient Encapsulation, 1–36. https://doi.org/10.1002/9781118863985.ch1
- Azubuike, C. P., & Okhamafe, A. O. (2012). Physicochemical, spectroscopic and thermal properties of microcrystalline cellulose derived from corn cobs. Journal of Recycling of Organic Waste in Agriculture, 1(9), 1–7. https://doi.org/10.1186/2251-7715-1-9
- Bai, L., Greca, L. G., Xiang, W., Lehtonen, J., Huan, S., Nugroho, R. W. N., Tardy, B. L., & Rojas, O. J. (2019). Adsorption and assembly of cellulosic and lignin Colloids at oil/waterinterfaces. Langmuir, 35(3), 571–588. https://doi.org/10.1021/acs.langmuir.8b01288
- Bai, L., Huan, S., Xiang, W., & Rojas, O. J. (2018). Pickering emulsions by combining cellulose nanofibrils and nanocrystals: Phase behavior and depletion stabilization. Green Chemistry, 20(7), 1571–1582. https://doi.org/10.1039/c8gc00134k
- Barbosa-Cánovas, G. V., Ortega-Rivas, E., Juliano, P., & Yan, H. (2005). Food powders physical properties, processing, and functionality. https://doi.org/10.1007/0-387-27613-0
- Benichou, A., Aserin, A., & Garti, N. (2004). Double emulsions stabilized with hybrids of natural polymers for entrapment and slow release of active matters. Advances in Colloid and Interface Science, 108–109, 29–41. https://doi.org/10.1016/j.cis.2003.10.013
- Berendsen, R., Güell, M., & Ferrando, C. (2015). Spray dried double emulsions containing procyanidin-rich extracts produced by premix membrane emulsification: Effect of interfacial composition. Food Chem, 178, 251–258. https://doi.org/10.1016/j.foodchem.2015.01.093
- Campelo, P. H., Junqueira, L. A., Resende, J. V. D., Zacarias, R. D., Fernandes, R. V. D. B., Botrel, D. A., & Borges, S. V. (2017). Stability of lime essential oil emulsion prepared using biopolymers and ultrasound treatment. International Journal of Food Properties, 20(March), S564–S579. https://doi.org/10.1080/10942912.2017.1303707
- Clayton, K. N., Salameh, J. W., Wereley, S. T., & Kinzer-Ursem, T. L. (2016). Physical characterization of nanoparticle size and surface modification using particle scattering diffusometry. Biomicrofluidics, 10(5). https://doi.org/10.1063/1.4962992
- Comunian, T. A., Thomazini, M., Alves, A. J. G., de Matos Junior, F. E., de Carvalho Balieiro, J. C., & Favaro-Trindade, C. S. (2013). Microencapsulation of ascorbic acid by complex coacervation: Protection and controlled release. Food Research International, 52(1), 373–379. https://doi.org/10.1016/j.foodres.2013.03.028
- Costa, C., Medronho, B., Filipe, A., Mira, I., Lindman, B., Edlund, H., & Norgren, M. (2019). Emulsion formation and stabilization by biomolecules: The leading role of cellulose. Polymers (Basel), 11(10). https://doi.org/10.3390/polym11101570.
- Das, S., & Chaudhury, A. (2011). Recent advances in lipid nanoparticle formulations with solid matrix for oral drug delivery. AAPS PharmScitech, 12(1), 62–76. https://doi.org/10.1208/s12249-010-9563-0
- Dhar, N., Akhlaghi, S. P., & Tam, K. C. (2012). Biodegradable and biocompatible polyampholyte microgels derived from chitosan, carboxymethyl cellulose and modified methyl cellulose. Carbohydrate Polymers, 87(1), 101–109. https://doi.org/10.1016/j.carbpol.2011.07.022
- Do, H. T. T., & Nguyen, H. V. H. (2018). Effects of spray-drying temperatures and ratios of gum arabic to microcrystalline cellulose on antioxidant and physical properties of mulberry juice powder. Beverages, 4(4), 101. https://doi.org/10.3390/beverages4040101
- Fang, S., Zhao, X., Liu, Y., Liang, X., & Yang, Y. (2019). Fabricating multilayer emulsions by using OSA starch and chitosan suitable for spray drying: Application in the encapsulation of β-carotene. Food Hydrocolloids, 93(May 2018), 102–110. https://doi.org/10.1016/j.foodhyd.2019.02.024
- Floury, J., Desrumaux, A., & Lardières, J. (2000). Effect of high-pressure homogenization on droplet size distributions and rheological properties of model oil-in-water emulsions. Innovative Food Science and Emerging Technologies, 1(2), 127–134. https://doi.org/10.1016/S1466-8564(00)00012-6
- Ghorab, M. K., Toth, S. J., Simpson, G. J., Mauer, L. J., & Taylor, L. S. (2014). Water-solid interactions in amorphous maltodextrin-crystalline sucrose binary mixtures. Pharmaceutical Development and Technology, 19(2), 247–256. https://doi.org/10.3109/10837450.2013.775157
- Grishkewich, N., Mohammed, N., Tang, J., & Tam, K. C. (2017). Recent advances in the application of cellulose nanocrystals. Current Opinion in Colloid & Interface Science, 29, 32–45. https://doi.org/10.1016/j.cocis.2017.01.005
- Gulcin, İ. (2020). Antioxidants and antioxidant methods: An updated overview. 94(3), 651–715. https://doi.org/10.1007/s00204-020-02689-3
- Habibi, Y., Lucia, L. A., & Rojas, O. J. (2010). Cellulose nanocrystals: Chemistry, self-assembly, and applications. Chemical Reviews, 110(6), 3479–3500. https://doi.org/10.1021/cr900339w
- Hino, T., Shimabayashi, S., Tanaka, M., Nakano, M., & Okochi, H. (2001). Improvement of encapsulation efficiency of water-in-oil-in-water emulsion with hypertonic inner aqueous phase. Journal of Microencapsulation, 18(1), 19–28. https://doi.org/10.1080/026520401750038575
- Holmberg, K., Jönsson, B., Kronberg, B., & Lindman, B. (2004). Emulsions and emulsifiers (2nd ed.). John Wiley & Sons Ltd. https://doi.org/10.1002/0470856424.ch2
- Hong, I. K., Kim, S. I., & Lee, S. B. (2018). Effects of HLB value on oil-in-water emulsions: Droplet size, rheological behavior, zeta-potential, and creaming index. Journal of Industrial and Engineering Chemistry, 67, 123–131. https://doi.org/10.1016/j.jiec.2018.06.022
- Imeson, A. (2010). Food stabilisers, thickeners and gelling agents (1st ed.). Blackwell Publishing. https://doi.org/10.1002/9781444314724
- Kanha, N., Regenstein, J. M., Surawang, S., Pitchakarn, P., & Laokuldilok, T. (2021). Properties and kinetics of the in vitro release of anthocyanin-rich microcapsules produced through spray and freeze-drying complex coacervated double emulsions. Food Chemistry, 340(August 2020), 127950. https://doi.org/10.1016/j.foodchem.2020.127950
- Kargar, M., Fayazmanesh, K., Alavi, M., Spyropoulos, F., & Norton, I. T. (2012). Investigation into the potential ability of Pickering emulsions (food-grade particles) to enhance the oxidative stability of oil-in-water emulsions. Journal of Colloid and Interface Science, 366(1), 209–215. https://doi.org/10.1016/j.jcis.2011.09.073
- Krawczyk, G. R., Venables, A., & Tuason, D. (2009). Microcrystalline cellulose, no. Mcc. Woodhead Publishing Limited. https://doi.org/10.1533/9781845695873.740
- Lamba, H., Sathish, K., & Sabikhi, L. (2015). Double emulsions: Emerging delivery system for Plant Bioactives. Food and Bioprocess Technology, 8(4), 709–728. https://doi.org/10.1007/s11947-014-1468-6
- Leister, N., & Karbstein, H. P. (2020). Evaluating the stability of double emulsions— A review of the measurement techniques for the systematic investigation of instability mechanisms. Colloids and Interfaces, 4(1), 1–18. https://doi.org/10.3390/colloids4010008
- McClements, D. J. (2015). Encapsulation, protection, and release of hydrophilic active components: Potential and limitations of colloidal delivery systems. Advances in Colloid and Interface Science, 219, 27–53. https://doi.org/10.1016/j.cis.2015.02.002
- McClements, D. J. (2016). Food emulsions (3rd ed.). CRC Press: https://doi.org/10.1201/b18868
- Mlalila, N., Swai, H., Kalombo, L., & Hilonga, A. (2014). Effects of spray-drying on w/o/w multiple emulsions prepared from a stearic acid matrix. Nanotechnology, Science and Applications, 4(7), 105–112. https://doi.org/10.2147/nsa.s72083
- Molyneux, P. (2004). The use of the stable free radical diphenylpicryl-hydrazyl (DPPH) for estimating antioxidant activity. Songklanakarin Journal of Science and Technology, 26(December 2003), 211–219. https://doi.org/10.1287/isre.6.2.144
- Nanocomposix. (2020). Guidelines for zeta zeta potential analysis of Nanoparticles. NANOCOMPOSIX.COM. Retrieved April 24, 2022, from https://cdn.shopify.com/s/files/1/0257/8237/files/nanoComposix_Guidelines_for_Zeta_Potential_Analysis_of_Nanoparticles.pdf?13692.
- Nasatto, P. L., Pignon, F., Silveira, J. L. M., Duarte, M. E. R., Noseda, M. D., & Rinaudo, M. (2015). Methylcellulose, a cellulose derivative with original physical properties and extended applications. Polymers (Basel), 7(5), 777–803. https://doi.org/10.3390/polym7050777
- Nurhadi, B., Sulaeman, M. Y., & Mahani. (2023). Antioxidant stability of vitamin C in double Pickering emulsion W/O/W with microcrystalline cellulose. International Journal of Food Properties, 26(1), 567–580. https://doi.org/10.1080/10942912.2023.2173228
- Parhizkar, E., Rashedinia, M., Karimi, M., & Alipour, S. (2018). Design and development of vitamin C-encapsulated proliposome with improved in-vitro and ex-vivo antioxidant efficacy. Journal of Microencapsulation, 35(3), 301–311. https://doi.org/10.1080/02652048.2018.1477845
- Premi, M., & Sharma, H. K. (2017). Effect of different combinations of maltodextrin, gum arabic and whey protein concentrate on the encapsulation behavior and oxidative stability of spray dried drumstick (Moringa oleifera) oil. International Journal of Biological Macromolecules, 105, 1232–1240. https://doi.org/10.1016/j.ijbiomac.2017.07.160
- Santos, M. G., Bozza, F. T., Thomazini, M., & Favaro-Trindade, C. S. (2015). Microencapsulation of xylitol by double emulsion followed by complex coacervation. Food Chemistry, 171, 32–39. https://doi.org/10.1016/j.foodchem.2014.08.093
- Sapei, L., Naqvi, M. A., & Rousseau, D. (2012). Stability and release properties of double emulsions for food applications. Food Hydrocolloids, 27(2), 316–323. https://doi.org/10.1016/j.foodhyd.2011.10.008
- Schilder, W. H., Tanumihardja, E., Leferink, A. M., van den Berg, A., & Olthuis, W. (2020). Determining the antioxidant properties of various beverages using staircase voltammetry. Heliyon, 6(6), e04210. https://doi.org/10.1016/j.heliyon.2020.e04210
- Schuh, V., Allard, K., Herrmann, K., Gibis, M., Kohlus, R., & Weiss, J. (2013). Impact of carboxymethyl cellulose (CMC) and microcrystalline cellulose (MCC) on functional characteristics of emulsified sausages. Meat Science, 93(2), 240–247. https://doi.org/10.1016/j.meatsci.2012.08.025
- Shaddel, R., Hesari, J., Azadmard-Damirchi, S., Hamishehkar, H., Fathi-Achachlouei, B., & Huang, Q. (2018). Double emulsion followed by complex coacervation as a promising method for protection of black raspberry anthocyanins. Food Hydrocolloids, 77, 803–816. https://doi.org/10.1016/j.foodhyd.2017.11.024
- Shokri, J., & Adibki, K. (2013). Application of cellulose and cellulose derivatives in Pharmaceutical Industries. Cellulose - Medical, Pharmaceutical and Electronic Applications. https://doi.org/10.5772/55178
- Snoussi, A., Chouaibi, M., Bouzouita, N., & Hamdi, S. (2020). Microencapsulation of catechin using water-in-oil-in-water (W1/O/W2) double emulsions: Study of release kinetics, rheological, and thermodynamic properties. Journal of Molecular Liquids, 311, 113304. https://doi.org/10.1016/j.molliq.2020.113304
- Spyropoulos, F., Duffus, L. J., Smith, P., & Norton, I. T. (2019). Impact of Pickering Intervention on the stability of W1/O/W2 double emulsions of relevance to foods. Langmuir, No CMCC, 35(47), 15137–15150. https://doi.org/10.1021/acs.langmuir.9b01995
- Stamford, N. P. J. (2012). Stability, transdermal penetration, and cutaneous effects of ascorbic acid and its derivatives. Journal of Cosmetic Dermatology, 11(4), 310–317. https://doi.org/10.1111/jocd.12006
- Su, J., Flanagan, J., Hemar, Y., & Singh, H. (2006). Synergistic effects of polyglycerol ester of polyricinoleic acid and sodium caseinate on the stabilisation of water-oil-water emulsions. Food Hydrocolloids, 20(2–3) SPEC. ISS, 261–268. https://doi.org/10.1016/j.foodhyd.2004.03.010.
- Toledo, R. T. (2007). Fundamentals of food process engineering. (3rd ed.).
- Toledo Hijo, A. A. C., Da Costa, J. M. G., Silva, E. K., Azevedo, V. M., Yoshida, M. I., & Borges, S. V. (2015). Physical and thermal properties of oregano (Origanum vulgare L.) essential oil microparticles. Journal of Food Process Engineering, 38(1), 1–10. https://doi.org/10.1111/jfpe.12120
- Vicente, J., Pereira, L. J. B., Bastos, L. P. H., de Carvalho, M. G., & Garcia-Rojas, E. E. (2018). Effect of xanthan gum or pectin addition on Sacha Inchi oil-in-water emulsions stabilized by ovalbumin or tween 80: Droplet size distribution, rheological behavior and stability. International Journal of Biological Macromolecules, 120, 339–345. https://doi.org/10.1016/j.ijbiomac.2018.08.041
- Wang, F., Liu, N., Li, K., Ma, T., Ren, F., & Luo, J. (2019). Effects of enzyme-modified soybean beverage on the composition, yield, functionality and microstructure of Cheddar cheese-like products. Lwt, 116(August), 108498. https://doi.org/10.1016/j.lwt.2019.108498
- Wei, Z., & Huang, Q. (2020). Development of high internal phase Pickering emulsions stabilised by ovotransferrin–gum arabic particles as curcumin delivery vehicles. International Journal of Food Science & Technology, 55(5), 1891–1899. https://doi.org/10.1111/ijfs.14340
- Winuprasith, T., & Suphantharika, M. (2015). Properties and stability of oil-in-water emulsions stabilized by microfibrillated cellulose from mangosteen rind. Food Hydrocolloids, 43, 690–699. https://doi.org/10.1016/j.foodhyd.2014.07.027
- Xiao, J., Li, Y., & Huang, Q. (2016). Recent advances on food-grade particles stabilized Pickering emulsions: Fabrication, characterization and research trends. Trends Food Science Technology, 55, 48–60. https://doi.org/10.1016/j.tifs.2016.05.010
- Xia, T., Xue, C., & Wei, Z. (2021). Physicochemical characteristics, applications and research trends of edible Pickering emulsions. Trends Food Science Technology, 107(November 2020), 1–15. https://doi.org/10.1016/j.tifs.2020.11.019
- Xie, J., Luo, Y., Chen, Y., Liu, Y., Ma, Y., Zheng, Q., Yue, P., & Yang, M. (2019a). Redispersible Pickering emulsion powder stabilized by nanocrystalline cellulose combining with cellulosic derivatives. Carbohydrate Polymers, 213(November 2018), 128–137. https://doi.org/10.1016/j.carbpol.2019.02.064.
- Xie, J., Luo, Y., Chen, Y., Liu, Y., Ma, Y., Zheng, Q., Yue, P., & Yang, M. (2019b). Redispersible Pickering emulsion powder stabilized by nanocrystalline cellulose combining with cellulosic derivatives. Carbohydrate Polymers, 213, 128–137. https://doi.org/10.1016/j.carbpol.2019.02.064
- Xu, D., Yuan, F., Wang, X., Li, X., Hou, Z., & Gao, Y. (2011). The effect of whey protein isolate-dextran conjugates on the freeze-thaw stability of oil-in-water emulsion. Journal of Dispersion Science and Technology, 32(1), 77–83. https://doi.org/10.1080/01932690903546785
- Xu, D., Zhang, J., Cao, Y., Wang, J., & Xiao, J. (2016). Influence of microcrystalline cellulose on the microrheological property and freeze-thaw stability of soybean protein hydrolysate stabilized curcumin emulsion. LWT - Food Science and Technology, 66, 590–597. https://doi.org/10.1016/j.lwt.2015.11.002
- Yaginuma, Y., & Kijima, T. (2006). Effects of microcrystalline cellulose on suspension stability of cocoa beverage. Journal of Dispersion Science and Technology, 27(7), 941–948. https://doi.org/10.1080/01932690600766306
- Yegya Raman, A. K., & Aichele, C. P. (2020). Influence of non-ionic surfactant addition on the stability and rheology of particle-stabilized emulsions. Colloids and Surfaces A, Physicochemical and Engineering Aspects, 585, 124084. https://doi.org/10.1016/j.colsurfa.2019.124084
- Yousefi, S., Emam-Djomeh, Z., Mousavi, M., Kobarfard, F., & Zbicinski, I. (2015). Developing spray-dried powders containing anthocyanins of black raspberry juice encapsulated based on fenugreek gum. Advanced Powder Technology, 26(2), 462–469. https://doi.org/10.1016/j.apt.2014.11.019
- Zhang, W., Huang, Q., Yang, R., Zhao, W., & Hua, X. (2021). 2-O-D-glucopyranosyl-L-ascorbic acid: Properties, production, and potential application as a substitute for L-ascorbic acid. Journal of Functional Foods, 82, 104481. https://doi.org/10.1016/j.jff.2021.104481
- Zhao, M., Huang, X., Zhang, H., Zhang, Y., Gänzle, M., Yang, N., Nishinari, K., & Fang, Y. (2020). Probiotic encapsulation in water-in-water emulsion via heteroprotein complex coacervation of type-A gelatin/sodium caseinate. Food Hydrocolloids, 105(July 2019), 105790. https://doi.org/10.1016/j.foodhyd.2020.105790